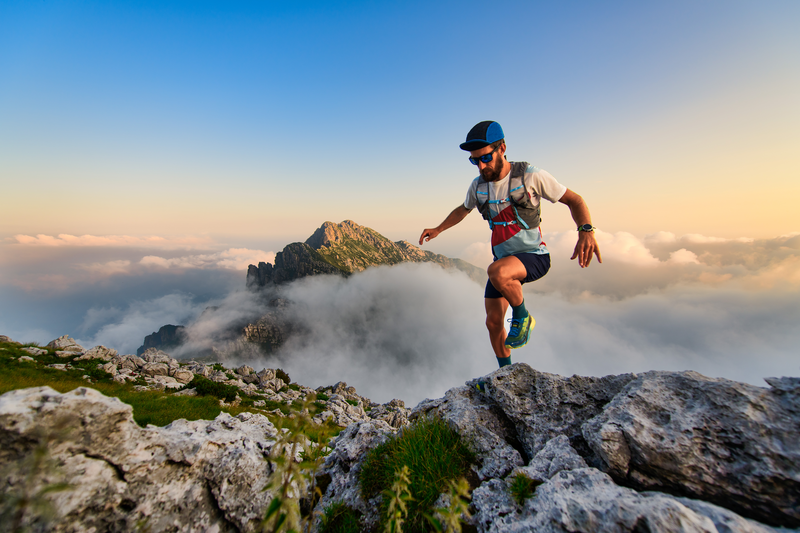
95% of researchers rate our articles as excellent or good
Learn more about the work of our research integrity team to safeguard the quality of each article we publish.
Find out more
REVIEW article
Front. Physiol.
Sec. Renal Physiology and Pathophysiology
Volume 16 - 2025 | doi: 10.3389/fphys.2025.1516466
The final, formatted version of the article will be published soon.
You have multiple emails registered with Frontiers:
Please enter your email address:
If you already have an account, please login
You don't have a Frontiers account ? You can register here
Background: Diabetic nephropathy (DN), a leading cause of end-stage renal disease, exerts a substantial burden on healthcare systems globally. Emerging evidence highlights ferroptosis -an iron-dependent form of cell death driven by lipid peroxidation and glutathione depletion -as a critical contributor to DN progression via oxidative stress, tubular injury, and glomerular dysfunction. Despite increasing research interest, a comprehensive synthesis of research trends and mechanistic insights is lacking. Objective: This study integrated bibliometric analysis with a mechanistic review to map the evolving ferroptosis landscape in DN, identify research hotspots, and propose future directions for therapeutic development. Methods: In total, 86 publications (2018-2023) were retrieved from the Web of Science Core Collection and analyzed using CiteSpace and VOSviewer. Co-occurrence networks, citation trends, and keyword bursts were examined to delineate global contributions, collaborative networks, and emerging themes. Results: Annual publication numbers surged 12-fold after 2020, with China contributing the highest proportion (60.4%), and led by institutions such as Zhengzhou University. The United States of America and Germany showed high centrality in collaborative networks. Key research themes included glutathione peroxidase 4 (GPX4)-mediated antioxidant defenses, acyl-CoA synthetase long-chain family member 4 (ACSL4)-mediated lipid remodeling, and iron dysregulation. Frontiers in Endocrinology (nine articles) and Free Radical Biology and Medicine (highest citation count: 171) emerged as pivotal publication platforms. Mechanistic analyses identified three ferroptosis defense axes (GPX4, FSP1/CoQ10, and GCH1/BH4) and cell type-specific vulnerabilities in tubular, podocyte, and endothelial cells. Preclinical agents, including ginkgolide B (GB) and dapagliflozin, effectively restored iron homeostasis and attenuated oxidative damage. Conclusion: Ferroptosis is a promising therapeutic target for DN, yet its clinical translation remains in its infancy. Future efforts should prioritize large-scale clinical trials, single-cell mechanistic profiling, and interdisciplinary integration to bridge molecular insights with precision therapies. This study provides a roadmap for advancing ferroptosis-targeted interventions for DN, emphasizing global collaborations and biomarker-driven strategies.
Keywords: ferroptosis, diabetic nephropathy, bibliometric analysis, visual analysis, GPx4
Received: 29 Oct 2024; Accepted: 25 Mar 2025.
Copyright: © 2025 Wei, Qin, Lin, Wang, Chen, Chen, Yan, Wei, Zhang and Wei. This is an open-access article distributed under the terms of the Creative Commons Attribution License (CC BY). The use, distribution or reproduction in other forums is permitted, provided the original author(s) or licensor are credited and that the original publication in this journal is cited, in accordance with accepted academic practice. No use, distribution or reproduction is permitted which does not comply with these terms.
* Correspondence:
Tangwen Wei, School of Public Health, Guilin Medical University, Guilin, China
Zhichang Zhang, Department of Computer Science, College of Intelligent Medicine, China Medical University, Shenyang, 110122, Liaoning Province, China
Bing Wei, Guilin Medical University, Guilin, China
Disclaimer: All claims expressed in this article are solely those of the authors and do not necessarily represent those of their affiliated organizations, or those of the publisher, the editors and the reviewers. Any product that may be evaluated in this article or claim that may be made by its manufacturer is not guaranteed or endorsed by the publisher.
Research integrity at Frontiers
Learn more about the work of our research integrity team to safeguard the quality of each article we publish.