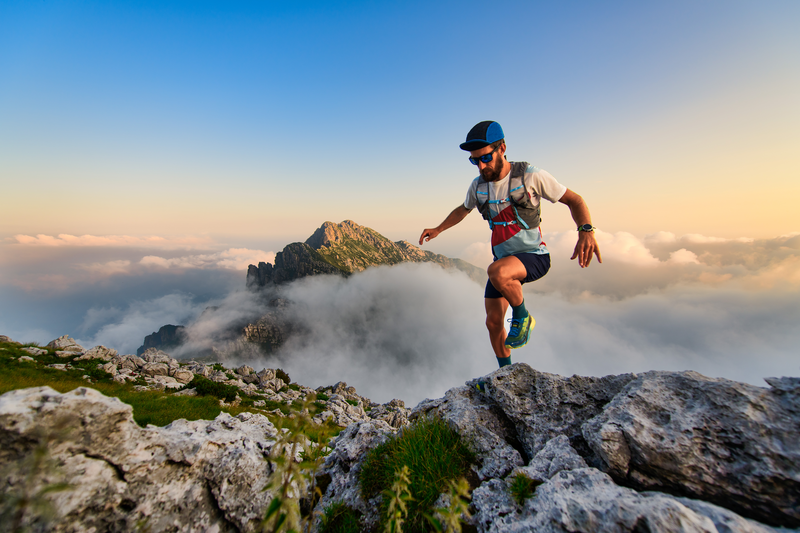
94% of researchers rate our articles as excellent or good
Learn more about the work of our research integrity team to safeguard the quality of each article we publish.
Find out more
ORIGINAL RESEARCH article
Front. Physiol.
Sec. Gastrointestinal Sciences
Volume 16 - 2025 | doi: 10.3389/fphys.2025.1494863
This article is part of the Research Topic (Epi)Genetic Alterations and Their Physiological Consequences in Metabolic Dysfunction-associated Steatotic Liver Disease: A Crucial Step Towards Precision Medicine in MASLD View all 3 articles
The final, formatted version of the article will be published soon.
You have multiple emails registered with Frontiers:
Please enter your email address:
If you already have an account, please login
You don't have a Frontiers account ? You can register here
Metabolic dysfunction-associated steatotic liver disease (MASLD) is the most prevalent chronic liver disease globally. However, the role of folate in MASLD remains controversial. This study aimed to investigate the association between two folate indicators (serum folate and red blood cell [RBC] folate) and MASLD prevalence using data from the 2017–2020 National Health and Nutrition Examination Survey (NHANES). A total of 3,879 participants without liver disease or significant alcohol consumption were included. Hepatic steatosis was assessed via transient elastography, with MASLD defined as controlled attenuation parameter (CAP) ≥285 dB/m and the presence of at least one cardiometabolic risk factor. Logistic regression and generalized additive models (GAM) were employed to evaluate associations between folate levels and MASLD, with subgroup analyses stratified by age, gender, and body mass index (BMI).After full adjustment for confounders, RBC folate exhibited a significant positive association with MASLD (OR=1.111, 95% CI: 1.015–1.216 per 1-unit increase). In contrast, serum folate showed a transient negative association in minimally adjusted models (OR=0.869, 95% CI: 0.802–0.941), which disappeared after further adjustments. Subgroup analyses confirmed that age, gender, and BMI did not modify the RBC folate–MASLD relationship. These findings suggest that elevated RBC folate levels are independently associated with MASLD prevalence, whereas serum folate may lack clinical relevance due to susceptibility to confounding factors. RBC folate, as a stable biomarker of long-term folate status, may serve as a superior indicator for investigating folate–MASLD associations.
Keywords: Metabolic dysfunction-associated steatotic liver disease, Red blood cell folate, Folate, Controlled attenuation parameters, National Health and Nutrition Examination Survey
Received: 11 Sep 2024; Accepted: 28 Feb 2025.
Copyright: © 2025 Liao, Yu, Wang, Zhang and Yu. This is an open-access article distributed under the terms of the Creative Commons Attribution License (CC BY). The use, distribution or reproduction in other forums is permitted, provided the original author(s) or licensor are credited and that the original publication in this journal is cited, in accordance with accepted academic practice. No use, distribution or reproduction is permitted which does not comply with these terms.
* Correspondence:
Ke Yu, Department of General Medicine, The General Hospital of Western Theater Command, ChengDu, China
Disclaimer: All claims expressed in this article are solely those of the authors and do not necessarily represent those of their affiliated organizations, or those of the publisher, the editors and the reviewers. Any product that may be evaluated in this article or claim that may be made by its manufacturer is not guaranteed or endorsed by the publisher.
Research integrity at Frontiers
Learn more about the work of our research integrity team to safeguard the quality of each article we publish.