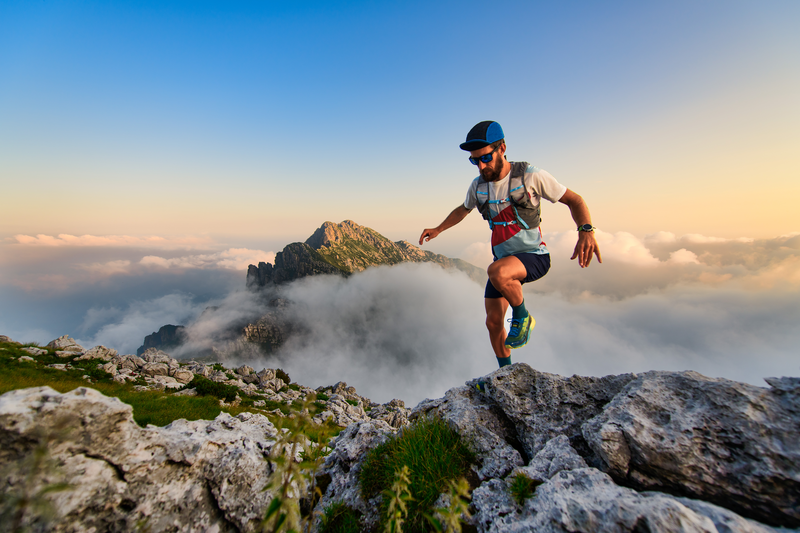
94% of researchers rate our articles as excellent or good
Learn more about the work of our research integrity team to safeguard the quality of each article we publish.
Find out more
ORIGINAL RESEARCH article
Front. Physiol.
Sec. Exercise Physiology
Volume 16 - 2025 | doi: 10.3389/fphys.2025.1448495
The final, formatted version of the article will be published soon.
You have multiple emails registered with Frontiers:
Please enter your email address:
If you already have an account, please login
You don't have a Frontiers account ? You can register here
Purpose: The aim of this study was to investigate the effects of a functional strength training (FST) program on ice speed and agility in young elite male ice hockey players.Methods: Forty-three ice hockey players, aged 15 to 18 years participated in the study. The athletes were randomly assigned to either a functional strength training group (FSTG), which completed an additional FST program consisting of two 60-minute training sessions per week, or a control group (CG), which participated in other team games and swimming sessions, each lasting 60 minutes. Ice skating speed was assessed using the professional Smart Speed measurement system in forward and backward skating tests over 5, 15, and 30 meters, as well as in an agility test.The intervention elicited significant performance improvements in ice skating speed and agility in the FSTG compared to the CG. Significant time Γ group interactions were observed in the 5-meter (p=0.041, ππ 2 =0.098), 15-meter (p=0.047, ππ 2 = 0.093), and 30-meter (p=0.011, ππ 2 =0.149) forward skating tests, highlighting differential responses between groups. Post hoc analysis confirmed significant improvements in the FSTG, particularly in the 15-meter and 30-meter tests, where post-test results were superior to those of the CG. No significant effects were found for the backward skating tests. Regarding agility, a significant main effect of time (p=0.023, ππ 2 =0.12) and group (p=0.001, ππ 2 =0.226) was detected. In the full speed test, only a group effect (p=0.026, ππ 2 =0.116) was observed, with no significant time x group interaction or time effects.Conclusions: These results underscore the effectiveness of FST in enhancing forward skating speed and agility.
Keywords: Ice hockey, speed, agility, smart speed system, Functional strength training
Received: 13 Jun 2024; Accepted: 25 Feb 2025.
Copyright: Β© 2025 Bieniec and Grabara. This is an open-access article distributed under the terms of the Creative Commons Attribution License (CC BY). The use, distribution or reproduction in other forums is permitted, provided the original author(s) or licensor are credited and that the original publication in this journal is cited, in accordance with accepted academic practice. No use, distribution or reproduction is permitted which does not comply with these terms.
* Correspondence:
MaΕgorzata Grabara, Institute of Sport Sciences, Jerzy Kukuczka Academy of Physical Education in Katowice, Katowice, Poland
Disclaimer: All claims expressed in this article are solely those of the authors and do not necessarily represent those of their affiliated organizations, or those of the publisher, the editors and the reviewers. Any product that may be evaluated in this article or claim that may be made by its manufacturer is not guaranteed or endorsed by the publisher.
Research integrity at Frontiers
Learn more about the work of our research integrity team to safeguard the quality of each article we publish.