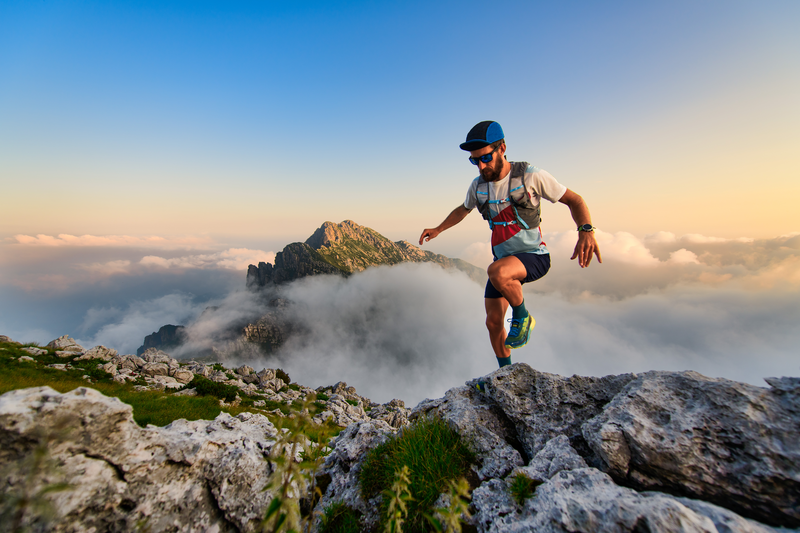
95% of researchers rate our articles as excellent or good
Learn more about the work of our research integrity team to safeguard the quality of each article we publish.
Find out more
REVIEW article
Front. Physiol.
Sec. Red Blood Cell Physiology
Volume 16 - 2025 | doi: 10.3389/fphys.2025.1445757
The final, formatted version of the article will be published soon.
You have multiple emails registered with Frontiers:
Please enter your email address:
If you already have an account, please login
You don't have a Frontiers account ? You can register here
Fetomaternal haemorrhage is the same immunity that occurs when foetal and maternal blood are incompatible. It is critical to accurately quantify maternal haemorrhaging in order to prevent hemolytic disease in the infant. At this time, the rosette test and K-B test are the most frequently used techniques for detecting foetal red blood cells in the mother's blood. However, the sensitivity of the rosette test is low, and due to its complex operation and high subjectivity, the K-B test cannot be used as a routine clinical detection method. This review therefore focuses primarily on the clinical landscape and future prospects of methods for detecting fetomaternal haemorrhage. In a general sense, this may bring to light the most promising strategy and encourage the development of technology for fetomaternal haemorrhage in order to guarantee the early detection and prevention of hemolytic disease in newborns.
Keywords: fetomaternal haemorrhage ( FMH ), Detection method, hemolytic disease in newborns(HDN), Fetal Hemoglobin, Flow Cytometry
Received: 25 Nov 2024; Accepted: 09 Jan 2025.
Copyright: © 2025 Li, Li and Liu. This is an open-access article distributed under the terms of the Creative Commons Attribution License (CC BY). The use, distribution or reproduction in other forums is permitted, provided the original author(s) or licensor are credited and that the original publication in this journal is cited, in accordance with accepted academic practice. No use, distribution or reproduction is permitted which does not comply with these terms.
* Correspondence:
Tiemei Liu, China-Japan Union Hospital, Jilin University, Changchun, 130033, Jilin Province, China
Disclaimer: All claims expressed in this article are solely those of the authors and do not necessarily represent those of their affiliated organizations, or those of the publisher, the editors and the reviewers. Any product that may be evaluated in this article or claim that may be made by its manufacturer is not guaranteed or endorsed by the publisher.
Research integrity at Frontiers
Learn more about the work of our research integrity team to safeguard the quality of each article we publish.