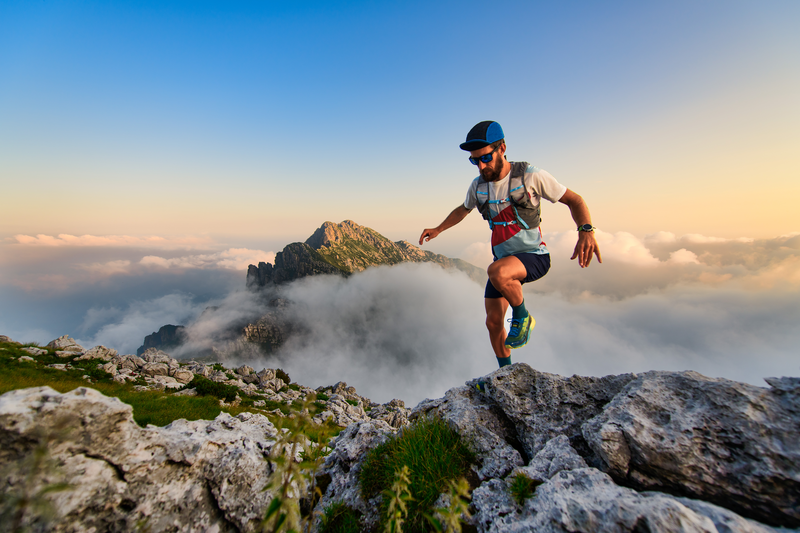
94% of researchers rate our articles as excellent or good
Learn more about the work of our research integrity team to safeguard the quality of each article we publish.
Find out more
ORIGINAL RESEARCH article
Front. Physiol. , 22 October 2024
Sec. Exercise Physiology
Volume 15 - 2024 | https://doi.org/10.3389/fphys.2024.1483142
This article is part of the Research Topic Physical, Physiological and Technical Development in Youth Athletes View all 8 articles
Introduction: Football is a physically demanding sport that requires effective recovery strategies to maintain performance level and prevent injuries. This study investigated if a single 1-h hyperbaric oxygen therapy (HBOT) session affects recovery and performance after a football match in elite youth players.
Methods: Twenty elite youth football players (age 17.3 ± 0.5 years) were randomly assigned to a HBOT group or a control group (CON). They played a 90-min football game and underwent either a 60-min HBOT or placebo intervention. Before (T1), at the end of the match (T2), 1 h after HBOT or CON session (T3), and 12 h after HBOT session (T4), subjects underwent biochemical (serum samples (myoglobin (MB), creatine kinase (CK), lactate dehydrogenase (LDH), alanine aminotransferase (ALT), and aspartate aminotransferase (AST)) and performance measurements (linear speed at 5 m, 10 m and 20 m, squat jump (SJ), countermovement jump (CMJ) and countermovement jump with arm swing (CMJa)). The Hooper Index (HI) was collected and heart rate was measured during the game.
Results: The football match induced significant increases in all biochemical markers, but no significant differences were found between the HBOT and control group in biochemical or performance parameters at any time point. However, there was a significant interaction effect between time and group for HI (p = 0.012, η2 = 0.124), with the HBOT group showing significantly lower HI values (8.6 ± 2.41) than the control group (11.0 ± 3.23) at 1 h post-HBOT.
Discussion: A single 1-h session of HBOT did not significantly affect recovery or performance parameters in elite youth football players, though it did show a moderate positive affect on the HI at 1 h post-HBOT. Further studies should explore the impact of either longer or sequential HBOT sessions on recovery.
Football is a physically demanding, competitive sport that causes stress on various physiological systems (Reilly and Ekblom, 2005). Previous studies (Silva et al., 2013; Viana-Gomes et al., 2018) showed that a single match causes significant increases in oxidative stress and cellular damage indicators in the plasma of professional football players. This can lead to acute, albeit usually small, decrements in sprint and jump performance (Abaïdia and Dupont, 2018). The tight schedule, the intermittent nature of the sport, performing muscle-damaging actions, and limited time for recovery can lead to an increased risk of fatigue, delayed-onset muscle soreness (DOMS) and injuries (Barnett, 2006; Cheung et al., 2003). In addition, previous studies (Abaïdia and Dupont, 2018; Magalhaes et al., 2010) reported that complete recovery after one match requires up to 72 h. Therefore, effective and fast recovery is the key to uphold performance levels.
Considerable research attention has been given to strategies to accelerate acute recovery in football players. Nutrition and antioxidant supplements, sleep, cold water immersion, active recovery, and compression garments have been suggested (Dupuy et al., 2018; Nédélec et al., 2013; Santos et al., 2016) to reduce anti-inflammatory responses and fatigue immediately after the match, as well as to prevent further functional impairment. However, the area of effective recovery strategies remains unclear due to the limited number of studies that investigated elite players (Barnett, 2006) and the variety of modalities without empirical evidence of which one should be followed. Since the literature indicates the essential role of fast recovery, but lacks clear findings (Kellmann, 2010), an increased interest in hyperbaric oxygen therapy (HBOT) has emerged in recent years (Mihailovic et al., 2023). HBOT has been reported to accelerate cell regeneration and tissue repair, which should help eliminate fatigue and restore endurance capacity. More precisely, HBOT is a treatment in which 100% oxygen is supplied under elevated pressure. Such treatment increases dissolved oxygen levels in the blood and results in a high partial pressure of oxygen in peripheral tissues, which is beneficial for conditions associated with low oxygen environments, potentially stimulating the recovery process (Hodges et al., 2003). HBOT can improve the oxygenation of skeletal muscles, which accelerates the production of adenosine triphosphate (ATP) in addition to the metabolic purification of metabolites that cause fatigue (Sperlich et al., 2017). Moreover, HBOT is proven to be safe and effective in the non-athletic population as a treatment for accelerating the healing process and reducing local hypoxia and inflammation (Moghadam et al., 2020). Until now, HBOT has been applied in various ways, ranging from acute (single) sessions, such as 60-min interventions (Park et al., 2018; Branco et al., 2016), to more chronic protocols involving multiple sessions per week over extended periods (Hadanny et al., 2022; Mihailovic et al., 2023). The choice of protocol often depends on the goals, such as acute recovery or long-term adaptation.
HBOT administration has been proposed as an adjuvant treatment for improving muscle repair and recovery from exercise-induced muscle damage (Ishii et al., 2005). Surprisingly, although oxygen has the most important role during recovery, only two studies (Branco et al., 2016; Mihailovic et al., 2023) have investigated the post-exercise impact of HBOT on biochemical recovery parameters or performance in athletes. Branco et al. (2016) investigated the effects of a single session HBOT on hormonal and cell damage markers in professional jiu-jitsu athletes and failed to find a significant influence of HBOT, while Park et al. (2018) found positive effects of a single 60-min HBOT on the removal of peripheral fatigue symptoms induced by maximal exercise in college football players. Similarly, in terms of the acute effects of HBOT on performance, previous studies (Hadanny et al., 2022; Hodges et al., 2003; McGavock et al., 1999; Mihailovic et al., 2023) indicated equivocal findings. While early studies (Hodges et al., 2003; McGavock et al., 1999) suggested no effects of a single HBOT session on performance, later research (Mihailovic et al., 2023; Park et al., 2018) seems to indicate that HBOT was able to improve cardiac parasympathetic reactivation by HBOT applications over several weeks and recovery of peripheral fatigue by a single HBOT session.
The role of HBOT on recovery parameters and performance in elite football players is still poorly understood, and to date, no study has specifically examined the effects of a single session of HBOT after a football match. Since football is an intermittent sport with high physical, physiological, and metabolic demands, HBOT might be considered a promising post-exercise recovery treatment. Therefore, this study aimed to investigate the effects of a single HBOT session on recovery and performance after a football match in elite youth football players. We hypothesized that a single session of HBOT would significantly enhance recovery and improve performance compared to a placebo condition.
Twenty elite youth male football players (age: 17.3 ± 0.5 years; football experience: 10.2 ± 1.7 years) participated in the study, and informed consent was obtained from their parents or legal guardians. Participants were free of injuries and medical conditions contraindicated by HBOT. All participants were informed of the study procedures and provided written parental consent prior to participation. Participants were advised to refrain from severe physical activity for 24 h and to abstain from breakfast, caffeine, and alcohol prior to the first blood collection.
The study was conducted using a randomized, double-blind design. The participants were randomly assigned to either the HBOT group or the control (CON) group. All participants were evaluated for biochemical parameters, physical performance tests and Hooper index (HI). The participants were tested four times (Figure 1): at baseline, pre-match (T1), at the end of the match, post-match (T2), 1 hour after the HBOT session (T3), and 12 h after the HBOT session (T4). Fasted blood samples were collected before breakfast. After a standardized light meal and rest period, participants were evaluated for physical performance with the linear speed and vertical jump (VJ) height tests before playing a football match (T1). Participants were randomized in a 1:1 fashion into two groups based on their playing positions to play against each other. In the afternoon the match took place on an outdoor football field and lasted for 90 min at an average ambient temperature of 26°C. It was a simulated game replacing a regular training session, with 22 players. Both teams used a 5-three to two line up. Goalkeepers were excluded from the analysis because of the different physical demands of this position. The same examination was performed after the match (T2), followed by HBOT or CON treatment. Finally, blood samples and physical fitness were evaluated 1 h (T3) and 12 h (T4) after the intervention. In addition, heart rate (HR; Polar Team System H7 (Polar Electro Oy, Kempele, Finland)) was constantly tracked during the match to determine its intensity. Procedures were conducted with the requirements and approval of the Ethical Committee of Faculty of Sport and Physical Education, University of Niš (ref. 04–651/2; approval date 23 May 2022) and registered at ClinicalTrials.gov under NCT06112210.
Blood samples were collected from the antecubital vein with participants in a seated position. A vacutainer tube was used to collect blood samples (Vacusera 5 mL Serum Gel and Clot Activator, Disera A.S. Izmir-Turkey) and centrifuged immediately at 3,000 rpm for 10 min to isolate the serum. Myoglobin (MB) was determined with Siemens IMMULITE 1000 (Siemens Healthcare Diagnostics, UK), while creatine kinase (CK), lactate dehydrogenase (LDH), alanine aminotransferase (ALT), and aspartate aminotransferase (AST) were analyzed using a biochemistry analyzer (A25 Biosystems Chemistry Analyzer).
Squat jump (SJ), countermovement jump (CMJ) and countermovement jump with arm swing (CMJa) tests were performed to measure the VJ height. The Optojump system (Microgate, Bolzano, Italy) was used to determine vertical VJ height; its validity and reliability had previously been shown (Glatthorn et al., 2011). Previously, the procedure as well as the validity and reliability of the tests in football players were examined (Claudino et al., 2017; Garrett et al., 2020). Briefly, the SJ was executed in a semi squat position with the knees bent at 90° and the arms resting on the hips. Participants held this stance for one to 3 seconds before the maximal VJ. Moreover, the CMJ and the CMJa were evaluated while standing straight up, with equal weight distributed over both feet. The hands were free to move when the CMJa was conducted. Furthermore, the arms were secured to the hips during the CMJ to prevent their impact on jump height. Afterwards, participants squatted down to a 90-degree flexion and executed a maximal VJ without pausing at the moment of direction change. Each participant was provided with instructions to perform all jumps with maximal effort. Furthermore, each jump was attempted three times, with the best result being included in the statistical analysis.
Linear speed was measured from a standing position at 5 m, 10 m, and 20 m, as previously described (Buchheit et al., 2010; Dugdale et al., 2019). The Witty photocell system (Witty, System, Microgate, Bolzano, Italy) was used to assess the performance. In addition, to reduce the influence of the hand swing when passing through the gate, photocells were positioned at 10 m and 20 m from the starting line, 0.4 m above the ground, and with an accuracy of 0.001 m/s (Yeadon et al., 1999). Participants were instructed to exert full effort when passing through all gates.
Both the HBOT and placebo protocols were performed in a Barox HBOT chamber (Yaklasim Makina San. Ve Tic. Ltd. Sti). The participants were taken to the chamber immediately after the football match, where they sat in individual chairs and oxygen was delivered through individual masks. The HBOT group was exposed to 100% oxygen at 2.2 ATA (atmospheres absolute), while the CON group was exposed to normobaric ambient pressure (1 ATA). Both sessions lasted 60 min. This protocol was selected to follow current literature (Babul et al., 2003; Mekjavic et al., 2000; Shimoda et al., 2015; Webster et al., 2002).
HI was used for the subjective assessment of fatigue and players’ wellbeing. Subjects were unfamiliar with the HI and therefore it was thoroughly explained before the start of the experiment. The HI is a summation of the four subjective ratings: sleep (concerning the night preceding the evaluation), fatigue, stress and DOMS on a scale of 1–7 (Hooper et al., 1995). Specifically, ‘1’ represents “very, very good state”, and ‘7’ represents “very, very bad state”. After the participants completed the questionnaire, the HI was calculated and used for analysis. Moreover, HI has been shown to be a valid and reliable tool for monitoring fatigue in professional football players (Clemente et al., 2017; Thorpe et al., 2015).
Data analysis was performed with the Statistical Package for the Social Sciences (v29.0; SPSS Inc., Chicago, IL, United States). The mean ± standard deviation (SD), Kolmogorov‒Smirnov test to examine normality of distribution, and Levene’s test to examine homogeneity of variance were determined for all outcome measures. Changes in biochemical parameters and physical fitness parameters were compared between subjects over four time points for the HBOT and CON group using a repeated measures analysis of variance (RM-ANOVA). Finally, to evaluate the magnitude of the observed differences, the effect size was calculated (eta squared, η2) and interpreted as follows: <0.2 (small), >0.2 and <0.8 (moderate) and >0.8 (large) (Cohen, 1988). A power analysis was conducted using G*Power based on an effect size of 0.63 for CK levels from a similar study (Qu et al., 2024). With an α level of 0.05 and a desired power of 0.80, the analysis indicated that a total sample size of 22 participants would be required to detect statistically significant differences between the HBOT and CON group.
The main characteristics of the participants (height, weight, fat mass, muscle mass and body mass index (BMI)) as well as time spent (%) in various heart zones during the match are summarized in Table 1 and Figure 2.
Figure 2. Time spent (%) in various heart rate zones as a percentage of maximum heart rate (HRmax) during the match. Note: comparisons are presented as HBOT vs. CON.
Table 2 and Figure 3 shows descriptive data for both groups through four time points. The results indicated no significant differences between the HBOT and CON group in biochemical parameters at T1. Furthermore, there was no interaction effect between time and group for any of the measured variables (p > 0.05).
Figure 3. Biochemical parameters measured throughout four time points: pre-match (T1), post-match (T2), 1 hour after HBOT (T3) and 12 h after HBOT (T4).
The match induced a significant increase (T1 vs. T2) in MB (p = 0.003), CK (p < 0.001), LDH (p < 0.001), ALT (p < 0.001), and in AST (p < 0.001).
The results indicated no significant differences between HBOT and CON group in physical fitness tests at T1. Furthermore, there was no interaction effect between time and group for any of the measured variables (p > 0.05) (Table 3).
The match had no effect on most of the performance parameters except for SJ (T1 vs. T2; p = 0.012).
The results indicated no significant differences between HBOT and CON group in HI at T1. However, there was an interaction effect between time and group for HI (p = 0.012, η2 = 0.124). More precisely, the HBOT group had significantly lower HI values (8.6 ± 2.41) than the CON group (11.0 ± 3.23) at T3 (Figure 4). The match also presented a significant increase (T1 vs. T2) in HI (p < 0.001).
Figure 4. Hooper index (HI) values measured throughout four time points: pre-match (T1), post-match (T2), 1 hour after HBOT (T3) and 12 h after HBOT (T4). Note: comparisons are presented as HBOT vs. CON.
The aim of this study was to determine the effect of a single session of HBOT on recovery parameters in elite youth football players. Briefly, the match clearly induced the anticipated biochemical changes indicating fatigue. However, the results indicated that there were no significant differences between the HBOT and CON group at T3 (after the intervention) for markers of muscle damage (MB, CK, LDH, ALT, and AST) and performance parameters (linear speed at 5 m, 10 m and 20 m, CMJ, CMJa, and SJ). Similarly, at T4, there were no significant differences between the groups for the same variables. Interestingly, the results revealed a significant difference between groups at T3 for HI, with a moderate positive effect of HBOT on this parameter.
Serum markers (MB, CK, LDH, ALT, and AST) have been extensively researched as parameters of muscle cell damage in elite football players (Ascensão et al., 2008; Ispirlidis et al., 2008; Meyer and Meister, 2011). The study’s findings revealed that MB, CK, LDH, AST, and ALT concentrations increased in both groups immediately following the football match. Although the concentrations of LDH, AST and ALT decreased after HBOT and continued in the same manner 12 h after HBOT, the results demonstrated no statistically significant effect of a single session of HBOT. Conversely, the most relevant marker of muscle damage, CK, had a drastic increase at all time points compared to baseline without a significant difference between groups. Existing studies (Branco et al., 2016; Harrison et al., 2001; Huang et al., 2021; Woo et al., 2020) examining post-exercise interventions have provided contradictory findings. While our findings are consistent with previous results showing no positive effect of HBOT on biochemical recovery parameters (Branco et al., 2016; Harrison et al., 2001; Huang et al., 2021), Woo et al. (2020) found that HBOT applied during the post-exercise recovery period is effective for treating exercise-induced muscle damage. It is noteworthy that none of these studies have assessed acute recovery using HBOT in elite youth football players immediately after a competitive match. Football is a very strenuous sport with high physiological and metabolic demands, where some markers of cell damage increase up to 72 h after the match and then return to their baseline values (Fatouros et al., 2010; Silva et al., 2013). More precisely, changes in direction and rapid accelerations and decelerations impose a significant eccentric load on the muscles, resulting in microinjuries and the release of CK and MB up to 72 h after a football match. In addition, their concentration depends on many factors, such as level of playing, period of season, individual responses, and previous activities (Brancaccio et al., 2007; Silva et al., 2013). In the present study, the participants were advised to refrain from stressful activities for 24h, which means that a certain concentration of CK remained in the blood. Furthermore, the participants evaluated in this study were in the off-season period, which probably resulted in higher concentrations of CK in the blood after the match. Therefore, our study indicates that a single 60 min session of HBOT may not be sufficient to improve biochemical recovery parameters in elite youth football players. The similarity in blood parameters between the HBOT and CON group could be due to the short duration and single session of HBOT, the natural and effective recovery processes in elite youth athletes, the potential insensitivity of the measured biochemical markers, or the timing of the measurements. To better understand the effects of HBOT, future studies should consider multiple sessions, a larger sample size, and possibly more sensitive or additional markers of recovery.
HBOT increases dissolved oxygen levels in the blood and results in a high partial pressure of oxygen in peripheral tissues, it could potentially stimulate the recovery process (Hodges et al., 2003). Since HBOT improves the oxygenation of skeletal muscles, it may accelerate the production of ATP in addition to the metabolic purification of metabolites that cause fatigue (Sperlich et al., 2017). Therefore, we hypothesized that HBOT treatment would positively affect the main physical fitness indicators of performance such as linear speed and vertical jump height. However, the findings of our study indicate no effect of a single session on these parameters when compared to post-match measurements in youth elite football players. One reason for this finding could be that our simulated game did not affect these parameters in the first place. In general literature describes a modest deterioration of jump and speed performance (Abaïdia and Dupont, 2018), although this is not always the case (Krustrup et al., 2010). Several factors, such as fitness level, game status (winning vs. losing), potential rewards and environmental conditions, indeed make these parameters variable (Abaïdia and Dupont, 2018). One could also argue that the lack of impact is caused by a too low intensity during the game, although this is not substantiated through our heart rate data and the biochemical changes that occurred. The lack of effect after HBOT in the current study is congruent with the work of (McGavock et al., 1999; Hodges et al., 2003), who found no significant effect of acute HBOT treatment on performance. More precisely, previous studies (Hodges et al., 2003; McGavock et al., 1999) tried to determine the acute effects of HBOT at 2.5 ATA for 90 min on cardiorespiratory fitness and concluded that the experimental groups did not improve or deteriorate performance on the maximal and submaximal running tests. These findings contrast with a previous study (Mihailovic et al., 2023) reporting post-HBOT treatment as an efficient way to improve power output during cycling. However, the different parameters and populations assessed within studies investigating the single-session effect of HBOT on post-exercise performance make it difficult to compare the results. Although several studies have examined the acute effects of HBOT on performance, to date, no study has specifically examined the acute effects of HBOT on several parameters of physical performance in elite youth football players, who exhibit fewer performance impairments and metabolic disturbances than lower-level players. More precisely, the large muscle groups involved in running or jumping become more resistant in this population due to intense training (Reilly et al., 2008). Also, young elite players (U20) have a shorter and more efficient recovery. Nevertheless, it is crucial to draw attention to the beneficial findings of a recent study (Burgos et al., 2016) investigating the effects of 3 weeks of HBOT training on oxidative stress markers and endurance in young football players. Specifically, the authors (Burgos et al., 2016) found that 3 weeks of HBOT did not cause an increase in oxidative stress but improved endurance capacity. Therefore, if utilized for a long period of time, HBOT may have significant ergogenic effects in professional football players.
Our results showed a significant positive effect in HI after the HBOT compared to CON 1 hour after the treatment. Since the HI parameter is the most widely used parameter in football for subjective evaluation of match-induced fatigue, this indicates that although we were unable to induce significant differences in biochemical or performance parameters, we were successful in reducing the perception of fatigue after the match. The HI has been employed as a tool to detect changes in wellbeing in football players, with specific focus on fatigue, stress, sleep quality and DOMS, over the course of a season (Silva R. M. et al., 2022) or in the early-season versus in-season (Silva A. F. et al., 2022). Furthermore, this confirms previous findings that the HI index is more sensitive than physiological parameters, such as heart rate variability (Rabbani et al., 2019) or blood parameters as measured in our study, to track fatigue in a specific football population.
The major strength of this study is that it was the first double-blind randomized controlled study investigating the effects of a single session of HBOT on biochemical recovery and performance parameters in elite youth football players. Furthermore, the evaluation of an extensive range of parameters and the protocol used are strengths to consider. However, some limitations of our study should be acknowledged when interpreting the findings. First, the findings should be interpreted with caution due to the specific pool of participants included, representing a small sample size because of the methodological constraint of employing one football game and the size of the Barox HBOT chamber. Given the actual sample size in this study (n = 20), the study may have been underpowered to detect medium effect sizes for other outcomes. Second, the studies’ comparison with current literature is difficult because of the limited number of studies investigating the acute effects of a single session of HBOT. Third, we advised the participants to refrain from any form of physical activity for a period of 24h, but we did not monitor or control their activities during the period of 48 h. Finally, although elite football players were recruited in our study, our findings are not generalizable to senior male professional football players, female football players, or athletes competing in other sports. Our research does not support the effectiveness of a single session of HBOT as a recovery model due to the absence of significant effects and the cost of treatment and equipment. However, large cohort studies examining acute effects on male and female football players and athletes competing across a wider range of sports should be conducted in the future. In addition, future research will have to further investigate acute HBOT protocols of different durations. Finally, future research will have to concentrate on the effects of sequential HBOT sessions on recovery and performance parameters.
In summary, our findings showed that a single session of HBOT did not have significant effects on selected biochemical recovery and performance parameters in elite youth football players. We did observe a lower HI score after the HBOT session compared to the placebo group, suggesting that HBOT may have a moderate positive effect on perceived recovery and wellbeing. Nevertheless, our findings may suggest a need for multiple HBOT sessions or a larger sample size to observe significant changes. Future studies are needed to confirm or refute our findings and to determine the optimal use of HBOT as a recovery intervention in football.
The raw data supporting the conclusions of this article will be made available by the authors, without undue reservation.
The studies involving humans were approved by Ethical Committee of Faculty of Sport and Physical Education, University of Niš (ref. 04-651/2). The studies were conducted in accordance with the local legislation and institutional requirements. Written informed consent for participation in this study was provided by the participant’s legal guardians/next of kin. Written informed consent was obtained from the individual(s), and minor(s) legal guardian/next of kin, for the publication of any potentially identifiable images or data included in this article.
MG: Conceptualization, Investigation, Writing–original draft. TS: Conceptualization, Investigation, Supervision, Visualization, Writing–review and editing. AL: Methodology, Writing–original draft. SA: Conceptualization, Investigation, Resources, Writing–review and editing. BR: Formal Analysis, Methodology, Writing–review and editing. ŠB: Conceptualization, Funding acquisition, Investigation, Methodology, Project administration, Supervision, Visualization, Writing–original draft, Writing–review and editing.
The author(s) declare that financial support was received for the research, authorship, and/or publication of this article. This study was funded by the Slovenian Research Agency (postdoctoral research project Z3-3212 and research and infrastructure program P3-0323).
The authors would like to thank the participants and medical staff for their cooperation throughout the study. Bart Roelands is a Collen-Francqui research professor and a member of the Strategic Research Program Exercise and the Brain in Health and Disease: The Added Value of Human-Centered Robotics (SRP77).
The authors declare that the research was conducted in the absence of any commercial or financial relationships that could be construed as a potential conflict of interest.
All claims expressed in this article are solely those of the authors and do not necessarily represent those of their affiliated organizations, or those of the publisher, the editors and the reviewers. Any product that may be evaluated in this article, or claim that may be made by its manufacturer, is not guaranteed or endorsed by the publisher.
Abaïdia A., Dupont G. (2018). Recovery strategies for football players. Swiss Sport Exerc Med. 66, 28–36.
Ascensão A., Rebelo A., Oliveira E., Marques F., Pereira L., Magalhães J. J. C. b. (2008). Biochemical impact of a soccer match—analysis of oxidative stress and muscle damage markers throughout recovery. Clin. Biochem. 41 (10-11), 841–851. doi:10.1016/j.clinbiochem.2008.04.008
Babul S., Rhodes E. C., Taunton J. E., Lepawsky M. (2003). Effects of intermittent exposure to hyperbaric oxygen for the treatment of an acute soft tissue injury. Clin. J. Sport Med. 13 (3), 138–147. doi:10.1097/00042752-200305000-00003
Barnett A. (2006). Using recovery modalities between training sessions in elite athletes: does it help? Sports Med. 36, 781–796. doi:10.2165/00007256-200636090-00005
Brancaccio P., Maffulli N., Limongelli F. M. (2007). Creatine kinase monitoring in sport medicine. Br. Med. Bull. 81-82 (1), 209–230. doi:10.1093/bmb/ldm014
Branco B. H., Fukuda D. H., Andreato L. V., Santos J. F., Esteves J. V., Franchini E. (2016). The effects of hyperbaric oxygen therapy on post-training recovery in jiu-jitsu athletes. PLoS One 11 (3), e0150517. doi:10.1371/journal.pone.0150517
Buchheit M., Mendez-Villanueva A., Simpson B. M., Bourdon P. C. (2010). Match running performance and fitness in youth soccer. Int. J. Sports Med. 31 (11), 818–825. doi:10.1055/s-0030-1262838
Burgos C., Henriquez-Olguin C., Andrade D. C., Ramirez-Campillo R., Araneda O. F., White A., et al. (2016). Effects of exercise training under hyperbaric oxygen on oxidative stress markers and endurance performance in young soccer players: a pilot study. J. Nutr. Metab. 2016, 5647407. doi:10.1155/2016/5647407
Cheung K., Hume P., Maxwell L. (2003). Delayed onset muscle soreness: treatment strategies and performance factors. Sports Med. 33 (2), 145–164. doi:10.2165/00007256-200333020-00005
Claudino J. G., Cronin J., Mezencio B., McMaster D. T., McGuigan M., Tricoli V., et al. (2017). The countermovement jump to monitor neuromuscular status: a meta-analysis. J. Sci. Med. Sport 20 (4), 397–402. doi:10.1016/j.jsams.2016.08.011
Clemente F. M., Mendes B., Nikolaidis P. T., Calvete F., Carrico S., Owen A. L. (2017). Internal training load and its longitudinal relationship with seasonal player wellness in elite professional soccer. Physiol. Behav. 179, 262–267. doi:10.1016/j.physbeh.2017.06.021
Cohen J. J. H. N. J. (1988). Statistical power analysis for the behavioral sciences. Lawrence Erlbaum, 75–108.
Dugdale J. H., Arthur C. A., Sanders D., Hunter A. M. (2019). Reliability and validity of field-based fitness tests in youth soccer players. Eur. J. Sport Sci. 19 (6), 745–756. doi:10.1080/17461391.2018.1556739
Dupuy O., Douzi W., Theurot D., Bosquet L., Dugue B. (2018). An evidence-based approach for choosing post-exercise recovery techniques to reduce markers of muscle damage, soreness, fatigue, and inflammation: a systematic review with meta-analysis. Front. Physiol. 9, 403. doi:10.3389/fphys.2018.00403
Fatouros I. G., Chatzinikolaou A., Douroudos I. I., Nikolaidis M. G., Kyparos A., Margonis K., et al. (2010). Time-course of changes in oxidative stress and antioxidant status responses following a soccer game. J. Strength Cond. Res. 24 (12), 3278–3286. doi:10.1519/JSC.0b013e3181b60444
Garrett J. M., Graham S. R., Eston R. G., Burgess D. J., Garrett L. J., Jakeman J., et al. (2020). Comparison of a countermovement jump test and submaximal run test to quantify the sensitivity for detecting practically important changes within high-performance Australian rules football. Int. J. Sports Physiol. Perform. 15 (1), 68–72. doi:10.1123/ijspp.2019-0150
Glatthorn J. F., Gouge S., Nussbaumer S., Stauffacher S., Impellizzeri F. M., Maffiuletti N. A. (2011). Validity and reliability of Optojump photoelectric cells for estimating vertical jump height. J. Strength Cond. Res. 25 (2), 556–560. doi:10.1519/JSC.0b013e3181ccb18d
Hadanny A., Hachmo Y., Rozali D., Catalogna M., Yaakobi E., Sova M., et al. (2022). Effects of hyperbaric oxygen therapy on mitochondrial respiration and physical performance in middle-aged athletes: a blinded, randomized controlled trial. Sports Med. Open 8 (1), 22. doi:10.1186/s40798-021-00403-w
Harrison B. C., Robinson D., Davison B. J., Foley B., Seda E., Byrnes W. C. (2001). Treatment of exercise-induced muscle injury via hyperbaric oxygen therapy. Med. Sci. Sports Exerc 33 (1), 36–42. doi:10.1097/00005768-200101000-00007
Hodges A. N., Delaney S., Lecomte J. M., Lacroix V. J., Montgomery D. L. (2003). Effect of hyperbaric oxygen on oxygen uptake and measurements in the blood and tissues in a normobaric environment. Br. J. Sports Med. 37 (6), 516–520. doi:10.1136/bjsm.37.6.516
Hooper S. L., Mackinnon L. T., Howard A., Gordon R. D., Bachmann A. W. (1995). Markers for monitoring overtraining and recovery. Med. Sci. Sports Exerc 27 (1), 106–112. doi:10.1249/00005768-199501000-00019
Huang X., Wang R., Zhang Z., Wang G., Gao B. (2021). Effects of pre-post- and intra-exercise hyperbaric oxygen therapy on performance and recovery: a systematic review and meta-analysis. Front. Physiol. 12, 791872. doi:10.3389/fphys.2021.791872
Ishii Y., Deie M., Adachi N., Yasunaga Y., Sharman P., Miyanaga Y., et al. (2005). Hyperbaric oxygen as an adjuvant for athletes. Sports Med. 35, 739–746. doi:10.2165/00007256-200535090-00001
Ispirlidis I., Fatouros I. G., Jamurtas A. Z., Nikolaidis M. G., Michailidis I., Douroudos I., et al. (2008). Time-course of changes in inflammatory and performance responses following a soccer game. Clin. J. Sport Med. 18 (5), 423–431. doi:10.1097/JSM.0b013e3181818e0b
Kellmann M. (2010). Preventing overtraining in athletes in high-intensity sports and stress/recovery monitoring. Scand. J. Med. Sci. Sports 20 (Suppl. 2), 95–102. doi:10.1111/j.1600-0838.2010.01192.x
Krustrup P., Zebis M., Jensen J. M., Mohr M. (2010). Game-induced fatigue patterns in elite female soccer. J. Strength Cond. Res. 24 (2), 437–441. doi:10.1519/JSC.0b013e3181c09b79
Magalhaes J., Rebelo A., Oliveira E., Silva J. R., Marques F., Ascensao A. (2010). Impact of Loughborough Intermittent Shuttle Test versus soccer match on physiological, biochemical and neuromuscular parameters. Eur. J. Appl. Physiol. 108 (1), 39–48. doi:10.1007/s00421-009-1161-z
McGavock J. M., Lecomte J. L., Delaney J. S., Lacroix V. J., Hardy P., Montgomery D. L. (1999). Effects of hyperbaric oxygen on aerobic performance in a normobaric environment. Undersea Hyperb. Med. 26 (4), 219–224.
Mekjavic I. B., Exner J. A., Tesch P. A., Eiken O. (2000). Hyperbaric oxygen therapy does not affect recovery from delayed onset muscle soreness. Med. Sci. Sports Exerc 32 (3), 558–563. doi:10.1097/00005768-200003000-00002
Meyer T., Meister S. (2011). Routine blood parameters in elite soccer players. Int. J. Sports Med. 32 (11), 875–881. doi:10.1055/s-0031-1280776
Mihailovic T., Bouzigon R., Bouillod A., Grevillot J., Ravier G. (2023). Post-exercise hyperbaric oxygenation improves recovery for subsequent performance. Res. Q. Exerc Sport 94 (2), 427–434. doi:10.1080/02701367.2021.2002797
Moghadam N., Hieda M., Ramey L., Levine B. D., Guilliod R. (2020). Hyperbaric oxygen therapy in sports musculoskeletal injuries. Med. Sci. Sports Exerc 52 (6), 1420–1426. doi:10.1249/MSS.0000000000002257
Nédélec M., McCall A., Carling C., Legall F., Berthoin S., Dupont G. (2013). Recovery in soccer: part II—recovery strategies. Sports Med. 43, 9–22. doi:10.1007/s40279-012-0002-0
Park S.-H., Park S.-J., Shin M.-S., Kim C.-K. (2018). The effects of low-pressure hyperbaric oxygen treatment before and after maximal exercise on lactate concentration, heart rate recovery, and antioxidant capacity. J. Exerc Rehabil. 14 (6), 980–984. doi:10.12965/jer.1836468.234
Qu C., Xu M., Lorenzo S., Huang P., Rao Z., Geng X., et al. (2024). Effects of mild hyperbaric oxygen therapy on timing sequence recovery of muscle fatigue in Chinese university male athletes. J. Exerc. Sci. Fit. 22 (4), 305–315. doi:10.1016/j.jesf.2024.04.005
Rabbani A., Clemente F. M., Kargarfard M., Chamari K. (2019). Match fatigue time-course assessment over four days: usefulness of the hooper index and heart rate variability in professional soccer players. Front. Physiol. 10, 109. doi:10.3389/fphys.2019.00109
Reilly T., Drust B., Clarke N. (2008). Muscle fatigue during football match-play. Sport Med. 38, 357–367. doi:10.2165/00007256-200838050-00001
Reilly T., Ekblom B. (2005). The use of recovery methods post-exercise. J. Sports Sci. 23 (6), 619–627. doi:10.1080/02640410400021302
Santos S. A., Silva E. T., Caris A. V., Lira F. S., Tufik S., Dos Santos R. V. (2016). Vitamin E supplementation inhibits muscle damage and inflammation after moderate exercise in hypoxia. J. Hum. Nutr. Diet. 29 (4), 516–522. doi:10.1111/jhn.12361
Shimoda M., Enomoto M., Horie M., Miyakawa S., Yagishita K. (2015). Effects of hyperbaric oxygen on muscle fatigue after maximal intermittent plantar flexion exercise. J. Strength Cond. Res. 29 (6), 1648–1656. doi:10.1519/jsc.0000000000000809
Silva A. F., Oliveira R., Cataldi S., Clemente F. M., Latino F., Badicu G., et al. (2022a). Weekly variations of well-being and interactions with training and match intensities: a descriptive case study in youth male soccer players. Int. J. Environ. Res. Public Health 19 (5), 2935. doi:10.3390/ijerph19052935
Silva J. R., Ascensao A., Marques F., Seabra A., Rebelo A., Magalhaes J. (2013). Neuromuscular function, hormonal and redox status and muscle damage of professional soccer players after a high-level competitive match. Eur. J. Appl. Physiol. 113 (9), 2193–2201. doi:10.1007/s00421-013-2633-8
Silva R. M., Clemente F. M., González-Fernández F. T., Nobari H., Oliveira R., Silva A. F., et al. (2022b). Relationships between internal training intensity and well-being changes in youth football players. Healthcare 10 (10), 1814. doi:10.3390/healthcare10101814
Sperlich B., Zinner C., Hauser A., Holmberg H. C., Wegrzyk J. (2017). The impact of hyperoxia on human performance and recovery. Sports Med. 47 (3), 429–438. doi:10.1007/s40279-016-0590-1
Thorpe R. T., Strudwick A. J., Buchheit M., Atkinson G., Drust B., Gregson W. (2015). Monitoring fatigue during the in-season competitive phase in elite soccer players. Int. J. Sports Physiol. Perform. 10 (8), 958–964. doi:10.1123/ijspp.2015-0004
Viana-Gomes D., Rosa F., Mello R., Paz G., Miranda H., Salerno V. (2018). Oxidative stress, muscle and liver cell damage in professional soccer players during a 2-game week schedule. Sci. and Sports 33 (5), e221–e228. doi:10.1016/j.scispo.2018.02.013
Webster A. L., Syrotuik D. G., Bell G. J., Jones R. L., Hanstock C. C. (2002). Effects of hyperbaric oxygen on recovery from exercise-induced muscle damage in humans. Clin. J. Sport Med. 12 (3), 139–150. doi:10.1097/00042752-200205000-00001
Woo J., Min J. H., Lee Y. H., Roh H. T. (2020). Effects of hyperbaric oxygen therapy on inflammation, oxidative/antioxidant balance, and muscle damage after acute exercise in normobaric, normoxic and hypobaric, hypoxic environments: a pilot study. Int. J. Environ. Res. Public Health 17 (20), 7377. doi:10.3390/ijerph17207377
Keywords: hyperbaric oxygen therapy, football, performance, recovery, young athletes, blood analysis
Citation: Gušić M, Stantić T, Lazić A, Andrašić S, Roelands B and Bogataj Š (2024) Effects of hyperbaric oxygen therapy on recovery after a football match in young players: a double-blind randomized controlled trial. Front. Physiol. 15:1483142. doi: 10.3389/fphys.2024.1483142
Received: 19 August 2024; Accepted: 09 October 2024;
Published: 22 October 2024.
Edited by:
Roberto Modena, University of Verona, ItalyReviewed by:
YinHang Cao, Shanghai University of Sport, ChinaCopyright © 2024 Gušić, Stantić, Lazić, Andrašić, Roelands and Bogataj. This is an open-access article distributed under the terms of the Creative Commons Attribution License (CC BY). The use, distribution or reproduction in other forums is permitted, provided the original author(s) and the copyright owner(s) are credited and that the original publication in this journal is cited, in accordance with accepted academic practice. No use, distribution or reproduction is permitted which does not comply with these terms.
*Correspondence: Špela Bogataj, c3BlbGEuYm9nYXRhakBrY2xqLnNp
Disclaimer: All claims expressed in this article are solely those of the authors and do not necessarily represent those of their affiliated organizations, or those of the publisher, the editors and the reviewers. Any product that may be evaluated in this article or claim that may be made by its manufacturer is not guaranteed or endorsed by the publisher.
Research integrity at Frontiers
Learn more about the work of our research integrity team to safeguard the quality of each article we publish.