- 1School for Doctoral Studies and Research, Universidad Europea de Madrid, Madrid, Spain
- 2Research Group on Exercise Therapy and Functional Rehabilitation, Faculty of Sports Sciences, Universidad Europea de Madrid, Madrid, Spain
- 3Faculty of Physiotherapy and Nursing of Toledo, Universidad de Castilla-La Mancha, Toledo, Spain
- 4Department of Sport Sciences, Faculty of Sport Sciences, Universidad Europea de Madrid, Villaviciosa de Odón, Spain
- 5Physiotherapy Research Group of Toledo (GIFTO), Faculty of Physiotherapy and Nursing, Universidad de Castilla-La Mancha, Toledo, Spain
- 6Faculty of Health Sciences, Universidad Francisco de Vitoria, Madrid, Spain
- 7Department of Physiotherapy, Faculty of Sport Sciences, Universidad Europea de Madrid, Villaviciosa de Odón, Spain
- 8Clínica Sierra Varona SL, Toledo, Spain
- 9Department of Physical Therapy, Camilo José Cela University, Madrid, Spain
- 10Department of Physical Therapy, Universidad Alfonso X El Sabio, Villanueva de la Cañada, Spain
Introduction: Inspiratory muscle fatigue has been shown to have effects on the autonomic nervous system and physical condition. This study aimed to evaluate the influence of an inspiratory muscle fatigue protocol on respiratory muscle strength and heart rate variability in healthy youths.
Materials and Methods: A randomized controlled clinical trial, employing double-blinding, was conducted with twenty-seven participants aged 18–45 years, non-smokers and engaged in sports activity at least three times a week for a minimum of 1 year. Participants were randomly assigned to three groups: Inspiratory Muscle Fatigue group, Activation group, and Control group. Measurements of heart rate variability, diaphragmatic ultrasound, and maximum inspiratory pressure were taken at two stages: before the intervention and immediately after treatment.
Results: In our results with respect to baseline to post-treatment, the inspiratory muscle fatigue group showed lower values in the Sniff contraction velocity variable (10.96 cm/s ± 1.99–8.34 cm/s ± 1.23; p < 0.01) and higher values in the activation group (10.59 cm/s ± 0.89–12.66 cm/s ± 1.15; p < 0.01) with respect to the control group (10.27 cm/s ± 1.48–9.97 cm/s ± 1.42). On the other hand, the inspiratory muscle fatigue group showed higher values in the Low frequency variable (49.37 n.u. ± 13.91 to 69.48 n.u. ± 8.22; p < 0.01) and lower values in the activation group (57.92 n.u. ± 8.37 to 41.59 n.u. ± 11.21; p < 0.01) with respect to the control group (50.83 n.u. ± 17.30 to 52.10 n.u. ± 20.64). Additionally, significant correlations were found between respiratory variables and heart rate variability variables.
Conclusion: Acute fatigue of the inspiratory musculature appears to negatively impact heart rate variability and inspiratory muscle strength in healthy youths.
Clinical Trial Registration: https://clinicaltrials.gov/study/NCT06278714; Identifier: NCT06278714.
1 Introduction
Heart rate variability (HRV) quantifies the fluctuations in the time intervals between successive heartbeats, known as RR intervals, as recorded in electrocardiographic or heart rate analyses. This variability reflects the autonomic nervous system’s ability to regulate cardiac function and serves as a crucial indicator of homeostasis and the balance between the sympathetic and parasympathetic systems (Arakaki et al., 2023). Both branches have a significant influence on heart rate. The parasympathetic system, through the release of acetylcholine, decreases heart rate and increases HRV by slowing diastolic depolarization, while the sympathetic system, through the release of epinephrine and norepinephrine, increases heart rate and diastolic depolarization (Wehrwein et al., 2016). HRV is related to various variables such as executive function, decision-making, emotional regulation, and various pathological conditions such as dementia or stroke, among others (Arakaki et al., 2023). In the sports field, HRV is an important marker of exercise-induced fatigue (Ni et al., 2022), allowing for the control of training intensity and determining the adaptations produced by it (Al Haddad et al., 2009; Aubert et al., 2003), HRV can be used as a simple, non-invasive, and validated tool, playing a role in sports programming and recovery, as well as in monitoring internal load (Sanchez-Sanchez et al., 2021).
There is a significant relationship between the respiratory system and the cardiac system, where, for example, both the frequency and depth of breathing affect HRV, producing an increase in HRV and greater parasympathetic activation when breathing is slow and deep, and a decrease in HRV when breathing is rapid and shallow, with a consequent increase in sympathetic activity (Gasior et al., 2016). Regarding the respiratory muscles, particularly the diaphragm, there is also an interaction with the cardiac system, influencing the improvement of venous return, increasing HRV, and decreasing resting and recovery heart rate, due, among other factors, to the diaphragm’s relationship with vagal tone and the parasympathetic nervous system (Kocjan et al., 2017). In contrast, acute respiratory muscle fatigue can limit exercise capacity and have negative cardiovascular effects (Welch et al., 2018). This fatigue increases sympathetic activity, redistributes blood flow to the respiratory muscles, and increases cardiac output associated with these muscles, heightening the perception of effort and decreasing performance and exercise tolerance (Sheel et al., 2018; Romer and Polkey, 1985).
Various studies in the scientific literature have investigated the implication of respiratory muscle fatigue through resistive loads on cardiorespiratory condition (Welch et al., 2018; Smith et al., 2017). However, the analysis of HRV in relation to inspiratory muscle fatigue is poor or nonexistent. The hypothesis of this study is that acute inspiratory muscle fatigue protocol may have a negative effect on inspiratory muscle strength and heart rate variability, while activation of the inspiratory muscles has a positive impact on inspiratory muscle strength and heart rate variability. Consequently, given the multiple correlations and the physiological and athletic importance of this variable, the objective of our study was to objectively evaluate the relationship between acute inspiratory muscle fatigue, inspiratory muscle strength, and HRV.
2 Materials and methods
2.1 Study design
This study utilized a randomized parallel clinical trial design and was conducted at the Physiotherapy Laboratory of the University of Castilla La Mancha (Toledo, Spain), in accordance with the Consolidated Standards of Reporting Trials (CONSORT) guidelines (Cuschieri, 2019). Informed consent was obtained from all participants. The study was conducted in accordance with the Declaration of Helsinki and approved by the Research Ethics Committee of the Complejo Hospitalario Universitario de Toledo (ID: 1070), and was registered on ClinicalTrials.gov (NCT06278714).
2.2 Participants
Using the randomization.com application, twenty-seven young, healthy participants were enlisted in the research and allocated randomly. Three groups of participants were formed: the activation group (AG), the control group (CG), and the inspiratory muscle fatigue group (IMFG). The person who performed this randomization did not take part in the study. The group assignments of the participants were concealed from the assessor and the data analyzer. Individuals between the ages of 18 and 45 who did not smoke and had played sports at least three times a week for a minimum of 1 year met the inclusion criteria. Any medical condition that precluded physical activity, cognitive impairments, chronic diseases (cardiorespiratory, neurological, metabolic, oncological, etc.), middle-inner ear pathology or tympanic membrane perforation, lower limb surgery within the previous year, and individuals experiencing an active episode of lower limb pain were among the exclusion criteria. G*Power Software (version 3.1.9.2) was used to determine the sample size based on maximum inspiratory pressure (MIP) ratings that were acquired from a previous investigation (Holtzhausen et al., 2018). Based on a medium effect size (f = 0.25 or partial Eta squared = 0.06) and alpha and beta errors of 0.05 and 0.2, respectively, the computations were performed. Because of the research design, a dropout rate of fourty percent was expected; therefore, a total sample size of twenty-seven participants, split into three groups (n = 9), was needed.
2.3 Intervention
Using a threshold valve device (Big Breathe®; GH Innotek Co., Ltd., Busan, Republic of Korea), the IMFG undertook a program designed to produce inspiratory muscle fatigue. Subjects breathed against submaximal inspiratory loads of 60% of their maximal inspiratory pressure (MIP) until not being capable to produce airflow in at least three consecutive maximum inspiratory attempts (Welch et al., 2018). Using the same threshold device used by the IMFG, the AG performed two sets of thirty repetitions at 40% of their MIP, in accordance with a previous study (Ozdal, 2016). Conversely, there was no intervention provided to the CG. For 10 min that the intervention and activation groups needed to complete their procedure, the participants in this cohort remained sitting.
2.4 Outcomes
MIP measurements, diaphragmatic ultrasound, and heart rate variability assessments were conducted at two different time points: pre-intervention (T1) and immediately post-intervention (T2).
2.5 Primary outcomes
2.5.1 Maximal inspiratory pressure
Participants in a sitting posture were assessed for MIP using the MicroRPM® Respiratory Pressure Measurement Device (MicroMedical, United Kingdom). In order to guarantee ventilation through the mouth, nasal passageways were blocked. The participants could only complete up to six maneuvers in a single try, with a 1-minute break in between. Out of three attempts, the highest consistently repeatable result with less than 5% fluctuation was found (Laveneziana et al., 2019).
2.5.2 Diaphragmatic thickness and thickening fraction
The diaphragmatic thickness was measured with an ultrasonic linear probe (L13-3s) operating in the 3.2–12.3 MHz frequency range. The subjects were laying supine, and the probe was placed perpendicular to the chest wall. Measurements were made between the eighth and ninth intercostal gaps at two particular anatomical landmarks: the anterior and mid-axillary lines. In the juxtaposed region, the diaphragm was seen using B-mode ultrasonography (Figures 1A, B). The thickness was measured three times at peak inspiration (Thickinsp) and end of expiration (Thickexp), and the mean results were noted. The baseline was determined to be the diaphragmatic thickness at the conclusion of expiration. The thickening fraction (TF%) was computed using the following formula: Thickness at the end of maximal inspiration - Thickness at the end of expiration)/Thickness at the end equals TF (Santana et al., 2020).
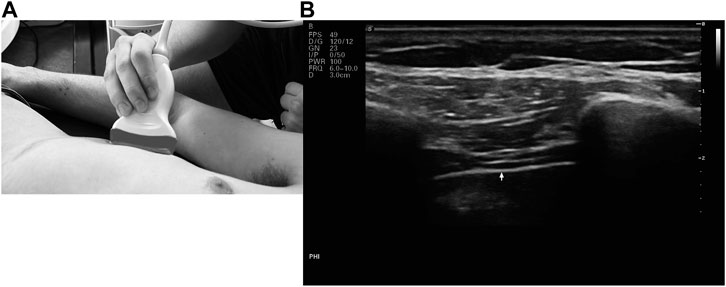
Figure 1. (A) Placement of the linear probe on the rib cage (B) B-mode ultrasound image of the diaphragm.
2.5.3 Diaphragmatic movement curve
The diaphragmatic movement was evaluated using a convex probe (C5-1s) that ran at 1.2–6 MHz. The liver served as an acoustic window for the probe, which was positioned longitudinally at the right costal margin along the mid-clavicular line. The subjects were lying supine, and the probe was facing the subject’s head. The diaphragmatic movement curve during maximum deep breathing and sniff breathing was recorded using M-mode imaging (Figure 2). For every kind of breathing, parameters such maximal contraction velocity (Velinsp and Velsniff), inspiratory time (Timeinsp and Timesniff), and diaphragmatic excursion (Mobinsp and Mobsniff) were examined. The average value for each parameter was collected after three consecutive breathing cycles were analyzed (Santana et al., 2020).
2.6 Secondary outcomes
2.6.1 Heart rate variability
A heart rate monitor (Polar H10; Polar Electro Oy, Kempele, Finland) was used to analyze HRV. Participants were placed supine on a stretcher in a quiet room with gentle lighting and a temperature of around 25°C. For 3 minutes, cardiac electrical signals were recorded using a chest band. Participants were told not to consume coffee, alcohol, tobacco, or engage in vigorous physical activity for 12 h prior to the intervention. They were also instructed not to speak and not to move voluntarily while the analysis was being conducted (Schaffarczyk et al., 2022). All measurements were taken during the same time frame, between 9 and 11 a.m. Kubios HRV Analysis Software 3.1.0 for Windows (Biomedical Signal and Medical Imaging Analysis Group, Department of Applied Physics, University of Kuopio, Finland) was utilized for the analysis. Six features were examined: the sympathovagal balance index (LF/HF), power in both low frequency (LF; 0.04–0.15 Hz) and high frequency (HF; 0.15–0.40 Hz), both in normalized units (nu), the standard deviation of all normal-to-normal intervals (SDNN), and the square root of the mean of the sum of squared differences between adjacent normal-to-normal intervals (RMSSD).
2.7 Statistical analysis
IBM SPSS Statistics v.22.0 was used for the statistical analysis. A significant criterion of p < 0.05 was used. The Kolmogorov-Smirnov test helped to determine each variable’s normality, and the results showed that all of the variables had a normal distribution. To examine the demographic features, descriptive statistics were utilized, and the measurements were reported as mean ± SD. For the outcome variables, a 2-way repeated measures ANOVA was used to analyze the interaction between the Experimental group, Activation group, Control group, and the assessment time (Baseline, Post-treatment). When differences were found, post hoc Bonferroni multiple comparisons testing were used. Pearson’s correlation coefficient was used to ascertain the relationship between respiratory variables (MIP, Thickinsp and Mobsniff) and between HRV variables (RR, SDNN, LF, HF, LF/HF and RMSSD). Cohen’s scale (Cohen, 1969) was utilized to evaluate effect size (ES), which was classified as low (<0.20), medium (0.50), and high (>0.80).
3 Results
3.1 Demographic data
Twenty-seven adults were recruited for the study on March 2024 and participate between May and June 2024. They were distributed among IMFG (4-men, 5-women), AG (5-men, 4-women) and CG (4-men, 5-women). There were no dropouts due to the intervention or measurements. The CONSORT flow chart was included (Figure 3). No significant differences were found between IMFG, AG and CG in demographic characteristics (Table 1). Pearson’s correlation revealed a significant association between respiratory variables and HRV variables. The results of this correlation are shown in Table 2.
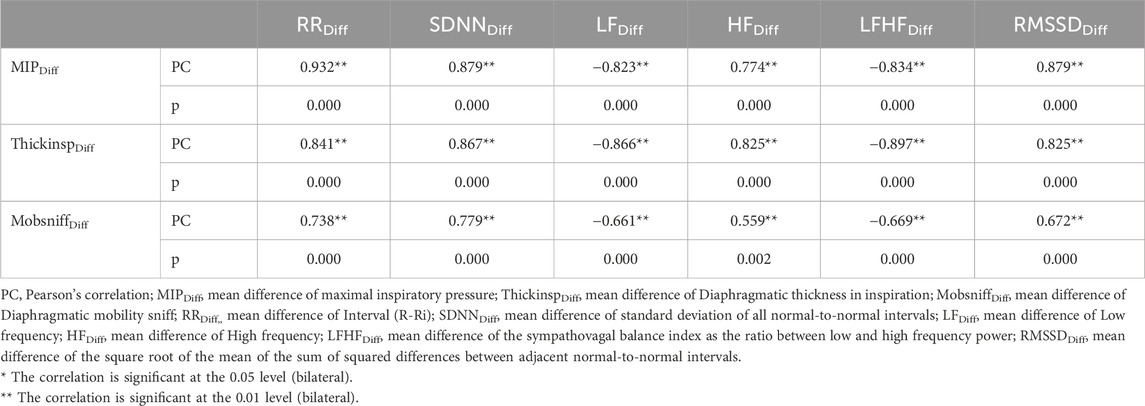
Table 2. Pearson’s correlation between respiratory variables and between heart rate variability variables.
3.2 Changes in respiratory variables
Results for primary outcomes are presented in Table 3.
In the analysis of the MIP variable, the IMFG analysis, there was a decreased between baseline and post-treatment of −8.89 ± 1.54 cmH2O (p < 0.01; ES = 0.63; 95% CI of the difference = −9.97 to −7.81). In contrast, the AG showed an increased between baseline and post-treatment of 2.89 ± 1.96 cmH2O (p < 0.01; ES = 0.15; 95% CI of the difference = 1.81–3.97). In the analysis of the Thickinsp variable, the IMFG analysis, there was a decreased between baseline and post-treatment of −0.03 ± 0.01 cm (p < 0.01; ES = 0.43; 95% CI of the difference = −0.04 to −0.03). In contrast, the AG showed an increased between baseline and post-treatment of 0.02 ± 0.01 cm (p < 0.01; ES = 0.24; 95% CI of the difference = 0.00–0.02). In the analysis of the Mobsniff variable, the IMFG had lower values than the CG (p < 0.01), and the AG had higher values compared to the CG after performing the treatment (p < 0.01). Within the IMFG analysis, there was a decreased between baseline and post-treatment of −1.01 ± 0.21 cm (p < 0.01; ES = 2.29; 95% CI of the difference = −1.10 to −0.91). In contrast, the AG showed an increased between baseline and post-treatment of 0.49 ± 0.10 cm (p < 0.01; ES = 1.43; 95% CI of the difference = 0.39–0.58).
3.3 Changes in heart rate variability
Results for primary outcomes are presented in Table 4.
In the analysis of the LF variable, the IMFG had higher values than the AG and CG after performing the treatment (p < 0.05). Within the IMFG analysis, there was a increased between baseline and post-treatment of 20.11% ± 13.64% (p < 0.01; ES = 1.45; 95% CI of the difference = 13.67–26.56). In contrast, the AG showed a decreased between baseline and post-treatment of −16.32% ± 7.37% (p < 0.01; ES = 1.95; 95% CI of the difference = −22.77 to −9.88). In the analysis of the HF variable, the IMFG had lower values than the AG and CG after performing the treatment (p < 0.05). Within the IMFG analysis, there was a increased between baseline and post-treatment of −21.95% ± 13.75% (p < 0.01; ES = 1.60; 95% CI of the difference = −28.96 to −14.94). In contrast, the AG showed an increased between baseline and post-treatment of 7.75% ± 9.89% (p < 0.05; ES = 0.59; 95% CI of the difference = 0.74–14.75).
4 Discussion
The results of this research suggest a decrease in inspiratory muscle strength, diaphragm thickness and mobility, as well as an increase in sympathetic activity in the acute inspiratory muscle fatigue group. Conversely, the results obtained in the AG suggest an improvement in inspiratory muscle strength, diaphragm thickness and mobility, in addition to an increase in parasympathetic activity. Again, it should be noted that the results shown in this study are driven by an acute intervention and therefore aim to demonstrate or help understand the acute responses of inspiratory muscle fatigue and inspiratory muscle activation in relation to HRV.
As previously mentioned, acute inspiratory muscle fatigue can condition exercise capacity and performance through various pathways, including neurological, cardiovascular, and metabolic (Romer and Polkey, 1985), and is more prevalent in clinical populations than in healthy subjects (Matecki et al., 2001). Through fatigue stimulation, it triggers peripheral vasoconstriction induced by an increase in sympathetic reflex activity, producing a redistribution of blood flow from the peripheral muscles to the respiratory muscles to maintain ventilatory function based on the required demand (Sheel et al., 2018). The assessment of respiratory muscle strength can be measured through different tools. Measuring MIP is a validated and evidenced method for evaluating inspiratory muscle strength, using portable pressure meters and maneuvers that are easy to learn and tolerate for patients (Laveneziana et al., 2019; Volianitis et al., 2001), and is correlated with transdiaphragmatic pressure in healthy subject (McCool et al., 1997). A moreover, the improvement of this variable is associated with enhanced athletic performance and is related to variables such as maximum oxygen consumption and lactate (HajGhanbari et al., 2013; Fernandez-Lazaro et al., 2021). As for diaphragmatic ultrasound, it is a non-invasive and validated test for assessing diaphragmatic strength and mobility (Zhou et al., 2023), being a reproducible and effective technique in healthy subjects (Wilches-Luna et al., 2022; Scarlata et al., 2019) and correlated with other respiratory variables such as inspiratory muscle strength and transdiaphragmatic pressure (Koco et al., 2021) as well as with the contractile efficiency of these muscles (Sarwal et al., 2013). Regarding our study, a decrease in MIP and various ultrasound variables can be observed in the IMFG, suggesting that the members of this group achieved a status of inspiratory muscle fatigue. These results are consistent with those obtained in previous studies using similar protocols with threshold devices in subjects without pathology (Welch et al., 2018; Smith et al., 2017).
The autonomic nervous system (ANS), as a component of the central nervous system, plays an important physiological role in maintaining homeostasis and regulating various processes, including cardiac function (Duraes Campos et al., 2018). It has two main branches: the parasympathetic system, which decreases heart rate and increases HRV, and the sympathetic system, which increases heart rate and decreases HRV. HRV is an effective method for assessing the ANS, relating to the parasympathetic system through variables such as HF and RMSSD, to the sympathetic system through LF, and to the sympathetic-vagal balance through LF/HF (Perini and Veicsteinas, 2003). In sports, HRV is increasingly used as a mechanism for monitoring internal load due to its ease of use and validity (Sanchez-Sanchez et al., 2021), allowing for quantification of stress, adaptations produced by training, overtraining, fatigue, and recovery capacity in athletes (Lundstrom et al., 2023). It should be noted that, in many cases, lower HRV values may indicate good fitness, as the body exhibits greater arousal and readiness for physical activity (DeBlauw et al., 2023). However, if the inspiratory muscles are weak or fatigued, this sympathetic activation, through the metaboreflex, may induce negative effects that limit athletic performance by reducing blood flow to the extremities, muscle oxygen saturation, or jump performance, among other factors (St Croix et al., 2000; Ladrinan-Maestro et al., 2024). There is an interaction between the respiratory and cardiac systems known as respiratory sinus arrhythmia (RSA), wherein slower and deeper breaths increase RSA, decrease heart rate, and increase HRV (Denver et al., 2007). Based on our results, there appears to be an acute decrease in parasympathetic activity in the IMFG, evidenced by lower HF and RMSSD values, while sympathetic activity increases, shown by higher LF values and decreased RR interval and SDNN, as well as a significant correlation between respiratory variables and HRV variables. This situation can be hypothesized by the aforementioned metaboreflex and the cardiorespiratory interaction mediated by RSA. Increases in sympathetic predominance in athletes can lead to fatigue and overtraining, as well as hormonal imbalances resulting in higher concentrations of stress substances such as cortisol and catecholamines (Clark and Mach, 2016). These results are consistent with another previous and similar study, where acute inspiratory muscle fatigue had negative cardiac effects, increasing blood pressure and heart rate (Welch et al., 2018). In contrast, in the AG, there is an acute improvement in parasympathetic status with higher HF and RMSSD values, a better sympathetic-vagal balance with increased LF/HF, and a decrease in sympathetic activity through lower LF values. Greater parasympathetic predominance can favor sports recovery processes, reducing cortisol levels, improving sleep, and promoting tissue regeneration and recovery processes, thus leading to better training adaptation (Casanova-Lizon et al., 2022). These results align with previous studies where healthy subjects underwent only one session of inspiratory muscle training, resulting in an acute increased parasympathetic activity and sympathetic-vagal balance (Tanriverdi et al., 2021; Rodrigues et al., 2021).
The results obtained in this study may have several interesting practical applications in the respiratory and sports fields. On one hand, the evidence regarding the consequences of acute inspiratory muscle fatigue in young athletes highlights the importance of training these muscles to avoid this negative impact on the autonomic level through sympathetic hyperactivation. On the other hand, the inclusion of an inspiratory muscle activation protocol seems to have potential benefits when applied before sports activities, due to its effects on respiratory muscle strength and HRV.
This study presents several limitations that should be noted. Firstly, although devices like the one used in this study for HRV measurement are validated, the most objective option for measuring HRV remains the electrocardiogram. Similarly, the most objective measurements of respiratory strength are transdiaphragmatic and esophageal pressures. Secondly, these data should be interpreted with caution as they were obtained through an acute intervention with short-term effects. Lastly, certain aspects that could be relevant were not considered, such as fasting vs. postprandial state, chronic caffeine or alcohol consumption, menstrual cycle in women, and dietary control. Therefore, future studies with larger samples and longitudinal measurements are needed to corroborate these results and gather more information as well as explore the different effects of these interventions on amateur and professional athletes, in order to demonstrate how respiratory muscle fatigue and activation affect performance depending on the level of training, as well as to analyze the differences between men and women. The results of this study may serve as a starting point for future research that provides more evidence about the involvement of the respiratory system in sports performance and the central nervous system.
In conclusion, acute inspiratory muscle fatigue appears to have a negative impact on HRV, increasing sympathetic activity and reducing parasympathetic activity, as well as disrupting the sympathetic-vagal balance. On the other hand, activation of the inspiratory muscles seems to improve the sympathetic-vagal balance, enhance parasympathetic activation, and reduce sympathetic activity in healthy young subjects. Further research is necessary to provide more evidence on this topic.
Data availability statement
The raw data supporting the conclusions of this article will be made available by the authors, without undue reservation.
Ethics statement
The studies involving humans were approved by Research Ethics Committee of the Complejo Hospitalario Universitario de Toledo. Toledo (Spain). The studies were conducted in accordance with the local legislation and institutional requirements. The participants provided their written informed consent to participate in this study.
Author contributions
AL-M: Conceptualization, Data curation, Investigation, Methodology, Project administration, Resources, Supervision, Validation, Visualization, Writing–original draft, Writing–review and editing. JS-I: Data curation, Formal Analysis, Investigation, Methodology, Software, Validation, Writing–review and editing. DM-V: Investigation, Methodology, Supervision, Validation, Visualization, Writing–review and editing, Data curation, Resources. AS-S: Investigation, Methodology, Supervision, Validation, Visualization, Writing–review and editing, Conceptualization, Writing–original draft.
Funding
The authors declare that no financial support was received for the research, authorship, and/or publication of this article.
Conflict of interest
The authors declare that the research was conducted in the absence of any commercial or financial relationships that could be construed as a potential conflict of interest.
Publisher’s note
All claims expressed in this article are solely those of the authors and do not necessarily represent those of their affiliated organizations, or those of the publisher, the editors and the reviewers. Any product that may be evaluated in this article, or claim that may be made by its manufacturer, is not guaranteed or endorsed by the publisher.
References
Al Haddad H., Laursen P. B., Ahmaidi S., Buchheit M. (2009). Nocturnal heart rate variability following supramaximal intermittent exercise. Int. J. Sports Physiol. Perform. 4 (4), 435–447. doi:10.1123/ijspp.4.4.435
Arakaki X., Arechavala R. J., Choy E. H., Bautista J., Bliss B., Molloy C., et al. (2023). The connection between heart rate variability (HRV), neurological health, and cognition: a literature review. Front. Neurosci. 17, 1055445. doi:10.3389/fnins.2023.1055445
Aubert A. E., Seps B., Beckers F. (2003). Heart rate variability in athletes. Sports Med. 33 (12), 889–919. doi:10.2165/00007256-200333120-00003
Casanova-Lizon A., Manresa-Rocamora A., Flatt A. A., Sarabia J. M., Moya-Ramon M. (2022). Does exercise training improve cardiac-parasympathetic nervous system activity in sedentary people? A systematic review with meta-analysis. Int. J. Environ. Res. Public Health 19 (21), 13899. doi:10.3390/ijerph192113899
Clark A., Mach N. (2016). Exercise-induced stress behavior, gut-microbiota-brain axis and diet: a systematic review for athletes. J. Int. Soc. Sports Nutr. 13, 43. doi:10.1186/s12970-016-0155-6
Cohen J. Statistical power analysis for the behavioral sciences. New York: Academic Press; 1969, 415 p.
Cuschieri S. (2019). The CONSORT statement. Saudi J. Anaesth. 13 (Suppl. 1), S27-S30–S30. doi:10.4103/sja.SJA_559_18
DeBlauw J. A., Stein J. A., Blackman C., Haas M., Makle S., Echevarria I., et al. (2023). Heart rate variability of elite female rowers in preparation for and during the national selection regattas: a pilot study on the relation to on water performance. Front. Sports Act. Living 5, 1245788. doi:10.3389/fspor.2023.1245788
Denver J. W., Reed S. F., Porges S. W. (2007). Methodological issues in the quantification of respiratory sinus arrhythmia. Biol. Psychol. 74 (2), 286–294. doi:10.1016/j.biopsycho.2005.09.005
Duraes Campos I., Pinto V., Sousa N., Pereira V. H. (2018). A brain within the heart: a review on the intracardiac nervous system. J. Mol. Cell Cardiol. 119, 1–9. doi:10.1016/j.yjmcc.2018.04.005
Fernandez-Lazaro D., Gallego-Gallego D., Corchete L. A., Fernandez Zoppino D., Gonzalez-Bernal J. J., Garcia Gomez B., et al. (2021). Inspiratory muscle training program using the PowerBreath (R): does it have ergogenic potential for respiratory and/or athletic performance? A systematic review with meta-analysis. Int. J. Environ. Res. Public Health 18 (13), 6703. doi:10.3390/ijerph18136703
Gasior J. S., Sacha J., Jelen P. J., Zielinski J., Przybylski J. (2016). Heart rate and respiratory rate influence on heart rate variability repeatability: effects of the correction for the prevailing heart rate. Front. Physiol. 7, 356. doi:10.3389/fphys.2016.00356
HajGhanbari B., Yamabayashi C., Buna T. R., Coelho J. D., Freedman K. D., Morton T. A., et al. (2013). Effects of respiratory muscle training on performance in athletes: a systematic review with meta-analyses. J. Strength Cond. Res. 27 (6), 1643–1663. doi:10.1519/JSC.0b013e318269f73f
Holtzhausen S., Unger M., Lupton-Smith A., Hanekom S. (2018). An investigation into the use of ultrasound as a surrogate measure of diaphragm function. Heart Lung 47 (4), 418–424. doi:10.1016/j.hrtlng.2018.04.010
Kocjan J., Adamek M., Gzik-Zroska B., Czyzewski D., Rydel M. (2017). Network of breathing. Multifunctional role of the diaphragm: a review. Adv. Respir. Med. 85 (4), 224–232. doi:10.5603/ARM.2017.0037
Koco E., Soilemezi E., Sotiriou P., Savvidou S., Tsagourias M., Pnevmatikos I., et al. (2021). Ultrasonographic assessment of diaphragmatic contraction and relaxation properties: correlations of diaphragmatic displacement with oesophageal and transdiaphragmatic pressure. BMJ Open Respir. Res. 8 (1), e001006. doi:10.1136/bmjresp-2021-001006
Ladrinan-Maestro A., Sanchez-Infante J., Martin-Vera D., Sanchez-Sierra A. (2024). Influence of an inspiratory muscle fatigue protocol on healthy youths on respiratory muscle strength, vertical jump performance and muscle oxygen saturation: a randomized controlled trial. J. Transl. Med. 22 (1), 732. doi:10.1186/s12967-024-05555-3
Laveneziana P., Albuquerque A., Aliverti A., Babb T., Barreiro E., Dres M., et al. (2019). ERS statement on respiratory muscle testing at rest and during exercise. Eur. Respir. J. 53 (6), 1801214. doi:10.1183/13993003.01214-2018
Lundstrom C. J., Foreman N. A., Biltz G. (2023). Practices and applications of heart rate variability monitoring in endurance athletes. Int. J. Sports Med. 44 (1), 9–19. doi:10.1055/a-1864-9726
Matecki S., Topin N., Hayot M., Rivier F., Echenne B., Prefaut C., et al. (2001). A standardized method for the evaluation of respiratory muscle endurance in patients with Duchenne muscular dystrophy. Neuromuscul. Disord. 11 (2), 171–177. doi:10.1016/s0960-8966(00)00179-6
McCool F. D., Conomos P., Benditt J. O., Cohn D., Sherman C. B., Hoppin F. G. (1997). Maximal inspiratory pressures and dimensions of the diaphragm. Am. J. Respir. Crit. Care Med. 155 (4), 1329–1334. doi:10.1164/ajrccm.155.4.9105075
Ni Z., Sun F., Li Y. (2022). Heart rate variability-based subjective physical fatigue assessment. Sensors (Basel) 22 (9), 3199. doi:10.3390/s22093199
Ozdal M. (2016). Acute effects of inspiratory muscle warm-up on pulmonary function in healthy subjects. Respir. Physiol. Neurobiol. 227, 23–26. doi:10.1016/j.resp.2016.02.006
Perini R., Veicsteinas A. (2003). Heart rate variability and autonomic activity at rest and during exercise in various physiological conditions. Eur. J. Appl. Physiol. 90 (3-4), 317–325. doi:10.1007/s00421-003-0953-9
Rodrigues G. D., Gurgel J. L., Goncalves T. R., da Silva Soares P. P. (2021). Acute effects of inspiratory loading in older women: where the breath meets the heart. Respir. Physiol. Neurobiol. 285, 103589. doi:10.1016/j.resp.2020.103589
Romer L. M., Polkey M. I. (1985). Exercise-induced respiratory muscle fatigue: implications for performance. J. Appl. Physiol. 104 (3), 879–888. doi:10.1152/japplphysiol.01157.2007
Sanchez-Sanchez J., Botella J., Felipe Hernandez J. L., Leon M., Paredes-Hernandez V., Colino E., et al. (2021). Heart rate variability and physical demands of in-season youth elite soccer players. Int. J. Environ. Res. Public Health 18 (4), 1391. doi:10.3390/ijerph18041391
Santana P. V., Cardenas L. Z., Albuquerque A. L. P., Carvalho C. R. R., Caruso P. (2020). Diaphragmatic ultrasound: a review of its methodological aspects and clinical uses. J. Bras. Pneumol. 46 (6), e20200064. doi:10.36416/1806-3756/e20200064
Sarwal A., Walker F. O., Cartwright M. S. (2013). Neuromuscular ultrasound for evaluation of the diaphragm. Muscle Nerve 47 (3), 319–329. doi:10.1002/mus.23671
Scarlata S., Mancini D., Laudisio A., Raffaele A. I. (2019). Reproducibility of diaphragmatic thickness measured by M-mode ultrasonography in healthy volunteers. Respir. Physiol. Neurobiol. 260, 58–62. doi:10.1016/j.resp.2018.12.004
Schaffarczyk M., Rogers B., Reer R., Gronwald T. (2022). Validity of the polar H10 sensor for heart rate variability analysis during resting state and incremental exercise in recreational men and women. Sensors (Basel) 22 (17), 6536. doi:10.3390/s22176536
Sheel A. W., Boushel R., Dempsey J. A. (2018). Competition for blood flow distribution between respiratory and locomotor muscles: implications for muscle fatigue. J. Appl. Physiol. (1985) 125 (3), 820–831. doi:10.1152/japplphysiol.00189.2018
Smith J. R., Alexander A. M., Hammer S. M., Didier K. D., Kurti S. P., Broxterman R. M., et al. (2017). Cardiovascular consequences of the inspiratory muscle metaboreflex: effects of age and sex. Am. J. Physiol. Heart Circ. Physiol. 312 (5), H1013-H1020–H20. doi:10.1152/ajpheart.00818.2016
St Croix C. M., Morgan B. J., Wetter T. J., Dempsey J. A. (2000). Fatiguing inspiratory muscle work causes reflex sympathetic activation in humans. J. Physiol. 529 (Pt 2), 493–504. doi:10.1111/j.1469-7793.2000.00493.x
Tanriverdi A., Kahraman B. O., Ozsoy I., Ozpelit E., Savci S. (2021). Acute effects of inspiratory muscle training at different intensities in healthy young people. Ir. J. Med. Sci. 190 (2), 577–585. doi:10.1007/s11845-020-02353-w
Volianitis S., McConnell A. K., Koutedakis Y., McNaughton L., Backx K., Jones D. A. (2001). Inspiratory muscle training improves rowing performance. Med. Sci. Sports Exerc 33 (5), 803–809. doi:10.1097/00005768-200105000-00020
Wehrwein E. A., Orer H. S., Barman S. M. (2016). Overview of the anatomy, physiology, and pharmacology of the autonomic nervous system. Compr. Physiol. 6 (3), 1239–1278. doi:10.1002/cphy.c150037
Welch J. F., Archiza B., Guenette J. A., West C. R., Sheel A. W. (2018). Sex differences in diaphragmatic fatigue: the cardiovascular response to inspiratory resistance. J. Physiol. 596 (17), 4017–4032. doi:10.1113/JP275794
Wilches-Luna E. C., Pabon-Munoz F. E., Arias-Campo J. M., Caballero-Lozada A. F. (2022). Inter-rater reliability of the measurement of diaphragmatic excursion and fraction of diaphragmatic thickening by ultrasonography in healthy volunteers. Rev. Esp. Anestesiol. Reanim. Engl. Ed. 69 (9), 536–543. doi:10.1016/j.redare.2021.06.003
Zhou E. F., Fu S. N., Huang C., Huang X. P., Wong A. Y. L. (2023). Reliability and validity of ultrasonography in evaluating the thickness, excursion, stiffness, and strain rate of respiratory muscles in non-hospitalized individuals: a systematic review. BMC Oral Health 23 (1), 959. doi:10.1186/s12903-023-03558-y
Keywords: inspiratory muscle fatigue, sport, autonomic cardiac control, heart rate variability, respiratory muscle strength
Citation: Ladriñán-Maestro A, Sánchez-Infante J, Martín-Vera D and Sánchez-Sierra A (2024) Influence of an inspiratory muscle fatigue protocol on healthy youths on respiratory muscle strength and heart rate variability. A randomized controlled trial. Front. Physiol. 15:1457019. doi: 10.3389/fphys.2024.1457019
Received: 29 June 2024; Accepted: 14 August 2024;
Published: 23 August 2024.
Edited by:
Steffen Schulz, Charité University Medicine Berlin, GermanyReviewed by:
Alain Boussana, Marien Ngouabi University, Republic of CongoStephen Ives, Skidmore College, United States
Copyright © 2024 Ladriñán-Maestro, Sánchez-Infante, Martín-Vera and Sánchez-Sierra. This is an open-access article distributed under the terms of the Creative Commons Attribution License (CC BY). The use, distribution or reproduction in other forums is permitted, provided the original author(s) and the copyright owner(s) are credited and that the original publication in this journal is cited, in accordance with accepted academic practice. No use, distribution or reproduction is permitted which does not comply with these terms.
*Correspondence: Alberto Sánchez-Sierra, alberto.sanchez@universidadeuropea.es