- 1Zoology Department, Faculty of Science, Assiut University, Assiut, Egypt
- 2Molecular Biology Research & Studies Institute, Assiut University, Assiut, Egypt
Despite numerous studies on microplastics, the biological impacts of polypropylene microplastics (PP-MPs) and its toxicity on freshwater fish have yet to be fully revealed. The purpose of this research was to look at the potentially harmful effects of PP-MPs in freshwater African catfish Clarias gariepinus and bioremediation using Spirulina. After acclimatization to laboratory conditions, 108 fish (125 ± 3 gm and 27 ± 2 cm) were assigned into triplicate six experimental groups (12 fish/group), a control group, Spirulina group (SP), PP-MP-treated groups (0.14 and 0.28 mg/l PP-MPs), and PP-MP + Spirulina-treated groups (0.14 mg/l PP-MPs + 200 mg/L SP and 0.28 mg/l PP-MPs +200 mg/L SP) for 15-day exposure and 45-day recovery after that. The hematological parameters exhibiting significance (RBCs, Hct, Hb, and MCV) or non-significance (MCH and MCHC) either decreased with the increase in PP-MP doses from 0.0 in the control to 0.28 mg/L red blood cells (RBCs), hematocrit (Hct), mean corpuscular hemoglobin (MCH), mean corpuscular hemoglobin concentration (MCHC), hemoglobin (Hb) and platelets or increased with such an increase in doses (mean corpuscular volume (MCV)). The liver enzyme activity, aspartate aminotransferase (AST), alkaline phosphatase (ALP), and alanine aminotransferase (ALT) exhibited non-significant (p ≥ 0.05) or significant (p < 0.05) increases in (0.14 and 0.28 mg/L) PP-MP-exposed groups, respectively, except ALP. Furthermore, there was a significant (p < 0.05) or non-significant (p ≥ 0.05) increase in 0.14 and 0.28 mg/l PP-MP +200 mg/L-exposure groups, respectively, compared to the control group and the same exposure group without Spirulina. In comparison to the control group, PP-MPs (0.14 and 0.28 mg/L) induced a significant (p < 0.05) increase in the percentage of poikilocytosis and nuclear abnormalities of RBCs. The liver tissue from fish exposed to PP-MPs exhibited varying degrees of pathological changes. These results indicated that these pathological changes increased with PP-MP concentration, suggesting that the effect of PP-MPs was dose-dependent. After 45 days of recovery under normal conditions, it was obvious that there was a significant improvement in the percentage of poikilocytosis and nuclear abnormalities of RBCs, as well as a non-significant improvement in hemato-biochemical parameters and liver tissue.
1 Introduction
Plastic debris is a significant concern to marine and freshwater ecosystems, acting as a vector for microorganisms (Lobelle and Cunliffe, 2011), a source of gastrointestinal tract (GIT) blockage (Jabeen et al., 2018), and a cause of death (Setälä et al., 2014), eventually affecting humans. Plastic polymers of many different types are produced and released into the environment. In Europe, polyethylene (PE) accounted for 28% of the total output, with PP accounting for 19%, polyvinylchloride (PVC) accounting for 10%, and polystyrene accounting for 7% (Europe, 2017). Considering that PP is the second-most popular plastic resin in terms of manufacturing volume in Europe and worldwide, this is surprising (Statista, 2021), and PP is among the most frequently discovered microplastics in the environment (Peng et al., 2020). PP is a suitable thermoplastic polymer with excellent qualities such as dimensional solidity, fire resistance, simplicity, and a high thermal distortion temperature (Alsabri et al., 2022). PP accounts for 16% of the global plastics industry and is frequently used to manufacture end products such as plastic packaging for customers (Bora et al., 2020). PP has potential applications in the areas of powder technology, composite biotechnology, catalysis, optoelectronics, wastewater treatment (Pankhania et al., 1994), surface coating technology (Huang et al., 2007), flame retardants (Shao et al., 2014), food packaging (Ramos et al., 2012), medical purposes (Martakis et al., 1994), and toys (Specht, 2011). PP is primarily integrated in these goods by molding or extrusion procedures (Zhang and Horrocks, 2003).
According to De Sá et al. (2018), PP was included in just 12.1% of the 157 peer-reviewed ecotoxicity studies published in 2018 involving 612 different microplastics on aquatic species. However, it can be concluded that both PP fibers and fragments at levels observed in the environment have the potential to harm organisms (Horn et al., 2020; Kim et al., 2020). PP was discovered in 1954, and because it has the lowest density among plastics, it was soon adopted (Andrady and Neal, 2009). It is classified as a thermoplastic because, when heated to its melting point, it transforms into a liquid (Papageorgiou et al., 2015). PP is regarded as one of the most promising commodities due to its physical qualities, versatility, and ecologically benign record as a thermoplastic polymer (Ramesh and Vinodh, 2020). PP sales are expected to increase at an annual pace of 6.3% between 2013 and 2019 (Papageorgiou et al., 2015). Furthermore, by 2019, PP revenues are predicted to exceed $124.01 billion, with a 6.3% annual growth rate (Papageorgiou et al., 2015). According to Thummabancha et al. (2016), PP can be classified into three categories depending on its physical and chemical properties: homopolymer (HPP) is semi-crystalline and composed of just propylene monomers; random copolymer (RCP) comprises ethylene as a co-monomer in the PP chains at levels between 1% and 8%; and finally, impact copolymer (ICP) incorporates a 45%–65% ethylene co-mixed RCP phase. In the plastics industry, HPP is the most often used PP. According to one study, the market share for various types of PP is as follows: HPP 65%–75%, ICP 20%–30%, and RCP 5%–10% (Maddah, 2016).
It is known that according to Food and Drug Administration (FDA) guidelines, PP particles are safe polymers (Haya and Maher, 2013); however, other research studies show that heat (Song et al., 2003) and propylene gas (Quest et al., 1984) are the primary reasons for their toxicity. Despite the fact that much research has been undertaken to evaluate propylene gas-related toxicity, there are few studies that estimate the direct toxicity of PP particles (Bobori et al., 2022). Some studies have suggested that the potential toxicity of microplastic particles, such as hemolytic effects on red blood cells (RBCs), can be controlled by concentration, exposure period, shape, and surface charge (Hamed et al., 2019a; Hwang et al., 2019; Sayed et al., 2021a; Hamed et al., 2022; Soliman et al., 2023). As a result, Hwang et al. (2019) investigated RBC hemolysis following direct contact with PP particles of varying sizes (Mazzaglia et al., 2018). PP have some negative effects such as gut retention, reduced development and eating rates, changed metabolic rates and processes, alterations to the molt process, reduced reproduction, immune response induction, oxidative stress and antioxidant responses, altered gut microbiota, and (very rarely) mortality (De Sá et al., 2018; do Nascimento et al., 2023; Jeyavani et al., 2023; Yang et al., 2023; Yedier et al., 2023). As a result, plastic garbage is ubiquitous in the atmosphere, and it has been proposed as an ecological indicator for the “Anthropocene Era” (Zalasiewicz et al., 2016). According to many literature reviews on laboratory ecotoxicity investigations, PP has received least attention (Maity and Pramanick, 2020; Peng et al., 2020).
Spirulina is a freshwater single-cell microscopic microalga with antioxidant enzymes that can prevent free radical formation (Seshadri et al., 1991) and an immune stimulant that is cost-effective and has fewer adverse effects when compared to manufactured treatments (Belay, 2020). Furthermore, Spirulina platensis (SP) has been demonstrated to protect against the toxicity of various well-known drugs, including aspirin (Mahmoud and Abd El-Ghffar, 2019), methotrexate (Khafaga and El-Sayed, 2018), D-galactosamine, acetaminophen, and rifampicin (Martin and Sabina, 2016). Spirulina is high in protein (50%–70% on dry mass basis), carotenoids, chlorophyll, pigments, essential fatty acids (alpha-linolenic, gamma-linolenic, and linoleic acid) (Peiretti and Meineri, 2011), photosynthetic pigments (Bermejo et al., 2008), vitamins (Hoseini et al., 2013), and minerals (Babadzhanov et al., 2004) such as Ca, K, Cr, Cu, Mn, Fe, P, Mg, Na, Zn, and Se, making Spirulina an effective feed supplement (Yousefi et al., 2019). Spirulina has recently been used successfully in fish farming as part of an integrated strategy for food supplementation and wastewater treatment to control water quality (Zhang et al., 2020; Sayed et al., 2022b). Spirulina has been reported to mitigate phytohormone toxicity, such as gibberellic acid, by Sayed et al. (2023a), and its protective role against chronic toxicity of chlorpyrifos in C. gariepinus was reported by Mokhbatly et al. (2020). Spirulina alleviated alterations caused by the toxicity of hydroxychloroquine in C. gariepinus (Sayed et al., 2021b), toxic effects of sodium dodecyl sulfate in the C. gariepinus (Sayed and Authman, 2018), and lead nitrate cytotoxicity and genotoxicity in C. gariepinus (Hamed et al., 2019b). However, until now, there are no reports about the potential protective role of Spirulina against PP-MP toxicity, particularly in economically important fish.
The African catfish (C. gariepinus), a widely distributed freshwater fish, can be found in both natural and developed aquatic habitats, according to Mohamed et al. (2022) and Hamed et al. (2023). Additionally, because of its well-documented biology, widespread usage in aquaculture, use as a component of human food, and a good fish model for toxicological, immunological, and histopathological studies (Mekkawy et al., 2010; Osman et al., 2019; Mukherjee et al., 2022), it has been employed as a valuable research tool.
There is a gap in the studies regarding the laboratory ecotoxicity of PP-MPs as microplastics released from paper cups. Therefore, the primary goal of this research is to investigate the effects of PP-MPs on African catfish C. gariepinus. More research should be done to determine the environmental risks of PP-MPs released from paper cups as well as how to deal with this new source of environmental burden.
2 Materials and methods
2.1 Chemicals and Spirulina platensis
PP-MPs are microsized, colored, and spherical with a mean size of approximately 35 µm and were purchased from Sigma-Aldrich.
Spirulina tablets were purchased from Japan Algae Co., Ltd.
2.2 Particle characterization
A methodology for characterizing PP-MPs was carried out via scanning electron microscopy at the scanning electron microscope unit (SEMU), Assiut University. The FTIR spectra were obtained, analyzed, and then compared with a set of reference data to identify the peaks according to Fang et al. (2012) and verify the PP-MPs.
2.3 Specimen collection
A juvenile population of African catfish (C. gariepinus) was collected from the aquaponic unit and then transported to 100-L tanks filled with dechlorinated water in the Fish Pollution Laboratory at Assiut University and allowed to acclimatize for 4 weeks. Fish were kept under regulated conditions throughout the studies, including 28°C water, 7.4 pH, 18.14 mg L-1 dissolved oxygen, 0.478 μS/cm conductivity, and a 12-h light: dark photoperiod. The fish were fed a commercial pellet diet of 3% of their body weight each day (crude protein, 30%; energy, 3890 kcal/kg; fibers, 5.71%; and crude fat, 3.08) provided by SKRETTING Company, Egypt, and the water was changed every day to get rid of impurities from metabolic waste. The African catfish were examined both before and after acclimatization to guarantee their health according to Batts et al. (2020).
2.4 Experimental design
After acclimatization to laboratory conditions, C. gariepinus fish (125 ± 3 gm and 27 ± 2 cm) were assigned to six triplicate experimental groups (18 fish/group, 6 fish in each replicate): a control group, a Spirulina group (200 mg/L), PP-MP-treated groups (0.14 and 0.28 mg/l PP-MPs), and PP-MP + Spirulina-treated groups (0.14 mg/l PP-MPs +200 mg/L SP and 0.28 mg/l PP-MPs +200 mg/L SP) for 15 days. Each group of fish was kept in its own aquarium with 70 L of water, with half of the water changed, and the PP-MP concentrations were reduced every 2 days and 45-day recovery after that. The doses of PP-MPs were selected according to Lei et al. (2018) and Jemec Kokalj et al. (2022) and the Spirulina dose according to Sayed and Authman (2018).
2.5 Blood sample collection
Six fish were randomly chosen from each group (two fish from each aquarium per sampling period) and subjected to anesthesia using ice at the end of both the exposure and recovery periods to mitigate stress (Wilson et al., 2009). Blood samples were drawn from the caudal veins and placed in heparinized and non-heparinized tubes for hemato-biochemical analysis. The same goes for blood smears, which were created on tidy glass slides and counted in five fields in each of the three slides of each fish group (about 1,500 cells).
2.6 Hemato-biochemical parameters
A hemocytometer was used to count the red blood cells (RBCs) (Stevens, 1997). To measure the hematocrit (Hct), Hesser’s microhematocrit method from 1960 was applied (Hesser, 1960). Lee et al. (1999)’s assessment of hemoglobin levels was used. Using the formulas recommended by Lee et al. (1999), the hematimetric indices were calculated from RBC counts, hemoglobin, and hematocrit. Mean corpuscular volume (MCV), mean corpuscular hemoglobin (MCH), and mean corpuscular hemoglobin concentration (MCHC) were calculated using the following equations (Bain et al., 2016):
Blood in non-heparinized tubes was allowed to coagulate at 4°C before being separated by centrifugation at 5,000 rpm for 20 min at 4°C to isolate the serum. Using kits from Bio Diagnostic in Egypt, the biochemical parameters such as alkaline phosphatase (ALP), aspartic aminotransferase (AST), and alanine aminotransferase (ALT) were measured.
2.7 Histological and histopathological assessment
Following exposure and recovery, the liver of C. gariepinus was dissected and preserved in a 10% neutral formalin solution. These samples were then dehydrated using a graded ethanol series (70%, 75%, 80%, 90%, and 100%). The samples were embedded in paraffin at 62°C and cut into 5-µm-thick sections with a precision microtome (MT-990; RMC Products, United States of America). Harris’ hematoxylin and eosin staining was used on the sections (Biancotti et al., 2020). The samples were examined using an Olympus BX-51 light microscope (Olympus Corp., Japan) in conjunction with an ARTCAM-150 PIII digital camera.
2.8 Statistical analysis
The fundamental statistics, means, standard errors, and ranges were estimated. The pattern of variation was investigated using one-way analysis of variance with the SPSS software (IBM-SPSS, 2012) at a significance level of 0.05. For multiple comparisons, the Tukey’s HSD test and Dunnett’s test were used.
3 Results
3.1 Characterization of PP-MP particles
The morphology of the PP-MP particles was confirmed by the SEM images; different spherical forms were seen (Figures 1B, C). The detection of chemical bonding in PP-MP plastics was aided by FTIR spectroscopy (Figure 1A). Similar peak values were seen in the FTIR spectra for PP-MPs at 3426 cm-1 (O–H stretching vibration), 2,957 cm-1 (CH3 asymmetrical stretching), 2,918 cm-1 (CH2 asymmetrical stretching), 2,850 cm-1 (CH2 stretching), 1,463 cm-1 (CH3 symmetrical bending), 1,290 cm-1 (CH3 rocking), 1,150 cm-1 (C–H wagging), 964 cm-1 (CH3 rocking), and 839 cm-1 (C–H rocking), and no extra bands related to impurities were detected, which confirms the high purity of this sample. The FTIR peak value of PP-MP polymers was reported by Tiwari et al. (2019) and Sathish et al. (2020), which is consistent with our results.
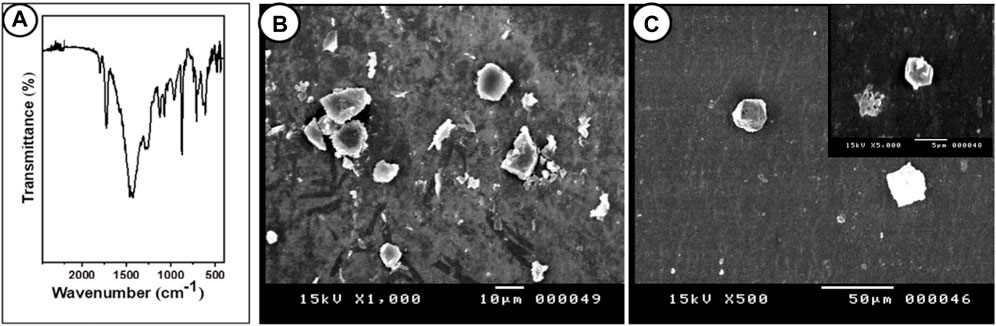
Figure 1. FTIR and SEM images of PP particles. (A) Fourier-transform infrared spectroscopy (FTIR) of polypropylene microplastics, and (B, C) SEM images of PP particles (scale bar = 10 μm).
3.2 Effects on hemato-biochemical parameters
The hematological parameters of C. gariepinus exposed to PP-MPs (0.14 mg/L), PP-MPs (0.28 mg/L), PP-MP (0.14 mg/L) + Spirulina (SP) (200 mg/L), PP-MP (0.28 mg/L) + Spirulina (200 mg/L), and Spirulina (200 mg/L) as a positive control for 15 days are documented in Table 1. Except for MCV, MCH, and MCHC, all parameters showed significant differences (p < 0.05) between the treated groups and the control group. The hematological parameters exhibiting significance (RBCs, Hct, Hb, and MCV) or non-significance (MCH and MCHC) either decreased with the increase in PP-MP doses from 0.0 to 0.28 mg/L in the control group (RBCs, Hct, MCH, MCHC, Hb, and platelets) or increased with such an increase in doses (MCV), as shown in Table 1. In the 200 mg/L SP-exposed group, there was a non-significant (p ≥ 0.05) increase or decrease in all parameters compared to the control group. Furthermore, in the 0.14 mg/l PP-MP +200 mg/L SP-exposed group, there was a non-significant increase (p ≥ 0.05) in all parameters compared to the control and the same exposed group without Spirulina, except Hb. Moreover, in the 0.28 mg/l PP-MP +200 mg/L SP-exposed group, there was a significant decrease (p < 0.05) compared to the control group and non-significant (p ≥ 0.05) increase compared to the same exposed group without Spirulina in all parameters, except MCV and MCH. The liver enzyme activities, particularly of aspartate aminotransferase (AST), alkaline phosphatase (ALP), and alanine aminotransferase (ALT), are recorded in Table 1. The parameters (AST, ALT, and ALP) exhibiting a non-significant (p ≥ 0.05) increase in the PP-MP (0.14 mg/L)-exposed group and a significant increase in the PP-MP (0.28 mg/L)-exposed group except (ALP) were significantly decreased compared to the control group. In the 200 mg/L SP-exposed group, there was a non-significant (p ≥ 0.05) increase or decrease in these parameters compared to the control group. Furthermore, in the 0.14 mg/l PP-MP +200 mg/L SP-exposed group, there was a significant increase (p < 0.05) in all parameters compared to the control group and the same exposed group without Spirulina. Moreover, in the 0.28 mg/l PP-MP +200 mg/L SP-exposed group, there was a non-significant (p ≥ 0.05) increase compared to the control group and non-significant decrease compared to the same exposed group without Spirulina in all parameters except ALP, as shown in Table 1.
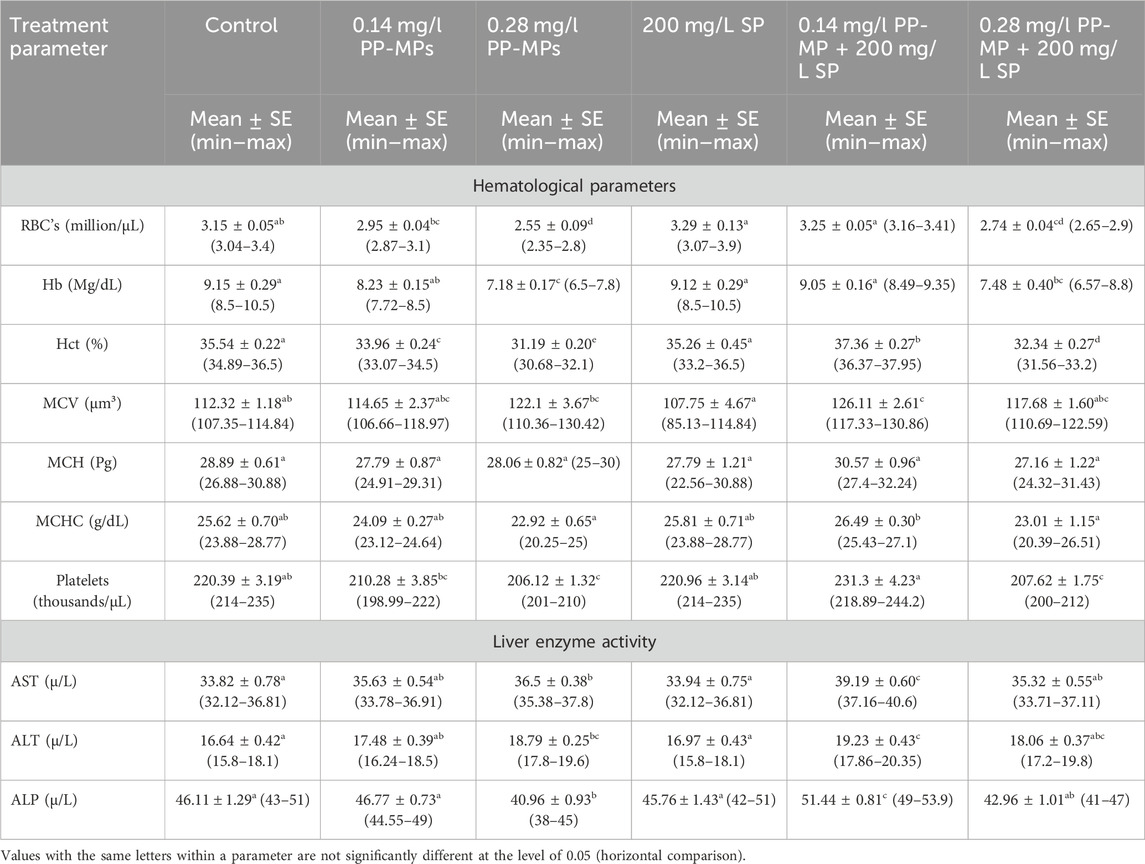
Table 1. Hematological and biochemical parameters (liver enzyme activity) of African catfish (C. gariepinus) exposed to PP-MPs (0.14 mg/L), PP-MPs (0.28 mg/L), PP-MP (0.14 mg/L) + Spirulina (200 mg/L), PP-MP (0.28 mg/L) + Spirulina (200 mg/L), and Spirulina (200 mg/L) (as a positive control) for 15 days.
After 45 days of recovery, comparable patterns of notable fluctuations toward an increase or decrease were noted in the hematological parameters, except in the 0.28 mg/l PP-MP +200 mg/L SP-exposed group; after the recovery period, there was a non-significant (p ≥ 0.05) decrease in all parameters compared to this dose in the exposure period Table 2. The parameters (AST, ALT, and ALP) exhibiting comparable patterns of notable fluctuations toward an increase or decrease except in 0.28 mg/l PP-MP +200 mg/L SP-exposed group showed a significant (p < 0.05) increase in these parameters after the recovery period compared to this dose to the same exposed group without Spirulina in the exposure period Table 2.
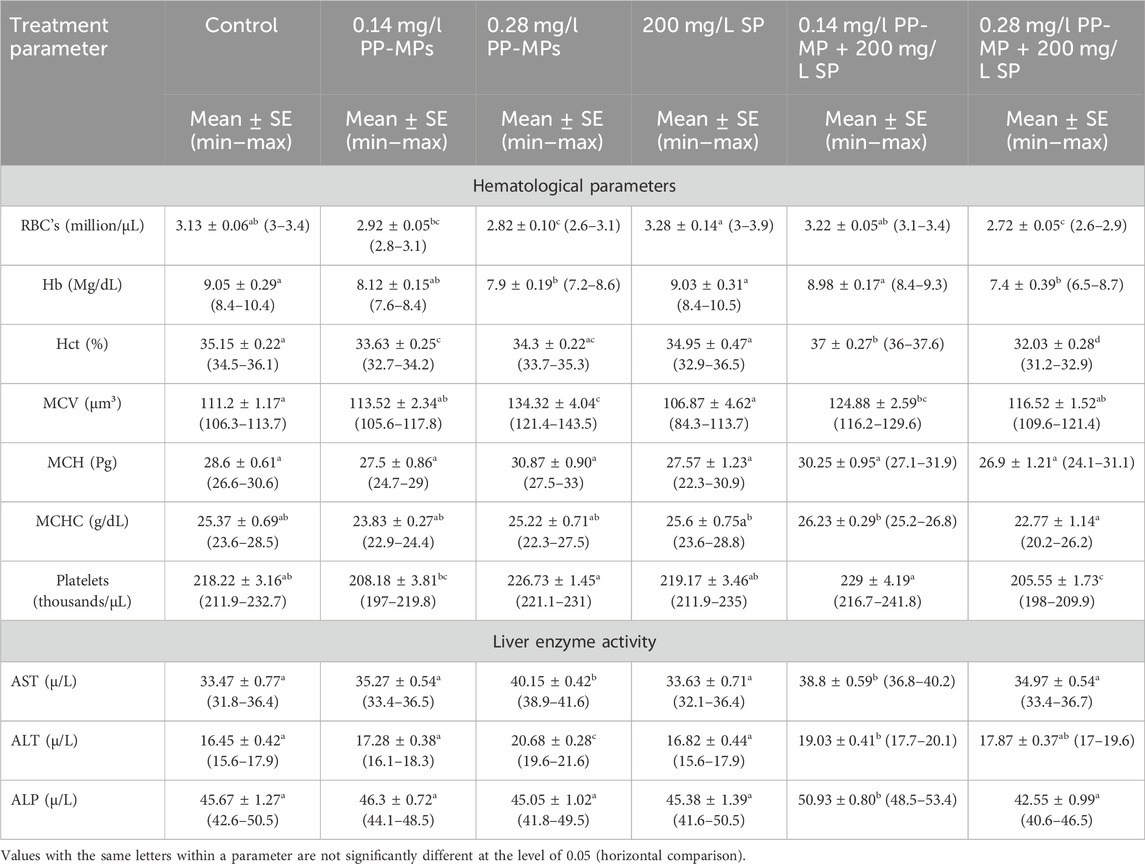
Table 2. Hematological and biochemical parameters (liver enzyme activity) of African catfish (C. gariepinus) exposed to PP-MPs (0.14 mg/L), PP-MPs (0.28 mg/L), PP-MP (0.14 mg/L) + Spirulina (200 mg/L), PP-MP (0.28 mg/L) + Spirulina (200 mg/L), and Spirulina (200 mg/L) (as a positive control) after 45 days of recovery.
3.3 Effects on the erythron profiles
According to Figure 2A, the erythrocytes (Er) in this species’ blood were spherical and nucleated, with a rounded nucleus positioned in the center. Additionally, based on their size, there were small lymphocytes (SL), with a darkly stained nucleus and a non-granular, weakly basophilic cytoplasm. In the present study, erythrocyte morphological abnormalities were recorded in that species exposed to PP-MPs (0.14 mg/L), PP-MPs (0.28 mg/L), PP-MP (0.14 mg/L) + Spirulina (200 mg/L), PP-MP (0.28 mg/L) + Spirulina (200 mg/L), and Spirulina (200 mg/L) as a positive control for 15 days. These deformations (Figures 2B–E) included echinocytes or crenated cell (Cr), acanthocytes (Ac), sickle cell (Sk), swelled cells (Sc), elliptocytes (EL), keratocytes (Kr), teardrop-like cells (Tr), hemolyzed cells (Hec), genuine cells (Gc), eccentric nucleus (Ecn), macronucleated cells (Mac), micronucleus (Mn), bionuclei (Bin), blebbed nucleus (Beln), and notched nucleus (Nn). PP-MPs (0.14 mg/L and 0.28 mg/L) caused a significant (p < 0.05) increase in the percentage of poikilocytosis and nuclear abnormalities of RBCs in relation to the control group, as shown in Table 3. Likewise, there were still significant (p < 0.05) increases in the percentage of poikilocytosis and nuclear abnormalities of RBCs in the fish exposed to PP-MPs (0.14 mg/L and 0.28 mg/L) and co-treated with Spirulina (200 mg/L) compared to the control group except (Tr, Gc, and Kr) in the PP-MP (0.14 mg/L) + SP (200 mg/L) group, which indicated that the Spirulina (200 mg/L) dose was insufficient to treat the effects of PP-MPs. In contrast to fish exposed to PP-MPs alone, fish treated with Spirulina showed a significant (p < 0.05) decrease in the percentage of poikilocytosis and nuclear abnormalities of RBCs. Significant differences were still evident between the groups after the recovery period in terms of the percentage of poikilocytosis and nuclear abnormalities of RBCs except for Tr in PP-MPs (0.14 mg/L) and PP-MP (0.14 mg/L) + SP (200 mg/L) groups, Kr in PP-MP (0.14 mg/L) + SP (200 mg/L) and PP-MP (0.28 mg/L) + SP (200 mg/L) groups, and the Gc in PP-MP (0.14 mg/L) + SP (200 mg/L) group, demonstrating that the effects of PP-MPs did not entirely disappear after the recovery period, as shown in Table 4. With respect to the control group, the SP (200 mg/L) group showed insignificant variations in most parameters. After 45 days of recovery under normal conditions, the percentage of poikilocytosis and nuclear abnormalities of RBCs showed a noticeable improvement, and the recovery rate was 83.7% with PP-MPs (0.14 mg/L), 83.3% with PP-MPs (0.28 mg/L), 81.4% with PP-MP (0.14 mg/L) + SP (200 mg/L), and 80.6% with PP-MPs] (0.28 mg/L) + SP (200 mg/L) (Table 5), suggesting that the effects of Spirulina disappeared after exposure. These differences in the percentage of poikilocytosis and nuclear abnormalities of RBCs increased with PP-MP concentrations, suggesting that the effects of PP-MPs on erythrocyte deformations and nuclear abnormalities were dose-dependent (Tables 3, 4).
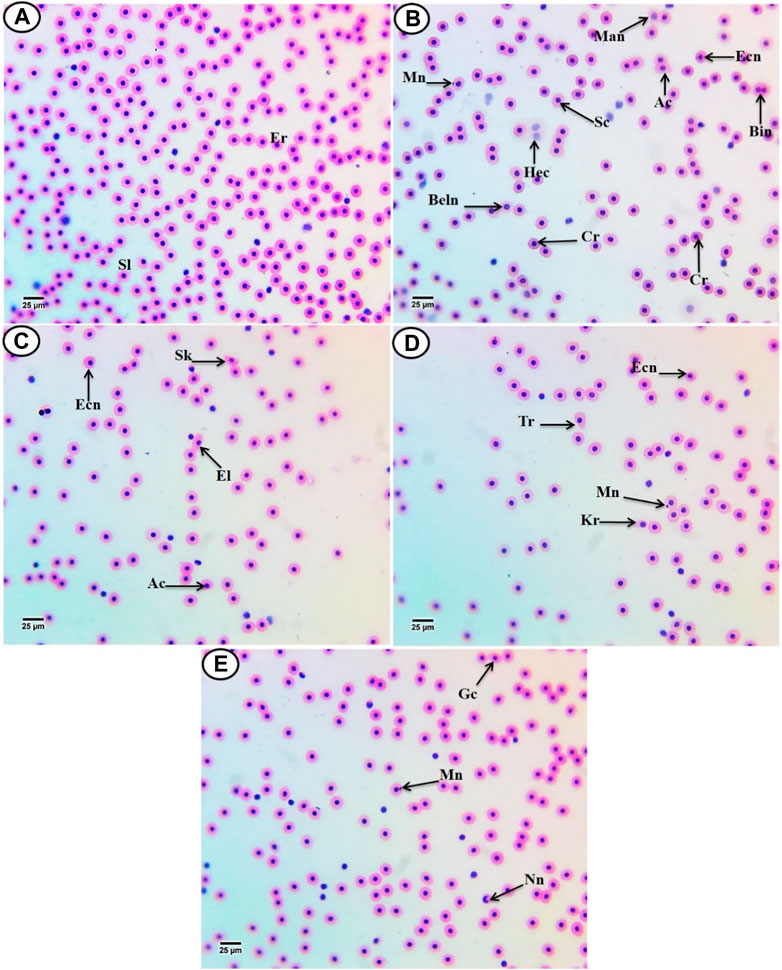
Figure 2. Hematoxylin and eosin-stained blood film from C. gariepinus. (A) Control group showing normal erythrocytes with a rounded-shape nucleus (Er) and differential leukocytes (small lymphocytes (SL)). [From (B–E)] Blood film of treated C. gariepinus showing the deformed ones: Cr, echinocytes or crenated cell; Ac, acanthocytes; Sk, sickle cells; Sc, swelled cells; El, elliptocytes; Kr, keratocytes; Tr, teardrop-like cells; Hec, hemolyzed cells; Gc, genuine cell; Ecn, eccentric nucleus; Mac, macronucleated cells; Mn, micronucleus; Bin, bionuclei; Beln, blebbed nucleus, and Nn, notched nucleus. Scale bar = 25 μm.
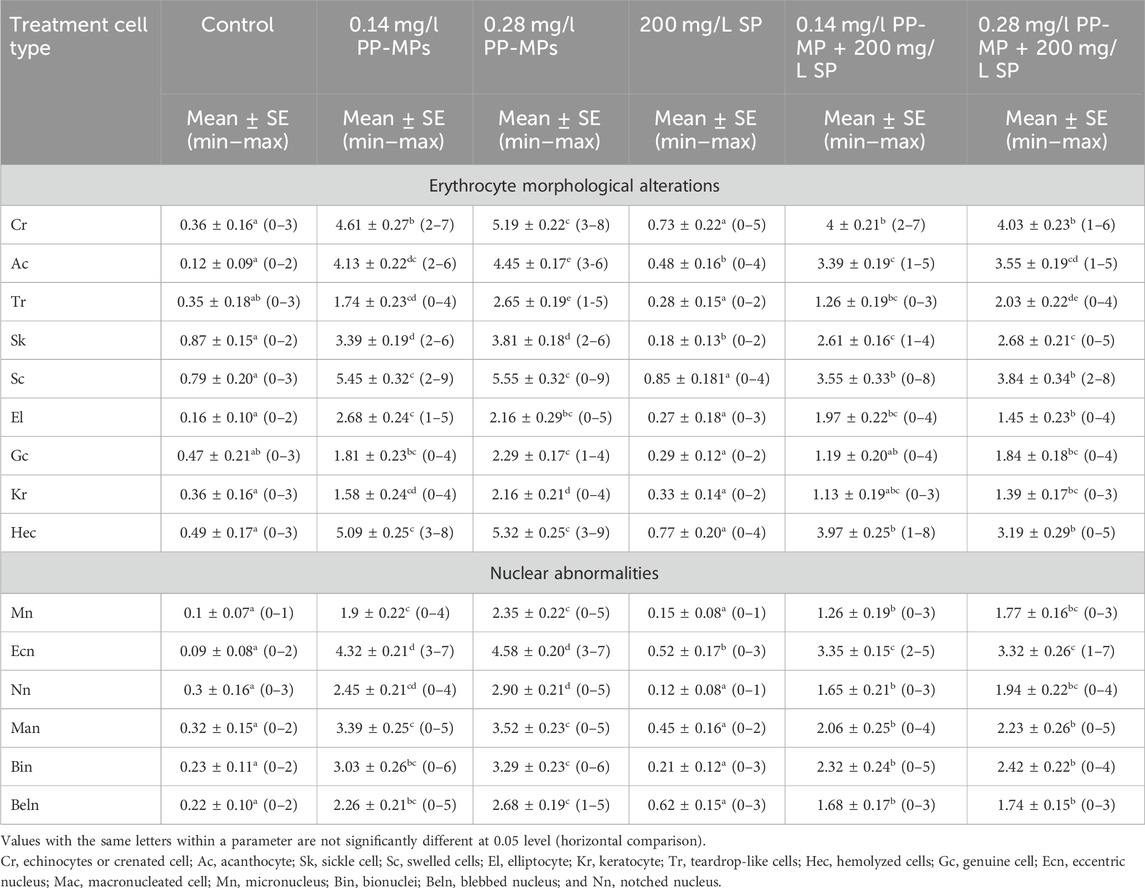
Table 3. Erythrocyte morphological alterations and nuclear abnormalities (mean ± SE and min–max range) in African catfish (C. gariepinus) after PP-MP exposure for 15 days and treatment with SP (200 mg/L). Significant differences from the control group values were accepted at p < 0.05.
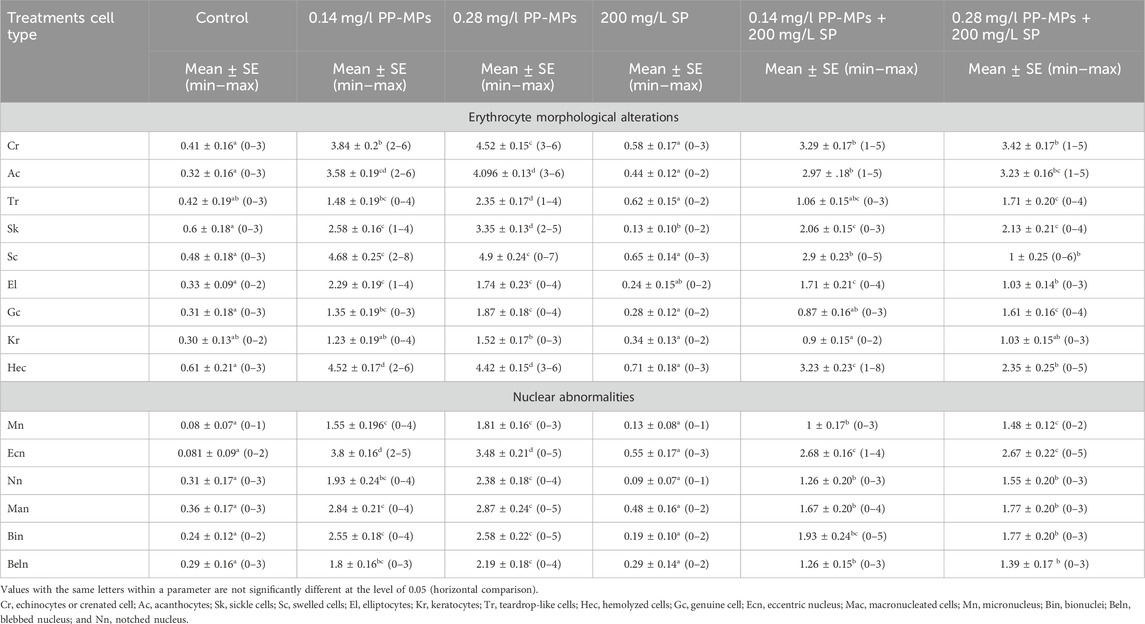
Table 4. Erythrocyte morphological alterations and nuclear abnormalities (mean ± SE and min–max range) in African catfish (C. gariepinus) after PP-MP exposure for 15 days and treatment with SP (200 mg/L) followed by 45 days of recovery. Significant differences from the control group values were accepted at p < 0.05.
3.4 Effects on the liver tissue and the role of Spirulina
Sections of C. gariepinus liver stained with hematoxylin and eosin and treated to SP (200 mg/L), PP-MPs (0.28 mg/L), PP-MP (0.28 mg/L) + SP (200 mg/L), PP-MPs (0.14 mg/L), and PP-MP (0.14 mg/L) + SP (200 mg/L) as a positive control for 15 days are shown in (Figures 3A–E). The control group displayed characteristic C. gariepinus liver tissue histological features, such as polygonal and regularly organized wedge-shaped hepatocytes with central nuclei surrounding the central vein and blood sinusoids (Figure 3A). While liver sections from fish treated with SP (200 mg/L) showed a considerably improved tissue structure, some hepatic structure changes were observed, such as a dilated central vein that was full of blood corpuscles, and besides that, there were melanomacrophage centers, hepatocytes aggregated and arranged in rosette shapes with their nuclei in an apical position, and some hepatocytes with pyknotic nuclei (Figure 3B).
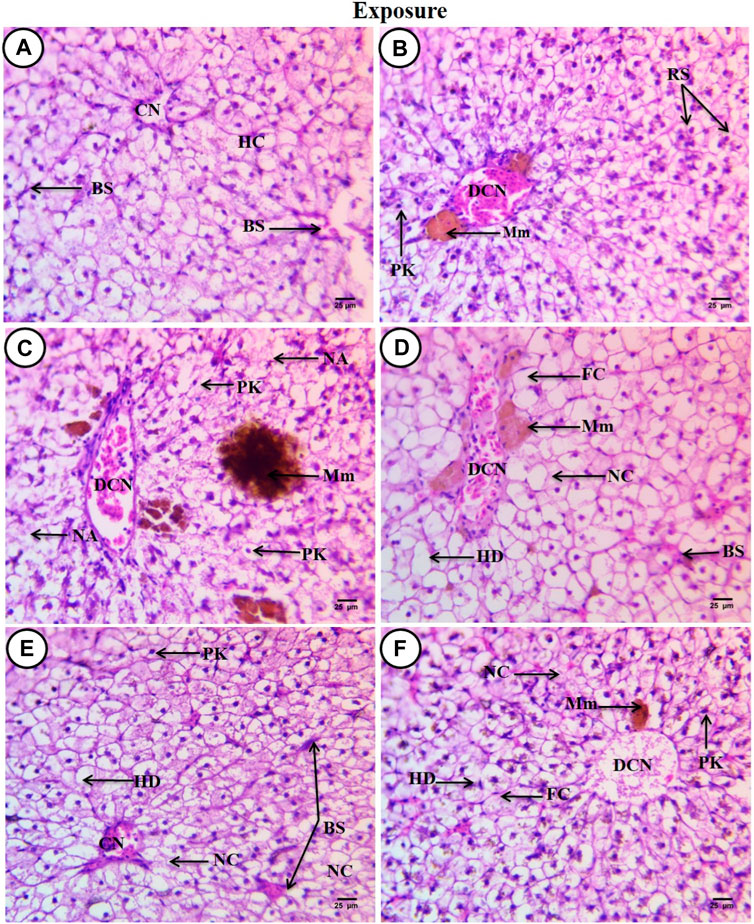
Figure 3. Sections of the liver from control and treated fish stained with hematoxylin and eosin (×400) after an exposure period of 15 days. (A) Section from the control fish group revealing typical histological features of the liver tissue of C. gariepinus. (B) Liver section from the Spirulina (200 mg/L)-exposed fish. (C) Liver section from a fish exposed to PP (0.28 mg/L) showing varying degrees of damage. (D) Liver section from a fish exposed to PP (0.28 mg/L) plus Spirulina. (E) Liver section from a fish exposed to polypropylene (0.14 mg/L). (F) Liver section from a fish exposed to PP (0.14 mg/L) plus Spirulina. Labeled structures are as follows: BS, blood sinusoids; CV, central vein; HC, hepatocytes; DCV, dilatation of the central vein; Mm, melanomacrophage centers; PK, pyknotic nucleus; RS, rosette shape hepatocytes; NA, necrotic area; HD, hydropic degeneration; FC, fatty cells with an eccentric flat nucleus, and NC, necrotic cells.
In contrast, sections from C. gariepinus exposed to PP-MPs (0.28 mg/L) showed varying degrees of damage, including a dilated central vein full of red blood corpuscles and leukocytes, deformation and severe degeneration in hepatic structures, necrosis of main bulk of hepatic cells, vacuolated cells with pyknotic nuclei with fine heterogamous acidophilic in cytoplasm, and increased necrotic area. In addition, it showed the ill-defined hepatocyte boundary or blood sinusoids and increase in the melanomacrophage center in the number and density of the staining degree (Figure 3C). While the PP-MPs (0.28 mg/L) plus Spirulina-exposed group showed great amelioration in hepatic structures when compared to the PP-MP (0.28 mg/L)-exposed group without Spirulina, some pathological signs were observed such as hydropic degeneration of the main bulk of hepatocytes with a central or eccentric nuclei, few fatty cells with an eccentric flat nucleus, few necrotic areas, a dilated central vein with different types of blood corpuscles, and beside it lie patches of melanomacrophage centers with yellow pigments (Figure 3D). The liver tissue from the PP-MP (0.14 mg/L)-exposed group exhibited little pathological changes compared to the PP-MP (0.28 mg/L)-exposed group, such as few aggregated cells completely lost their cytoplasmic materials and nuclei or necrosed, enlargement of cells around the central vein which was full of blood corpuscles, other cells appeared polygonal or hydropic degenerated with central, eccentric, or pyknotic nuclei, and shrunken blood sinusoids were noticed (Figure 3E). While liver sections from fish exposed to PP-MPs (0.14 mg/L) plus Spirulina also showed little amelioration in hepatic structure changes when compared with the same exposed group without Spirulina, there were pathological signs like dilated central veins which contained degenerated blood corpuscles (debris), surrounded by small-sized hepatocytes, and, beside them, small melanomacrophage centers. In addition, there was an increase in dispersed pigment granules inside hepatocytes, hydropic degeneration with or without nuclei, while other cells completely necrosed with an ill-defined boundary or contained pyknotic nuclei. A few necrotic areas and fatty cells were observed (Figure 3F).
After a recovery period of 45 days, the control group displayed characteristic C. gariepinus liver tissue histological features (Figure 4A). Liver sections from fish exposed to SP (200 mg/L SP) showed more or less improvement when compared with the same exposed group, although some hepatic structures changed, such as a dilated central vein full of blood corpuscles, aggregated hepatocytes, hydropic degeneration with pyknotic nuclei, the presence of necrotic areas, and increased small-sized blood sinusoids (Figure 4B). Liver sections from fish exposed to PP-MPs (0.28 mg/L) after the recovery period showed great amelioration in hepatic structures when compared with the same exposed group, such as disappearance of blood vessel dilation, decrease in melanomacrophage centers and necrotic areas, and central veins surrounded by few inflammatory cells. Although some cellular hydropic degeneration was observed in many hepatocytes with eccentric nuclei located beside the blood sinusoids, only a few vacuolated and necrotic cells and other cells contained pyknotic nuclei (Figure 4C). While the PP-MP (0.28 mg/L) plus Spirulina-exposed group after the recovery period showed great amelioration in hepatic structures when compared with the same group without Spirulina, less improvement was noticed when compared with the same exposed group. Still, there were some pathological signs, such as congested blood sinusoids, hydropic degeneration of hepatocytes with clear contours and central or eccentric nuclei, and other cells containing pyknotic nuclei (Figure 4D). The liver tissue from the PP-MP (0.14 mg/L)-exposed group after the recovery period exhibited little improvement, and many cells contained mitotic nuclei when compared with the same exposed group, but some pathological changes were still present, such as an increase in the size of congested blood sinusoids and melanomacrophage centers, many necrotic hepatocytes, hydropic degeneration, and few cells containing vesicular or pyknotic nuclei (Figure 4E). Moreover, the PP-MPs (0.14 mg/L) plus Spirulina-exposed group after the recovery period exhibited amelioration when compared with the same exposed group. In addition, some marked hepatic structures changes were observed, such as hydropic degeneration with pyknotic nuclei, other cells completely necrosed with ill-defined boundaries, few necrotic areas, small melanomacrophage centers and dispersed pigment granules inside hepatocytes, and increase in the number of small blood sinusoids (Figure 4F).
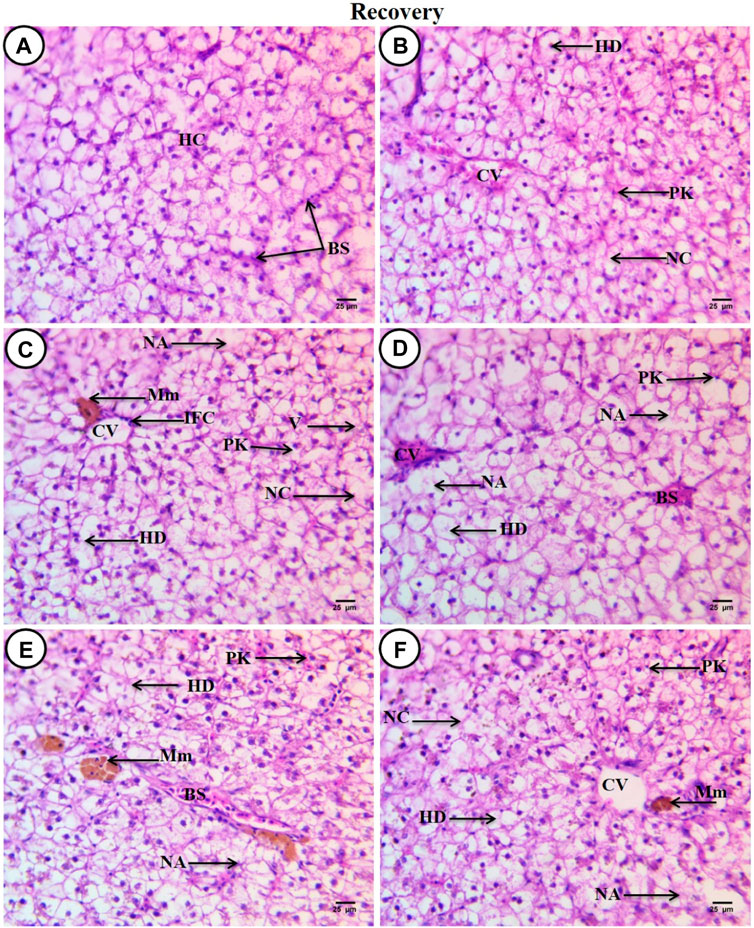
Figure 4. Sections of liver from control and treated fish stained with hematoxylin and eosin (×400) after a recovery period of 45 days. (A) Section from the control fish group revealing typical histological features of the liver tissue of C. gariepinus. (B) Liver section from a fish exposed to Spirulina (200 mg/L) after a recovery period of 45 days. (C) Liver section from a fish exposed to PP (0.28 mg/L) after a recovery period of 45 days showing great amelioration in hepatic structures. (D) Liver section from a fish exposed to PP (0.28 mg/L) plus Spirulina after a recovery period of 45 days. (E) Liver section from a fish exposed to PP (0.14 mg/L) after a recovery period for 45 days showing little improvement. (F) Liver section from a fish exposed to PP (0.14 mg/L) plus Spirulina after a recovery period of 45 days showing more or less amelioration. Labeled structures are as follows: BS, blood sinusoids; HC, hepatocytes; CV, central vein; PK, pyknotic nuclei; NC, necrotic cells; HD, hydropic degeneration; NA, necrotic area; IFC, inflammatory cells; V, vacuolated cell, and Mm, melanomacrophage centers.
4 Discussion
Environmental contaminants in the aquatic surroundings disrupt osmotic pressure and fish metabolism as well as have an impact on hematological parameters such as RBC counts, Hb, and Ht levels (Lee et al., 2023). As a result, vital information regarding the general health of fish that have been subjected to environmental stress can be obtained by studying their blood (Burgos-Aceves et al., 2019; Kim et al., 2021a). For instance, blood flow and composition may be considerably affected once MPs are translocated to the circulatory system (Scanes et al., 2019). According to Hirt and Body-Malapel (2020), MPs interact with the fish intestine and can reach the bloodstream, causing systemic problems. As stated by Thummabancha et al. (2016), hematological indices are the primary indicators of fish health. In this study, the parameters (RBCs, Hct, and Hb) exhibited a significance decrease with the increase in PP-MP doses from 0.0 to 0.28 mg/L in the control group. These findings were corroborated with those of Kim et al. (2021b), Hamed et al. (2019a), and Hamed et al. (2022). In the presence of MPs, similar results were obtained with Nile tilapia (Oreochromis niloticus) (Hamed et al., 2019a). According to Choi et al. (2021), MP concentrations in the blood lead to higher levels of hemolysis because the sharp surfaces of MPs physically disrupt RBC membranes and result in cell lysis. Additionally, O. niloticus’s RBC count, Hb, and Ht levels significantly decreased after exposure to MPs, according to studies by Hamed et al. (2019a) and Ismail et al. (2021). These studies hypothesized that the changes in hematological parameters were caused by MP toxicity, which also caused damage to hematopoietic tissue and RBC hemolysis. Accordingly, the observed alterations in RBC count, Hb, and Ht levels in the current study were probably caused by blood hemolysis and blood cell death brought on by PP-MPs toxicity, which led to anemia, as explained by Lee et al. (2023), after the exposure of Pseudobagrus fulvidraco to PE-MPs. The results in the MCV, MCH, and MCHC did not show any statistically significant changes (p < 0.05) between control fish and those treated with the two dosages of microplastics of 0.28 and 0.14 mg/L of PP-MPs (Table 1). Nascimento et al. (2023) found comparable results, observing non-significant statistical differences between control Nile tilapia (O. niloticus) and those treated with two doses of microplastics of 100 and 500 μg of PP-MPs. Erythrocyte degeneration has been identified as a pathological state in fish exposed to toxicants (Adeyemo, 2005). Erythrocytes have a critical role in oxygen transport (Benedik and Hamlin, 2014). Erythrocyte deformations result in low oxygen levels, which disrupt the circulatory system and induce respiratory dysfunction, both of which can modify erythrocyte morphology (Brown et al., 1994; Benedik and Hamlin, 2014). In the present work, the blood cell alterations of varying degrees were observed in C. gariepinus, depending on the concentration of PP-MPs and co-treatment with Spirulina. Similar findings were mentioned by Hamed et al. (2022), Hamed et al. (2021), and Sayed et al. (2021a) induced by polyethylene polymers of various sizes (nano, micro, and macro) in juveniles, Cyprinus carpio. Numerous explanations have been offered to explain the morphological alterations in blood cells induced by stress (Harper and Wolf, 2009; Mekkawy et al., 2011). There are various ways that red blood cell poikilocytosis can occur, including problems in phospholipid metabolism (Dacie et al., 1975) or acanthocytosis in cases of liver cirrhosis (Cooper, 1980). Teardrop cells may also arise as a result of splenic macrophages removing precipitated hemoglobin or autophagocytic vacuoles from RBCs (Bike, 1993). Sickle cells have been identified in both hemoglobin sickle cell sickness and anoxia (Dacie et al., 1975). Crenation of red blood cells occurs as a result of cellular energy (ATP) depletion caused by dehydration or expansion of the outer membrane leaflets (Stello et al., 2003). The vacuoles detected in erythrocytes could be attributed to unequal hemoglobin distribution, and enlarged blood cells were identified as a symptom of necrosis. This conclusion was comparable to that of Soliman and Sayed (2020).
B-carotene in Spirulina improves the recovery of RBCs and minimizes cell lysis and changes (Sayed and Authman, 2018; Hamed et al., 2019b; Sayed et al., 2023a; Sayed et al., 2023b). Spirulina platensis includes iron and vitamins, which regulate red blood cell synthesis (Hemalatha, 2012), as well as polysaccharides, which promote RBC renewal (Mohamed et al., 2014). Furthermore, phycocyanin, a pigment, stimulates the erythropoietin (EPO) hormone, which is responsible for erythropoiesis (Zhang, 1994). However, recent data revealed that co-treatment with Spirulina did not significantly reduce the effects of PP-MPs, indicating that the Spirulina dose (200 mg/L) was insufficient.
PP-MPs have been described in the literature to be among the most prevalent types of plastics in aquatic environments, with a significant carbon peak in EDX analysis (Wang et al., 2017; Furfaro et al., 2022; Yedier et al., 2023). PP-MPs produce oxidative stress, organ damage, and decrease survival in Danio rerio and Oreochromis mossambicus in freshwater (Lei et al., 2018; Jeyavani et al., 2023). Furthermore, Jeyavani et al. (2023) showed oxidative stress, DNA damage, increased apoptosis, and histological alterations in O. mossambicus liver tissues subjected to a diet enriched with PP-MPs. Fish serum’s ALT and AST levels are used as markers of their hepatic functioning (Abdel-Latif et al., 2020), can serve as early warning signs of significant changes in stressed organisms, and are useful indicators of an animal’s overall health status (Garima and Himanshu, 2015). These enzymes were primarily located in the cytoplasm of the hepatocyte, but they were also released into the bloodstream in cases of liver damage (Amacher, 1998). Based on liver damage, in this study, some blood indicators (ALP, AST, and ALT) exhibited a non-significant (p ≥ 0.05) increase in the PP-MP-exposed group (0.14 mg/L) and a significant increase in the PP-MP (0.28 mg/L)-exposed group when compared to the control group. The results obtained were consistent with what was observed in catfish (C. gariepinus) after exposure to polystyrene nanoplastics (PS-NPs) (Sayed et al., 2021a; Abdelbaky et al., 2022; Soliman et al., 2023), after exposure to microplastic particles in African catfish (C. gariepinus), and after juvenile of common carp C. carpio were exposed to three distinct sizes of polyethylene plastics: nano, micro, and macro (Hamed et al., 2022).
Many fish species, such as the common goby (Pomatoschistus microps) (Oliveira et al., 2013), common carp (C. carpio) (Haghi Nematdoost and Banaee, 2017; Ammar et al., 2023), Nile tilapia (O. niloticus) (Hamed et al., 2019a), catfish (C. gariepinus) (Sayed et al., 2021a), and red tilapia (O. niloticus) (Yang et al., 2023), have all experienced changes in their biochemical parameters as a result of MP exposure. Serum biomarkers generally function as indicators of organ failure and biological process disruption. These biomarkers were still increased when Spirulina supplementation was used with (0.14 and 0.28 mg/L) PP-MPs, which indicated that a Spirulina dose of 200 mg/L was insufficient to treat the effects of PP-MPs. When these enzymes are released from the cell and increase in blood levels, they are thought to be markers of damage to the cell membranes (Banaee et al., 2014). Damage to hepatocytes, which causes detoxication and the hepatocytes’ subsequent export into the bloodstream, may be the result of these enzymatic alterations (Sayed et al., 2022b).
Increased blood concentrations of these enzymes signify pathological alterations in the permeability and/or cytotoxicity of hepatocyte cell membranes, implying the detrimental impact of PP-MPs on the integrity of the liver (do Nascimento et al., 2023). These results corroborated to those of Hamed et al. (2019a), who found a significant increase in juvenile O. niloticus exposed to microplastic and do Nascimento et al. (2023) who noted similar findings in Nile tilapia following ingestion of PP-MPs. According to Kim et al. (2021b), an elevation in AST may lead to hormonal imbalances, lipid metabolic disorders, and liver-related lipoproteins, ultimately resulting in elevated levels of cholesterol and triglycerides in fish. The disruption of cell membranes and mitochondria resulted in a significant increase in ALT levels in C. carpio exposed to MPs, as reported by Banaee et al. (2019). Another significant enzyme in fish metabolism is ALP, which is necessary for several physiological processes such as cell division, protein phosphorylation, and metabolite transport. Fish metabolism can therefore be impacted by variations in ALP activity (Chinnadurai et al., 2022). Additionally, Haghi Nematdoost and Banaee (2017) found a large increase in ALP levels when C. carpio was exposed to MPs, and they hypothesized that this was due to hepatocyte necrosis and degeneration caused by MP toxicity.
Karami et al. (2016) highlighted the significant role of the liver as the organ responsible for metabolizing fats and describe how microplastics can impair its functioning. In the present work, the liver tissue from C. gariepinus exposed to PP-MPs (0.28 mg/L) showed different levels of damage, such as dilated central vein full of red blood corpuscles and leucocytes, deformation and severe degeneration in hepatic structures, necrosis of the main bulk of hepatic cells, vacuolated cells with pyknotic nuclei with fine heterogamous acidophilic in the cytoplasm, and increased necrotic areas, while the liver tissue from the PP-MP (0.14 mg/L)-exposed group exhibited little pathological changes compared to the the PP-MP (0.28 mg/L)-exposed group. These results indicated that these pathological changes increased with PP-MP concentration, suggesting that the effect of PP-MPs on liver tissue was dose-dependent. In support of our results, Jeyavani et al. (2023) reported that PP-MP exposure showed that the degeneration of liver cells was directly correlated with the exposure doses to PP-MPs, as evidenced by a decrease in sinusoid infiltration with leukocytes, hepatocyte necrosis, hepatocyte vacuole formation, and dilated sinusoidal atrophy. In addition, according to Espinosa et al. (2019), European sea bass can sustain liver tissue damage after up to 3 weeks of exposure to polyvinylchloride and polyethylene microplastics. In accordance with, Jeyavani et al. (2023), the present work showed that the catfish (C. gariepinus) that were exposed to PP-MPs for up to 15 days produce ROS levels that cause oxidative stress, which damages the liver tissues. Co-treatment with Spirulina decreased these detrimental effects on liver function, possibly as a result of its capacity to scavenge and suppress ROS (Kuriakose and Kurup, 2011; Soliman et al., 2021).
After 45 days of recovery under normal conditions, it was clear that there was a considerable improvement in the percentage of poikilocytosis and nuclear abnormalities of RBCs, as well as a non-significant improvement in hemato-biochemical parameters and liver tissue. This indicated the threat of PP-MPs, which typically end up in the environment and persist in freshwater ecosystems. The current study’s findings showed that the morphological restoration of C. gariepinus liver tissue took longer than the recovery of hemato-biochemical parameters. These seem to be significant adaptive reactions of PP-MP-intoxicated fish, implying the potential compensatory responses of other organic systems that enable fish to rapidly return to normal plasma ion concentration and blood parameters, as reported by Cerqueira and Fernandes (2002) after recovery from copper exposure of the tropical fish Prochilodus scrofa and Amorim et al. (2023) after recovery in O. niloticus fish exposed to urban effluents. Nonetheless, metabolic costs to maintain homeostasis can exceed the critical level for fish and have serious consequences for the energy balance, reproduction, and survival of the fish (Cerqueira and Fernandes, 2002). A defense state that preserves the hepatocellular membrane’s structural integrity is presented by a reduction in ALT and AST activity during recovery (Pari and Amali, 2005). This fact was linked to the improvement in liver cell damage and necrosis during recovery treatments due to the absence of toxic stress (Meraj et al., 2017).
5 Conclusion
Exposure to PP-MPs at 0.14 and 0.28 mg/L concentrations for 15 days showed deleterious effects, resulting in disruption in hematological and biochemical parameters, alteration in erythrocyte profile, poikilocytosis, and nuclear abnormalities of RBCs and changes in the liver functions. The current study outcomes displayed that, despite a 45-day recovery period, the effects of PP-MPs were not completely lost after the recovery period, indicating the threat of PP-MPs released from various sources, particularly paper cups, which are widely used in our daily lives and typically end up in the environment and persist in freshwater ecosystems. This reflects the adverse effects of PP-MPs on aquatic biota, particularly fish, and may get wider attention from scientists due to the potential health implications. The Spirulina (200 mg/L) dose was insufficient to significantly treat the effects of PP-MPs, and its effects disappeared after exposure. Thus, Spirulina microalga must be widespread at a high concentration in aquatic environments for contribution of removal or protection against the toxicity of PP-MPs, which have deleterious effects on aquatic organisms.
Data availability statement
The original contributions presented in the study are included in the article/Supplementary material; further inquiries can be directed to the corresponding author.
Ethics statement
The animal studies including the experimental design, procedures, and fish handling were all approved by the MBRSI-Research Ethics Committee (IORG0010947- 22-2023-0027), Assiut University, Egypt. The studies were conducted in accordance with the local legislation and institutional requirements. Written informed consent was obtained from the owners for the participation of their animals in this study.
Author contributions
ZE: conceptualization, data curation, formal analysis, investigation, methodology, software, validation, visualization, writing–original draft, and writing–review and editing. UM: conceptualization, supervision, writing–original draft, and writing–review and editing. AE-DS: conceptualization, data curation, formal analysis, investigation, methodology, project administration, resources, software, supervision, validation, writing–original draft, and writing–review and editing.
Funding
The author(s) declare that no financial support was received for the research, authorship, and/or publication of this article.
Conflict of interest
The authors declare that the research was conducted in the absence of any commercial or financial relationships that could be construed as a potential conflict of interest.
The author(s) declared that they were an editorial board member of Frontiers, at the time of submission. This had no impact on the peer review process and the final decision.
Publisher’s note
All claims expressed in this article are solely those of the authors and do not necessarily represent those of their affiliated organizations, or those of the publisher, the editors, and the reviewers. Any product that may be evaluated in this article, or claim that may be made by its manufacturer, is not guaranteed or endorsed by the publisher.
References
Abdelbaky S. A., Zaky Z. M., Yahia D., Ali M., Sayed A.E.-D. H., Abd-Elkareem M., et al. (2022). Ameliorative effects of selenium and Chlorella vulgaris against polystyrene nanoplastics-induced hepatotoxicity in african catfish (Clarias gariepinus). J. Adv. Veterinary Res. 12.
Abdel-Latif H. M., Abdel-Tawwab M., Khafaga A. F., Dawood M. A. (2020). Dietary oregano essential oil improved the growth performance via enhancing the intestinal morphometry and hepato-renal functions of common carp (Cyprinus carpio) fingerlings. Aquaculture 526, 735432. doi:10.1016/j.aquaculture.2020.735432
Adeyemo O. (2005). Haematological and histopathological effects of cassava mill effluent in Clarias gariepinus. Afr. J. Biomed. Res. 8, 179–183. doi:10.4314/ajbr.v8i3.35747
Alsabri A., Tahir F., Al-Ghamdi S. G. (2022). Environmental impacts of polypropylene (PP) production and prospects of its recycling in the GCC region. Mater. Today Proc. 56, 2245–2251. doi:10.1016/j.matpr.2021.11.574
Amacher D. E. (1998). Serum transaminase elevations as indicators of hepatic injury following the administration of drugs. Regul. Toxicol. Pharmacol. 27, 119–130. doi:10.1006/rtph.1998.1201
Ammar E., Hamed M., Mohamed M. S., Sayed A.E.-D. H. (2023). The synergetic effects of 4-nonylphenol and polyethylene microplastics in Cyprinus carpio juveniles using blood biomarkers. Sci. Rep. 13, 11635. doi:10.1038/s41598-023-38636-2
Amorim N. P. L., de Assis R. A., Dos Santos C. G. A., Benvindo-Souza M., Borges R. E., de Souza Santos L. R. (2023). Erythrocyte recovery in Oreochromis niloticus fish exposed to urban effluents. Bull. Environ. Contam. Toxicol. 112, 15–03833. doi:10.1007/s00128-023-03833-2
Andrady A. L., Neal M. A. (2009). Applications and societal benefits of plastics. Philosophical Trans. R. Soc. B Biol. Sci. 364, 1977–1984. doi:10.1098/rstb.2008.0304
Babadzhanov A., Abdusamatova N., Yusupova F., Faizullaeva N., Mezhlumyan L., Malikova M. K. (2004). Chemical composition of Spirulina platensis cultivated in Uzbekistan. Chem. Nat. Compd. 40, 276–279. doi:10.1023/b:conc.0000039141.98247.e8
Bain B. J., Bates I., Laffan M. A. (2016). Dacie and lewis practical haematology E-book. Expert consult: online and print. Elsevier Health Sci.
Banaee M., Soltanian S., Sureda A., Gholamhosseini A., Haghi B. N., Akhlaghi M., et al. (2019). Evaluation of single and combined effects of cadmium and micro-plastic particles on biochemical and immunological parameters of common carp (Cyprinus carpio). Chemosphere 236, 124335. doi:10.1016/j.chemosphere.2019.07.066
Banaee M., Sureda A., Zohiery F., Hagi B. N., Garanzini D. S. (2014). Alterations in biochemical parameters of the freshwater fish, Alburnus mossulensis, exposed to sub-lethal concentrations of Fenpropathrin. Int. J. Aquatic Biol. 2, 58–68. doi:10.22034/ijab.v2i2.32
Batts W. N., Lovy J., Getchell R., Faisal M., Standish I., Warg J. V., et al. (2020). 2.2. 7 viral hemorrhagic septicemia. Fish Health Sect. Blue Book—Suggested Proced. Detect. Identif. Certain Finfish Shellfish Pathogens.
Belay A. (2020). The potential application of Spirulina (Arthrospira) as a nutritional health and therapeutic supplement in health management. J. Am. Nutraceutical Assoc. 5, 27–48.
Benedik P. S., Hamlin S. K. (2014). The physiologic role of erythrocytes in oxygen delivery and implications for blood storage. Crit. Care Nurs. Clin. North Am. 26, 325–335. doi:10.1016/j.ccell.2014.04.002
Bermejo P., Piñero E., Villar Á. M. (2008). Iron-chelating ability and antioxidant properties of phycocyanin isolated from a protean extract of Spirulina platensis. Food Chem. 110, 436–445. doi:10.1016/j.foodchem.2008.02.021
Biancotti J., Kumar S., de Vellis J., Drapeau V., Després J., Bouchard C., et al. (2020) Theory and practice of his-tological techniques. Edinburgh: Churchill Livingstone, 2615–2628. Cognitive Impairments of Sleep-Deprived Ovariectomized (OVX) Female Rats by Voluntary Exercise 11.
Bike R. (1993) Haematology: clinical and laboratory practice 1: 12 Morphology of erythron. St. Louis, Missouri.
Bobori D. C., Feidantsis K., Dimitriadi A., Datsi N., Ripis P., Kalogiannis S., et al. (2022). Dose-dependent cytotoxicity of polypropylene microplastics (PP-MPs) in two freshwater fishes. Int. J. Mol. Sci. 23, 13878. doi:10.3390/ijms232213878
Bora R., Wang R., You F. (2020). Waste polypropylene plastic recycling toward climate change mitigation and circular economy: energy, environmental, and technoeconomic perspectives. ACS Sustain. Chem. Eng. 8, 16350–16363. doi:10.1021/acssuschemeng.0c06311
Brown C. M., Novin F., Purich D. L. (1994). Calcium oxalate crystal morphology: influence of phospholipid micelles with compositions based on each leaflet of the erythrocyte membrane. J. Cryst. growth 135, 523–532. doi:10.1016/0022-0248(94)90143-0
Burgos-Aceves M. A., Lionetti L., Faggio C. (2019). Multidisciplinary haematology as prognostic device in environmental and xenobiotic stress-induced response in fish. Sci. Total Environ. 670, 1170–1183. doi:10.1016/j.scitotenv.2019.03.275
Cerqueira C. C., Fernandes M. N. (2002). Gill tissue recovery after copper exposure and blood parameter responses in the tropical fish Prochilodus scrofa. Ecotoxicol. Environ. Saf. 52, 83–91. doi:10.1006/eesa.2002.2164
Chinnadurai K., Prema P., Veeramanikandan V., Kumar K. R., Nguyen V.-H., Marraiki N., et al. (2022). Toxicity evaluation and oxidative stress response of fumaronitrile, a persistent organic pollutant (POP) of industrial waste water on tilapia fish (Oreochromis mossambicus). Environ. Res. 204, 112030. doi:10.1016/j.envres.2021.112030
Choi D., Hwang J., Bang J., Han S., Kim T., Oh Y., et al. (2021). In vitro toxicity from a physical perspective of polyethylene microplastics based on statistical curvature change analysis. Sci. Total Environ. 752, 142242. doi:10.1016/j.scitotenv.2020.142242
Cooper R. (1980). Hemolytic syndromes and red cell membrane abnormalities in liver disease. Semin. Hematol. 17, 103–112.
De Sá L. C., Oliveira M., Ribeiro F., Rocha T. L., Futter M. N. (2018). Studies of the effects of microplastics on aquatic organisms: what do we know and where should we focus our efforts in the future? Sci. Total Environ. 645, 1029–1039. doi:10.1016/j.scitotenv.2018.07.207
do Nascimento L. S., de Oliveira S. L., da Costa C. C., Aracati M. F., Rodrigues L. F., Charlie-Silva I., et al. (2023). Deleterious effects of polypropylene microplastic ingestion in nile Tilapia (Oreochromis niloticus). Bull. Environ. Contam. Toxicol. 111, 13. doi:10.1007/s00128-023-03772-y
Espinosa C., Esteban M. Á., Cuesta A. (2019). Dietary administration of PVC and PE microplastics produces histological damage, oxidative stress and immunoregulation in European sea bass (Dicentrarchus labrax L.). Fish Shellfish Immunol. 95, 574–583. doi:10.1016/j.fsi.2019.10.072
Fang J., Zhang L., Sutton D., Wang X., Lin T. (2012). Needleless melt-electrospinning of polypropylene nanofibres. J. Nanomater. 2012, 1–9. doi:10.1155/2012/382639
Furfaro G., D’Elia M., Mariano S., Trainito E., Solca M., Piraino S., et al. (2022). SEM/EDX analysis of stomach contents of a sea slug snacking on a polluted seafloor reveal microplastics as a component of its diet. Sci. Rep. 12, 10244. doi:10.1038/s41598-022-14299-3
Garima P., Himanshu P. (2015). Impact of heavy metal, arsenic trioxide on biochemical profile of teleost, Clarias batrachus (linn.). Int J Life Sci. 3, 141–146.
Haghi Nematdoost B., Banaee M. (2017). Effects of micro-plastic particles on paraquat toxicity to common carp (Cyprinus carpio): biochemical changes. Int. J. Environ. Sci. Technol. 14, 521–530. doi:10.1007/s13762-016-1171-4
Hamed M., Martyniuk C. J., Said R. E. M., Soliman H. A. M., Badrey A. E. A., Hassan E. A., et al. (2023). Exposure to pyrogallol impacts the hemato-biochemical endpoints in catfish (Clarias gariepinus). Environ. Pollut. 333, 122074. doi:10.1016/j.envpol.2023.122074
Hamed M., Monteiro C. E., Sayed A.E.-D. H. (2022). Investigation of the impact caused by different sizes of polyethylene plastics (nano, micro, and macro) in common carp juveniles, Cyprinus carpio., using multi-biomarkers. Sci. Total Environ. 803, 149921. doi:10.1016/j.scitotenv.2021.149921
Hamed M., Osman A. G., Badrey A. E., Soliman H. A., Sayed A.E.-D. H. (2021). Microplastics-induced eryptosis and poikilocytosis in early-juvenile nile Tilapia (Oreochromis niloticus). Front. Physiology 12, 742922. doi:10.3389/fphys.2021.742922
Hamed M., Soliman H. A., Osman A. G., Sayed A.E.-D. H. (2019a). Assessment the effect of exposure to microplastics in Nile Tilapia (Oreochromis niloticus) early juvenile: I. blood biomarkers. Chemosphere 228, 345–350. doi:10.1016/j.chemosphere.2019.04.153
Hamed M., Soliman H. A., Sayed A.E.-D. H. (2019b). Ameliorative effect of Spirulina platensis against lead nitrate–induced cytotoxicity and genotoxicity in catfish Clarias gariepinus. Environ. Sci. Pollut. Res. 26, 20610–20618. doi:10.1007/s11356-019-05319-3
Harper C., Wolf J. C. (2009). Morphologic effects of the stress response in fish. ILAR J. 50, 387–396. doi:10.1093/ilar.50.4.387
Haya N., Maher C. (2013). Polypropylene mesh for pelvic organ prolapse surgery. Curr. Obstetrics Gynecol. Rep. 2, 129–138. doi:10.1007/s13669-013-0045-8
Hemalatha K. (2012). Studies on the protective efficacy of Spirulina against lead acetate induced hepatotoxicity in Rattus norvegicus. Int. J. Chem. Anal. Sci. 3, 1509–1512.
Hesser E. F. (1960). Methods for routine fish hematology. Progress. Fish-Culturist 22, 164–171. doi:10.1577/1548-8659(1960)22[164:mfrfh]2.0.co;2
Hirt N., Body-Malapel M. (2020). Immunotoxicity and intestinal effects of nano-and microplastics: a review of the literature. Part. fibre Toxicol. 17, 57–22. doi:10.1186/s12989-020-00387-7
Horn D. A., Granek E. F., Steele C. L. (2020). Effects of environmentally relevant concentrations of microplastic fibers on Pacific mole crab (Emerita analoga) mortality and reproduction. Limnol. Oceanogr. Lett. 5, 74–83. doi:10.1002/lol2.10137
Hoseini S. M., Khosravi-Darani K., Mozafari M. R. (2013). Nutritional and medical applications of spirulina microalgae. Mini Rev. Med. Chem. 13, 1231–1237. doi:10.2174/1389557511313080009
Huang J., Murata H., Koepsel R. R., Russell A. J., Matyjaszewski K. (2007). Antibacterial polypropylene via surface-initiated atom transfer radical polymerization. Biomacromolecules 8, 1396–1399. doi:10.1021/bm061236j
Hwang J., Choi D., Han S., Choi J., Hong J. (2019). An assessment of the toxicity of polypropylene microplastics in human derived cells. Sci. Total Environ. 684, 657–669. doi:10.1016/j.scitotenv.2019.05.071
Ismail R. F., Saleh N. E., Sayed A.E.-D. H. (2021). Impacts of microplastics on reproductive performance of male tilapia (Oreochromis niloticus) pre-fed on Amphora coffeaeformis. Environ. Sci. Pollut. Res. 28, 68732–68744. doi:10.1007/s11356-021-14984-2
Jabeen K., Li B., Chen Q., Su L., Wu C., Hollert H., et al. (2018). Effects of virgin microplastics on goldfish (Carassius auratus). Chemosphere 213, 323–332. doi:10.1016/j.chemosphere.2018.09.031
Jemec Kokalj A., Dolar A., Drobne D., Marinšek M., Dolenec M., Škrlep L., et al. (2022). Environmental hazard of polypropylene microplastics from disposable medical masks: acute toxicity towards Daphnia magna and current knowledge on other polypropylene microplastics. Microplast nanoplast 2, 021–00020. doi:10.1186/s43591-021-00020-0
Jeyavani J., Sibiya A., Stalin T., Vigneshkumar G., Al-Ghanim K. A., Riaz M. N., et al. (2023). Biochemical, genotoxic and histological implications of polypropylene microplastics on freshwater fish Oreochromis mossambicus: an aquatic eco-toxicological assessment. Toxics 11, 282. doi:10.3390/toxics11030282
Karami A., Romano N., Galloway T., Hamzah H. (2016). Virgin microplastics cause toxicity and modulate the impacts of phenanthrene on biomarker responses in African catfish (Clarias gariepinus). Environ. Res. 151, 58–70. doi:10.1016/j.envres.2016.07.024
Khafaga A. F., El-Sayed Y. S. (2018). Spirulina ameliorates methotrexate hepatotoxicity via antioxidant, immune stimulation, and proinflammatory cytokines and apoptotic proteins modulation. Life Sci. 196, 9–17. doi:10.1016/j.lfs.2018.01.010
Kim J.-H., Yu Y.-B., Choi J.-H. (2021a). Toxic effects on bioaccumulation, hematological parameters, oxidative stress, immune responses and neurotoxicity in fish exposed to microplastics: a review. J. Hazard. Mater. 413, 125423. doi:10.1016/j.jhazmat.2021.125423
Kim S.-H., Kim G.-H., Kim J.-S., Kim J.-H., Jeon Y.-H., Cho J.-H., et al. (2021b). Changes in hematological parameters and plasma components of olive flounder, Paralichthys olivaceus exposed to acute microplastics. Korean J. Environ. Biol. 39, 344–353. doi:10.11626/kjeb.2021.39.3.344
Kim S. W., Waldman W. R., Kim T.-Y., Rillig M. C. (2020). Effects of different microplastics on nematodes in the soil environment: tracking the extractable additives using an ecotoxicological approach. Environ. Sci. Technol. 54, 13868–13878. doi:10.1021/acs.est.0c04641
Kuriakose G. C., Kurup M. G. (2011). Antioxidant and antihepatotoxic effect of Spirulina laxissima against carbon tetrachloride induced hepatotoxicity in rats. Food and Funct. 2, 190–196. doi:10.1039/c0fo00163e
Lee G. R., Foerster J., Lukens a. (1999). “Wintrobe’s clinical hematology,” in Thrombocytopenia caused by immunologic platelet destruction. S. P. Levin 10th Edn (Baltimore: Williams and Wilkins), 1583–1611.
Lee J.-H., Kang J.-C., Kim J.-H. (2023). Toxic effects of microplastic (Polyethylene) on fish: accumulation, hematological parameters and antioxidant responses in Korean Bullhead, Pseudobagrus fulvidraco. Sci. Total Environ. 877, 162874. doi:10.1016/j.scitotenv.2023.162874
Lei L., Wu S., Lu S., Liu M., Song Y., Fu Z., et al. (2018). Microplastic particles cause intestinal damage and other adverse effects in zebrafish Danio rerio and nematode Caenorhabditis elegans. Sci. Total Environ. 619, 1–8. doi:10.1016/j.scitotenv.2017.11.103
Lobelle D., Cunliffe M. (2011). Early microbial biofilm formation on marine plastic debris. Mar. Pollut. Bull. 62, 197–200. doi:10.1016/j.marpolbul.2010.10.013
Maddah H. A. (2016). Polypropylene as a promising plastic: a review. Am. J. Polym. Sci. 6, 1–11. doi:10.5923/j.ajps.20160601.01
Mahmoud Y. I., Abd El-Ghffar E. A. (2019). Spirulina ameliorates aspirin-induced gastric ulcer in albino mice by alleviating oxidative stress and inflammation. Biomed. Pharmacother. = Biomedecine Pharmacother. 109, 314–321. doi:10.1016/j.biopha.2018.10.118
Maity S., Pramanick K. (2020). Perspectives and challenges of micro/nanoplastics-induced toxicity with special reference to phytotoxicity. Glob. Change Biol. 26, 3241–3250. doi:10.1111/gcb.15074
Martakis N., Niaounakis M., Pissimissis D. (1994). Gamma-sterilization effects and influence of the molecular weight distribution on the postirradiation resistance of polypropylene for medical devices. J. Appl. Polym. Sci. 51, 313–328. doi:10.1002/app.1994.070510214
Martin S. J., Sabina E. P. (2016). Amelioration of anti-tuberculosis drug induced oxidative stress in kidneys by Spirulina fusiformis in a rat model. Ren. Fail. 38, 1115–1121. doi:10.1080/0886022X.2016.1184940
Mazzaglia A., Zagami R., Romeo A., Ceraolo F., Vazzana M., Castriciano M. A., et al. (2018). Supramolecular adducts of anionic porphyrins and a biocompatible polyamine: effect of photodamage-on human red blood cells. J. Nanosci. Nanotechnol. 18, 7269–7274. doi:10.1166/jnn.2018.15747
Mekkawy I. A., Mahmoud U. M., Osman A. G., Sayed A.E.-D. H. (2010). Effects of ultraviolet A on the activity of two metabolic enzymes, DNA damage and lipid peroxidation during early developmental stages of the African catfish, Clarias gariepinus (Burchell, 1822). Fish physiology Biochem. 36, 605–626. doi:10.1007/s10695-009-9334-6
Mekkawy I. A., Mahmoud U. M., Sayed A.E.-D. H. (2011). Effects of 4-nonylphenol on blood cells of the African catfish Clarias gariepinus (Burchell, 1822). Tissue cell 43, 223–229. doi:10.1016/j.tice.2011.03.006
Meraj M., Ali M. N., Ganai B. A., Bhat F. A., Ganaie H. A., Ahmed A. (2017). Alterations in liver marker enzymes and recovery ability of cyprinids as indicators of aquatic pollution. Environ. Sci. Pollut. Res. Int. 24, 19149–19158. doi:10.1007/s11356-017-9572-6
Mohamed I. A., Hamed M., Abdel-Tawab H. S., Mansour S., Soliman H. A. M., Lee J.-S., et al. (2022). Multi-biomarkers approach to assess the toxicity of novel insecticide (Voliam flexi®) on Clarias gariepinus: from behavior to immunotoxicity. Fish Shellfish Immunol. 125, 54–64. doi:10.1016/j.fsi.2022.04.051
Mohamed W. A., Ismail S. A., El-Hakim Y. M. A. (2014). Spirulina platensis ameliorative effect against GSM 900-MHz cellular phone radiation-induced genotoxicity in male Sprague-Dawley rats. Comp. Clin. Pathol. 23, 1719–1726. doi:10.1007/s00580-014-2003-x
Mokhbatly A.-A. A., Assar D. H., Ghazy E. W., Elbialy Z., Rizk S. A., Omar A. A., et al. (2020). The protective role of spirulina and β-glucan in African catfish (Clarias gariepinus) against chronic toxicity of chlorpyrifos: hemato-biochemistry, histopathology, and oxidative stress traits. Environ. Sci. Pollut. Res. 27, 31636–31651. doi:10.1007/s11356-020-09333-8
Mukherjee D., Saha S., Chukwuka A. V., Ghosh B., Dhara K., Saha N. C., et al. 2022. Antioxidant enzyme activity and pathophysiological responses in the freshwater walking catfish, Clarias batrachus Linn under sub-chronic and chronic exposures to the neonicotinoid, Thiamethoxam. Sci. Total Environ. 836, 5. doi:10.1016/j.scitotenv.2022.155716
Oliveira M., Ribeiro A., Hylland K., Guilhermino L. (2013). Single and combined effects of microplastics and pyrene on juveniles (0+ group) of the common goby Pomatoschistus microps (Teleostei, Gobiidae). Ecol. Indic. 34, 641–647. doi:10.1016/j.ecolind.2013.06.019
Osman A., Hamed M., Sayed A. (2019). Protective role of Spirulina platensis against UVA-induced haemato-biochemical and cellular alterations in Clarias gariepinus. J. Photochem. Photobiol. B Biol. 191, 59–64. doi:10.1016/j.jphotobiol.2018.12.013
Pankhania M., Stephenson T., Semmens M. J. (1994). Hollow fibre bioreactor for wastewater treatment using bubbleless membrane aeration. Water Res. 28, 2233–2236. doi:10.1016/0043-1354(94)90037-x
Papageorgiou D. G., Chrissafis K., Bikiaris D. N. (2015). β-nucleated polypropylene: processing, properties and nanocomposites. Polym. Rev. 55, 596–629. doi:10.1080/15583724.2015.1019136
Pari L., Amali D. R. (2005). Protective role of tetrahydrocurcumin (THC) an active principle of turmeric on chloroquine induced hepatotoxicity in rats. J. Pharm. Pharm. Sci. 8, 115–123.
Peiretti P., Meineri G. (2011). Effects of diets with increasing levels of Spirulina platensis on the carcass characteristics, meat quality and fatty acid composition of growing rabbits. Livest. Sci. 140, 218–224. doi:10.1016/j.livsci.2011.03.031
Peng L., Fu D., Qi H., Lan C. Q., Yu H., Ge C. (2020). Micro-and nano-plastics in marine environment: source, distribution and threats—a review. Sci. Total Environ. 698, 134254. doi:10.1016/j.scitotenv.2019.134254
Quest J. A., Tomaszewski J. E., Haseman J. K., Boorman G. A., Douglas J. F., Clarke W. J. (1984). Two-year inhalation toxicity study of propylene in F344/N rats and B6C3F1 mice. Toxicol. Appl. Pharmacol. 76, 288–295. doi:10.1016/0041-008x(84)90010-3
Ramesh P., Vinodh S. (2020). State of art review on life cycle assessment of polymers. Int. J. Sustain. Eng. 13, 411–422. doi:10.1080/19397038.2020.1802623
Ramos M., Jiménez A., Peltzer M., Garrigós M. C. (2012). Characterization and antimicrobial activity studies of polypropylene films with carvacrol and thymol for active packaging. J. food Eng. 109, 513–519. doi:10.1016/j.jfoodeng.2011.10.031
Sathish M. N., Jeyasanta I., Patterson J. (2020). Microplastics in salt of Tuticorin, southeast coast of India. Archives Environ. Contam. Toxicol. 79, 111–121. doi:10.1007/s00244-020-00731-0
Sayed A.E.-D. H., Authman M. M. N. (2018). The protective role of Spirulina platensis to alleviate the Sodium dodecyl sulfate toxic effects in the catfish Clarias gariepinus (Burchell, 1822). Ecotoxicol. Environ. Saf. 163, 136–144. doi:10.1016/j.ecoenv.2018.07.060
Sayed A.E.-D. H., Hamed M., Badrey A. E., Soliman H. A. (2021a). Bioremediation of hemotoxic and oxidative stress induced by polyethylene microplastic in Clarias gariepinus using lycopene, citric acid, and chlorella. Comp. Biochem. Physiology Part C Toxicol. Pharmacol. 250, 109189. doi:10.1016/j.cbpc.2021.109189
Sayed A.E.-D. H., Hamed M., El-Sayed A. A., Nunes B., Soliman H. A. (2023a). The mitigating effect of Spirulina (Arthrospira platensis) on the hemotoxicity of gibberellic acid on juvenile tilapia (Oreochromis niloticus). Environ. Sci. Pollut. Res. 30, 25701–25711. doi:10.1007/s11356-022-23844-6
Sayed A.E.-D. H., Hamed M., Soliman H. A. (2021b). Spirulina platensis alleviated the hemotoxicity, oxidative damage and histopathological alterations of hydroxychloroquine in catfish (Clarias gariepinus). Front. Physiology 12, 683669. doi:10.3389/fphys.2021.683669
Sayed A.E.-D. H., Hana M. N., Hamed M., Abdel-Latif H. M., Lee J.-S., Soliman H. A. (2023b). Protective efficacy of dietary natural antioxidants on microplastic particles-induced histopathological lesions in African catfish (Clarias gariepinus). Environ. Sci. Pollut. Res. 30, 24424–24440. doi:10.1007/s11356-022-23789-w
Sayed A.E.-D. H., Taher H., Soliman H. A., Salah El-Din A.E.-D. (2022b). Immunological and hemato-biochemical effects on catfish (Clarias gariepinus) exposed to dexamethasone. Front. Physiology 1958. doi:10.3389/fphys.2022.1018795
Scanes E., Wood H., Ross P. (2019). Microplastics detected in haemolymph of the Sydney rock oyster Saccostrea glomerata. Mar. Pollut. Bull. 149, 110537. doi:10.1016/j.marpolbul.2019.110537
Seshadri C. V., Umesh B. V., Manoharan R. (1991). Beta-carotene studies in spirulina. Bioresour. Technol. 38, 111–113. doi:10.1016/0960-8524(91)90140-f
Setälä O., Fleming-Lehtinen V., Lehtiniemi M. (2014). Ingestion and transfer of microplastics in the planktonic food web. Environ. Pollut. 185, 77–83. doi:10.1016/j.envpol.2013.10.013
Shao Z.-B., Deng C., Tan Y., Chen M.-J., Chen L., Wang Y.-Z. (2014). An efficient mono-component polymeric intumescent flame retardant for polypropylene: preparation and application. ACS Appl. Mater. interfaces 6, 7363–7370. doi:10.1021/am500789q
Soliman H. A., Hamed M., Sayed A.E.-D. H. (2021). Investigating the effects of copper sulfate and copper oxide nanoparticles in Nile tilapia (Oreochromis niloticus) using multiple biomarkers: the prophylactic role of Spirulina. Environ. Sci. Pollut. Res. 28, 30046–30057. doi:10.1007/s11356-021-12859-0
Soliman H. A., Salaah S. M., Hamed M., Sayed A.E.-D. H. (2023). Toxicity of co-exposure of microplastics and lead in African catfish (Clarias gariepinus). Front. Veterinary Sci. 10, 1279382. doi:10.3389/fvets.2023.1279382
Soliman H. A., Sayed A.E.-D. H. (2020). Poikilocytosis and tissue damage as negative impacts of tramadol on juvenile of Tilapia (Oreochromis niloticus). Environ. Toxicol. Pharmacol. 78, 103383. doi:10.1016/j.etap.2020.103383
Song Y., Begley T., Paquette K., Komolprasert V. (2003). Effectiveness of polypropylene film as a barrier to migration from recycled paperboard packaging to fatty and high-moisture food. Food Addit. Contam. 20, 875–883. doi:10.1080/02652030310001597592
Statista (2021). Statista. Available at: https://www.statista.com/statistics/1103529/global-polypropyleneproduction/.
Stello S. L., Latimer K. S., Bain P. J., Krimer P. M. (2003) The significance of echinocytosisin blood smears. Department of pathology. Athens: College of Veterinary Medicine, University of Georgia.
Thummabancha K., Onparn N., Srisapoome P. (2016). Analysis of hematologic alterations, immune responses and metallothionein gene expression in Nile tilapia (Oreochromis niloticus) exposed to silver nanoparticles. J. Immunotoxicol. 13, 909–917. doi:10.1080/1547691X.2016.1242673
Tiwari M., Rathod T., Ajmal P., Bhangare R., Sahu S. (2019). Distribution and characterization of microplastics in beach sand from three different Indian coastal environments. Mar. Pollut. Bull. 140, 262–273. doi:10.1016/j.marpolbul.2019.01.055
Wang Z.-M., Wagner J., Ghosal S., Bedi G., Wall S. (2017). SEM/EDS and optical microscopy analyses of microplastics in ocean trawl and fish guts. Sci. Total Environ. 603, 616–626. doi:10.1016/j.scitotenv.2017.06.047
Wilson J. M., Bunte R. M., Carty A. J. (2009). Evaluation of rapid cooling and tricaine methanesulfonate (MS222) as methods of euthanasia in zebrafish (Danio rerio). J. Am. Assoc. Lab. Anim. Sci. 48, 785–789.
Yang J., Karbalaei S., Hussein S. M., Ahmad A. F., Walker T. R., Salimi K. (2023). Biochemical effects of polypropylene microplastics on red tilapia (Oreochromis niloticus) after individual and combined exposure with boron. Environ. Sci. Eur. 35, 71. doi:10.1186/s12302-023-00771-y
Yedier S., Yalçınkaya S. K., Bostancı D. (2023). Exposure to polypropylene microplastics via diet and water induces oxidative stress in Cyprinus carpio. Aquat. Toxicol. 259, 106540. doi:10.1016/j.aquatox.2023.106540
Yousefi R., Saidpour A., Mottaghi A. (2019). The effects of Spirulina supplementation on metabolic syndrome components, its liver manifestation and related inflammatory markers: a systematic review. Complementary Ther. Med. 42, 137–144. doi:10.1016/j.ctim.2018.11.013
Zalasiewicz J., Waters C. N., Do Sul J. A. I., Corcoran P. L., Barnosky A. D., Cearreta A., et al. (2016). The geological cycle of plastics and their use as a stratigraphic indicator of the Anthropocene. Anthropocene 13, 4–17. doi:10.1016/j.ancene.2016.01.002
Zhang C. (1994). The effects of polysaccharide and phycocyanin from Spirulina platensis variety on peripheral blood and hematopoietic system of bone marrow in mice. Second Asia-Pacific Conf. Alga Biotechnol., 25–27.
Zhang F., Man Y. B., Mo W. Y., Wong M. H. (2020). Application of Spirulina in aquaculture: a review on wastewater treatment and fish growth. Rev. Aquac. 12, 582–599. doi:10.1111/raq.12341
Keywords: polypropylene, hemato-biochemical, liver, fish, Spirulina
Citation: Eid Z, Mahmoud UM and Sayed AE-DH (2024) Deleterious effects of polypropylene released from paper cups on blood profile and liver tissue of Clarias gariepinus: bioremediation using Spirulina. Front. Physiol. 15:1380652. doi: 10.3389/fphys.2024.1380652
Received: 01 February 2024; Accepted: 29 April 2024;
Published: 22 May 2024.
Edited by:
Rui Jia, Chinese Academy of Fishery Sciences (CAFS), ChinaReviewed by:
Gopalakrishnan Singaram, Krishkash Envirotech Private Limited, IndiaMajid Rasta, China Three Gorges University, China
Marco Antonio De Andrade Belo, Universidade Camilo Castelo Branco, Brazil
Serdar Yedier, Ordu University, Türkiye
Copyright © 2024 Eid, Mahmoud and Sayed. This is an open-access article distributed under the terms of the Creative Commons Attribution License (CC BY). The use, distribution or reproduction in other forums is permitted, provided the original author(s) and the copyright owner(s) are credited and that the original publication in this journal is cited, in accordance with accepted academic practice. No use, distribution or reproduction is permitted which does not comply with these terms.
*Correspondence: Alaa El-Din H. Sayed, YWxhYXNheWVkQGF1bi5lZHUuZWc=