- School of Sports Medicine and Rehabilitation, Beijing Sport University, Beijing, China
Background: High-intensity resistance training is known to be the most effective method for enhancing muscle strength and thickness, but it carries potential injury risks. Blood flow restriction (BFR) combined with resistance training has been proposed as a safer alternative method for improving muscle strength and thickness.
Methods: A meta-analysis was conducted, including 20 studies from five databases that met the inclusion criteria, to assess the efficacy of BFR combined with resistance training compared to traditional resistance training (NOBFR). The analysis focused on changes in muscle strength and thickness. Subgroup analysis and meta-regression were performed to explore the effects of tourniquet width and pressure.
Results: The findings showed that BFR combined with resistance training is comparable to traditional resistance training in enhancing muscle strength [0.11, 95%CI: (−0.08 to 0.29), I2 = 0%] and muscle thickness [−0.07, 95% CI: (−0.25 to 0.12), I2 = 0%]. Subgroup analysis indicated no significant differences in muscle strength (P = 0.66) and thickness (P = 0.87) between low-intensity BFR training and other intensity levels. Meta-regression suggested that tourniquet width and pressure might affect intervention outcomes, although the effects were not statistically significant (P > 0.05).
Conclusion: BFR combined with resistance training offers a viable alternative to high-intensity resistance training with reduced injury risks. We recommend interventions of 2-3 sessions per week at 20%–40% of 1 RM, using a wider cuff and applying an arterial occlusion pressure of 50%–80% to potentially enhance muscle strength and thickness. It is also recommended to release tourniquet pressure during rest intervals to alleviate discomfort. This protocol effectively improves muscle strength with minimal cardiac workload and reduced risk of adverse events.
Systematic Review Registration:: [https://www.crd.york.ac.uk/prospero/display_record.php?ID=CRD42023495465], identifier [CRD42023495465].
1 Introduction
Muscle, as a major component of the locomotor system, muscle mass determines the athletic capability and sports performance (van der Zwaard et al., 2021; Khare et al., 2023). Muscle mass is critical for both athletes and chronically ill people. For athletes, muscle mass determines performance to influence competitive results. For chronically ill or elderly populations, muscle mass correlates with longevity (Cruz-Jentoft and Sayer, 2019; Fabero-Garrido et al., 2022a; Alizadeh Pahlavani, 2022). Traditional resistance training has been validated as an effective non-pharmacological intervention for enhancing muscle mass and strength (Fragala et al., 2019). High-intensity (HI) resistance training has demonstrated superior effect on muscle strength and thickness improvement compared to moderate-to low-intensity (LI) resistance training (Csapo and Alegre, 2016). However, it is imperative to note that HI resistance training may induce pain and injuries to populations with chronic disease, such as hypertension or osteoarthritis (Wang et al., 2022a; Wang et al., 2022b). Consequently, it is crucial to explore alternative approaches that yield benefits akin to HI resistance training while mitigating associated risks.
Recent studies have revealed that LI resistance training, when combined with blood flow restriction (BFR), triggers heightened metabolic stress, thereby modulating signal transduction in musculoskeletal cells and achieving muscle strength and thickness improvements comparable to those achieved through HI resistance training (Krzysztofik et al., 2019; Koc et al., 2022; Krzysztofik et al., 2022). BFR is an intervention method that involves the application of restrictive equipment to reduce blood flow in the proximal segment of the limb (Lorenz et al., 2021). This intervention indirectly influences cellular metabolism by accumulating metabolites and simulates a localized hypoxic environment during the reduction of blood flow in the proximal limb segment (Krzysztofik et al., 2019; Lorenz et al., 2021). The combination of BFR with resistance exercise has been implemented across diverse populations in both clinical and non-clinical setting (Krzysztofik et al., 2019; Krzysztofik et al., 2022; Colapietro et al., 2023). Consequently, BFR coupled with LI resistance training emerges as a safe alternative treatment, presenting a viable option to traditional resistance training protocols for enhancing muscle and physical functions among patients in the clinical setting or sedentary populations.
Despite the growing utilization of BFR in combination with resistance training in various studies and clinical settings, there remains a conspicuous absence of standardized criteria regarding training protocols and BFR equipment among participants. Key parameters such as occlusion pressure, cuff width, and the choice of resistance level are pivotal in determining the effectiveness of BFR interventions. Variations in treatment protocols, including occlusion pressure [often recommended to be between 50%–80% of arterial occlusion pressure (Li et al., 2023)], cut width, the number of sets and repetitions performed, total training sessions, and duration, may contribute to differences in the treatment’s efficacy within clinical applications (Brumitt et al., 2021a). Moreover, a dearth of evidence exists to comprehensively evaluate the impact of BFR combined with resistance training at various intensity levels on improving muscle strength and thickness. This study employs meta-regression analysis to evaluate the impact of these parameters on muscle strength and thickness improvement, aiming to provide a clearer understanding of how different training and equipment characteristics affect outcomes. The influence of training and equipment characteristics on muscle function improvement remains a crucial area requiring further exploration and in-depth discussion.
This study aims to address these gaps by examining the influence of treatment characteristics on muscle strength and thickness improvement through a systematic review and meta-analysis. A subsequent meta-regression analysis intends to rigorously investigate the association between specific protocol details and muscular improvement. The insights gleaned from this study not only contribute to the current understanding of resistance training with BFR but also furnish valuable information for designing effective and targeted treatment programs.
2 Method
This review was prospectively registered with PROSPERO (ref. CRD42023495465) and was reported in accordance with the Preferred Reporting Items for Systematic Reviews and Meta-Analyses (PRISMA) guidelines (Moher et al., 2009) and the Cochrane Handbook (JPT et al., 2024).
The screening of studies, quality assessment, and data extraction were independently conducted by two researchers, FM and JH. Any discrepancies in assessments were resolved through discussion. In instances where a consensus could not be reached, a third researcher, YW, was consulted to facilitate an agreement.
2.1 Data sources and study selection
The search was initiated on 8 October 2023, using multiple databases including PubMed, Web of Science, EBSCO, Embase, and the Cochrane Library. The search involved keywords such as “Blood Flow Restriction,” “hypoxia,” and “resistance training,” and was limited to studies published between 1985 and 2023. The detailed search strategies for each database are summarized in Table 1.
An example of the study search strategy and the results obtained using the PubMed database is provided in Supplementary Appendix Table S1.
Prior to commencing the screening process, studies were included if they met the following criteria: 1) participants were healthy adults aged 18+ years; 2) the intervention involved resistance training combined with BFR; 3) the intervention of control group received usual care without BFR (NOBFR); 4) the study outcomes centered on muscle thickness (cross-sectional area (CSA) or girth) and muscle strength (1 repetition maximum (RM) or maximal voluntary torque (MVT)); 5) the studies were randomized controlled trials; 6) the studies were available in English.
Studies were exclued, if: 1) participants had chronic diseases or pain, such as hypertension; 2) the intervention was acute exercise and aerobic training with BFR; 3) the studies were observational or cohort trails, conference reports, and review articles; 4) the studies were published in a language other than English.
The searched studies were evaluated against these criteria in two phases: 1) assessment of each study’s abstract and title and 2) evaluation of the full text of potentially relevant studies. The search and screening process was documented using the PRISMA flow diagram (Page et al., 2021). The researchers used Endnote software for the screening process.
2.2 Quality assessment and data extraction
2.2.1 Assessment of bias
The methodological quality of the included studies underwent rigorous assessment by the researchers using two tools: the Cochrane Risk of Bias 2 (RoB 2) tool (Higgins et al., 2011) and the PEDro (Physiotherapy Evidence Database) scale (Maher et al., 2003). Each tool’s guidelines were followed during the assessment process, evaluating five key domains: the randomization process, confounding factors, sample selection, missing data, and measurement of outcomes. Utilizing the Cochrane RoB 2 tool, the risk was classified as “low,” “some concerns,” or “high,” providing a detailed overview of the methodological quality of the included studies. Additionally, the 11-item PEDro scale (http://www.pedro.fhs.usyd.edu.au) was employed to gauge methodological quality (de Morton, 2009), with items scored as either present (“1”) or non-present (“0”), allowing for a comprehensive assessment of each study. The methodological quality was categorized as “low” (total score less than 4), “some concerns,” and “high” (total score greater than 8). The evaluation results are detailed in Figure 2; Table 1. This rigorous approach ensures the reliability and validity of the meta-analysis findings.
2.2.2 Data extraction and synthesis
Data extracted from each included study were divided into two categories: participant characteristics and trial characteristics. Participant characteristics encompassed crucial information such as sample age, body weight, BMI, and sample size. It is noted that several studies included multiple intervention groups and self-control groups. To prevent duplication of samples, the sample size of self-control studies was averaged equally. For studies with different intervention groups, each intervention was reported separately, and the control group’s sample size was also equally distributed. Trail characteristics encompassed training intensity, tourniquet width, occlusion pressure, tourniquet application time, training volume, rest duration between sets, training duration, frequency, and the specific outcomes obtained in each study. Continuous numerical data were extracted using mean and standard deviation (SD). Further details regarding the extracted data are showed in Table 2. Investigation into the effect of trial characteristics of BFR intervention on muscle strength and thickness improvement necessitated the transformation of certain trail characteristics into binary variables for data analysis. Specifically, the training intensity with BFR was dichotomized into “Low-intensity with BFR” (50% 1 RM) or “Other intensity with BFR” [including middle-(50–69% 1RM) and high-intensity with BFR (70–84% 1RM)]. The inflation of the tourniquet during exercise interventions was categorized as either “Inflated for the entire exercise protocol” or “Inflated during exercise and deflated during rest periods.”
For the meta-analysis and meta-regression analysis, this study utilized the mean and SD of the post-intervention test results for each study’s intervention and control groups. The outcomes measured included including muscle CSA and girth, as well as muscle strength (including 1 RM and MVT). Notably, the meta-analysis was conducted separately for muscle thickness and muscle strength.
2.3 Data analysis
The initial meta-analysis of the extracted data took into consideration the methodological heterogeneity arising from diverse muscle measurement and testing methods. To address this, a standardized mean difference (SMD) (Bakbergenuly et al., 2020) was employed for data analysis. This approach utilizes statistical units to standardize various clinical units, effectively reducing discrepancies caused by differing testing methods and clinical units (Andrade, 2020). The SMD calculation is the following:
Following this, the present study evaluated the heterogeneity of the included studies’ results using Cochran’s Q statistic (Schulzke and Patole, 2021), a well-established tool for accurately gauging statistical heterogeneity, alongside Higgins and Thompson’s I2 statistic to quantify the heterogeneity level (Bowden et al., 2011). Meanwhile, forest plots were used for visually representation of the analysis results. The I2 statistic was used to quantify the degree of heterogeneity across studies, with values of 25%, 50%, and 75% denoting low, moderate, and high heterogeneity (Higgins et al., 2003), respectively. In instances where the I2 value exceeded 75%, indicating significant inconsistency among these studies, a reassessment was conducted using a random-effects model. After that, publication bias and sensitivity analyses were performed to comprehend the reasons behind the observed heterogeneity. Specifically, to evaluate the potential for publication bias, a meta-bias assessment was conducted in the study. A funnel plot was constructed and the Egger’s test was used to detect statistically significant publication bias (P < 0.1) (JPT et al., 2024). Furthermore, a sensitivity analysis was executed to explore the impact of each study on bias. Studies demonstrating high inconsistency were considered for potential exclusion in the final analysis. Researchers assigned weights to each included study, where studies with high inconsistency were weighted as 0 while others were weighted as 1 for the formal analysis.
2.4 Meta-regression analysis
This study conducted a meta-regression analysis to delve into the relationship between trial characteristics and the improvement of muscle strength and thickness. Meta-regression enables the exploration of potential sources of heterogeneity by quantifying the impact of various factors on intervention effects. The Knapp-Hartung modification was incorporated to bolster the robustness of the analysis. The primary objective of this analysis was to discern significant associations between individual trial characteristics and the outcomes, thereby offering valuable insights into the factors influencing muscle response across the included studies.
2.5 Statistical analysis
This study employed a combination of software tools to execute various aspects of the data analysis process. Endnote (Clarivate, Philadelphia, United States) was utilized for reference management and the screening process. The methodological quality assessment of the included studies was conducted using Excel to implement RoB 2, and the Robvis package (McGuinness and Higgins, 2020) in R Studio (R version 4.2.3; RStudio, PBC, Boston, MA, United States) was adopted for visualizing the RoB assessment. The results were exhibited in two figures: a summary of five domains and a traffic light figure displaying each study’s risk. R studio served as the primary tool for conducting the meta-analysis, publication bias analysis, sensitivity analysis, and figure generation. Stata 18 (StataCorp, College Station, TX, United States) was utilized specifically for the meta-regression analysis to explore the relationships between moderators and outcomes. All results were calculated with 95% confidence intervals (CI). For the determination of statistical significance in BFR intervention effects, the threshold was set at P < 0.05. In addition, statistical significance for heterogeneity was defined as P < 0.1 or I2 > 75%, and that for publication bias was defined as P < 0.1.
3 Results
3.1 Search results
The study identified 20 randomized controlled trails that met the specified inclusion criteria. Comprehensive searches using keywords across five databases yielded a total of 5,590 literature records. Following a careful screening process, 3,528 duplicate studies were removed, employing both automated and manual procedures facilitated by Endnote. Following this, three researchers conducted an initial screening of the included studies based on the predetermined criteria, assessing the title and abstract of each literature record. Among these, 80 records were excluded due to incomplete reporting of the study, and 284 records were screened in full text. During the full-text assessment, 23 articles were excluded due to insufficient content, as they only included change data between pre-training and post-training without detailed experimental results. Additionally, 50 studies were excluded due to inappropriate intervention for this review, such as BFR combined with aerobic training or walking trials, and 42 trials were excluded due to inappropriate participants, such as pre- and post-knee surgery patients. The detailed screening process is illustrated in Figure 1.
3.2 Risk assessment for study quality
The methodological quality of the included studies has been meticulously assessed and is presented in Figure 2; Table 2. According to the RoB 2 assessment, ten studies (50%) were determined to exhibit a low risk of bias in their methodology, while only two studies (10%) were deemed to have a high risk. The remaining studies were found to have some concerns regarding their methodological quality. In addition, the PEDro scale scores of the included studies ranged from 6 to 9, with an average score of 7.6 ± 0.75. This range indicated a predominantly high methodological quality across the selected studies. Specifically, nine studies (45%) exhibited some concerns with their methodological quality, while five studies (25%) failed to employ random allocation groups. It is noteworthy that only one study (Lixandrão et al., 2015) scored 6 points and was assessed as having some concerns at RoB 2. This particular study might have contributed to the observed heterogeneity and potentially influenced the overall robustness of the outcomes.
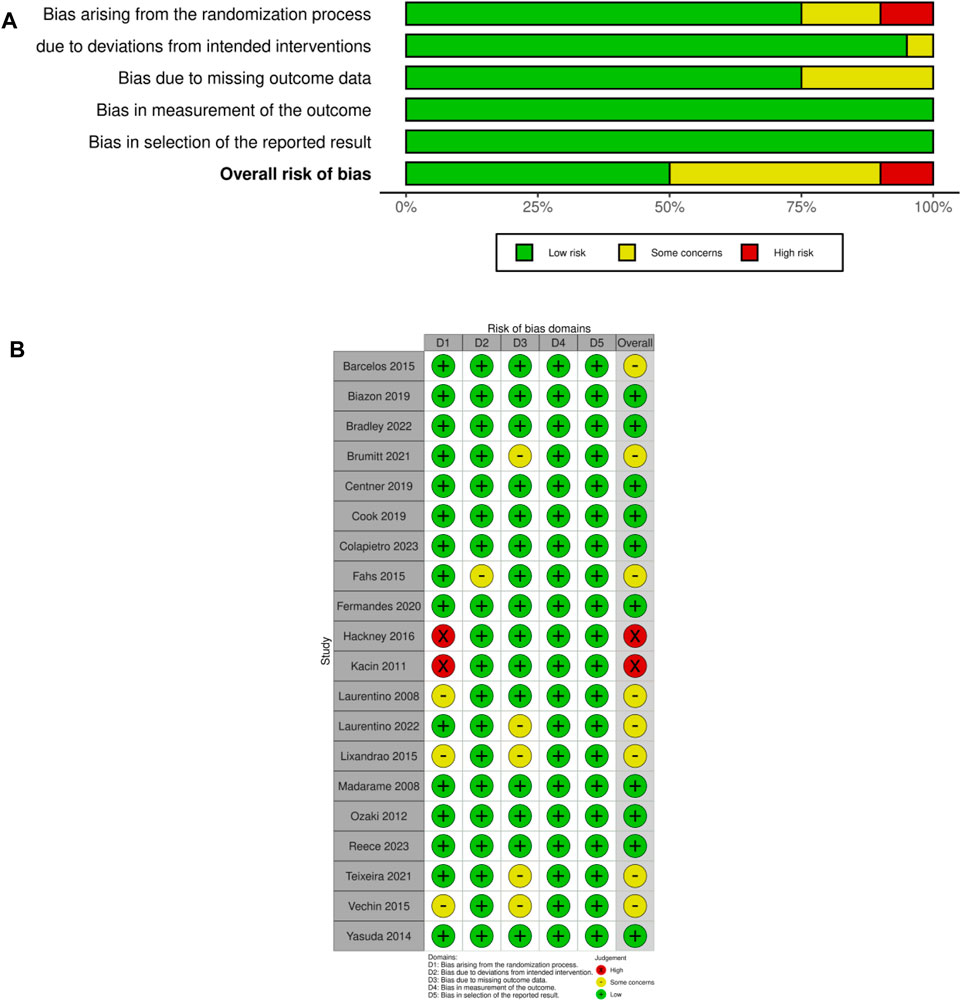
Figure 2. (A) RoB 2 summary plot; (B) RoB 2 traffic light figure. Note: The figures were produced using R packages (McGuinness and Higgins, 2020).
3.3 Participant and trial characteristics
A total of 28 intervention groups from the 20 studies were reported in the analysis. The overall meta-analysis of the 20 studies included 515 participants, with study sizes varying from 4 to 19 subjects. Participant demographics revealed an age of 29.92 ± 2.22 (95% CI, 25.56–34.27), an body weight of 69.97 ± 1.59 (95% CI, 66.85–73.08, Q statistic = 52.75, P for heterogeneity = 0.07), and a BMI of 23.89 ± 0.66 (95% CI, 22.60–25.18, Q statistic = 65.98, P for heterogeneity <0.0001). Table 3 shows the participant characteristics and trials characteristics of the 20 included studies that met the selection criteria.
The current BFR training protocol is based on traditional resistance training protocol standards. The frequency of training sessions among the included studies varied between 2 and 4 times a week, with only one study implementing a training frequency of 4 times per week. Regarding the intensity of BFR combined with resistance training, a range of 20%–80% of 1 RM was observed among the intervention groups in the included studies. Among these groups, 23 intervention groups had intensities below 60% of 1 RM, indicating low-intensity resistance training (Schoenfeld et al., 2017). Only 2 intervention groups had intensities of 60%–80% of 1 RM, suggesting middle-intensity training. The remaining groups exhibited the BFR of 80% of 1RM, representing high-intensity training. Notably, the limited number of middle-intensity groups precluded specific meta-regression analysis. Therefore, these groups were amalgamated with the high-intensity groups as “other intensity with BFR” for further analysis. Considering the notable disparity in other trial characteristics across intervention groups, these were treated as covariates in subsequent meta-regression analyses.
It was observed that the intervention groups in the included studies utilized different types of tourniquets, with only one study (Brumitt et al., 2021b) omitting specific details regarding the tourniquet used. These tourniquets were tailored in length to match the cross-sectional area of participants’ arms or legs. Consequently, the present study analyzed the influence of tourniquet width on BFR training. In addition to blood pressure monitor’s accompanying pressurized belt, two different types of tourniquet products were applied in different studies, including the Delphi Personalized Tourniquet System with a tourniquet width of 11.43 cm in two studies (Bradley et al., 2022; Colapietro, 2023) and Hokanson TD312 Calculating Cuff Inflator with a tourniquet width of 6 cm in another two studies (Hackney et al., 2016; Cook and Cleary, 2019). In addition, 14 studies (20 intervention groups) had tourniquets inflated throughout training, and 6 studies (8 intervention groups) inflated the tourniquets during exercise and deflated them during the rest periods.
3.4 Effect estimates of BFR intervention to NOBFR intervention from meta-analysis
3.4.1 Heterogeneity and possible publication bias
The fixed-effect and random-effects meta-analyses were performed to assess the overall heterogeneity. The assessment revealed a moderate heterogeneity (I2 = 41%, P = 0.01) for muscle thickness results across the studies and a low heterogeneity (I2 = 0%, P = 0.90) for the muscle strength results. The funnel plot of muscle thickness results highlighted significant heterogeneity in one studies (Yasuda et al., 2014) (Figure 3A), and the Egger’s test results indicated a lack of statistically significant asymmetry in the funnel plot (t = 1.43, df = 26, P = 0.16). Subsequently, the forest plot of sensitivity analysis showed that one study (Yasuda et al., 2014) significantly affected the robustness of the overall results, whereas two studies (Kacin and Strazar, 2011; Hackney et al., 2016) with a high risk assessed by RoB 2 did not impact the overall robustness (Figure 3B). Upon the exclusion of the study contributing to high heterogeneity, the overall heterogeneity reduced to a low level across all intervention groups (I2 = 0%, P = 1.00) (Figure 4). Both the funnel plot and the forest plot of sensitivity analysis for muscle strength showed that none of the studies significantly affected the robustness of the overall results (Figures 4A, B). The Egger’s test did not detect significant asymmetry in the funnel plot.
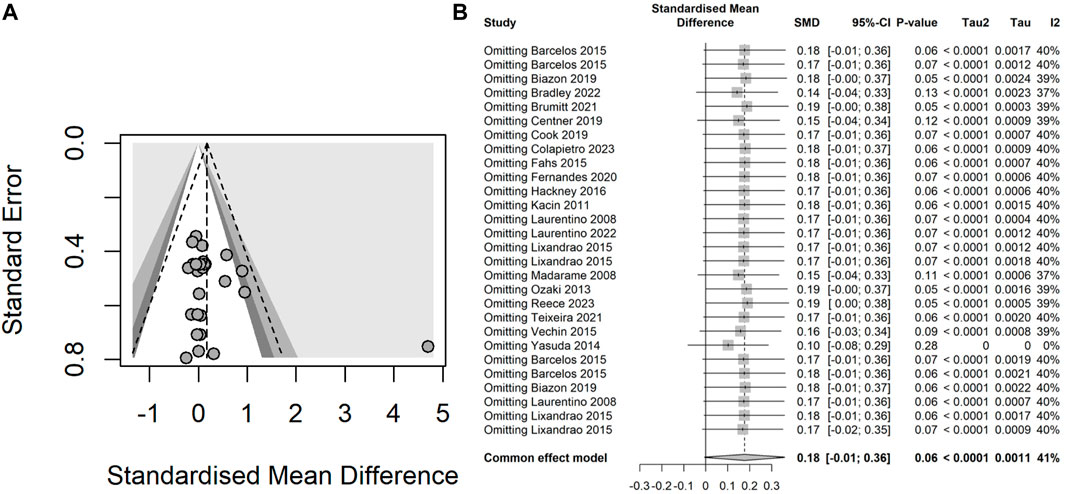
Figure 3. (A) Funnel plot illustrating the heterogeneity of studies within the intervention groups concerning muscle thickness results; (B) Forest plot demonstrating the effect on the overall robustness of the results upon the exclusion of each individual intervention for muscle thickness.
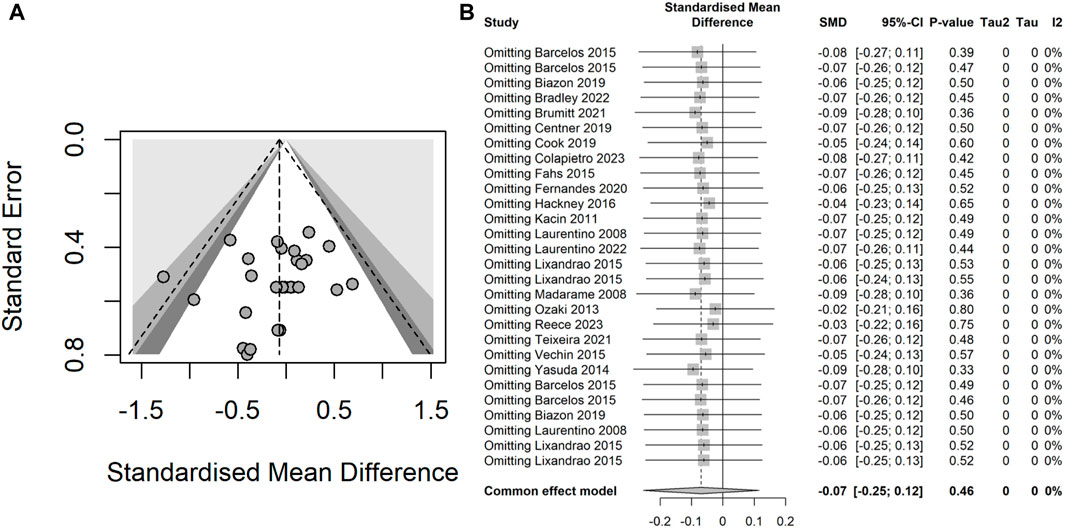
Figure 4. (A) Funnel plot illustrating the heterogeneity of studies within the intervention groups concerning muscle strength results; (B) Forest plot demonstrating the effect on the overall robustness of the results upon the exclusion of each individual intervention for muscle strength.
3.4.2 Subgroup meta-analysis of intervention intensity
The meta-analysis findings uncovered no significant discrepancy between resistance training with BFR and conventional resistance training in terms of enhancements in muscle thickness and strength. The overall contrast in muscle thickness improvement between BFR and conventional training stood at 0.11 (95% CI: −0.08–0.29, I2 = 0%), indicating a no significance impact of conventional training on muscle thickness improvement. However, this difference was not statistically significant (z = 1.03, P = 0.302). Subgroup analysis revealed that BFR training combined with other intensity resistance training displayed a better effect on muscle thickness improvement (0.00, 95% CI: −0.53–0.52, I2 = 0%) than BFR training with low-load resistance training (0.12, 95% CI: −0.07–0.32, I2 = 0%). Nonetheless, this disparity failed to reach statistical significance (
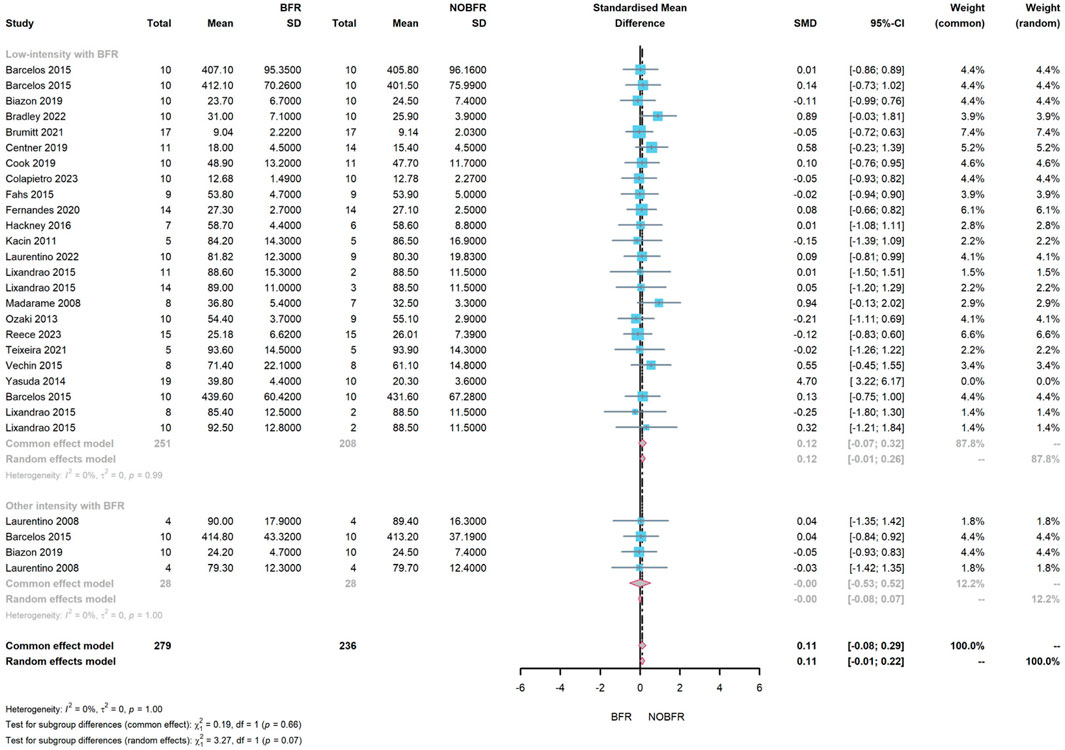
Figure 5. Forest plot demonstrating a subgroup meta-analysis assessing the effect of BFR combined with resistance training at low and other intensities on muscle thickness improvement.
Regarding muscle strength improvement, the overall difference between BFR and routine resistance exercise was −0.07 (95% CI: −0.25–0.12, I2 = 0%). This suggested that resistance exercise with BFR marginally surpassed routine resistance exercise in enhancing muscle strength (z = 0.74, P = 0.461). Subgroup meta-analysis showed that BFR combined with other intensity resistance exercise exhibited a better improvement effect on muscle strength (−0.02, 95% CI: −0.62–0.58, I2 = 0%) than the low-intensity resistance exercise with BFR (−0.07, 95% CI: −0.27–0.12, I2 = 0%). However, this difference did not reach statistical significance (
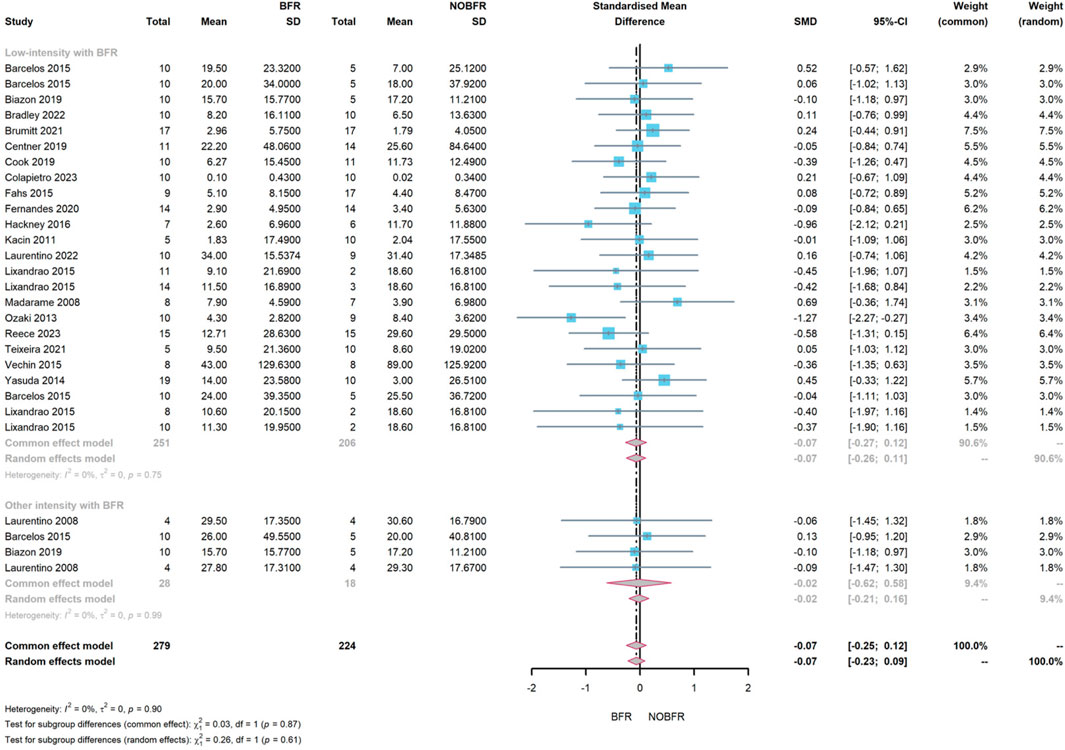
Figure 6. Forest plot illustrating a subgroup meta-analysis evaluating the effect of BFR combined with resistance training at low and other intensities on muscle strength improvement.
3.4.3 Meta-regression for BFR intervention
The meta-regression analysis incorporated a total of 7 variables to examine their effects on muscle thickness and strength improvement (see Tables 4, 5). The regression models are presented in Figure 7. The meta-regression results indicated that none of the moderators exhibited statistically significant links with muscle thickness improvement (P > 0.05). Only the occlusion pressure of tourniquets showed a marginal effect on muscle thickness improvement (coefficient estimate = 0.006, 95% CI = 0–0.012, adjusted R2 = 0.30), which was not statistically significant (P = 0.057). The regression model suggested a slight increase in muscle thickness with increased tourniquet occlusion pressure (see Figure 7). Conversely, the remaining moderators did not statistically significant improve muscle thickness. Furthermore, the moderators did not yield significant impacts on muscle strength improvement (P > 0.05. Adjusted R2 = 0) (see Table 5).
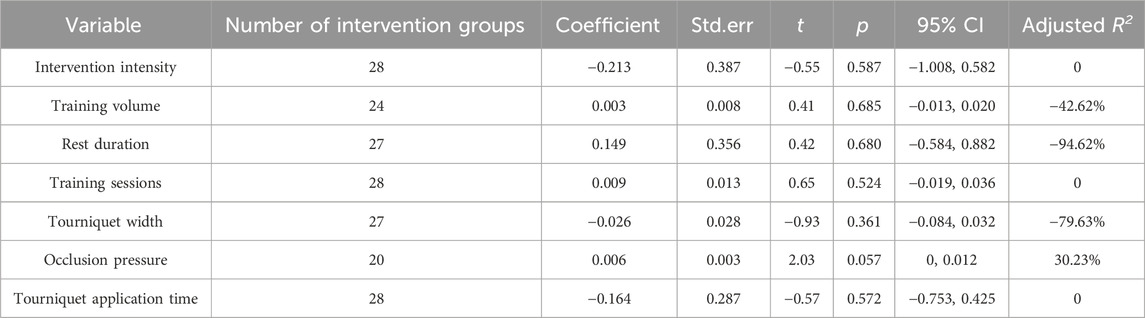
Table 4. Meta-regression of moderators concerning the effects of BFR combined with resistance training on muscle thickness improvement.
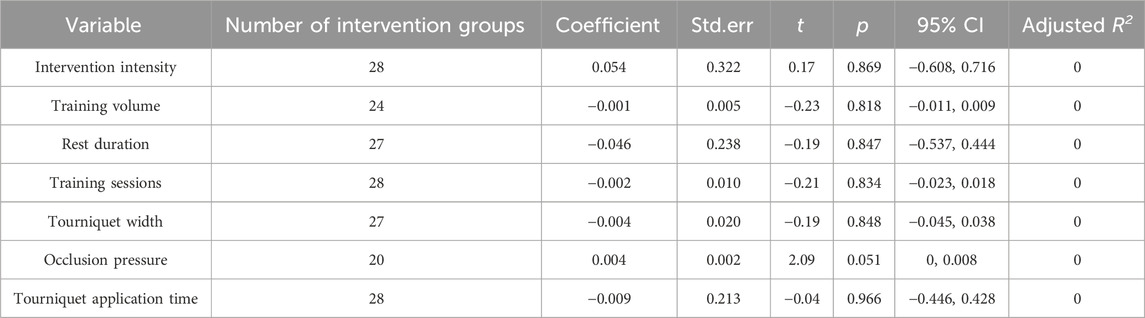
Table 5. Meta-regression of moderators concerning the effects of BFR combined with resistance training on muscle strength improvement.
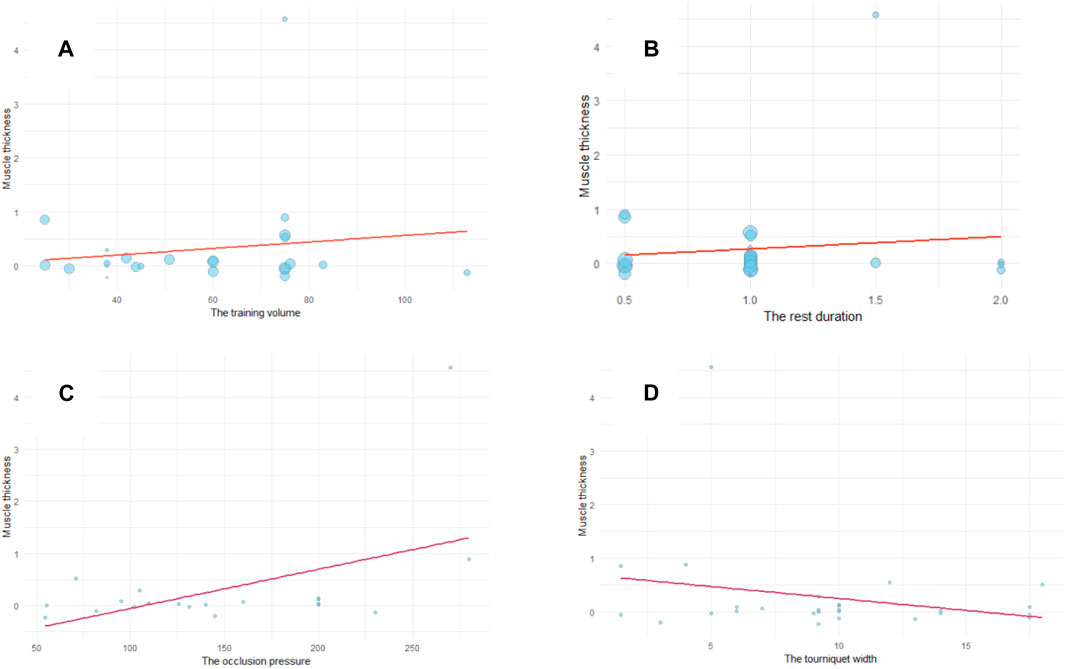
Figure 7. Meta-regression modelling of the effects of (A) training volume (B) training rest duration (C) tourniquet occlusion pressure, and (D) tourniquet width on muscle thickness improvement by BFR combined with resistance training.
4 Discussion
This meta-analysis aimed to assess the efficacy of muscle thickness and strength improvement by comparing resistance training combined with BFR to traditional resistance training. The comprehensive analysis revealed no significant difference between these two intervention methods. Notably, resistance training with BFR at other intensities (middle- and high-intensity) marginally outperformed low-intensity resistance with BFR in muscle strength and thickness improvement. However, no significant difference was noted in this regard between higher-intensity and lower-intensity resistance with BFR. These findings suggest that from a safety standpoint, higher-intensity resistance exercises with BFR might not be imperative for fragile populations. Moreover, the meta-regression results suggested a potential impact of tourniquet occlusion pressure on intervention outcomes, while trial characteristics extracted from literature review showed no significant association with muscle thickness and strength improvement. Nevertheless, these trial characteristics provide crucial reference information for establishing standards for BFR combined with resistance training in the future.
The meta-analysis results further revealed that resistance training coupled with BFR exerted a comparable influence to traditional resistance on muscle strength and thickness improvement. Although high-intensity resistance training stands out as the most efficacious method for enhancing muscle strength and thickness, its applicability is constrained by potential risks, especially for fragile populations. Several studies (Krzysztofik et al., 2019; Koc et al., 2022; Krzysztofik et al., 2022) have indicated that resistance training with BFR offers a promising alternative to traditional methods, demonstrating comparable effects. Older adults and hospitalized patients commonly grapple with muscle loss and weakness owing to a lack of exercise (Ruiz et al., 2008), often associated with a decline in type II muscle fibers (Park et al., 2022). High-intensity resistance training, although effective in improving muscle strength through neural adaptions, may pose injury risks, particularly for old adults or patients, given the acute hemodynamic response it triggers (Nascimento et al., 2022). The repeated elevation in blood pressure increases endothelial shear, thrombin, and fibrin (Hansen et al., 2022), heightening the risk of venous thrombosis (Hansen et al., 2022). In contrast, resistance training coupled with BFR stimulates muscular hypertrophy by recruiting type II muscular fibers due to the localized hypoxia environment, thus enhancing muscle strength (Pour et al., 2017). This method, nevertheless, has also been reported to elevate blood pressure and provoke certain atypical vascular reactions (Nascimento et al., 2022), such as ischemia-reperfusion injury (Cristina-Oliveira et al., 2020). However, a recent study (Nascimento et al., 2019) reported contrasting findings, suggesting that resistance training with BFR did not induce coagulation activity; instead, it demonstrated an increase in fibrinolytic activity. This suggests that this intervention may not pose an elevated risk of thrombus formation. In addition, resistance training with BFR may have potential effects on endothelial function and vascular regeneration (Zhang et al., 2022), although further evidence is needed for confirmation. Despite these considerations, resistance training with BFR has already been applied in some clinical settings for functional rehabilitation (Hughes et al., 2019; Killinger et al., 2020; Anandavadivelan et al., 2024). However, aspects such as training intensity and some trial characteristics necessitate further elucidation.
The standardization of resistance training protocols with BFR remains elusive, with factors like training intensity, duration, and tourniquets specifications during the intervention potentially influencing intervention effectiveness. In view of this, this study conducted subgroup meta-analysis and meta-regression to examine the relationships between trial characteristics and muscle hypertrophy. The subgroup analysis aimed at assessing the influence of different intensities on muscle changes. Notably, limited research has explored the effects of other intensities, especially high-intensity resistance training with BFR, on muscle changes, underscoring the necessity for further validation of its impact. Among the studies included in our analysis, only three (comprising four intervention groups) implemented moderate- (60%–80% of 1 RM) and high-intensity (over 80% of 1 RM) resistance training with BFR. Subgroup analysis was conducted to compare the effects of low-intensity (below 60% of 1 RM) resistance training with BFR against other intensities (moderate and high intensities) of resistance training with BFR, aiming to elucidate the differential effects on muscle changes. The results revealed no significant difference in muscle strength and thickness improvements between low-intensity and moderate- and high-intensity resistance training with BFR. Studies by Biazon et al. (2019); Laurentino et al. (2008) reported that high-intensity resistance training with BFR did not notably outperform high-intensity resistance training without BFR in inducing muscle hypertrophy. However, Barrett, 2017 discovered that 70% of 1 RM resistance training with BFR did not lead to greater muscle hypertrophy than moderate-to-intensity training alone during a 12-week training period. This discrepancy might be attributed to participant characteristics, as Barrett’s study involved highly trained males, potentially limiting substantial gains in muscle strength and thickness compared to untrained populations. Differing trial characteristics, such as trial volume, might also play a role in this divergence. Barrent’s study Barrett (2017) adjusted the training volume based on participants’ individual conditions, such as premature fatigue; in contrast, studies by Biazon et al. (2019); Laurentino et al. (2008) employed a fixed training protocol for each participant.
Further substantiation is required to affirm the impact of moderate- and high-intensity resistance training with BFR on muscle hypertrophy. Compared to low-intensity resistance training with BFR, high-intensity resistance training with BFR presnets heightened challenges to participants’ physical condition and exerts greater stress on the cardiovascular system, potentially posing risk during training (Hansen et al., 2022). In light of current findings, advocating for the application of low-intensity resistance training with BFR in clinical settings and for populations unable to endure high-intensity training seems prudent. Bradley et al. (2022) demonstrated that low-load BFR training (30% 1 RM) resulted in lower Rating of Perceived Exertion (RPF) scores than training at 70% 1RM. For individuals lacking training experience or unable to withstand high-intensity training, implementing low-load training with BFR may alleviate discomfort during training. Additionally, low-intensity resistance training with BFR exerts notably less strain on joints and soft tissues than high-intensity resistance training (Patterson et al., 2019). It is worth noting that 20% 1 RM is equivalent to the intensity of daily activities (Almeida et al., 2022), but studies involving training intensities below 20% 1 RM are currently lacking. Training at intensities lower than 20% 1 RM may not induce sufficient biological pressure to stimulate muscle hypertrophy. Therefore, low-intensity resistance training with BFR is recommended for daily training due to its safety and effectiveness.
The meta-regression results revealed an absence of significant impact from the training protocol characteristics on the outcomes. This observation may be attributed to the minor variations in training protocols among the studies included in our analysis. The intervention protocols in these studies were derived from modifications to traditional resistance training foundation, prescribing a training frequency of 2-3 sessions per week with a minimum duration of 4 weeks. Notably, evidence from several studies suggests that this approach can elicit muscle hypertrophy within 2 weeks (Hill et al., 2018; Teixeira et al., 2022). Six studies adhered consistently to a specific design for BFR training sets and repetitions of 30/15/15/15 (four sets). In addition to the details of the training protocols, certain characteristics of the tourniquet used during training may also wield influence over the intervention outcomes.
In addition to training intensity, our meta-regression explored the link between certain tourniquets characteristics and their effects on muscle hypertrophy. The analysis unvialed no significant association between deflating the tourniquet during rest periods and its impact on muscle hypertrophy. However, these findings were drawn from trials focusing on LI resistance training with BFR, with limited representation of other-intensity resistance training with BFR. Consequently, the existing evidence might not be sufficient to establish a definitive relationship between tourniquet inflation status and the effects of high-intensity resistance training with BFR on muscle hypertrophy. Laurentino et al. (2008), for instance, reported discomfort and early fatigue associated with inflated tourniquets during high-intensity resistance training, potentially influencing muscle hypertrophy improvements (Das and Paton, 2022). The practice of deflating tourniquets during rest periods may contribute to maintaining adequate training volume for muscle hypertrophy in certain contexts (Barrett, 2017).
The width and occlusion pressure of tourniquets have surfaced as potential influencers of intervention efficacy. Presently, there are no standardized criteria for tourniquet usage in BFR training. Among the literature included, only two companies offered blocking tourniquet products tailored for training, each with differing specifications. While tourniquet width did not exhibit a significant association with muscle changes, it potentially impacted the intervention’s effectiveness. The regression model indicated slight reduction in intervention improvement with an increase in tourniquets width. Although direct investigation into the effect of tourniquet width on intervention effectiveness was lacking, previous research suggested that wider tourniquets may exert greater occlusion pressure due to decreased pressure required to occlude blood vessels. Loenneke et al. (2012) observed that the pressure required to occlude blood flow decreases proportionally with increasing cuff width. However, it is essential to note that these results may be influenced by the cuff material in the study, as narrower tourniquets demonstrated better elasticity compared to wider ones. Thus, wider tourniquets might induce better localized hypoxia, potentially enhancing muscle growth stimulation (Michal et al., 2020). The studies encompassed in our analysis did not account for different occlusion pressures resulting from varying widths, which could potentially affect the intervention’s effectiveness. This emphasizes the need for future research to explore the synergistic effects of tourniquet width and pressure, as well as the influence of tourniquet material on intervention outcomes. Additionally, considering that a narrower cuff may lead to localized muscle damage due to increased strain (Das and Paton, 2022), it follows that a wider cuff would be more practical for application.
Regarding the pressure applied by tourniquets, the results of the meta-regression indicated that it did not exhibit a significant association with intervention outcomes. However, the regression model highlighted that increased occlusion pressure correlated with more effective muscle hypertrophy improvement. Higher restriction pressure (70%–100% LOP) may indicate a lower blood supply, leading to increased metabolic strain during exercise, potentially resulting in an enhanced muscle strength and hypertrophy (Fabero-Garrido et al., 2022b; Cognetti et al., 2022). Nevertheless, careful selection of the occlusion pressure is crucial. Most studies in our analysis utilized an arterial occlusion pressure (AOP) ranging from 40% to 80%. It has been suggested that an AOP of 50%–80% is optimal for BFR training (Das and Paton, 2022), and an AOP exceeding 80% may elevate the risk of adverse events during patient training, such as the potential induction of venous thrombosis (Stavres et al., 2018). Furthermore, higher cuff pressure may lead to discomfort, post-exercise soreness, and reduced total exercise volume. Notably, 8 studies (40%) included in our analysis did not apply individualized pressure settings to participants, potentially resulting in training-related pain (Koc et al., 2022). Hence, careful consideration regarding the width and occlusion pressure of tourniquets is vital during training interventions to minimize potential adverse effects while achieving desired muscle growth.
5 Conclusion
Low intensity resistance training with BFR is a safe and effective training program. This training method induces muscle hypertrophy in a short period of time and alleviates the process of muscle wasting by stimulating and engaging type II muscle fibre contractions. It also reduces the risk of venous thrombosis. However, it is important to note that although this training programme is similar to high-intensity resistance training, it may place a greater cardiovascular workload, which may lead to adverse events. In order to improve the improvement in muscle strength and dimensions with this intervention protocol, this study analysed the results of existing studies. Based on the results, this study suggests that the prescription of the programme could be guided by the following parameters: 2-3 training sessions per week at an intensity of 20%–40% of 1 RM. In addition, it is recommended to use a wider cuff and to apply 50%–80% of the arterial occlusion pressure during training to stimulate improvements in muscle strength and thickness. In addition, releasing tourniquet pressure during rest periods is beneficial to reduce participant discomfort during training.
While our investigation provides valuable insights, the limited number of studies examining the combination of high-intensity resistance training and BFR restricts the generalization of our findings, particularly in the context of high-intensity training. Furthermore, our findings highlight the need for further exploration into the effects of tourniquet width and pressure on intervention outcomes. Subsequently, studies should endeavor to investigate the synergistic effects of tourniquet pressure and width on intervention effects. Additionally, the characteristics of tourniquet materials, such as elasticity, and their impact on intervention outcomes remain an understudied area that warrants attention.
Data availability statement
The original contributions presented in the study are included in the article/Supplementary Material, further inquiries can be directed to the corresponding author.
Author contributions
FM: Data curation, Investigation, Methodology, Writing–original draft, Writing–review and editing. JH: Data curation, Methodology, Writing–original draft. YW: Methodology, Supervision, Writing–review and editing.
Funding
The author(s) declare that no financial support was received for the research, authorship, and/or publication of this article.
Conflict of interest
The authors declare that the research was conducted in the absence of any commercial or financial relationships that could be construed as a potential conflict of interest.
Publisher’s note
All claims expressed in this article are solely those of the authors and do not necessarily represent those of their affiliated organizations, or those of the publisher, the editors and the reviewers. Any product that may be evaluated in this article, or claim that may be made by its manufacturer, is not guaranteed or endorsed by the publisher.
Supplementary material
The Supplementary Material for this article can be found online at: https://www.frontiersin.org/articles/10.3389/fphys.2024.1379605/full#supplementary-material
References
Alizadeh Pahlavani H. (2022). Exercise therapy for people with sarcopenic obesity: myokines and adipokines as effective actors. Front. Endocrinol. (Lausanne) 13, 811751. doi:10.3389/fendo.2022.811751
Almeida B. J. G., Alvarez I. F., Pires de Campos Biazon T. M., Ugrinowitsch C., Augusto Libardi C. (2022). Effects of blood flow restriction combined with resistance training or neuromuscular electrostimulation on muscle cross-sectional area. J. Sport Rehabilitation 31, 319–324. doi:10.1123/jsr.2021-0101
Anandavadivelan P., Cardinale D., Blomhoff R., Sunde B., Lassen K., Kleive D., et al. (2024). Blood flow restriction Exercise in the perioperative setting to Prevent loss of muscle mass in patients with pancreatic, biliary tract, and liver cancer: study protocol for the PREV-Ex randomized controlled trial. Trials 25, 356. doi:10.1186/s13063-024-08207-5
Andrade C. (2020). Mean difference, standardized mean difference (SMD), and their use in meta-analysis: as simple as it gets. J. Clin. psychiatry 81, 20f13681. doi:10.4088/JCP.20f13681
Bakbergenuly I., Hoaglin D. C., Kulinskaya E. (2020). Estimation in meta-analyses of mean difference and standardized mean difference. Stat. Med. 39, 171–191. doi:10.1002/sim.8422
Barcelos L. C., Prado Nunes P. R., Marquez Ferreira de Souza L. R., de Oliveira A. A., Furlanetto R., Marocolo M., et al. (2015). Low-load resistance training promotes muscular adaptation regardless of vascular occlusion, load, or volume. Eur. J. Appl. Physiology 115, 1559–1568. doi:10.1007/s00421-015-3141-9
Barrett K. (2017). The effects of moderate intensity strength training coupled with blood flow restriction: a 12 Week intervention. Dissertation/Thesis.
Biazon TMPC, Ugrinowitsch C., Soligon S. D., Oliveira R. M., Bergamasco J. G., Borghi-Silva A., et al. (2019). The association between muscle deoxygenation and muscle hypertrophy to blood flow restricted training performed at high and low loads. Front. Physiology 10, 446. doi:10.3389/fphys.2019.00446
Bowden J., Tierney J. F., Copas A. J., Burdett S. (2011). Quantifying, displaying and accounting for heterogeneity in the meta-analysis of RCTs using standard and generalised Qstatistics. BMC Med. Res. Methodol. 11, 41. doi:10.1186/1471-2288-11-41
Bradley K. M. M., Bunn J. A. A., Feito Y., Myers B. J. (2022). Effects of blood flow restriction training on muscle size, power, and VO2max in active adults. Transl. J. Am. Coll. Sports Med. 8. doi:10.1249/tjx.0000000000000219
Brumitt J., Hutchison M. K., Kang D., Alterado S. G. D., Berg T., Nguyen B. P., et al. (2021a). Rotator cuff strength is not augmented by blood flow restriction training. Phys. Ther. Sport 52, 305–311. doi:10.1016/j.ptsp.2021.10.013
Brumitt J., Hutchison M. K., Kang D., Alterado S. G. D., Berg T., Nguyen B. P., et al. (2021b). Rotator cuff strength is not augmented by blood flow restriction training. Phys. Ther. sport official J. Assoc. Chart. Physiother. Sports Med. 52, 305–311. doi:10.1016/j.ptsp.2021.10.013
Brumitt J., Hutchison M. K., Kang D., Alterado S. G. D., Berg T., Nguyen B. P., et al. (2021c). Rotator cuff strength is not augmented by blood flow restriction training. Phys. Ther. Sport 52, 305–311. doi:10.1016/j.ptsp.2021.10.013
Centner C., Lauber B., Seynnes O. R., Jerger S., Sohnius T., Gollhofer A., et al. (2019). Low-load blood flow restriction training induces similar morphological and mechanical Achilles tendon adaptations compared with high-load resistance training. J. Appl. Physiol. (1985) 127, 1660–1667. doi:10.1152/japplphysiol.00602.2019
Cognetti D. J., Sheean A. J., Owens J. G. (2022). Blood flow restriction therapy and its use for rehabilitation and return to sport: physiology, application, and guidelines for implementation. Arthrosc. Sports Med. Rehabil. 4, e71–e76. doi:10.1016/j.asmr.2021.09.025
Colapietro M. (2023). Comparative effects of blood flow restriction training and conventional resistance exercise on the hamstrings. Dissertation/Thesis.
Colapietro M., Portnoff B., Miller S. J., Sebastianelli W., Vairo G. L. (2023). Effects of blood flow restriction training on clinical outcomes for patients with acl reconstruction: a systematic review. Sports Health 15, 260–273. doi:10.1177/19417381211070834
Cook S. B., Cleary C. J. (2019). Progression of blood flow restricted resistance training in older adults at risk of mobility limitations. Front. physiology 10, 738. doi:10.3389/fphys.2019.00738
Cristina-Oliveira M., Meireles K., Spranger M. D., O'Leary D. S., Roschel H., Peçanha T. (2020). Clinical safety of blood flow-restricted training? A comprehensive review of altered muscle metaboreflex in cardiovascular disease during ischemic exercise. Am. J. Physiol. Heart Circ. Physiol. 318, H90–h109. doi:10.1152/ajpheart.00468.2019
Cruz-Jentoft A. J., Sayer A. A. (2019). Sarcopenia. Lancet 393, 2636–2646. doi:10.1016/S0140-6736(19)31138-9
Csapo R., Alegre L. M. (2016). Effects of resistance training with moderate vs heavy loads on muscle mass and strength in the elderly: a meta-analysis. Scand. J. Med. Sci. Sports 26, 995–1006. doi:10.1111/sms.12536
Das A., Paton B. (2022). Is there a minimum effective dose for vascular occlusion during blood flow restriction training? Front. Physiol. 13, 838115. doi:10.3389/fphys.2022.838115
de Morton N. A. (2009). The PEDro scale is a valid measure of the methodological quality of clinical trials: a demographic study. Aust. J. Physiother. 55, 129–133. doi:10.1016/S0004-9514(09)70043-1
Fabero-Garrido R., Gragera-Vela M., Del Corral T., Izquierdo-García J., Plaza-Manzano G., López-de-Uralde-Villanueva I. (2022a). Effects of low-load blood flow restriction resistance training on muscle strength and hypertrophy compared with traditional resistance training in healthy adults older than 60 Years: systematic review and meta-analysis. J. Clin. Med. 11, 7389. doi:10.3390/jcm11247389
Fabero-Garrido R., Gragera-Vela M., del Corral T., Izquierdo-García J., Plaza-Manzano G., López-de-Uralde-Villanueva I. (2022b). Effects of low-load blood flow restriction resistance training on muscle strength and hypertrophy compared with traditional resistance training in healthy adults older than 60 Years: systematic review and meta-analysis. J. Clin. Med. 11, 7389. doi:10.3390/jcm11247389
Fahs C. A., Loenneke J. P., Thiebaud R. S., Rossow L. M., Kim D., Abe T., et al. (2015). Muscular adaptations to fatiguing exercise with and without blood flow restriction. Clin. Physiol. Funct. Imaging 35, 167–176. doi:10.1111/cpf.12141
Fernandes D. Z., Weber V. M. R., da Silva M. P. A., de Lima Stavinski N. G., de Oliveira L. E. C., Casoto Tracz E. H., et al. (2020). Effects of blood flow restriction training on handgrip strength and muscular volume of young women. Int. J. Sports Phys. Ther. 15, 901–909. doi:10.26603/ijspt20200901
Fragala M. S., Cadore E. L., Dorgo S., Izquierdo M., Kraemer W. J., Peterson M. D., et al. (2019). Resistance training for older adults: position statement from the national strength and conditioning association. J. Strength and Cond. Res. 33, 2019–2052. doi:10.1519/JSC.0000000000003230
Hackney K. J., Downs M. E., Ploutz-Snyder L. (2016). Blood flow restricted exercise compared to high load resistance exercise during unloading. Aerosp. Med. Hum. Perform. 87, 688–696. doi:10.3357/amhp.4566.2016
Hansen D., Abreu A., Ambrosetti M., Cornelissen V., Gevaert A., Kemps H., et al. (2022). Exercise intensity assessment and prescription in cardiovascular rehabilitation and beyond: why and how: a position statement from the Secondary Prevention and Rehabilitation Section of the European Association of Preventive Cardiology. Eur. J. Prev. Cardiol. 29, 230–245. doi:10.1093/eurjpc/zwab007
Higgins J. P., Thompson S. G., Deeks J. J., Altman D. G. (2003). Measuring inconsistency in meta-analyses. Bmj 327, 557–560. doi:10.1136/bmj.327.7414.557
Higgins J. P. T., Altman D. G., Gøtzsche P. C., Jüni P., Moher D., Oxman A. D., et al. (2011). The Cochrane Collaboration’s tool for assessing risk of bias in randomised trials. BMJ 343, d5928. doi:10.1136/bmj.d5928
Hill E. C., Housh T. J., Keller J. L., Smith C. M., Schmidt R. J., Johnson G. O. (2018). Early phase adaptations in muscle strength and hypertrophy as a result of low-intensity blood flow restriction resistance training. Eur. J. Appl. Physiol. 118, 1831–1843. doi:10.1007/s00421-018-3918-8
Hughes L., Rosenblatt B., Haddad F., Gissane C., McCarthy D., Clarke T., et al. (2019). Comparing the effectiveness of blood flow restriction and traditional heavy load resistance training in the post-surgery rehabilitation of anterior cruciate ligament reconstruction patients: a UK national health service randomised controlled trial. Sports Med. 49, 1787–1805. doi:10.1007/s40279-019-01137-2
Higgins J. P. T., Thomas J., Chandler J., Cumpston M., Li T., Page M. J., et al. (2024). Cochrane Handbook for Systematic Reviews of Interventions version 6.4 (updated August 2023). Cochrane. Available at: http://www.training.cochrane.org/handbook.
Kacin A., Strazar K. (2011). Frequent low-load ischemic resistance exercise to failure enhances muscle oxygen delivery and endurance capacity. Scand. J. Med. Sci. Sports 21, e231–e241. doi:10.1111/j.1600-0838.2010.01260.x
Khare S. P., Reddy T. O., Kumar D. A., Sisodia D. A. (2023). Muscle architecture and sports performance. J. Sports Sci. Nutr. 4, 254–257. doi:10.33545/27077012.2023.v4.i2d.224
Killinger B., Lauver J. D., Donovan L., Goetschius J. (2020). The effects of blood flow restriction on muscle activation and hypoxia in individuals with chronic ankle instability. J. Sport Rehabil. 29, 633–639. doi:10.1123/jsr.2018-0416
Koc B. B., Truyens A., Heymans M., Jansen E. J. P., Schotanus M. G. M. (2022). Effect of low-load blood flow restriction training after anterior cruciate ligament reconstruction: a systematic review. Int. J. Sports Phys. Ther. 17, 334–346. doi:10.26603/001c.33151
Krzysztofik M., Wilk M., Wojdała G., Gołaś A. (2019). Maximizing muscle hypertrophy: a systematic review of advanced resistance training techniques and methods. Int. J. Environ. Res. Public Health 16, 4897. doi:10.3390/ijerph16244897
Krzysztofik M., Zygadło D., Trybek P., Jarosz J., Zając A., Rolnick N., et al. (2022). Resistance training with blood flow restriction and ocular health: a brief review. J. Clin. Med. 11, 4881. doi:10.3390/jcm11164881
Laurentino G., Ugrinowitsch C., Aihara A. V., Fernandes A. R., Parcell A. C., Ricard M., et al. (2008). Effects of strength training and vascular occlusion. Int. J. Sports Med. 29, 664–667. doi:10.1055/s-2007-989405
Laurentino G. C., Loenneke J. P., Ugrinowitsch C., Aoki M. S., Soares A. G., Roschel H., et al. (2022). Blood-flow-restriction-training-induced hormonal response is not associated with gains in muscle size and strength. J. Hum. Kinet. 83, 235–243. doi:10.2478/hukin-2022-0095
Li X., Li J., Qing L., Wang H., Ma H., Huang P. (2023). Effect of quadriceps training at different levels of blood flow restriction on quadriceps strength and thickness in the mid-term postoperative period after anterior cruciate ligament reconstruction: a randomized controlled external pilot study. BMC Musculoskelet. Disord. 24, 360. doi:10.1186/s12891-023-06483-x
Lixandrão M. E., Ugrinowitsch C., Laurentino G., Libardi C. A., Aihara A. Y., Cardoso F. N., et al. (2015). Effects of exercise intensity and occlusion pressure after 12 weeks of resistance training with blood-flow restriction. Eur. J. Appl. physiology 115, 2471–2480. doi:10.1007/s00421-015-3253-2
Loenneke J. P., Fahs C. A., Rossow L. M., Sherk V. D., Thiebaud R. S., Abe T., et al. (2012). Effects of cuff width on arterial occlusion: implications for blood flow restricted exercise. Eur. J. Appl. Physiol. 112, 2903–2912. doi:10.1007/s00421-011-2266-8
Lorenz D. S., Bailey L., Wilk K. E., Mangine R. E., Head P., Grindstaff T. L., et al. (2021). Blood flow restriction training. J. Athl. Train. 56, 937–944. doi:10.4085/418-20
Madarame H., Neya M., Ochi E., Nakazato K., Sato Y., Ishii N. (2008). Cross-transfer effects of resistance training with blood flow restriction. Med. Sci. Sports Exerc 40, 258–263. doi:10.1249/mss.0b013e31815c6d7e
Maher C. G., Sherrington C., Herbert R. D., Moseley A. M., Elkins M. (2003). Reliability of the PEDro scale for rating quality of randomized controlled trials. Phys. Ther. 83, 713–721. doi:10.1093/ptj/83.8.713
McGuinness L. A., Higgins J. P. T. (2020). Risk-of-bias VISualization (robins): an R package and Shiny web app for visualizing risk-of-bias assessments. Res. Synthesis Methods 12, 55–61. doi:10.1002/jrsm.1411
Michal W., Michal K., Marcin B. (2020). The influence of compressive gear on maximal load lifted in competitive powerlifting. Biol. Sport 37, 437–441. doi:10.5114/biolsport.2021.100145
Moher D., Liberati A., Tetzlaff J., Altman D. G. (2009). Preferred reporting items for systematic reviews and meta-analyses: the PRISMA statement. Ann. Intern. Med. 151, 264–W64. doi:10.7326/0003-4819-151-4-200908180-00135
Nascimento D. D. C., Petriz B., Oliveira S. D. C., Vieira D. C. L., Funghetto S. S., Silva A. O., et al. (2019). Effects of blood flow restriction exercise on hemostasis: a systematic review of randomized and non-randomized trials. Int. J. Gen. Med. 12, 91–100. doi:10.2147/ijgm.S194883
Nascimento D. D. C., Rolnick N., Neto I. V. S., Severin R., Beal F. L. R. (2022). A useful blood flow restriction training risk stratification for exercise and rehabilitation. Front. Physiol. 13, 808622. doi:10.3389/fphys.2022.808622
Ozaki H., Yasuda T., Ogasawara R., Sakamaki-Sunaga M., Naito H., Abe T. (2013). Effects of high-intensity and blood flow-restricted low-intensity resistance training on carotid arterial compliance: role of blood pressure during training sessions. Eur. J. Appl. Physiol. 113, 167–174. doi:10.1007/s00421-012-2422-9
Page M. J., McKenzie J. E., Bossuyt P. M., Boutron I., Hoffmann T. C., Mulrow C. D., et al. (2021). The PRISMA 2020 statement: an updated guideline for reporting systematic reviews. BMJ 372, n71. doi:10.1136/bmj.n71
Park S. Y., Yoon K. H., Hwang S. H., Ko T. S., Lee H. S. (2022). Aging-related changes in knee flexor muscle strength and cross-sectional area. Med. Baltim. 101, e31104. doi:10.1097/md.0000000000031104
Patterson S. D., Hughes L., Warmington S., Burr J., Scott B. R., Owens J., et al. (2019). Blood flow restriction exercise: considerations of methodology, application, and safety. Front. Physiol. 10, 533. doi:10.3389/fphys.2019.00533
Pour M. B., Joukar S., Hovanloo F., Najafipour H. (2017). Long-term low-intensity endurance exercise along with blood-flow restriction improves muscle mass and neuromuscular junction compartments in old rats. Iran. J. Med. Sci. 42, 569–576.
Reece T. M., Godwin J. S., Strube M. J., Ciccone A. B., Stout K. W., Pearson J. R., et al. (1985). Myofiber hypertrophy adaptations following 6 weeks of low-load resistance training with blood flow restriction in untrained males and females. J. Appl. Physiol. 134, 1240–1255. doi:10.1152/japplphysiol.00704.2022
Ruiz J. R., Sui X., Lobelo F., Morrow J. R., Jackson A. W., Sjöström M., et al. (2008). Association between muscular strength and mortality in men: prospective cohort study. Bmj 337, a439–a439. doi:10.1136/bmj.a439
Schoenfeld B. J., Grgic J., Ogborn D., Krieger J. W. (2017). Strength and hypertrophy adaptations between low-vs. High-load resistance training: a systematic review and meta-analysis. J. Strength and Cond. Res. 31, 3508–3523. doi:10.1519/JSC.0000000000002200
Schulzke S. (2021). “Assessing and exploring heterogeneity,” in Principles and practice of systematic reviews and meta-analysis. Editor S. Patole (Cham: Springer International Publishing), 33–41.
Stavres J., Singer T. J., Brochetti A., Kilbane M. J., Brose S. W., McDaniel J. (2018). The feasibility of blood flow restriction exercise in patients with incomplete spinal cord injury. Pm R. 10, 1368–1379. doi:10.1016/j.pmrj.2018.05.013
Teixeira E. L., Painelli V. S., Schoenfeld B. J., Silva-Batista C., Longo A. R., Aihara A. Y., et al. (2022). Perceptual and neuromuscular responses adapt similarly between high-load resistance training and low-load resistance training with blood flow restriction. J. strength Cond. Res. 36, 2410–2416. doi:10.1519/JSC.0000000000003879
van der Zwaard S., Brocherie F., Jaspers R. T. (2021). Under the hood: skeletal muscle determinants of endurance performance. Front. Sports Act. Living 3, 719434. doi:10.3389/fspor.2021.719434
Vechin F. C., Libardi C. A., Conceição M. S., Damas F. R., Lixandrão M. E., Berton R. P. B., et al. (2015). Comparisons between low-intensity resistance training with blood flow restriction and high-intensity resistance training on quadriceps muscle mass and strength in elderly. J. Strength Cond. Res. 29, 1071–1076. doi:10.1519/jsc.0000000000000703
Wang H.-N., Chen Y., Cheng L., Cai Y. H., Li W., Ni G. X. (2022a). Efficacy and safety of blood flow restriction training in patients with knee osteoarthritis: a systematic review and meta-analysis. Arthritis Care and Res. 74, 89–98. doi:10.1002/acr.24787
Wang H.-N., Chen Y., Cheng L., Wang S. T., Hu D. X., Wang L. N., et al. (2022b). Effect of low-load resistance training with different degrees of blood flow restriction in patients with knee osteoarthritis: study protocol for a randomized trial. Trials 23, 6. doi:10.1186/s13063-021-05946-7
Yasuda T., Fukumura K., Fukuda T., Uchida Y., Iida H., Meguro M., et al. (2014). Muscle size and arterial stiffness after blood flow-restricted low-intensity resistance training in older adults. Scand. J. Med. and Sci. Sports 24, 799–806. doi:10.1111/sms.12087
Keywords: resistance training (RT), muscle strength, muscle thickness, blood flow restriction (BFR), young adults
Citation: Ma F, He J and Wang Y (2024) Blood flow restriction combined with resistance training on muscle strength and thickness improvement in young adults: a systematic review, meta-analysis, and meta-regression. Front. Physiol. 15:1379605. doi: 10.3389/fphys.2024.1379605
Received: 31 January 2024; Accepted: 30 July 2024;
Published: 12 August 2024.
Edited by:
Ricardo Ferraz, University of Beira Interior, PortugalReviewed by:
Paulo Sergio Chagas Gomes, Universidade do Estado do Rio de Janeiro, BrazilHugo Luca Corrêa, Catholic University of Brasilia (UCB), Brazil
Hao-Nan Wang, Sichuan University, China
Copyright © 2024 Ma, He and Wang. This is an open-access article distributed under the terms of the Creative Commons Attribution License (CC BY). The use, distribution or reproduction in other forums is permitted, provided the original author(s) and the copyright owner(s) are credited and that the original publication in this journal is cited, in accordance with accepted academic practice. No use, distribution or reproduction is permitted which does not comply with these terms.
*Correspondence: Yan Wang, d3l3ZWl3ZWlAMTI2LmNvbQ==