- 1Institute of Human Movement Science, Sport and Health, University of Graz, Graz, Austria
- 2Institute of Sport Science, University of Klagenfurt, Klagenfurt am Wörthersee, Austria
- 3Institute of Interdisciplinary Exercise Science and Sports Medicine, MSH Medical School Hamburg, Hamburg, Germany
- 4School of Human Kinetics and Recreation, Memorial University of Newfoundland, St. John’s, NL, Canada
When improving athletic performance in sports with high-speed strength demands such as soccer, basketball, or track and field, the most common training method might be resistance training and plyometrics. Since a link between strength capacity and speed strength exists and recently published literature suggested chronic stretching routines may enhance maximum strength and hypertrophy, this review was performed to explore potential benefits on athletic performance. Based on current literature, a beneficial effect of static stretching on jumping and sprinting performance was hypothesized. A systematic literature search was conducted using PubMed, Web of Science and Google scholar. In general, 14 studies revealed 29 effect sizes (ES) (20 for jumping, nine for sprinting). Subgroup analyses for jump performance were conducted for short- long- and no stretch shortening cycle trials. Qualitative evaluation was supplemented by performing a multilevel meta-analysis via R (Package: metafor). Significant positive results were documented in six out of 20 jump tests and in six out of nine sprint tests, while two studies reported negative adaptations. Quantitative data analyses indicated a positive but trivial magnitude of change on jumping performance (ES:0.16, p = 0.04), while all subgroup analyses did not support a positive effect (p = 0.09–0.44). No significant influence of static stretching on sprint performance was obtained (p = 0.08). Stretching does not seem to induce a sufficient stimulus to meaningfully enhance jumping and sprinting performance, which could possibly attributed to small weekly training volumes or lack of intensity.
1 Introduction
Due to its paramount importance in team sports as well as track and field, a vast amount of research was performed to ascertain training routines to improve jumping and sprinting performance (Ramirez-Campillo et al., 2020; Byrne et al., 2022; Patti et al., 2022; Ramirez-Campillo et al., 2022). Indeed, authors described speed strength to be game-changing in soccer (Requena et al., 2014; Keiner et al., 2022b), basketball (Brughelli et al., 2008; Delextrat and Cohen, 2008; Delextrat and Cohen, 2009), or handball (Manchado et al., 2013; Wagner et al., 2014), underlining the relevance of developing appropriate and effective training routines. In accordance with the literature, several training routines included plyometric exercises (Markovic and Newton, 2007; Morris et al., 2022), while other studies suggested maximal strength capacity to positively influence speed strength (Lesinski et al., 2016; Wirth et al., 2016).
Accordingly, a high influence of lower body maximal strength in the squat was frequently reported. With r = 0.78, Wisloff et al. (Wisløff et al., 2004) reported a significant relationship between maximum strength in half squats and vertical jump performance in soccer players (Wisløff et al., 2004) while Warneke et al. (2022c) showed maximum strength in the deep squat and deadlift to be strongly correlated with vertical and horizontal jumping performance as well as linear and change of direction sprints. Therefore, it does not seem surprising that chronic resistance training routines were sufficient to meaningfully enhance speed strength performance in athletic populations (Behm et al., 2017; Lohmann et al., 2022).
Even though resistance training can be considered safe and effective, it is necessary to supervise the training to avoid poor exercise technique and inappropriate training loading (Kraemer and Ratamess, 2004; Faigenbaum and Myer, 2010). Therefore, developing facility and time independent, safe, and effective training alternatives seem to be beneficial (Morie et al., 2010; Schwendinger and Pocecco, 2020). Interestingly, recently published articles suggest high intensity (Panidi et al., 2023) or high volume (Arntz et al., 2023) static stretching can induce muscle hypertrophy and maximal strength increases, that were similar to resistance training adaptations, if performed appropriately (Warneke et al., 2023b). These results are in accordance with earlier research, indicating potential performance increases in response to chronic stretching routines (Shrier, 2004; Medeiros and Lima, 2017). Since Behm et al. (2023) also suggested static stretching to be a possible alternative to heavy strength training programs, the question arises about the practical relevance when aiming to enhance athletic performance. No previous systematic reviews have explored the possibility to induce meaningful improvements in jumping height and sprinting speed. As a consequence, this systematic review with meta-analysis was conducted to investigate the possibility of using static stretching to enhance the listed parameters in healthy active participants. Based on the current literature showing stretch-induced strength and muscle size increases, it was hypothesized that static stretching could provide a sufficient stimulus to enhance jumping and sprinting performance.
2 Materials and methods
Adhering to the PRISMA Guidelines (Page et al., 2021) (see Supplemental Material) a systematic literature search was conducted on the PubMed, Web of Science and Scopus databases, which was supplemented by the first 500 Google Scholar hits. The study did not match the study registration criteria of the PROSPERO database (i.e., health-related outcomes) as it investigated athletic performance outcomes. Therefore, the study protocol was not registered.
2.1 Eligibility criteria
After removing duplicates remaining study titles and abstract were screened using the following eligibility criteria. Studies were excluded if they 1) did not include a passive control group or 2) incorporated older participants (>65 years), patients or injured participants 3) investigated acute effects of stretching 4) did not perform static stretching. The cut-off to discriminate younger from old adults was set under consideration of the common World Health Organization (WHO) classification. Accordingly, adhering to the PICO (participant/patient, intervention, comparison, outcome) guidelines (Eriksen and Frandsen, 2018), the search term was built considering the following inclusion criteria:
Participants: young and healthy participants.
Intervention: at least 2 weeks of static stretching interventions.
Comparison/control: controlled study design using a passive control (without receiving an intervention instruction within the study).
Outcome: any type of jumping or sprinting performance, including change of direction and linear sprint testing.
2.1 1 Literature search
The literature search was performed in March 2023 and updated in November 2024. Three authors (PF, GP and TS) independently reviewed the search results. The search was conducted using the following search term.
2.2 Study selection
After performing the independent systematic literature search, each study selected for inclusion was additionally reviewed considering the inclusion criteria by another author (KW), who extracted the data afterwards. The final excel sheets were independently double checked by the authors (GP, PF).
2.3 Risk of bias
Quality assessment was performed following the “PEDro scale” criteria (Maher et al., 2003; de Morton, 2009). The quality assessment was independently performed by two authors (PF, TS), and in the case of differing results, a third reviewer decided (KW). The risk of publication bias was assessed by visually inspecting funnel plots.
GRADE working group criteria (Atkins et al., 2004) were applied to rate the certainty of evidence following categorizations of “very low” (effect estimate very uncertain), “low” (further research very likely changing the effect estimate), “moderate” (further research likely to change the effect estimate) or “high” (further research very unlikely to change the effect estimate). In accordance to the described criteria and the inclusion of (randomized) controlled trials, the quality of evidence was initially classified as high and adjusted afterwards based on possible study limitations (risk of bias). Attributes like inconsistency, uncertainty of directness, or imprecise data, reporting bias caused a downgrading, while strong evidence of association, evidence of a dose-response gradient and plausible confounders upgraded the certainty of evidence.
2.4 Statistical procedure
Data were extracted by PF and KW and double checked by GP. If no original values (means (M) and standard deviations (SD)) were provided in the full text, the corresponding authors were contacted. If no answer was received, values were imputed from graphics, if applicable. Otherwise, the study was not further considered for further calculation. Using collected M and SD from pre- and post-tests, changes were calculated, performing M(posttest)–M(pretest). Standard deviations were pooled as
Accounting for dependency of effect size with multiple outcomes, a multilevel meta-analysis was performed to pool the standardized mean differences (SMD) and 95% confidence intervals (CI) plotting the stretching intervention versus the passive control condition. Furthermore, subgroup analyses were performed for jump performance, distinguishing between the short stretch shortening cycle (SSC) activities by including a variety of drop jumps, the long SSC with different countermovement jumps and jumps without any assumed SSC like the squat jump. Outcome heterogeneity is expressed using τ2 for inter- and intragroup heterogeneity and is plotted in the graphical ES illustration. Pooled effect sizes (ES) were interpreted as follows: 0≤ES < 0.2 trivial, 0.2≤ES < 0.5 small, 0.5≤ES < 0.8 moderate and ES ≥ 0.8 large (Faraone, 2008). All calculations were performed with R using the package metafor to account for outcome dependencies of multiple outcomes.
3 Results
The literature search resulted in 14 articles (Figure 1). The quality assessment is illustrated in Table 1, study characteristics are provided in Table 2.
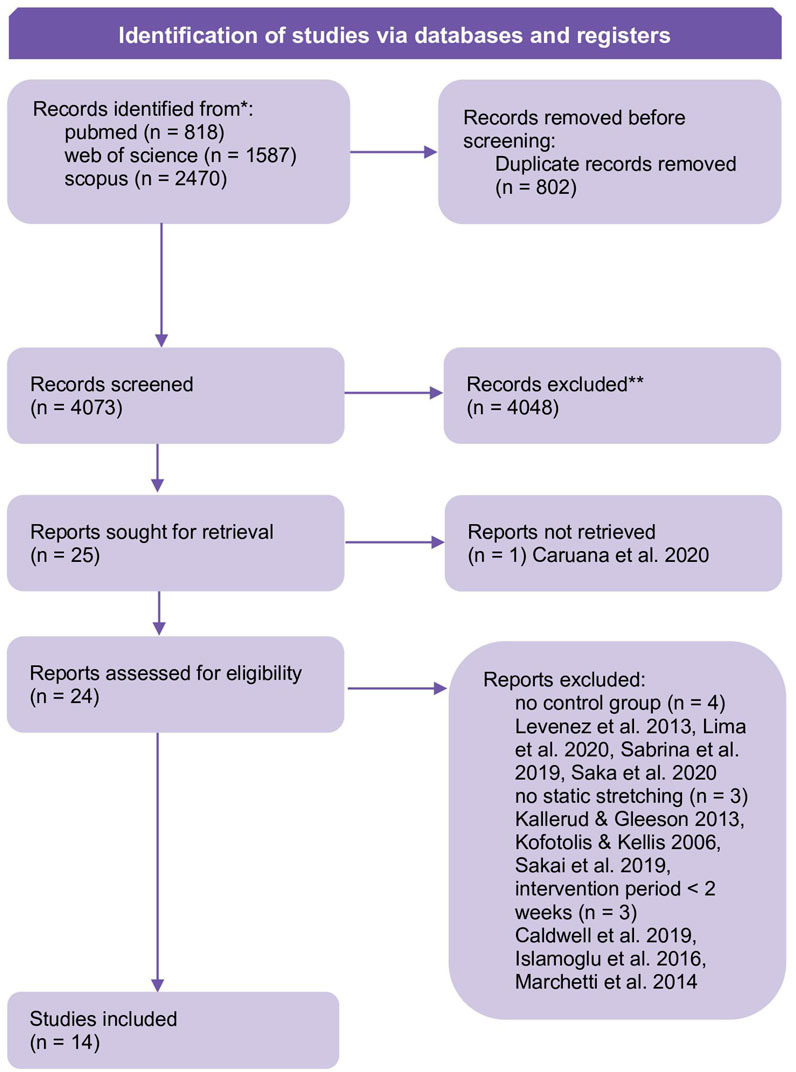
Figure 1. PRISMA flow chart adhering to Page et al. (2021) to illustrate the systematic literature search.
After excluding about 4000 articles based on title and abstract, the remaining 27 full texts were checked for eligibility. From the available 26 studies, four articles did not include a non-intervened passive control, while six others did not fulfill the minimum requirements of intervention periods or did not exclusively used static stretching (see Figure 1).
Eight studies exclusively examined jumping performance (Hunter and Marshall, 2002; Yuktasir and Kaya, 2009; Donti et al., 2021; Ikeda and Ryushi, 2021; Nakamura et al., 2021; Panidi et al., 2021; Alipasali et al., 2022; Warneke et al., 2022d), two studies (Rodriguez Fernandez et al., 2016; Alipasali et al., 2019) only sprint performance. Since four studies assessed both jumping and sprinting performance (Kokkonen et al., 2007; Bazett-Jones et al., 2008; Hadjicharalambous, 2016; Barbosa et al., 2020), the results regarding jumping performance from 12 studies and sprinting results from six studies were examined.
3.1 Qualitative study results
3.1.1 Jumping performance
The intervention period ranged between three (Barbosa et al., 2020) and 12 weeks (Panidi et al., 2021) in which stretching sessions were scheduled at least 3 days/week (Kokkonen et al., 2007; Barbosa et al., 2020; Ikeda and Ryushi, 2021; Nakamura et al., 2021; Panidi et al., 2021) to a maximum of 7 days/week (Warneke et al., 2022d). In one study, stretching was also performed twice a day (Hadjicharalambous, 2016).
Stretching duration per session ranged from 10 s (Alipasali et al., 2022) to 10 min (Warneke et al., 2022d) and included a minimum of one (Warneke et al., 2022d) and a maximum of four (Bazett-Jones et al., 2008) sets. The stretching intensity was determined via subjective pain scales and ranged from stretching without pain (Alipasali et al., 2022) to stretching at the point of discomfort (Panidi et al., 2021) or the limit of pain (Ikeda and Ryushi, 2021).
Six studies included drop jumps from five different heights, six countermovement jumps (single leg in one study (Panidi et al., 2021), vertical jump and standing jump in two cases each and rebound jump, triple hop, standing long or broad jump in one result each. For quantitative analysis, the jumping types were distinguished via the expected stretch-shortening cycle length (short and long SSC subgroup).
For jumping performance, effect sizes showed jumping performance increases ranging from 2.3% (Kokkonen et al., 2007) to 27 ± 30% (Panidi et al., 2021). Twelve (12) trivial and/or non-significant effect sizes from - 3.84% (Bazett-Jones et al., 2008) to 16.0% (Donti et al., 2021) were observed, while two effect sizes showed performance decreases from the pre-to the post-test with −3.7% (Barbosa et al., 2020) to −7.93% (Ikeda and Ryushi, 2021).
3.1.2 Sprint performance
Six studies were found investigating the effects of chronic stretching programs on sprint performance. A total of 257 subjects were included performing nine different sprint variants between 4.5 m (Alipasali et al., 2019) and 55 m (Bazett-Jones et al., 2008). Intervention durations ranged between 3 weeks (Barbosa et al., 2020) to 10 weeks (Kokkonen et al., 2007) stretching up to 6 days per week (Rodriguez Fernandez et al., 2016) with Hadjicharalambous (2016) stretching twice a day. Stretching durations varied between 10 s (Alipasali et al., 2019) and 45 s (Bazett-Jones et al., 2008). Exercises were performed repeatedly in two (Rodriguez Fernandez et al., 2016; Alipasali et al., 2019) to four (Bazett-Jones et al., 2008) sets, with rests ranging in duration from 10 s (Alipasali et al., 2019) up to 1 minute (Kokkonen et al., 2007; Bazett-Jones et al., 2008). The intensity of stretching was again determined by using individual pain perception, mostly reporting mild discomfort.
Six out of nine sprinting tests showed performance improvements of 1.3% (Kokkonen et al., 2007)–10% (Hadjicharalambous, 2016), while three tests did not reach the level of significance with 0.04% (Barbosa et al., 2020) to 2.2% (Hadjicharalambous, 2016) pre-to post-test changes.
3.2 Summary of the results
An overview for the results is illustrated in Table 3, providing an illustrated overview of the results considering the collected parameters and the described effects.
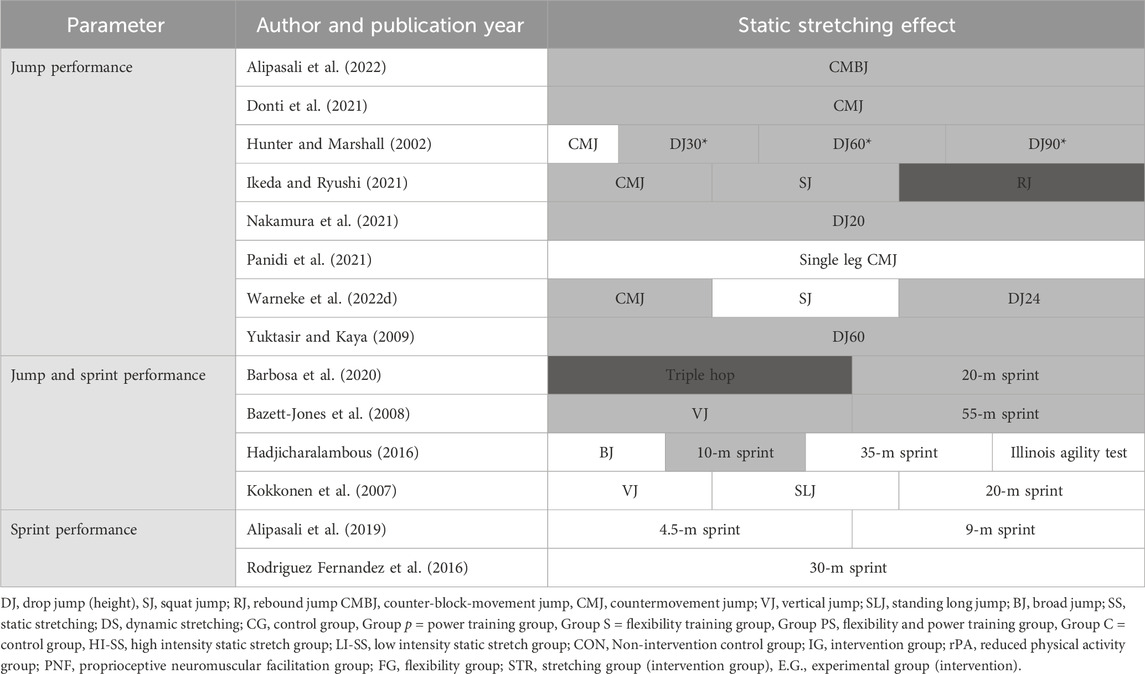
Table 3. Overview of direction of the effects, bright grey illustrates no significant change from pre-to post-test, no color (white) shows a significant improvement of the motor task while fields marked in dark grey showed stretching negatively impacted jumping or sprinting performance.
3.3 Quantitative analysis of the extracted effects
Quantitative analysis of the effects is illustrated in Figure 2, showing the forest plot for jumping performance (no, short, and long stretch-shortening cycle) (a) and sprinting performance (b).
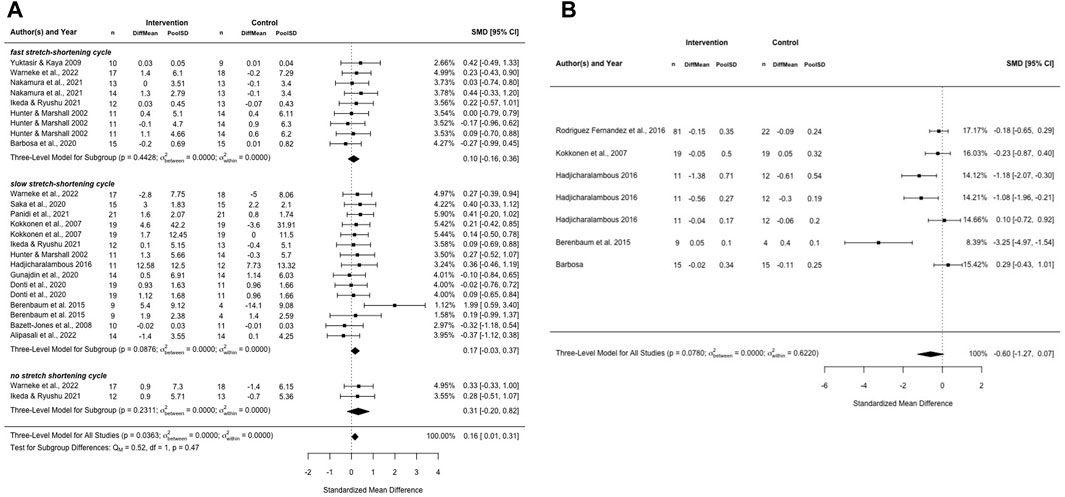
Figure 2. Forest plots for (A) chronic stretching on jumping performance, divided into fast stretch-shortening cycle, slow stretch-shortening cycle and no stretch-shortening cycle jumping actions and (B) chronic stretching on sprinting performance.
Including 25 effects with p = 0.036 there was an overall trivial magnitude effect with ES = 0.16 (0.01–0.31) with a high certainty of evidence. The individual subgroup analyses failed to reach significance for jump performance using the fast SSC (ES = 0.10, p = 0.449), slow SSC (ES = 0.17, p = 0.09) and no SSC (ES = 0.31, p = 0.23). Due to limited number of extracted effect sizes, no further subgroup analysis was performed. With ES = −0.60, p = 0.078, no significant sprinting improvements can be assumed.
Visual funnel plot inspection suggests a publication bias for sprinting performance, while in the jumping performance no asymmetry was observable (Figure 3). With p = 0.25 for jumping and p = 0.002 for sprinting, the Eggers test confirms these results.
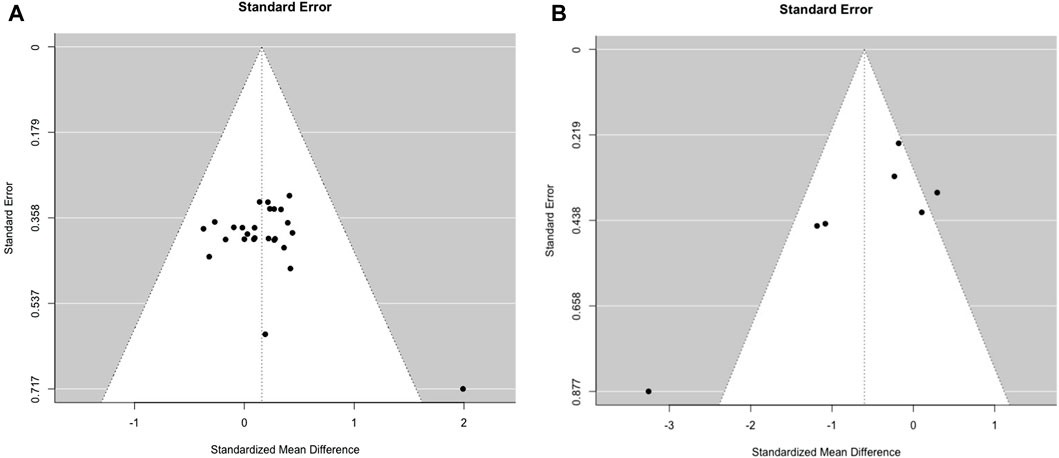
Figure 3. Funnel plots for visual inspection of a potential publication bias for (A) chronic stretching on jumping performance and (B) chronic stretching on sprinting performance.
4 Discussion
Since recent reviews pointed out chronic stretching programs were sufficient to induce maximal strength increases (Arntz et al., 2023) or muscle hypertrophy (Panidi et al., 2023), this review was conducted to investigate potential positive influence on jump and sprint performance. Fourteen out of 20 studies did not support previously suggested stretch-induced performance enhancements (Shrier, 2004; Medeiros and Lima, 2017), which was confirmed by an overall trivial ES of 0.16 (p = 0.04). Similarly, stretching showed no significant benefit for sprinting performance (p = 0.078), even though 6 out of 9 included studies indicated a positive influence.
From a physiological point of view, all jumps and sprinting need to apply high forces within a short time period requiring maximal activation via maximal fiber recruitment, frequency, and synchronization (Fleck and Kraemer, 2004; Zatsiorsky et al., 2020). Nevertheless, to account for neuromuscular differences and specific movement execution attributable to, among others, differences in the SSC, subgroup analyses were performed to reduce methodological heterogeneity and to increase comparability of the results. Nevertheless, due to a very limited number of studies to pool effects, training methods heterogeneity remains a limitation of the results. For instance, Donti et al. (2021) and Panidi et al. (2021) included adolescent females, while most other studies explored stretching effects in adults, using untrained (Kokkonen et al., 2007) to highly trained participants (Hadjicharalambous, 2016; Rodriguez Fernandez et al., 2016). Assuming every training program to be effective in untrained populations, the consistent positive direction of the effects for all included tests included by Kokkonen et al. (2007) seems not surprising. Nevertheless, even though some studies showed significant strength increases (Kokkonen et al., 2007; Warneke et al., 2022d), overall, static stretching seems not to induce a sufficient stimulus to enhance jumping and sprinting performance.
4.1 The role of maximum strength
According to Keiner et al. (2022a) “strength plays a key role in jumping and sprinting performance, as athletes need to exert great forces within short time intervals during push-off and landing.” Since recent literature showed chronic stretching routines to be sufficient to enhance maximal strength (Arntz et al., 2023) comparable with resistance training programs (Warneke et al., 2023a; Warneke et al., 2023b), a positive influence of athletic performance was reasonably hypothesized. In some of the included studies, maximum strength increases were detected as well. Kokkonen et al. (2007) showed knee extensor and knee flexor strength increases of 32.4% and 15.3%, respectively, while Warneke et al. (2022d) reported plantar flexors strength enhancements of about 10%. Even though strength increases in individual muscle groups have been previously observed, several factors seem to remain unaffected by applying mechanical tension to the muscle via stretching.
4.2 Training specificity
Jump and sprint are coordinated power activities involving both strength (force) and velocity. According to the concept of training specificity (Behm and Sale, 1993), training parameters should mimic task or activity characteristics (e.g., velocity, contraction types, movement patterns, joint angles). Whereas static stretching involves elongating soft tissues into a fixed length that is maintained for a prolonged period (i.e., 10–30 s), jumping and sprinting are high velocity stretch-shortening cycle(s) activities of relatively short durations (<1 s per jump or stride) with transition or amortization periods typically less than 250 m (Komi, 1984; Aura and Komi, 1986). Furthermore, jumping and sprinting performance must be considered a much more complex and multi-factorial construct. Assuming a significant correlation and a causal relationship of plantar flexors maximum strength and jumping performance of r = 0.52–0.54 (Warneke et al., 2022b), this correlation accounts for r2 = 25–27% of the variance. Whereas static stretching is primarily applied to individual muscle groups in a fixed position (Donti et al., 2021; Nakamura et al., 2021; Panidi et al., 2021; Warneke et al., 2022d), athletic movements consist of a well-coordinated interaction of several muscle groups including specific neuromuscular requirements. Hence, static stretching versus jumping and sprinting would elicit very different neuromuscular responses. Jumping and sprinting for maximal performance would involve high motor unit recruitment especially of the type II fast twitch motor units, which would be fired at high firing frequencies, with some degree of synchronization, while coordinating the activation of agonists, antagonists, synergists and stabilizer muscles (Behm, 1995). Although, Budini and Christova. (2023) proposed motor evoked potentials to be greater while stretching, which might attributable to muscle spindle induced corticomotor facilitation, Magnusson et al. (1996) reported no changes in hamstrings EMG activity during a 3 week stretch training protocol. Even with stretch-related increases in sympathetic nerve activity (Cui et al., 2006; Inami et al., 2014), these stretch-induced adaptations seem to be insufficient to affect jump and sprint performance. Hence, since static stretching does not mimic the specific active joint movements of jumping and sprinting, nor evoke maximal muscle activation, trivial to no significant stretching effects on speed strength performance might be attributable to insufficient neuromuscular activation patterns.
4.3 Comparison to resistance training routines
Accordingly, performing active multi-joint strength training exercises seems to activate the muscles in a more appropriate way. Referring to high load squat training to beneficially impact sprinting (Lohmann et al., 2022) and jumping performance (Keiner et al., 2022a; Keiner et al., 2022b), using high training intensities in resistance training might require maximal motor unit discharge frequency and synchronization, which is required to explosively accelerate the body (jumping, sprinting). Unsurprisingly, the squat and deadlift showed correlations up to r = 0.91 on vertical jumping height and r = −0.88 on sprinting performance, explaining about 80% of variance (Wisløff et al., 2004; Warneke et al., 2022c). When compared to the effects of resistance training on jumping and sprinting, stretch training effects fared poorly in comparison with trivial and non-significant effects respectively. Accordingly, current meta-analyses demonstrate strength training and plyometrics to be of superior effectiveness with ES = 0.6–0.8 for power training and 0.88–1.35 for strength training (Behm et al., 2017).
In specific training facilities and circumstances, stretching might have some practical applications as an alternative to resistance training (Behm et al., 2023). However, when aiming to improve athletic performance a more potent stimulus seems necessary, which was not apparent with the stretch training investigated in the currently available literature. Assuming a dose-response relationship for stretching volume to enhance maximal strength, it is possible that higher stretching volumes, frequencies (Arntz et al., 2023) or intensities (Panidi et al., 2021) would induce significant adaptations. Accordingly, the comparatively small effect sizes could also explain the lack of significance in the subgroup analyses for jumping performance. While the overall result reached statistical significance, the trivial magnitude effects might be influenced by the inclusion of further studies building a larger participant database.
4.4 The potential role of flexibility in speed strength performance
Stretching is well-known for increasing flexibility/range of motion in humans (Young et al., 2013; Medeiros and Martini, 2018). Apart from decreased muscle and/or tendon stiffness (Morse et al., 2008; Takeuchi et al., 2023) and changes in the pain/stretch threshold (Freitas and Mil-Homens, 2015; Freitas et al., 2018), animal stretch training studies demonstrate a significant increase in the number of sarcomeres in series, leading to a greater muscle length (Zöllner et al., 2012; Warneke et al., 2022a). On the one hand, Wirth (Wirth, 2011) described a possible positive influence of sarcomere in series accumulation on speed strength performance (i.e., jumping and sprinting performance) due to an optimized contraction property (Herring et al., 1984) induced by mechanical tension. Accordingly, Kruse et al. (2021) pointed out that enhancements in muscle lengths could improve the muscular energy production capacity with potential implications for power production This suggests that long-term adaptations of stretching might be used to advantage in training routines to increase muscle performance.
On the other hand, negative effects could be expected as recent literature pointed out a reduction in muscle-tendon stiffness (Takeuchi et al., 2023). One important mechanism leading to increased power in the SSC is the elastic kinetic energy storage in the parallel-elastic structures of the muscle tissue and series-elastic components of the tendons, pre-activation of the muscle and reflex properties of the muscle stretch reflex (Komi, 2003). Therefore, Kalkhoven & Watsford (2018) suggest a positive influence of higher stiffness in sub-elite footballers on sport specific performance, including maximal sprinting speed and (drop-) jump performance. Furthermore, Fukashiro et al. (2002) described higher stiffness in black (African ancestry) athletes compared to white (European ancestry) athletes and discussed the contribution of muscle stiffness on ground-contact time and the performance output in SSC determined performance parameters. Assuming reduced muscle stiffness in response to long-term stretching programs (Nakamura et al., 2021; Takeuchi et al., 2023) a negative effect on sport-specific performance including the SSC could be hypothesized. However, Kallerud et al. (2013) could not find any negative influences of long-term static stretching on SSC in their systematic review.
5 Limitations
Meta-analytical approaches in sport and exercise science can be controversial since invalid comparisons are sometimes performed, which can skew the conclusions. Nevertheless, pooling study effects increases the sample size and therefore enhances the statistical power. To provide a well-balanced perspective, an extended qualitative description of the study results was provided to account for heterogeneity in methods. The meta-analytical approaches might be seen as a supplementation to quantify results. Although some short duration stretching interventions sufficiently increased maximal strength (Nelson et al., 2012; Mizuno 2019), consistent stretch-induced strength and muscle size increases were reported after longer stretching durations (≥10 min per session) (Chen et al., 2011; Wohlann et al., 2023; Wohlann et al., 2024, Warneke et al., 2022a, Reiner et al., 2023; Warneke et al., 2022). However, stretching durations of the included studies primarily used short stretching durations of a few minutes. Consequently, especially when considering maximal strength was only one component in the multifactorial construct of jumping and sprinting performance, the included stretching studies did not involve stretching volumes that allow a reasonable assumption of increased athletic performance. However, since there were increases in the Warneke et al. (2022b) study using a weekly volume of 70 min of stretching, future research on stretch-induced jumping and sprinting performance enhancements should include larger stretching dosages. Furthermore, pooled linear sprint performance effects originate from different sprinting distances, starting with 4.5 m (acceleration phase) until 55 m (maximum velocity phase). Therefore, different physiological and biomechanical requirements are addressed, thus, the calculated effect size might be considered with care. However, it seems of limited value to divide these studies in further subgroups, as the number of pooled effects was small. In general, the current stretching study situation calls for further research.
6 Practical applications and conclusion
To date, no beneficial effects of chronic stretching on jumping and sprinting performance could be detected. Due to the limited number of studies and extracted effect sizes with questionable methodological homogeneity, further research with extended intervention periods and longer stretching durations with high intensities seems necessary, since those provided the highest magnitude maximum strength increases.
Data availability statement
The original contributions presented in the study are included in the article/supplementary material, further inquiries can be directed to the corresponding author.
Author contributions
KW: Conceptualization, Formal Analysis, Investigation, Methodology, Software, Visualization, Writing–original draft. PF: Data curation, Methodology, Writing – original draft, Data curation, Methodology, Writing–original draft. GP: Methodology, Resources, Writing–review and editing. AK: Methodology, Supervision, Writing–review and editing. DB: Methodology, Supervision, Writing–review and editing, Methodology, Supervision, Writing–review and editing. TS: Data curation, Methodology, Project administration, Supervision, Writing–review and editing.
Funding
The author(s) declare that financial support was received for the research, authorship, and/or publication of this article. The authors acknowledge the financial support by the University of Graz.
Acknowledgments
This research was funded in whole, or in part, by the Austrian Science Fund (FWF) (grant DOI 10.55776/J4484). For the purpose of open access, the author has applied a CC BY public copyright licence to any Author Accepted Manuscript version arising from this submission.
Conflict of interest
The authors declare that the research was conducted in the absence of any commercial or financial relationships that could be construed as a potential conflict of interest.
The author(s) declared that they were an editorial board member of Frontiers, at the time of submission. This had no impact on the peer review process and the final decision.
Publisher’s note
All claims expressed in this article are solely those of the authors and do not necessarily represent those of their affiliated organizations, or those of the publisher, the editors and the reviewers. Any product that may be evaluated in this article, or claim that may be made by its manufacturer, is not guaranteed or endorsed by the publisher.
References
Alipasali F., Papadopoulou S. D., Gissis I., Komsis G., Komsis S., Kyranoudis A., et al. (2019). The effect of static and dynamic stretching exercises on sprint ability of recreational male volleyball players. Int. J. Environ. Res. Public Health 16, 2835. doi:10.3390/ijerph16162835
Alipasali F., Papadopoulou S. D., Kyranoudis A. E., Gisis I. (2022). The effect of static and dynamic stretching exercises on the jumping ability of recreational male volleyball players. J. Phys. Edu Sport 22, 1026–1032. doi:10.7752/jpes.2022.04130
Arntz F., Markov A., Behm D. G., Behrens M., Negra Y., Nakamura M., et al. (2023). Chronic effects of static stretching exercises on muscle strength and power in healthy individuals across the lifespan: a systematic review with multi-level meta-analysis. Sports Med. 53, 723–745. doi:10.1007/s40279-022-01806-9
Atkins D., Best D., Briss P. A., Eccles M., Falck-Ytter Y., Flottorp S., et al. (2004). Grading quality of evidence and strength of recommendations. BMJ 328, 1490. doi:10.1136/bmj.328.7454.1490
Aura O., Komi P. V. (1986). The mechanical efficiency of locomotion in men and women with special emphasis on stretch-shortening cycle exercises. Eur. J. Appl. Physiol. Occup. Physiol. 55, 37–43. doi:10.1007/BF00422890
Barbosa G. M., Trajano G. S., Dantas G. A. F., Silva B. R., Vieira W. H. B. (2020). Chronic effects of static and dynamic stretching on hamstrings eccentric strength and functional performance: a randomized controlled trial. J. Strength Cond. Res. 34, 2031–2039. doi:10.1519/JSC.0000000000003080
Bazett-Jones D. M., Gibson M. H., McBride J. M. (2008). Sprint and vertical jump performances are not affected by six weeks of static hamstring stretching. J. Strength Cond. Res. 22, 25–31. doi:10.1519/JSC.0b013e31815f99a4
Behm D. G. (1995). Neuromuscular implications and applications of resistance training. J. Strength Cond. Res. 9, 264–274. doi:10.1519/1533-4287(1995)009<0264:niaaor>2.3.co;2
Behm D. G., Granacher U., Warneke K., Aragão-Santos J. C., Da Silva-Grigoletto M. E., Konrad A. (2023). Minimalist training: is lower dosage or intensity resistance training effective to improve physical fitness? A narrative review. Sports Med. 54, 289–302. doi:10.1007/s40279-023-01949-3
Behm D. G., Sale D. G. (1993). Velocity specificity of resistance training. Sports Med. 15, 374–388. doi:10.2165/00007256-199315060-00003
Behm D. G., Young J. D., Whitten J. H. D., Reid J. C., Quigley P. J., Low J., et al. (2017). Effectiveness of traditional strength vs. Power training on muscle strength, power and speed with youth: a systematic review and meta-analysis Front. Physiol. 8, 423. doi:10.3389/fphys.2017.00423
Brughelli M., Cronin J., Levin G., Chaouachi A. (2008). Understanding change of direction ability in sport: a review of resistance training studies. Sports Med. 38, 1045–1063. doi:10.2165/00007256-200838120-00007
Budini F., Christova M. (2023). Enhanced corticospinal excitability in the tibialis anterior during static stretching of the soleus in young healthy individuals. PLoS One 18, e0284289. doi:10.1371/journal.pone.0284289
Byrne P. J., Moody J. A., Cooper S.-M., Farrell E., Kinsella S. (2022). Short-term effects of “composite” training on strength, jump, and sprint performance in hurling players. J. Strength Cond. Res. 36, 2253–2261. doi:10.1519/JSC.0000000000003820
Chen C. H., Nosaka K., Chen H. L., Lin M. J., Tseng K. W., Chen T. C. (2019). Effects of flexibility training on eccentric exercise-induced muscle damage. Med. Sci. Sports. Exerc. 43 (3), 491–500. doi:10.1249/MSS.0b013e3181f315ad
Cui J., Blaha C., Moradkhan R., Gray K. S., Sinoway L. I. (2006). Muscle sympathetic nerve activity responses to dynamic passive muscle stretch in humans. J. Physiol. 576, 625–634. doi:10.1113/jphysiol.2006.116640
Delextrat A., Cohen D. (2008). Physiological testing of basketball players: toward a standard evaluation of anaerobic fitness. J. Strength Cond. Res. 22, 1066–1072. doi:10.1519/JSC.0b013e3181739d9b
Delextrat A., Cohen D. (2009). Strength, power, speed, and agility of women basketball players according to playing position. J. Strength Cond. Res. 23, 1974–1981. doi:10.1519/JSC.0b013e3181b86a7e
de Morton N. A. (2009). The PEDro scale is a valid measure of the methodological quality of clinical trials: a demographic study. Aust. J. Physiother. 55, 129–133. doi:10.1016/s0004-9514(09)70043-1
Donti O., Papia K., Toubekis A., Donti A., Sands W. A., Bogdanis G. C. (2021). Acute and long-term effects of two different static stretching training protocols on range of motion and vertical jump in preadolescent athletes. Biol. Sport 38, 579–586. doi:10.5114/biolsport.2021.101127
Eriksen M. B., Frandsen T. F. (2018). The impact of patient, intervention, comparison, outcome (PICO) as a search strategy tool on literature search quality: a systematic review. J. Med. Libr. Assoc. 106, 420–431. doi:10.5195/jmla.2018.345
Faigenbaum A. D., Myer G. D. (2010). Resistance training among young athletes: safety, efficacy and injury prevention effects. Br. J. Sports Med. 44, 56–63. doi:10.1136/bjsm.2009.068098
Faraone S. V. (2008). Interpreting estimates of treatment effects: implications for managed care. P T 33, 700–711.
Fleck S. J., Kraemer W. J. (2004). Designing resistance training programs. 3rd Edn. Champaign, IL: Human Kinetics.
Freitas S. R., Mendes B., Le Sant G., Andrade R. J., Nordez A., Milanovic Z. (2018). Can chronic stretching change the muscle-tendon mechanical properties? A Review. Scand. J. Med. Sci. Sports 28, 794–806. doi:10.1111/sms.12957
Freitas S. R., Mil-Homens P. (2015). Effect of 8-week high-intensity stretching training on biceps femoris architecture. J. Strength Cond. Res. 29, 1737–1740. doi:10.1519/JSC.0000000000000800
Fukashiro S., Abe T., Shibayama A., Brechue W. F. (2002). Comparison of viscoelastic characteristics in triceps surae between Black and White athletes. Acta Physiol. Scand. 175, 183–187. doi:10.1046/j.1365-201X.2002.00985.x
Hadjicharalambous M. (2016). The effects of regular supplementary flexibility training on physical fitness performance of young high-level soccer players. J. Sports Med. Phys. Fit. 56, 699–708.
Herring S. W., Grimm A. F., Grimm B. R. (1984). Regulation of sarcomere number in skeletal muscle: a comparison of hypotheses. Muscle Nerve 7, 161–173. doi:10.1002/mus.880070213
Hunter J. P., Marshall R. N. (2002). Effects of power and flexibility training on vertical jump technique. Med. Sci. Sports Exerc 34, 478–486. doi:10.1097/00005768-200203000-00015
Ikeda N., Ryushi T. (2021). Effects of 6-week static stretching of knee extensors on flexibility, muscle strength, jump performance, and muscle endurance. J. Strength Cond. Res. 35, 715–723. doi:10.1519/JSC.0000000000002819
Inami T., Shimizu T., Baba R., Nakagaki A. (2014). Acute changes in autonomic nerve activity during passive static stretching. Am. J. Sports Sci. Med. 2, 166–170. doi:10.12691/ajssm-2-4-9
Kalkhoven J. T., Watsford M. L. (2018). The relationship between mechanical stiffness and athletic performance markers in sub-elite footballers. J. Sports Sci. 36, 1022–1029. doi:10.1080/02640414.2017.1349921
Kallerud H., Gleeson N., Kallerud H., Gleeson Á. N., Gleeson N. (2013). Effects of stretching on performances involving stretch-shortening cycles. Sports Med. 43, 733–750. doi:10.1007/s40279-013-0053-x
Keiner M., Brauner T., Kadlubowski B., Sander A., Wirth K. (2022a). The influence of maximum squatting strength on jump and sprint performance: a cross-sectional analysis of 492 youth soccer players. Int. J. Environ. Res. Public Health 19, 5835. doi:10.3390/ijerph19105835
Keiner M., Kadlubowski B., Sander A., Hartmann H., Wirth K. (2022b). Effects of 10 months of speed, functional, and traditional strength training on strength, linear sprint, change of direction, and jump performance in trained adolescent soccer players. J. Strength Cond. Res. 36, 2236–2246. doi:10.1519/JSC.0000000000003807
Kokkonen J., Nelson A. G., Eldredge C., Winchester J. B. (2007). Chronic static stretching improves exercise performance. Med. Sci. Sports Exerc. 39, 1825–1831. doi:10.1249/mss.0b013e3181238a2b
Komi P. V. (1984). Physiological and biomechanical correlates of muscle function: effects of muscle structure and stretch-shortening cycle on force and speed. Exerc Sport Sci. Rev. 12, 81–122. doi:10.1249/00003677-198401000-00006
Kraemer W. J., Ratamess N. A. (2004). Fundamentals of resistance training: progression and exercise prescription. Med. Sci. Sports Exerc 36, 674–688. doi:10.1249/01.MSS.0000121945.36635.61
Kruse A., Rivares C., Weide G., Tilp M., Jaspers R. T. (2021). Stimuli for adaptations in muscle length and the length range of active force exertion—a narrative review. Front. Physiol. 12, 742034. doi:10.3389/fphys.2021.742034
Lesinski M., Prieske O., Granacher U. (2016). Effects and dose-response relationships of resistance training on physical performance in youth athletes: a systematic review and meta-analysis. Br. J. Sports Med. 50, 781–795. doi:10.1136/bjsports-2015-095497
Lohmann L. H., Warneke K., Schiemann S., Faber I. R. (2022). High-load squat training improves sprinting performance in junior elite-level soccer players: a critically appraised topic. Int. J. Athl. Ther. Train. 27, 276–281. doi:10.1123/ijatt.2021-0066
Magnusson S. P., Simonsen E. B., Aagaard P., Sørensen H., Kjaer M. (1996). A mechanism for altered flexibility in human skeletal muscle. J. Physiol. 497, 291–298. doi:10.1113/jphysiol.1996.sp021768
Maher C. G., Sherrington C., Herbert R. D., Moseley A. M., Elkins M. (2003). Reliability of the PEDro scale for rating quality of randomized controlled trials. Phys. Ther. 83, 713–721. doi:10.1093/ptj/83.8.713
Manchado C., Tortosa-Martínez J., Vila H., Ferragut C., Platen P. (2013). Performance factors in women's team handball: physical and physiological aspects--a review. J. Strength Cond. Res. 27, 1708–1719. doi:10.1519/JSC.0b013e3182891535
Markovic G., Newton R. U. (2007). Does plyometric training improve vertical jump height? A meta-analytical review * Commentary. Br. J. Sports Med. 41, 349–355. doi:10.1136/bjsm.2007.035113
Medeiros D. M., Lima C. S. (2017). Influence of chronic stretching on muscle performance: systematic review. Hum. Mov. Sci. 54, 220–229. doi:10.1016/j.humov.2017.05.006
Medeiros D. M., Martini T. F. (2018). Chronic effect of different types of stretching on ankle dorsiflexion range of motion: systematic review and meta-analysis. Foot(Edinb) 34, 28–35. doi:10.1016/j.foot.2017.09.006
Mizuno T. (2019). Combined effects of static stretching and electrical stimulation on joint range of motion and muscle strength. J. Strength. Cond. Res. 33 (10), 2694–2703. doi:10.1519/JSC.0000000000002260
Morie M., Reid K. F., Miciek R., Lajevardi N., Choong K., Krasnoff J. B., et al. (2010). Habitual physical activity levels are associated with performance in measures of physical function and mobility in older men. J. Am. Geriatr. Soc. 58, 1727–1733. doi:10.1111/j.1532-5415.2010.03012.x
Morris S. J., Oliver J. L., Pedley J. S., Haff G. G., Lloyd R. S. (2022). Comparison of weightlifting, traditional resistance training and plyometrics on strength, power and speed: a systematic review with meta-analysis. Sports Med. 52, 1533–1554. doi:10.1007/s40279-021-01627-2
Morse C. I., Degens H., Seynnes O. R., Maganaris C. N., Jones D. A. (2008). The acute effect of stretching on the passive stiffness of the human gastrocnemius muscle tendon unit. J. Physiology 586, 97–106. doi:10.1113/jphysiol.2007.140434
Nelson A. G., Kokkonen J., Winchester J. B., Kalani W., Peterson K., Kenly M. S., et al. (2012). A 10-week stretching program increases strength in the contralateral muscle. J. Strength. Cond. Res. 26 (3), 832–836. doi:10.1519/JSC.0b013e3182281b41
Nakamura M., Yoshida R., Sato S., Yahata K., Murakami Y., Kasahara K., et al. (2021). Comparison between high- and low-intensity static stretching training program on active and passive properties of plantar flexors. Front. Physiol. 12, 796497. doi:10.3389/fphys.2021.796497
Page M. J., McKenzie J. E., Bossuyt P. M., Boutron I., Hoffmann T. C., Mulrow C. D., et al. (2021). The PRISMA 2020 statement: an updated guideline for reporting systematic reviews. BMJ n71, n71. doi:10.1136/bmj.n71
Panidi I., Bogdanis G. C., Terzis G., Donti A., Konrad A., Gaspari V., et al. (2021). Muscle architectural and functional adaptations following 12-weeks of stretching in adolescent female athletes. Front. Physiol. 12, 701338. doi:10.3389/fphys.2021.701338
Panidi I., Donti O., Konrad A., Petros C. D., Terzis G., Mouratidis A., et al. (2023). Muscle architecture adaptations to static stretching training: a systematic review with meta-analysis. Sports Med. Open 9, 47. doi:10.1186/s40798-023-00591-7
Patti A., Giustino V., Hirose N., Messina G., Cataldi S., Grigoli G., et al. (2022). Effects of an experimental short-time high-intensity warm-up on explosive muscle strength performance in soccer players: a pilot study. Front. Physiol. 13, 984305. doi:10.3389/fphys.2022.984305
Ramirez-Campillo R., Andrade D. C., Nikolaidis P. T., Moran J., Clemente F. M., Chaabene H., et al. (2020). Effects of plyometric jump training on vertical jump height of volleyball players: a systematic review with meta-analysis of randomized-controlled trial. J. Sports Sci. Med. 19, 489–499.
Ramirez-Campillo R., García-Hermoso A., Moran J., Chaabene H., Negra Y., Scanlan A. T. (2022). The effects of plyometric jump training on physical fitness attributes in basketball players: a meta-analysis. J. Sport Health Sci. 11, 656–670. doi:10.1016/j.jshs.2020.12.005
Reiner M., Gabriel A., Sommer D., Bernsteiner D., Tilp M., Konrad A. (2023). Effects of a high-volume 7-week pectoralis muscle stretching training on muscle function and muscle stiffness. Sports. Med. Open. 9 (1), 40. doi:10.1186/s40798-023-00582-8
Requena B., Garcia I., Rquena F., Bressel E., Saez-Saez de Villarreal E., Cronin J. (2014). Association between traditional standing vertical jumps and a soccer-specific vertical jump. Eur. J. Sport Sci. 14, 398–405. doi:10.1080/17461391.2012.708790
Rodriguez Fernandez A., Sanchez J., Rodriguez Marroyo J. A., Villa J. G. (2016). Effects of seven weeks of static hamstring stretching on flexibility and sprint performance in young soccer players according to their playing position. J. Sports Med. Phys. Fit. 56, 345–351.
Schwendinger F., Pocecco E. (2020). Counteracting physical inactivity during the COVID-19 pandemic: evidence-based recommendations for home-based exercise. Int. J. Environ. Res. Public Health 17, 3909–3916. doi:10.3390/ijerph17113909
Shrier I. (2004). Does stretching improve performance? A systematic and critical review of the literature. Clin. J. Sport Med. 14, 267–273. doi:10.1097/00042752-200409000-00004
Takeuchi K., Nakamura M., Konrad A., Mizuno T. (2023). Long-term static stretching can decrease muscle stiffness: a systematic review and meta-analysis. Scand. J. Med. Sci. Sports 33, 1294–1306. doi:10.1111/sms.14402
Wagner H., Finkenzeller T., Würth S., von Duvillard S. P. (2014). Individual and team performance in team-handball: a review. J. Sports Sci. Med. 13, 808–816.
Warneke K., Freund P. A., Schiemann S. (2022a). Long-Lasting stretching induces muscle hypertrophy: a meta-analysis of animal studies. J. Sci. Sport Exerc 5, 289–301. doi:10.1007/s42978-022-00191-z
Warneke K., Keiner M., Lohmann L. H., Hillebrecht M., Wirth K., Schiemann S. (2022b). The influence of maximum strength performance in seated calf raises on counter movement jump and squat jump in elite junior basketball players. Sport Mont. 20, 63–68. doi:10.26773/smj.220610
Warneke K., Keiner M., Schiemann S., Lohmann L. H., Wirth K. (2022c). Influence of maximal strength performance in front squat and deadlift on linear sprint and jump performance in male youth elite basketball players. Ger. J. Sport Res. 53, 10–18. doi:10.1007/s12662-022-00863-6
Warneke K., Konrad A., Keiner M., Zech A., Nakamura M., Hillebrecht M., et al. (2022d). Using daily stretching to counteract performance decreases as a result of reduced physical activity—a controlled trial. Int. J. Environ. Res. Public Health 19, 15571. doi:10.3390/ijerph192315571
Warneke K., Lohmann L. H., Lima C. D., Hollander K., Konrad A., Zech A., et al. (2023a). Physiology of stretch-mediated hypertrophy and strength increases: a narrative review. Sports Med. 53, 2055–2075. doi:10.1007/s40279-023-01898-x
Warneke K., Wirth K., Keiner M., Lohmann L. H., Hillebrecht M., Brinkmann A., et al. (2023b). Comparison of the effects of long-lasting static stretching and hypertrophy training on maximal strength, muscle thickness and flexibility in the plantar flexors. Eur. J. Appl. Physiol. 123, 1773–1787. doi:10.1007/s00421-023-05184-6
Wirth K. (2011). Exzentrisches Krafttraining: auswirkungen auf unterschiedliche Maximal-und Schnellkraftparameter.
Wirth K., Hartmann H., Sander A., Mickel C., Szilvas E., Keiner M. (2016). The impact of back squat and leg-press exercises on maximal strength and speed-strength parameters. J. Strength Cond. Res. 30, 1205–1212. doi:10.1519/JSC.0000000000001228
Wisløff U., Castagna C., Helgerud J., Jones R., Hoff J. (2004). Strong correlation of maximal squat strength with sprint performance and vertical jump height in elite soccer players. Br. J. Sports Med. 38, 285–288. doi:10.1136/bjsm.2002.002071
Wohlann T., Warneke K., Hillebrecht M., Petersmann A., Ferrauti A., Schiemann S. (2023). Effects of daily static stretch training over 6 weeks on maximal strength, muscle thickness, contraction properties, and flexibility. Front. Sports. Act. Living. 5, 1139065. doi:10.3389/fspor.2023.1139065
Wohlann T., Warneke K., Kalder V., Behm D. G., Schmidt T., Schiemann S. (2024). Influence of 8-week of supervised static stretching or resistance training of pectoral major muscles on maximal strength, muscle thickness and range of motion. Eur. J. Appl. Physiol. 19. doi:10.1007/s00421-023-05413-y
Young R., Nix S., Wholohan A., Bradhurst R., Reed L. (2013). Interventions for increasing ankle joint dorsiflexion: a systematic review and meta-analysis. J. Foot Ankle Res. 6, 46–10. doi:10.1186/1757-1146-6-46
Yuktasir B., Kaya F. (2009). Investigation into the long-term effects of static and PNF stretching exercises on range of motion and jump performance. J. Bodyw. Mov. Ther. 13, 11–21. doi:10.1016/j.jbmt.2007.10.001
Zatsiorsky V. M., Kraemer J., Fry A. C. (2020). Science and practice of strength training. Hum. Kinet.
Keywords: long-term, athletic performance, stretch-shortening cycle, plyometrics, speed strength, stretch training, static stretching
Citation: Warneke K, Freundorfer P, Plöschberger G, Behm DG, Konrad A and Schmidt T (2024) Effects of chronic static stretching interventions on jumping and sprinting performance–a systematic review with multilevel meta-analysis. Front. Physiol. 15:1372689. doi: 10.3389/fphys.2024.1372689
Received: 18 January 2024; Accepted: 13 March 2024;
Published: 26 March 2024.
Edited by:
Marko Dragisa Stojanovic, University of Novi Sad, SerbiaReviewed by:
Valerio Giustino, University of Palermo, ItalyLuca Petrigna, University of Catania, Italy
Copyright © 2024 Warneke, Freundorfer, Plöschberger, Behm, Konrad and Schmidt. This is an open-access article distributed under the terms of the Creative Commons Attribution License (CC BY). The use, distribution or reproduction in other forums is permitted, provided the original author(s) and the copyright owner(s) are credited and that the original publication in this journal is cited, in accordance with accepted academic practice. No use, distribution or reproduction is permitted which does not comply with these terms.
*Correspondence: Andreas Konrad, QW5kcmVhcy5rb25yYWRAdW5pLWdyYXouYXQ=
†ORCID: Konstantin Warneke, orcid.org/0000-0003-4964-2867; Patrik Freundorfer, orcid.org/0000-0003-4964-2867; David G. Behm, orcid.org/0000-0002-9406-6056; Andreas Konrad, orcid.org/0000-0002-5588-1824; Tobias Schmidt, orcid.org/0000-0002-0172-6001