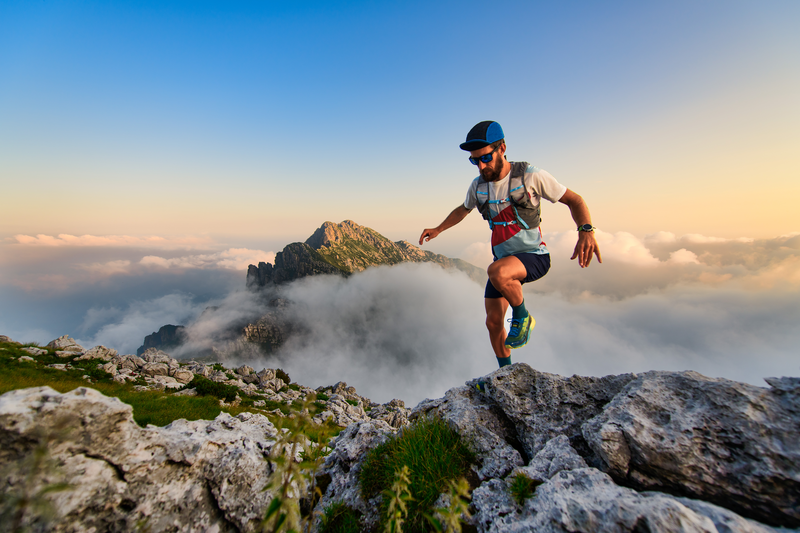
94% of researchers rate our articles as excellent or good
Learn more about the work of our research integrity team to safeguard the quality of each article we publish.
Find out more
ORIGINAL RESEARCH article
Front. Physiol. , 22 April 2024
Sec. Exercise Physiology
Volume 15 - 2024 | https://doi.org/10.3389/fphys.2024.1372020
Background: The use of elastomeric technology in sports garments is increasing in popularity; however, its specific impact on physiological and psychological variables is not fully understood. Thus, we aimed to analyze the physiological (muscle activation of the pectoralis major, triceps brachii, anterior deltoid, and rectus abdominis, capillary blood lactate, systolic and diastolic blood pressure, and heart rate) and psychological (global and respiratory rating of perceived exertion [RPE]) responses during an incremental treadmill test wearing a new sports garment for the upper body that incorporates elastomeric technology or a placebo garment.
Methods: Eighteen physically active young adults participated in two randomized sessions, one wearing the elastomeric garment and the other wearing a placebo. Participants performed in both sessions the same treadmill incremental test (i.e., starting at 8 km/h, an increase of 2 km/h each stage, stage duration of 3 min, and inclination of 1%; the test ended after completing the 18 km/h Stage or participant volitional exhaustion). The dependent variables were assessed before, during, and/or after the test. Nonparametric tests evaluated differences.
Results: The elastomeric garment led to a greater muscle activation (p < 0.05) in the pectoralis major at 16 km/h (+33.35%, p = 0.01, d = 0.47) and 18 km/h (+32.09%, p = 0.02, d = 0.55) and in the triceps brachii at 10 km/h (+20.28%, p = 0.01, d = 0.41) and 12 km/h (+34.95%, p = 0.04, d = 0.28). Additionally, lower lactate was observed at the end of the test (−7.81%, p = 0.01, d = 0.68) and after 5 min of recovery (−13.71%, p < 0.001, d = 1.00) with the elastomeric garment. Nonsignificant differences between the garments were encountered in the time to exhaustion, cardiovascular responses, or ratings of perceived exertion.
Conclusion: These findings suggest that elastomeric garments enhance physiological responses (muscle activation and blood lactate) during an incremental treadmill test without impairing physical performance or effort perception.
The literature shows that endurance exercise confers health and performance benefits such as improving or maintaining cardiovascular fitness and body composition (Gibala and Jones, 2013; Milanović et al., 2015; Wewege et al., 2017). Achieving an optimal training stimulus is key to obtaining and maximizing these benefits of exercise (Weston et al., 2014; Milanović et al., 2015). Recently, new sports tools (e.g., compression garments, gravity vests, and weight vests) have appeared to enhance training stimuli, and scientists have examined their potential positive effects on physically active individuals and professional athletes (Cipriani et al., 2014; Marriner et al., 2017; Feser et al., 2021). For instance, employing a compressive garment during exercise likely improves performance due to possible physiological effects (Marqués-Jiménez et al., 2018; Williams et al., 2021). Additionally, elastic devices have emerged as plausible materials to increase physical capacities because they are transportable, affordable, and have proven positive results (Gene-Morales et al., 2020; Babiloni-Lopez et al., 2022; Gene-Morales et al., 2022; Hammami et al., 2022; Saez-Berlanga et al., 2022). These devices have been commonly employed in strength training, and depending on how they are applied, they can both assist and resist movements. Through the use of elastics to resist movement, the participants achieved more muscle activation (Argus et al., 2011; Upton, 2011). Furthermore, the use of elastics as assistance also makes it possible to mobilize a load at a higher velocity (Tran et al., 2012). Taking this into consideration, the question arises as to whether new state-of-the-art tools can optimize the training stimulus. To our knowledge, there are still no scientific studies that examined the effects of performing an incremental treadmill test with a sports garment that incorporates elastomeric technology on parameters of the internal and external load.
In this regard, it is important to know how the use of an elastomeric garment can influence different aspects of physical performance and physiological and psychological responses. First, time to exhaustion is a key parameter of running performance in an incremental treadmill test (Scheer et al., 2018). Second, it is necessary to monitor muscle activity including surface electromyography (EMG), which records the electrical activity of the peripheral nervous system during muscle contractions (Hermens et al., 2000). This measure depends on the neuronal signals sent from motor neurons to muscles (Farina and Enoka, 2023). In this regard, afferent and efferent signals from the nervous system during skeletal muscle contraction are key to understanding exercise adaptations (Alix-Fages et al., 2022). Likewise, the metabolic and cardiovascular components of performance can alternatively be examined using metabolites such as blood lactate concentration and hemodynamic responses (e.g., blood pressure and heart rate) (Beneke et al., 2011; Gripp et al., 2021). Lactate concentration analysis is a valid option for quantifying training intensity, with blood lactate levels increasing in response to high-intensity training (Cerezuela-Espejo et al., 2018). Finally, it is crucial to determine whether the use of the elastomeric garment influences psychological variables (e.g., rating of perceived exertion [RPE]). Prior studies have informed that the RPE is correlated with various exercise-related factors such as heart rate and blood lactate (Scherr et al., 2013). In light of this, numerous subjective scales have been validated to assess and confirm the intensity of different types of exercise with various training devices, including elastic bands (Colado et al., 2012; Colado et al., 2014; Colado et al., 2018; Colado et al., 2020; Colado et al., 2023).
Therefore, this study aimed to evaluate: i) running performance (time to exhaustion); ii) physiological (muscle activation, capillary blood lactate concentration, blood pressure, and heart rate); and iii) psychological (global and respiratory rating of perceived exertion) responses during an incremental treadmill test performed wearing or not a new sports garment for the upper body that incorporates elastomeric technology. Considering that depending on whether the elastomers are shortened or stretched, the garment can offer extra resistance or assistance (Saez-Berlanga et al., 2024), we hypothesized that the use of this garment would optimize muscle activation levels in all of the muscles tested, without modifications in capillary blood lactate concentration, hemodynamic responses, perceived exertion rating, and time to exhaustion.
The sample size was determined using G*Power 3.1 software (Faul et al., 2007) based on previous studies (Gene-Morales et al., 2023). An a priori analysis was conducted to minimize the probability of type II error and calculate the minimum number of participants needed to reject the null hypothesis at the p < 0.05 level of confidence (Beck, 2013). The calculation showed that 18 volunteers were required to achieve a power of 0.80, a significance level of 0.05, and an effect size f(V) of 0.83.
The participants involved in the project were thoroughly briefed on the possible risks and discomfort associated with it, and they willingly signed a written consent form before the commencement of the study. The study protocol was approved by the Human Research Ethics Committee of the University of Valencia (H20190325095509 and H1460994903890) and was conducted in compliance with the principles outlined in the Declaration of Helsinki.
The study consisted of young adults aged 18–30 years with experience in training (at least three sessions of physical activity per week). These participants were required to be free of any history of osteoarticular or cardiovascular disease and of any cognitive, clinical, or neuromotor contraindications that would prevent them from performing the physical test. A total of 18 physically active men were selected through convenience sampling and willingly participated in the study. The participants were instructed to refrain from consuming any food, stimulants (e.g., energy drinks or coffee), or other ergogenic substances 3 hours before the sessions and to avoid engaging in intense physical activity or exercise for the lower limbs 24 h before the study. They were also encouraged to maintain good quality sleep the night before data collection.
A within-participants randomized crossover study design was used to examine the effects of treadmill exercise testing using a novel upper-body sports garment that incorporates elastomeric technology. Each participant was involved in three sessions 48 h apart, one of which was a familiarization and initial assessment, two of which were experimental sessions. The overall study design is illustrated in Figure 1. All sessions were conducted between 9:00 a.m. and 1:00 p.m. to avoid diurnal fluctuations in the performance or the other dependent variables. Each participant completed both experimental sessions within the same hour. The study was conducted over 8 weeks at the Physical Activity and Health Laboratory of the University of Valencia (Spain). All measurements were performed by the same researchers at the same sports laboratory. The minimum researchers-to-participant ratio was 4:1.
The familiarization session was used to (i) characterize the participants through anthropometric measurements and interviews; (ii) use the two sports garments (i.e., placebo and elastomeric garment); (iii) show and explain the operation of the treadmill as well as the incremental testing protocol; and (iv) instruct participants on how to report the overall body and respiratory rating of perceived exertion (RPE) at the end of each stage of the treadmill exercise test.
After a pre-exercise interview, anthropometric measurements, body weight, and fat percentage were obtained using a bioimpedance analyzer (Tanita BF-350, Tanita Corp., Tokyo, Japan). The height was determined to the nearest 0.5 cm during maximum inhalation using a wall stadiometer (Seca T214, Seca Ltd., Hamburg, Germany).
The participants were familiarized with the instruments used throughout the study, including a treadmill (ExciteLiveRun; Technogym, Cesena, Italy). To assess global and respiratory RPE, a modified Borg Scale 0–10 (Borg, 1982) was used, which was visible to the participants during the exercise. Finally, the participants were shown the two garments to use during the study: a sports garment with elastomeric technology (Pro-advance, Menatechpro System®, Madrid, Spain), and a placebo garment that appeared identical but did not include elastomers. Menatechpro System® elastomeric technology is a patented, advanced sportswear that generates elastic resistance in most planes of motion through the elongation of elastomers included in the garment. Specifically, the garment features front and back elastomers around the chest, which connect to the shoulders and extend down to the hands through each arm with two lines of elastomers. Additionally, due to this specific disposition of the elastomers, this garment could also assist in certain parts of the range of motion. Therefore, the garment’s ability to offer resistance, and/or assistance for diverse movements is determined by the way its elastomers are arranged. Furthermore, the elastomeric garment due to its characteristics can provide compression to the upper body. The Pro-advance model, which was employed in the current research, offers a maximum resistance of 8 kg at its maximum elongation, making it suitable for users who have prior training experience and aim to enhance their exercise intensity. Twelve similar garments (i.e., six placebo garments and six garments with elastomeric technology) were utilized for the study for a better adaptation of the garment to the anthropometric characteristics of each participant.
Subsequently, the participants were instructed to perform the standardized warm-up, which consisted of dynamic stretching, bodyweight strength exercises (e.g., lunges and squats), and a treadmill run of 5 min (starting at 7 km/h and increased by 1 km/h each minute) (Scheer et al., 2018). Participants were provided with a detailed explanation of the modified Borg scale (0–10) (Borg, 1982), where 10 was considered “maximum effort” and 0 “no effort.” Afterward, we explained the development of the test following the protocol of the study by Scheer et al. Scheer et al. (2018): starting at 8 km/h, an increase of 2 km/h each stage, stage duration of 3 min, and inclination of 1%. The test ended after the participant completed the 18 km/h Stage or at volitional exhaustion. A standardized pause of 30 just at the end of the second stage was implemented for capillary blood lactate measurement. The participants were instructed to self-manage the running technique according to their characteristics and experience. In addition, the participants received constant verbal and visual feedback to maintain proper body position during the test.
The study comprised two experimental sessions, one of which was conducted entirely with the elastomeric garment, while the other was conducted with a placebo garment. The order of the sessions was randomly assigned to each participant in the first session using a randomizer (https://random.org/lists). Both sessions consisted of performing the incremental treadmill exercise test as previously explained. Upon arrival at the laboratory in both experimental sessions, participants rested in a seated position for 10 min while listening to self-selected music to induce similar resting homeostatic conditions between the sessions.
In the first experimental session, participants performed the standardized warm-up, and after a 5-min rest, the participants executed the incremental treadmill exercise test. The neuromuscular activation of each muscle group was recorded during the first minute of each stage. The EMG measures started in the 10 km/h Stage. The capillary blood lactate concentration was evaluated at the end of the second stage to gauge intra-effort levels. At the end of each stage, the participants verbally mentioned the RPE as they ran. Immediately after the treadmill exercise test, blood lactate concentration and cardiovascular variables such as heart rate and systolic and diastolic blood pressure were measured. These measurements were conducted with the participant seated in an adjoining space separated from the exercise area by a partition to conceal the procedures. Intra-test analysis was performed with the participants standing with their feet placed on the borders of the treadmill. The same procedure was followed in the second experimental session.
We recorded the time to exhaustion with a stopwatch. The timing was stopped when the participant’s hands touched the treadmill frame, or a verbal request to stop the test was made.
The EMG signal was obtained using two synchronized two-channel handheld devices (Realtime Technologies Ltd., Dublin, Ireland) with a 16-bit analog-to-digital (A/D) conversion. EMG data were monitored using the validated (Hermens et al., 2000) mDurance software for Android (mDurance Solutions S.L., Granada, Spain). To ensure consistency in the standardized electrode placement, we followed the Surface Electromyography for the Non-Invasive Assessment of Muscles criteria (SENIAM) (Hermens et al., 2000) and previous studies in this field (Calatayud et al., 2014; Calatayud et al., 2017). Each participant was required to shave and clean the designated area using a cotton swab moistened with alcohol. Surface electrodes were placed on the anterior deltoid, clavicular fibers of the pectoralis major, upper rectus abdominis, and long head of the triceps brachii, according to previous studies (Calatayud et al., 2014). Chlorinated silver pre-gelled bipolar surface electrodes (Kendall™ Medi-Trace, Coividien, Barcelona, Spain) were placed at an inter-electrode distance of 10 mm. The reference electrode was positioned over the nearest bone prominence (in our study, the acromion and the superior iliac spine). To ensure consistent placement and reliable data, a mark was made on the skin of the participants around each electrode, using a permanent marker. One device recorded EMG data from the anterior deltoid and clavicular bundles of the pectoralis major muscle, while the other collected data from the upper rectus abdominis and long head of the triceps brachii muscles. The sampling rate was set at 1,024 Hz.
EMG signals were recorded and stored on a hard disk for subsequent analysis. The mDurance software used a fourth-order “Butterworth” bandpass filter to automatically filter the raw signals between 20 and 450 Hz. A high-pass cut-off frequency of 20 Hz was applied to minimize any potential “artifacts” that may occur during movement and affect the total power recorded by the EMG. Before the tests, the participants performed dynamic gestures typical of running to ensure proper signal saturation. From the second stage of the test (10 km/h), during the first minute of each stage, the average EMG signals (measured by the root-mean-square [RMS] value in microvolts) were collected for analysis. In the case that any of the participants did not complete integrally the first minute of the last stage, the EMG signal was collected for the time spent running during that last stage.
Blood lactate concentrations were measured from capillary blood obtained from the fingertips. Blood samples were collected (i) before the test, (ii) at the end of the second stage of the test (intra-test), (iii) immediately after the treadmill exercise test, and (iv) 5 min after the end of the test. The samples were analyzed using a portable lactate analyzer (Lactate Pro 2, Arkray Inc., Kyoto, Japan).
Heart rate and systolic and diastolic blood pressure were assessed before and after the test using a digital wrist blood pressure monitor (RS4-model; Omron Electronics Iberia SAU, Valencia, Spain).
Overall body and respiratory RPE were measured using a modified Borg Scale 0–10 (Borg, 1982). The scale was visible to participants throughout the sessions. To rate the effort participants had to answer at the end of each stage: “How hard does it feel now to run?” “How hard does it feel now to breathe?” “How intense is your overall sensation of breathing?” or “How intense is your sensation of unsatisfied inspiration?” (Aliverti et al., 2011; Lewthwaite and Jensen, 2021).
Statistical analyses were performed using the commercial software IBM SPSS (version 28.0; IBM Corp., Armonk, New York, United States). The results were reported as the mean ± standard deviation. A 95% confidence level (significance p ≤ 0.05) was considered statistically significant. The Shapiro–Wilk test was used to ascertain a normal distribution of the variables. All variables showed a non-normal distribution except time to exhaustion.
Therefore, differences between conditions (elastomeric garment or placebo) in time to exhaustion were assessed using a t-test. Additionally, Friedman’s test was used to assess the effects of time (measurements pre-, intra-, and post-test) in both conditions. The effect size for the Friedman test was assessed using Kendall’s coefficient of concordance (W) and interpreted according to Cohen’s guidelines (Cohen, 2009). Afterward, Wilcoxon’s comparisons were performed to assess differences between each time point and between conditions (elastomeric garment and placebo) at each time point. Effect sizes were calculated using Cohen’s d as previously described (Cohen, 2009). Cohen’s d was interpreted as a trivial effect (<0.20), small effect (0.20–0.50), moderate effect (0.50–0.80), and large effect (>0.80) (Cohen, 2009).
Eighteen healthy and trained participants were included in the study. None of the participants were excluded from this study. Descriptive data of the participants in this study were: 24.3 ± 4.4 years; height: 179.1 ± 4.1 cm; body mass: 76.4 ± 8.5 kg; body fat: 13.7% ± 4.6%; weekly training frequency: 3.6 ± 1.1 days/week.
The mean time to exhaustion in seconds for the incremental treadmill exercise test performed with the elastomeric garment and placebo garment was 1015.00 ± 95.56 and 1011.66 ± 93.06 s, respectively. The t-test revealed no significant differences between using the elastomeric garment or the placebo (p = 0.854).
Descriptive and inferential analyses of the neuromuscular activation outcomes included in the study are presented in Table 1.
Table 1. Neuromuscular activation outcomes to incremental treadmill test performed wearing the elastomeric garment or the placebo.
The factor time showed a significant effect on all the neuromuscular activation parameters both wearing the elastomeric garment (RMSpec, χ2 = 34.47, p < 0.001, W = 0.73; RMSdelt, χ2 = 41.67, p < 0.001, W = 0.87; RMStri, χ2 = 25.27, p < 0.001, W = 0.53; RMSabd, χ2 = 26.20, p < 0.001, W = 0.55) and placebo garment (RMSpec, χ2 = 39.80, p < 0.001, W = 0.83; RMSdelt, χ2 = 35.40, p < 0.001, W = 0.74; RMStri, χ2 = 32.47, p < 0.001, W = 0.68; RMSabd, χ2 = 30.93, p < 0.001, W = 0.64).
Wearing the elastomeric garment to perform the treadmill exercise test entailed greater RMSpec during the last two stages of the test (16 km/h, p = 0.01, d = 0.47; 18 km/h, p = 0.02, d = 0.55) and RMStri during the first two stages of the test (10 km/h, p = 0.01, d = 0.41; 12 km/h, p = 0.04, d = 0.28), compared to the same test performed with the placebo garment. Non-significant differences between using the elastomeric garment or the placebo were found in RMSdelt (all p ≥ 0.19, d ≤ 0.33) and RMSabd (all p ≥ 0.09, d ≤ 0.43).
A significant effect of time was found in the capillary blood lactate concentration (elastomeric garment, χ2 = 52.87, p < 0.001, W = 0.98; placebo garment, χ2 = 48.79, p < 0.001, W = 0.90).
As displayed in Figure 2; Table 2, wearing the elastomeric garment entailed significantly lower capillary blood lactate at the end of the test (p = 0.01, d = 0.68) and 5 min after the end of the test (p < 0.001, d = 1.00) compared to the placebo. Non-significant differences between garments were found during the test (p = 0.23, d = 0.36).
Figure 2. Variation of the capillary blood lactate through the incremental treadmill test performed wearing the elastomeric garment or the placebo. * Denotes significant difference from conditions (p<0.05).
Table 2. Capillary blood lactate and cardiovascular outcomes to incremental treadmill test performed wearing the elastomeric garment or placebo.
We observed a significant effect of time on the heart rate (elastomeric garment, χ2 = 18.00, p < 0.001, W = 1.00; placebo garment, χ2 = 18.00, p < 0.001, W = 1.00).
No differences between conditions (elastomeric garment, placebo) were found in the heart rate (p = 0.67, d = 0.10) (Table 2).
The factor time showed a significant effect on the blood pressure (elastomeric garment: systolic blood pressure, χ2 = 14.22, p < 0.001, W = 0.79; diastolic blood pressure, χ2 = 10.89, p < 0.001, W = 0.61; placebo garment: systolic blood pressure, χ2 = 4.76, p = 0.02, W = 0.27; diastolic blood pressure, χ2 = 8.00, p = 0.005, W = 0.44).
We encountered non-significant differences in the diastolic (p = 0.58, d = 0.17) and systolic blood pressure (p = 0.48, d = 0.15) between using the elastomeric garment or the placebo (Table 2).
A significant effect of time was found in the global RPE (elastomeric garment, χ2 = 64.51, p < 0.001, W = 0.99; placebo garment, χ2 = 64.67, p < 0.001, W = 0.99) and respiratory RPE (elastomeric garment, χ2 = 64.36, p < 0.001, W = 0.99; placebo garment, χ2 = 64.60, p < 0.001, W = 0.99).
Non-significant differences were encountered in the global RPE (all p ≥ 0.06, d ≤ 0.49) and respiratory RPE (all p ≥ 0.09, d ≤ 0.41) between using the elastomeric garment or the placebo (Table 3).
Table 3. Psychological responses to incremental treadmill test performed wearing elastomeric garment or placebo.
This is the first study to compare the effects of a new sports garment that incorporates elastomeric technology and a placebo garment on physiological and psychological responses in healthy trained adults during an incremental treadmill test. The main finding was that performing the incremental treadmill test with the elastomeric garment did not interfere with the performance (time to exhaustion) and significantly improved neuromuscular activation of the pectoralis major and triceps, and the capillary blood lactate clearance compared to the placebo garment. Considering that no previous study analyzed the effect of running wearing an elastomeric garment that covers the torso and arms, it was necessary to discuss our findings with other studies that analyzed the effect of any other type of external load during human locomotion (e.g., walking poles, compressive garments, hand weights).
First, the use of the elastomeric garment did not interfere with the performance (time to exhaustion) during an incremental treadmill test. Since there were no significant differences in the time to exhaustion, it is suggested that the elastomeric garment does not compromise physical performance during progressive running. This is of great importance since the objective of adding a garment is to improve performance and optimize positive physiological responses during training.
Regarding muscle activation, the elastomeric garment allowed participants to achieve greater muscle activation in the long head of the triceps during the first two stages (10 km/h, p = 0.01, d = 0.41; 12 km/h, p = 0.04, d = 0.28) and in the clavicular bundles of the pectoralis major during the last two stages (16 km/h, p = 0.01, d = 0.47; 18 km/h, p = 0.02, d = 0.55) compared to the placebo garment. These results for the triceps brachii are consistent with numerous studies (Knight and Caldwell, 2000; Sugiyama et al., 2013; Pellegrini et al., 2015) that examined differences in muscle activation and physiological responses between conventional treadmill and Nordic walking with an external upper body load (e.g., walking poles). These authors reported 16-fold (Pellegrini et al., 2015) and 3-fold increases (Knight and Caldwell, 2000; Sugiyama et al., 2013) in triceps brachii muscle activation during Nordic walking compared to standard walking. On the other hand, Hamner et al. (Hamner et al., 2010) examined the kinematics of running (constant speed of 3.96 m/s [≈14 km/h]) in a single healthy subject by analyzing 41 anatomical points (including the triceps brachii). They stated that the arms did not substantially contribute to either propulsion or support during running, with a maximum contribution of less than 1% of the maximum horizontal and vertical center-of-mass accelerations (Hamner et al., 2010). While the study by Hamner et al. (Hamner et al., 2010) provided a first approximation to the muscular contribution of the upper extremities in the propulsion and support phases during running, the results may not represent a general running strategy because they only analyzed one participant at a constant speed of 3.96 m/s (≈14 km/h). Finally, a possible reason for the increased activation of the long head of the triceps could be the additional 8 kg provided by the elastomeric garment. Considering the elastic capacity of the garment, it may be possible that the assistance capacity of the elastomeric garment at moderate speeds (8–10 km/h) is lower, causing greater work of the shoulder extensors, and therefore, greater muscle activation (e.g., the long head of the triceps brachii as it originates from the infraglenoid tubercle of the scapula) (Landin and Thompson, 2011). However, there were no significant differences in muscle activation in the later stages of the test, possibly because of the inertia of the movement that may be generated by a greater assistance capacity of the elastomeric garment at higher speeds (14 km/h, 16 km/h, and 18 km/h). This would be caused by a greater accumulation of elastic energy with increased inertia of trunk rotation. This study discovered greater muscle activation of the pectoralis major when using the elastomeric garment at the fastest test speeds (16 and 18 km/h), as indicated by statistical significance (p = 0.01, d = 0.47; p = 0.02, d = 0.55, respectively). Only a few studies reported data on pectoralis major activation during running (Milligan et al., 2014; Macadam et al., 2018). Thus, we propose that the increased resistance added by the elastomeric garment to the shoulder flexion during the higher stroke cadence at the highest speeds, compared to the lowest, may stimulate greater muscle activation of the pectoralis major.
Although there were significant increases in the activation of the rectus abdominis and anterior deltoid caused by the increase in speed during the test, it should be noted that there were no significant differences compared to the placebo garment. Regardless of wearing the elastomeric garment or the placebo, the evolution of muscle activation during the test coincided with previous studies (White and McNair, 2002). These authors indicate that the increase of trunk muscle activity during walking and slow running is low (0%–40%). In our study, from 10 to 12 km/h, it increased by 20.85% wearing the elastomeric garment, and by 7.56% wearing the placebo garment. According to Saunders et al. (Saunders et al., 2004), abdominal EMG increases with increasing running speed. In our study, from 10 to 18 km/h, activation of the rectus abdominis increased by 40.08% wearing the elastomeric garment, and by 39.61% wearing the placebo garment. Literature indicates that running involves a series of unilateral hip flexion and extension movements that can exert considerable destabilizing torque on the trunk (Saunders et al., 2004; Saunders et al., 2005; Behm et al., 2009). Therefore, one reason that could explain the greater activation of the rectus abdominis could be its stabilizing role against the lower extremity reaction moments and forces (Pellegrini et al., 2015). To counteract these forces, the abdominal musculature through the eccentric contraction phase decelerates lumbopelvic movement by increasing muscle activation levels (Saunders et al., 2005). Another aspect to consider is the increased number of exhalations during running (Saunders et al., 2004). Non-significant differences existed between the elastomeric garment and the placebo in the rectus abdominis activation. A possible reason may be that, according to the location of the elastomers, the elastomeric garment does not resist the direction of core movements (Kavcic et al., 2004) and, therefore, the effect of both garments on core muscle activity was the same. Regarding muscle activation of the deltoid during walking and running, the literature distinguishes two distinct approaches. Previous research suggests that the deltoid, together with the pectoralis major, is responsible for moving and stabilizing the upper arm at the glenohumeral joint to flex the shoulder during walking and running (Smoliga et al., 2010). However, other studies propose that the arm swing is mostly a passive response to the forces exerted on the torso by the leg swing and that the deltoid only acts to stabilize the shoulder through co-contraction or eccentric contraction, limiting its role in driving arm swing (Pontzer et al., 2009).
When capillary blood lactate is analyzed at the fingertip or earlobe, lactate concentration is an integrated indicator of (i) the release of lactate from muscle to blood, (ii) the distribution of blood lactate between the systemic circulation and blood tissue, and (iii) the uptake or depletion of lactate by muscles and other organs, such as the liver or heart (Sperlich et al., 2014). The results of our study indicate that the use of an elastomeric garment, which can also act as a compression garment, aids the recovery process by reducing the capillary blood lactate concentration after exercise. The use of the elastomeric garment (which can also act as a compression garment) generated significantly lower blood lactate immediately after the test (p = 0.001; d = 0.68) and after 5 min of rest (p < 0.001; d = 1.00) compared to the placebo. These differences are consistent with previous studies that compared the effect of a compressive garment versus a placebo garment during the execution of different physical exercise tests (Lovell et al., 2011; Sperlich et al., 2014). Other studies reported no influence of compression garments on lactate levels (Hamner et al., 2010; Sugiyama et al., 2013). Potential explanations for the lactate variations are that, during recovery, skeletal muscles facilitate blood return by rhythmic muscle contractions that create oscillations in intramuscular pressure, which increases blood flow and venous return (Notarius and Magder, 1996). Likewise, increased blood flow is thought to enhance lactate removal from exercising muscles, which facilitates faster redistribution to alternative sites such as the heart, liver, and non-working muscles (Menzies et al., 2010). Another possible explanation may be linked to arteriolar-venular communication. Hester and Hammer (Hester and Hammer, 2002) suggested that compression may increase shear stress and decrease the venular lumen, which would lead to the release of endothelial dilators and eventually generate dilation in the neighboring blood vessels (Reneman et al., 2006). The study by Rider et al. (Rider et al., 2014), suggested that the lower blood lactate concentration observed after wearing a compressive garment was the result of a shorter time under fatigue. However, considering that in our study, there were no significant differences between the time to exhaustion in both conditions (i.e., elastomeric garment and placebo garment), this is not a plausible explanation for our results. We did not find differences in the intra-test capillary blood lactate concentration between wearing the elastomeric garment and wearing the placebo garment. This is in contrast with the study by Lovell et al. (Lovell et al., 2011), who reported lower blood lactate during a running test performed wearing a compressive garment for the lower limbs compared to common running shorts. The lack of difference in the intra-test measurement in our study could be that the accumulated load (approx. 4 mins at 8–10 km/h) is insufficient to elicit significant acute metabolic changes in active, healthy, young participants with previous training experience. Another explanation for the non-significant differences in the intra-test lactate could be that the elastomeric garment is compressing the trunk and not the active muscles (lower limbs). In short, elastomeric garments that can also act as compression garments seem to maintain similar lactate levels during running, but significantly reduce lactate concentration immediately after and 5 minutes post-exercise.
The heart rate increased during the incremental treadmill test both with the elastomeric and placebo garments (Table 2). However, there were no differences in heart rate between the garments. This agrees with other studies in which similar conditions were applied (Ali et al., 2010; Gene-Morales et al., 2023). These findings could be related to the area of the body covered by the compression garment, as other studies have shown significant increases in heart rate when a full-body compression garment was worn (Sear et al., 2010), whereas compression garments that partially covered the body (e.g., torso, arms, thighs, calves) did not demonstrate modifications in heart rate (Ali et al., 2010; Mizuno et al., 2017), as was in our study. We did not find significant differences in systolic and diastolic blood pressure between conditions (Table 2), which is in accordance with other studies that did not report modifications in blood pressure after the application of compressive garments (Venckūnas et al., 2014; Book et al., 2016). Blood pressure is regulated by a central homeostatic mechanism, including cardiac output, blood volume, and peripheral resistance (Huonker et al., 1996). Previous studies have suggested that wearing graduated compression garments during and after exercise facilitates greater venous return, thus improving cardiac output and stroke volume, which in turn could improve blood flow (Liu et al., 2008). The studies by de Glanville et al. (de Glanville and Hamlin, 2012) and Liu et al. (Liu et al., 2008) used a pressure of approximately 10–14 mmHg at the ankle (Liu et al., 2008), calf, and thigh (de Glanville and Hamlin, 2012) without reporting significant differences between the conditions. Although previous studies have been successful in measuring specific pressures that generate changes in blood pressure (de Glanville and Hamlin, 2012), many other investigations have not analyzed the effect of applying a specific pressure to a specific region of the body. This association between mechanical pressure and blood pressure could explain certain discrepancies in the results, since in many investigations, mechanical pressure values were either too low to elicit the desired effects (Liu et al., 2008; de Glanville and Hamlin, 2012) or were not measured, as was the case in our study. Ultimately, the findings of this study indicate that the occlusion capacity of the elastomeric garment does not compromise the cardiovascular health of the participants, demonstrating its safety, in contrast to other compressive garments that are hemodynamically more demanding (Sear et al., 2010; Leoz-Abaurrea et al., 2016).
The incremental treadmill running protocol employed in this study did not result in significant differences between the conditions in global and respiratory effort perception. These results are in line with those of Ali et al. (Ali et al., 2010) and Sperlich et al. (Sperlich et al., 2010; Sperlich et al., 2014), who analyzed the effect of compressive garments on the lower and upper extremities in long-distance and sprint running tests. Another study by Vincent et al. (Vincent et al., 2018) stated that adding an external resistance through hand-held water bottles during running could elicit a higher perception of muscle exertion. Oppositely, we found that adding an external resistance through the elastomeric garment did not modify effort perception. Therefore, we consider these results to be positive since the elastomeric garment generated a series of improvements in physiological parameters without the participants perceiving the effort as more exhausting.
In conclusion, the elastomeric garment worn during the incremental treadmill test allowed participants to achieve greater muscle activation in determinate muscles and speeds without perceiving the exercise as more strenuous. Additionally, lower post-exercise capillary blood lactate levels were obtained with the elastomeric garment. Finally, it was demonstrated that using the elastomeric garment to run is as safe as running with a placebo garment regarding cardiovascular parameters. All these milestones mostly indicate that the elastomeric garment does not impair the running performance and could optimize determinate physiological parameters.
It is critical to acknowledge that the outcomes of this study are constrained by the specific dependent and independent variables analyzed. Consequently, further research that includes participants with diverse experience, physical fitness levels, and from both sexes is required. New variables could be examined: (i) maximal oxygen consumption, to estimate the actual metabolic stress depending on the fitness status of each participant; (ii) creatine kinase values and changes in muscle volume, stiffness, and circumference to assess muscle damage; (iii) the effects of the elastomeric garment on the skin to analyze thermoregulation; and (iv) aspects linked to sports biomechanics, such as running technique, joint angles, oscillatory patterns between limbs and muscles, and range of motion. Similarly, it would be interesting to analyze performance and muscle function in tests related to agility and jumping.
The raw data supporting the conclusion of this article will be made available by the authors, without undue reservation.
The studies involving humans were approved by Human Research Ethics Committee of the University of Valencia (H20190325095509 and H1460994903890). The studies were conducted in accordance with the local legislation and institutional requirements. The participants provided their written informed consent to participate in this study.
DJ: Formal Analysis, Writing–original draft, Writing–review and editing. AS-B: Writing–original draft, Writing–review and editing, Conceptualization, Data curation, Investigation. CB-L: Conceptualization, Writing–original draft, Writing–review and editing. FM-R: Writing–original draft, Writing–review and editing, Formal Analysis. PJ-M: Formal Analysis, Writing–original draft, Writing–review and editing. AS-H: Writing–original draft, Writing–review and editing, Conceptualization, Investigation. JM-P: Conceptualization, Investigation, Writing–original draft, Writing–review and editing. PF-N: Conceptualization, Investigation, Writing–original draft, Writing–review and editing. AJ: Conceptualization, Investigation, Writing–original draft, Writing–review and editing. JG-M: Conceptualization, Investigation, Writing–original draft, Writing–review and editing. IC-M: Writing–original draft, Writing–review and editing, Formal Analysis. JC: Conceptualization, Formal Analysis, Investigation, Writing–original draft, Writing–review and editing.
The author(s) declare that financial support was received for the research, authorship, and/or publication of this article. This work was supported by grants from the National Natural Science Foundation of China (grant 12250410237) and the Generalitat Valenciana of Spain (Angel Saez-Berlanga’s predoctoral grant CIACIF/2021/189, European Social Fund).
We thank the participants for their voluntary collaboration and Josep Romero Sánchez for his help in the laboratory. We would also like to thank Menatechpro System© for providing us with the elastomeric and placebo garments.
This research has been developed under the advice and technical support contract signed between the University of Valencia (Spain) and Menatechpro System©, with JC being the professor responsible for the University. The rest of the article’s coauthors are members of the Research Group led by JC.
All claims expressed in this article are solely those of the authors and do not necessarily represent those of their affiliated organizations, or those of the publisher, the editors and the reviewers. Any product that may be evaluated in this article, or claim that may be made by its manufacturer, is not guaranteed or endorsed by the publisher.
Ali A., Creasy R. H., Edge J. A. (2010). Physiological effects of wearing graduated compression stockings during running. Eur. J. Appl. Physiol. 109 (6), 1017–1025. doi:10.1007/s00421-010-1447-1
Ali A., Creasy R. H., Edge J. A. (2011). The effect of graduated compression stockings on running performance. J. Strength Cond. Res. 25 (5), 1385–1392. doi:10.1519/JSC.0b013e3181d6848e
Aliverti A., Kayser B., Mauro A. L., Quaranta M., Pompilio P., Dellacà R. L., et al. (2011). Respiratory and leg muscles perceived exertion during exercise at altitude. Respir. Physiol. Neurobiol. 177 (2), 162–168. doi:10.1016/j.resp.2011.03.014
Alix-Fages C., Del Vecchio A., Baz-Valle E., Santos-Concejero J., Balsalobre-Fernández C. (2022). The role of the neural stimulus in regulating skeletal muscle hypertrophy. Eur. J. Appl. Physiol. 122 (5), 1111–1128. doi:10.1007/s00421-022-04906-6
Argus C. K., Gill N. D., Keogh J. W., Blazevich A. J., Hopkins W. G. (2011). Kinetic and training comparisons between assisted, resisted, and free countermovement jumps. J. Strength Cond. Res. 25 (8), 2219–2227. doi:10.1519/JSC.0b013e3181f6b0f4
Babiloni-Lopez C., Gene-Morales J., Saez-Berlanga A., Ramirez-Campillo R., Moreno-Murcia J. A., Colado J. C. (2022). The use of elastic bands in velocity-based training allows greater acute external training stimulus and lower perceived effort compared to weight plates. Int. J. Environ. Res. Public Health. 19 (24), 16616. doi:10.3390/ijerph192416616
Beck T. W. (2013). The importance of a priori sample size estimation in strength and conditioning research. J. Strength Cond. Res. 27 (8), 2323–2337. doi:10.1519/JSC.0b013e318278eea0
Behm D. G., Cappa D., Power G. A. (2009). Trunk muscle activation during moderate- and high-intensity running. Appl. Physiol. Nutr. Metab. 34 (6), 1008–1016. doi:10.1139/H09-102
Beneke R., Leithäuser R. M., Ochentel O. (2011). Blood lactate diagnostics in exercise testing and training. Int. J. Sports Physiol. Perform. 6 (1), 8–24. doi:10.1123/ijspp.6.1.8
Book J., Prince C. N., Villar R., Hughson R. L., Peterson S. D. (2016). Investigating the impact of passive external lower limb compression on central and peripheral hemodynamics during exercise. Eur. J. Appl. Physiol. 116 (4), 717–727. doi:10.1007/s00421-016-3331-0
Borg G. A. (1982). Psychophysical bases of perceived exertion. Med. Sci. Sports Exerc 14 (5), 377–381. doi:10.1249/00005768-198205000-00012
Calatayud J., Borreani S., Colado J. C., Martin F., Rogers M. E. (2014). Muscle activity levels in upper-body push exercises with different loads and stability conditions. Phys. Sportsmed. 42 (4), 106–119. doi:10.3810/psm.2014.11.2097
Calatayud J., Casaña J., Martín F., Jakobsen M. D., Colado J. C., Gargallo P., et al. (2017). Trunk muscle activity during different variations of the supine plank exercise. Musculoskelet. Sci. Pract. 28, 54–58. doi:10.1016/j.msksp.2017.01.011
Cerezuela-Espejo V., Courel-Ibanez J., Moran-Navarro R., Martinez-Cava A., Pallares J. G. (2018). The relationship between lactate and ventilatory thresholds in runners: validity and reliability of exercise test performance parameters. Front. Physiol. 9, 1320. doi:10.3389/fphys.2018.01320
Cipriani D. J., Yu T. S., Lyssanova O. (2014). Perceived influence of a compression, posture-cueing shirt on cyclists’ ride experience and post-ride recovery. J. Chiropr. Med. 13 (1), 21–27. doi:10.1016/j.jcm.2014.01.007
Cohen J. (2009). “Statistical power analysis for the behavioral sciences,” in reprint. 2. (New York, NY: Psychology Press), 567.
Colado J. C., Furtado G. E., Teixeira A. M., Flandez J., Naclerio F. (2020). Concurrent and construct validation of a new scale for rating perceived exertion during elastic resistance training in the elderly. J. Sports Sci. Med. 19 (1), 175–186.
Colado J. C., Garcia-Masso X., Triplett N. T., Calatayud J., Flandez J., Behm D., et al. (2014). Construct and concurrent validation of a new resistance intensity scale for exercise with thera-band® elastic bands. J. Sports Sci. Med. 13 (4), 758–766.
Colado J. C., Garcia-Masso X., Triplett T. N., Flandez J., Borreani S., Tella V. (2012). Concurrent validation of the OMNI-resistance exercise scale of perceived exertion with Thera-band resistance bands. J. Strength Cond. Res. 26 (11), 3018–3024. doi:10.1519/JSC.0b013e318245c0c9
Colado J. C., Gené-Morales J., Jiménez-Martínez P., Flandez J., Ferri-Caruana A. M., Babiloni-Lopez C. (2023). Rating of perceived exertion in the first repetition is related to the total repetitions performed in elastic bands training. Mot. Control 27 (4), 830–843. doi:10.1123/mc.2023-0017
Colado J. C., Pedrosa F. M., Juesas A., Gargallo P., Carrasco J. J., Flandez J., et al. (2018). Concurrent validation of the OMNI-Resistance Exercise Scale of perceived exertion with elastic bands in the elderly. Exp. Gerontol. 103, 11–16. doi:10.1016/j.exger.2017.12.009
de Glanville K. M., Hamlin M. J. (2012). Positive effect of lower body compression garments on subsequent 40-kM cycling time trial performance. J. Strength Cond. Res. 26 (2), 480–486. doi:10.1519/JSC.0b013e318225ff61
Farina D., Enoka R. M. (2023). Evolution of surface electromyography: from muscle electrophysiology towards neural recording and interfacing. J. Electromyogr. Kinesiol 71, 102796. doi:10.1016/j.jelekin.2023.102796
Faul F., Erdfelder E., Lang A. G., Buchner A. (2007). G*Power 3: a flexible statistical power analysis program for the social, behavioral, and biomedical sciences. Behav. Res. Methods 39 (2), 175–191. doi:10.3758/bf03193146
Feser E. H., Korfist C., Lindley K., Bezodis N. E., Clark K., Cronin J. (2021). The effects of lower-limb wearable resistance on sprint performance in high school American football athletes: a nine-week training study. Int. J. Sports Sci. Coach. 16 (5), 1187–1195. doi:10.1177/17479541211003403
Gene-Morales J., Gené-Sampedro A., Salvador R., Colado J. C. (2020). Adding the load just above sticking point using elastic bands optimizes squat performance, perceived effort rate, and cardiovascular responses. J. Sports Sci. Med. 19 (4), 735–744.
Gene-Morales J., Gené-Sampedro A., Salvador R., Colado J. C. (2022). Effects of squatting with elastic bands or conventional resistance-training equipment at different effort levels in post-exercise intraocular pressure of healthy men. Biol. Sport 39 (4), 895–903. doi:10.5114/biolsport.2022.109955
Gene-Morales J., Saez-Berlanga A., Babiloni-Lopez C., Jiménez-Martínez P., Ferri-Carruana A. M., Martin-Rivera F., et al. (2023). Effects of an elastomeric technology garment on different external and internal load variables: a pilot study. Sci. J. Sport Perform. 2 (2), 165–176. doi:10.55860/bxnk5984
Gibala M. J., Jones A. M. (2013). Physiological and performance adaptations to high-intensity interval training. Nestle Nutr. Inst. Workshop Ser. 76, 51–60. doi:10.1159/000350256
Gripp F., Nava R. C., Cassilhas R. C., Esteves E. A., Magalhães C. O. D., Dias-Peixoto M. F., et al. (2021). HIIT is superior than MICT on cardiometabolic health during training and detraining. Eur. J. Appl. Physiol. 121 (1), 159–172. doi:10.1007/s00421-020-04502-6
Hammami R., Gene-Morales J., Abed F., Selmi M. A., Moran J., Colado J. C., et al. (2022). An eight-weeks resistance training programme with elastic band increases some performance-related parameters in pubertal male volleyball players. Biol. Sport 39 (1), 219–226. doi:10.5114/biolsport.2021.101601
Hamner S. R., Seth A., Delp S. L. (2010). Muscle contributions to propulsion and support during running. J. Biomech. 43 (14), 2709–2716. doi:10.1016/j.jbiomech.2010.06.025
Hermens H. J., Freriks B., Disselhorst-Klug C., Rau G. (2000). Development of recommendations for SEMG sensors and sensor placement procedures. J. Electromyogr. Kinesiol. 10 (5), 361–374. doi:10.1016/s1050-6411(00)00027-4
Hester R. L., Hammer L. W. (2002). Venular-arteriolar communication in the regulation of blood flow. Am. J. Physiol. Regul. Integr. Comp. Physiol. 282 (5), R1280–R1285. doi:10.1152/ajpregu.00744.2001
Huonker M., Halle M., Keul J. (1996). Structural and functional adaptations of the cardiovascular system by training. Int. J. Sports Med. 17 (3), S164–S172. doi:10.1055/s-2007-972919
Kavcic N., Grenier S., McGill S. M. (2004). Quantifying tissue loads and spine stability while performing commonly prescribed low back stabilization exercises. Spine (Phila Pa 1976) 29 (20), 2319–2329. doi:10.1097/01.brs.0000142222.62203.67
Knight C. A., Caldwell G. E. (2000). Muscular and metabolic costs of uphill backpacking: are hiking poles beneficial? Med. Sci. Sports Exerc 32 (12), 2093–2101. doi:10.1097/00005768-200012000-00020
Landin D., Thompson M. (2011). The shoulder extension function of the triceps brachii. J. Electromyogr. Kinesiol. 21 (1), 161–165. doi:10.1016/j.jelekin.2010.09.005
Leoz-Abaurrea I., Santos-Concejero J., Grobler L., Engelbrecht L., Aguado-Jiménez R. (2016). Running performance while wearing a heat dissipating compression garment in male recreational runners. J. Strength and Cond. Res. 30 (12), 3367–3372. doi:10.1519/JSC.0000000000001467
Lewthwaite H., Jensen D. (2021). Multidimensional breathlessness assessment during cardiopulmonary exercise testing in healthy adults. Eur. J. Appl. Physiol. 121 (2), 499–511. doi:10.1007/s00421-020-04537-9
Liu R., Lao T. T., Kwok Y. L., Li Y., Ying M. T. C. (2008). Effects of graduated compression stockings with different pressure profiles on lower-limb venous structures and haemodynamics. Adv. Ther. 25 (5), 465–478. doi:10.1007/s12325-008-0058-2
Lovell D. I., Mason D. G., Delphinus E. M., McLellan C. P. (2011). Do compression garments enhance the active recovery process after high-intensity running? J. Strength Cond. Res. 25 (12), 3264–3268. doi:10.1519/JSC.0b013e31821764f8
Macadam P., Cronin J. B., Uthoff A. M., Johnston M., Knicker A. J. (2018). Role of arm mechanics during sprint running: a review of the literature and practical applications. Strength and Cond. J. 40 (5), 14–23. doi:10.1519/ssc.0000000000000391
Marqués-Jiménez D., Calleja-González J., Arratibel-Imaz I., Delextrat A., Uriarte F., Terrados N. (2018). Influence of different types of compression garments on exercise-induced muscle damage markers after a soccer match. Res. Sports Med. 26 (1), 27–42. doi:10.1080/15438627.2017.1393755
Marriner C. R., Cronin J. B., Macadam P., Storey A. (2017). Redistributing load using wearable resistance during power clean training improves athletic performance. Eur. J. Sport Sci. 17 (9), 1101–1109. doi:10.1080/17461391.2017.1360396
Menzies P., Menzies C., McIntyre L., Paterson P., Wilson J., Kemi O. J. (2010). Blood lactate clearance during active recovery after an intense running bout depends on the intensity of the active recovery. J. Sports Sci. 28 (9), 975–982. doi:10.1080/02640414.2010.481721
Milanović Z., Sporiš G., Weston M. (2015). Effectiveness of high-intensity interval training (hit) and continuous endurance training for VO2max improvements: a systematic review and meta-analysis of controlled trials. Sports Med. 45 (10), 1469–1481. doi:10.1007/s40279-015-0365-0
Milligan A., Mills C., Scurr J. (2014). The effect of breast support on upper body muscle activity during 5 km treadmill running. Hum. Mov. Sci. 38, 74–83. doi:10.1016/j.humov.2014.06.001
Mizuno S., Arai M., Todoko F., Yamada E., Goto K. (2017). Wearing compression tights on the thigh during prolonged running attenuated exercise-induced increase in muscle damage marker in blood. Front. Physiol. 8, 834. doi:10.3389/fphys.2017.00834
Notarius C. F., Magder S. (1996). Central venous pressure during exercise: role of muscle pump. Can. J. Physiol. Pharmacol. 74 (6), 647–651. doi:10.1139/cjpp-74-6-647
Pellegrini B., Peyré-Tartaruga L. A., Zoppirolli C., Bortolan L., Bacchi E., Figard-Fabre H., et al. (2015). Exploring muscle activation during nordic walking: a comparison between conventional and uphill walking. PLoS One. 10 (9), e0138906. doi:10.1371/journal.pone.0138906
Pontzer H., Holloway J. H., Raichlen D. A., Lieberman D. E. (2009). Control and function of arm swing in human walking and running. J. Exp. Biol. 212 (4), 523–534. doi:10.1242/jeb.024927
Reneman R. S., Arts T., Hoeks A. P. G. (2006). Wall shear stress--an important determinant of endothelial cell function and structure--in the arterial system in vivo. Discrepancies with theory. J. Vasc. Res. 43 (3), 251–269. doi:10.1159/000091648
Rider B. C., Coughlin A. M., Hew-Butler T. D., Goslin B. R. (2014). Effect of compression stockings on physiological responses and running performance in division III collegiate cross-country runners during a maximal treadmill test. J. Strength Cond. Res. 28 (6), 1732–1738. doi:10.1519/JSC.0000000000000287
Saez-Berlanga Á., Babiloni-Lopez C., Ferri-Caruana A. M., Jiménez-Martínez P., Garcia-Ramos A., Flandez J., et al. (2024). A new sports garment with elastomeric technology optimizes physiological, mechanical, and psychological acute responses to pushing upper-limb resistance exercises. PeerJ 12, e17008. doi:10.7717/peerj.17008
Saez-Berlanga A., Gargallo P., Gene-Morales J., Babiloni C., Colado J. C., Juesas A. (2022). Multicomponent elastic training improves short-term body composition and balance in older women. Sci. J. Sport Perform. 1 (1), 4–13. doi:10.55860/neqh2786
Saunders S. W., Rath D., Hodges P. W. (2004). Postural and respiratory activation of the trunk muscles changes with mode and speed of locomotion. Gait Posture 20 (3), 280–290. doi:10.1016/j.gaitpost.2003.10.003
Saunders S. W., Schache A., Rath D., Hodges P. W. (2005). Changes in three dimensional lumbo-pelvic kinematics and trunk muscle activity with speed and mode of locomotion. Clin. Biomech. 20 (8), 784–793. doi:10.1016/j.clinbiomech.2005.04.004
Scheer V., Ramme K., Reinsberger C., Heitkamp H. C. (2018). VO2max testing in trail runners: is there a specific exercise test protocol? Int. J. Sports Med. 39 (6), 456–461. doi:10.1055/a-0577-4851
Scherr J., Wolfarth B., Christle J. W., Pressler A., Wagenpfeil S., Halle M. (2013). Associations between Borg’s rating of perceived exertion and physiological measures of exercise intensity. Eur. J. Appl. Physiol. 113 (1), 147–155. doi:10.1007/s00421-012-2421-x
Sear J. A., Hoare T. K., Scanlan A. T., Abt G. A., Dascombe B. J. (2010). The effects of whole-body compression garments on prolonged high-intensity intermittent exercise. J. Strength Cond. Res. 24 (7), 1901–1910. doi:10.1519/JSC.0b013e3181db251b
Smoliga J. M., Myers J. B., Redfern M. S., Lephart S. M. (2010). Reliability and precision of EMG in leg, torso, and arm muscles during running. J. Electromyogr. Kinesiol 20 (1), e1–e9. doi:10.1016/j.jelekin.2009.09.002
Sperlich B., Born D. P., Zinner C., Hauser A., Holmberg H. C. (2014). Does upper-body compression improve 3 × 3-min double-poling sprint performance? Int. J. Sports Physiol. Perform. 9 (1), 48–57. doi:10.1123/ijspp.2013-0137
Sperlich B., Haegele M., Achtzehn S., Linville J., Holmberg H. C., Mester J. (2010). Different types of compression clothing do not increase sub-maximal and maximal endurance performance in well-trained athletes. J. Sports Sci. 28 (6), 609–614. doi:10.1080/02640410903582768
Sugiyama K., Kawamura M., Tomita H., Katamoto S. (2013). Oxygen uptake, heart rate, perceived exertion, and integrated electromyogram of the lower and upper extremities during level and Nordic walking on a treadmill. J. Physiol. Anthropol. 32 (1), 2. doi:10.1186/1880-6805-32-2
Tran T. T., Brown L. E., Coburn J. W., Lynn S. K., Dabbs N. C. (2012). Effects of assisted jumping on vertical jump parameters. Curr. Sports Med. Rep. 11 (3), 155–159. doi:10.1249/JSR.0b013e31825640bb
Upton D. E. (2011). The effect of assisted and resisted sprint training on acceleration and velocity in Division IA female soccer athletes. J. Strength Cond. Res. 25 (10), 2645–2652. doi:10.1519/JSC.0b013e318201be16
Venckūnas T., Trinkūnas E., Kamandulis S., Poderys J., Grūnovas A., Brazaitis M. (2014). Effect of lower body compression garments on hemodynamics in response to running session. ScientificWorldJournal 2014, 353040. doi:10.1155/2014/353040
Vincent H. K., Zdziarski L. A., Fallgatter K., Negron G., Chen C., Leavitt T., et al. (2018). Running mechanics and metabolic responses with water bottles and bottle belt holders. Int. J. Sports Physiol. Perform. 13 (8), 977–985. doi:10.1123/ijspp.2017-0184
Weston M., Taylor K. L., Batterham A. M., Hopkins W. G. (2014). Effects of low-volume high-intensity interval training (HIT) on fitness in adults: a meta-analysis of controlled and non-controlled trials. Sports Med. 44 (7), 1005–1017. doi:10.1007/s40279-014-0180-z
Wewege M., van den Berg R., Ward R. E., Keech A. (2017). The effects of high-intensity interval training vs moderate-intensity continuous training on body composition in overweight and obese adults: a systematic review and meta-analysis. Obes. Rev. 18 (6), 635–646. doi:10.1111/obr.12532
White S. G., McNair P. J. (2002). Abdominal and erector spinae muscle activity during gait: the use of cluster analysis to identify patterns of activity. Clin. Biomech. 17 (3), 177–184. doi:10.1016/s0268-0033(02)00007-4
Keywords: treadmill test, variable resistance training, muscle activation, metabolic and cardiovascular responses, physical performance
Citation: Janicijevic D, Saez-Berlanga A, Babiloni-Lopez C, Martin-Rivera F, Jiménez-Martínez P, Silvestre-Herrero A, Martínez-Puente J, Ferradás-Nogueira P, Juesas A, Gene-Morales J, Chulvi-Medrano I and Colado JC (2024) Acute physiological and psychological responses during an incremental treadmill test wearing a new upper-body sports garment with elastomeric technology. Front. Physiol. 15:1372020. doi: 10.3389/fphys.2024.1372020
Received: 17 January 2024; Accepted: 18 March 2024;
Published: 22 April 2024.
Edited by:
Juan M. Cortell-Tormo, University of Alicante, SpainReviewed by:
Diego Marqués-Jiménez, University of Valladolid, SpainCopyright © 2024 Janicijevic, Saez-Berlanga, Babiloni-Lopez, Martin-Rivera, Jiménez-Martínez, Silvestre-Herrero, Martínez-Puente, Ferradás-Nogueira, Juesas, Gene-Morales, Chulvi-Medrano and Colado. This is an open-access article distributed under the terms of the Creative Commons Attribution License (CC BY). The use, distribution or reproduction in other forums is permitted, provided the original author(s) and the copyright owner(s) are credited and that the original publication in this journal is cited, in accordance with accepted academic practice. No use, distribution or reproduction is permitted which does not comply with these terms.
*Correspondence: Iván Chulvi-Medrano, aXZhbi5jaHVsdmlAdXYuZXM=
Disclaimer: All claims expressed in this article are solely those of the authors and do not necessarily represent those of their affiliated organizations, or those of the publisher, the editors and the reviewers. Any product that may be evaluated in this article or claim that may be made by its manufacturer is not guaranteed or endorsed by the publisher.
Research integrity at Frontiers
Learn more about the work of our research integrity team to safeguard the quality of each article we publish.