- 1Laboratory of Pharmacology, São Francisco University Medical School, Bragança Paulista, Brazil
- 2Department of Pharmacology, Escola Paulista de Medicina, Universidade Federal de São Paulo, São Paulo, Brazil
- 3The James Buchanan Brady Urological Institute and Department of Urology, The Johns Hopkins School of Medicine, Baltimore, MD, United States
- 4Hematology and Hemotherapy Center, University of Campinas, Campinas, Brazil
Patients with sickle cell disease (SCD) display an overactive bladder (OAB). Intravascular hemolysis in SCD is associated with various severe SCD complications. However, no experimental studies have evaluated the effect of intravascular hemolysis on bladder function. This study aimed to assess the effects of intravascular hemolysis on the micturition process and the contractile mechanisms of the detrusor smooth muscle (DSM) in a mouse model with phenylhydrazine (PHZ)-induced hemolysis; furthermore, it aimed to investigate the role of intravascular hemolysis in the dysfunction of nitric oxide (NO) signaling and in increasing oxidative stress in the bladder. Mice underwent a void spot assay, and DSM contractions were evaluated in organ baths. The PHZ group exhibited increased urinary frequency and increased void volumes. DSM contractile responses to carbachol, KCl, α-β-methylene-ATP, and EFS were increased in the PHZ group. Protein expression of phosphorylated endothelial NO synthase (eNOS) (Ser-1177), phosphorylated neuronal NO synthase (nNOS) (Ser-1417), and phosphorylated vasodilator-stimulated phosphoprotein (VASP) (Ser-239) decreased in the bladder of the PHZ group. Protein expression of oxidative stress markers, NOX-2, 3-NT, and 4-HNE, increased in the bladder of the PHZ group. Our study shows that intravascular hemolysis promotes voiding dysfunction correlated with alterations in the NO signaling pathway in the bladder, as evidenced by reduced levels of p-eNOS (Ser-1177), nNOS (Ser-1417), and p-VASP (Ser-239). The study also showed that intravascular hemolysis increases oxidative stress in the bladder. Our study indicates that intravascular hemolysis promotes an OAB phenotype similar to those observed in patients and mice with SCD.
1 Introduction
Sickle cell disease (SCD), an autosomal recessive genetic disorder, is characterized by abnormal hemoglobin S (HbS) production due to a single amino acid substitution in the β-globin chain (Kato et al., 2018). This genetic mutation triggers the polymerization of HbS under hypoxic or dehydrated conditions, forming sickle-shaped erythrocytes. These altered cells exhibit increased stiffness and a reduced lifespan, leading to intravascular and extravascular hemolysis, which are critical features of SCD and contribute to its diverse clinical manifestations (Kato et al., 2018). A significant molecular consequence of intravascular hemolysis is the reduction of nitric oxide (NO) bioavailability due to direct NO-hemoglobin interaction and increased reactive oxygen species (ROS) production, which act as NO scavengers (Reiter et al., 2002; Vona et al., 2021). This reduction in NO is associated with various severe SCD complications, including leg ulceration, pulmonary hypertension, priapism, and overactive bladder (OAB) (Nolan et al., 2005; Kato et al., 2006; Cita et al., 2016).
The functions of the urinary bladder, encompassing urine storage and voiding, are regulated by a complex interplay of neurotransmitters (Andersson and Arner, 2004). OAB, a clinical condition marked by persistent urgency to urinate, may occur with or without urge incontinence and is commonly accompanied by increased urination frequency and nocturia (Eapen and Radomski, 2016). Notably, in SCD patients, the prevalence of OAB is significant, with clinical studies suggesting that up to 40% of these patients may exhibit symptoms of OAB (Portocarrero et al., 2012; Anele et al., 2015). A common contributor to OAB is the heightened contraction of the detrusor smooth muscle during the urine storage phase, leading to detrusor overactivity (Michel and Chapple, 2009).
The NO-cyclic guanosine monophosphate (cGMP) signaling pathway plays an essential role in the normal functioning of the urinary tract. NO, synthesized by endothelial NO synthase (eNOS) and neuronal NO synthase (nNOS), is crucial for maintaining the tone and functionality of detrusor smooth muscle (Burnett et al., 1997; Mónica et al., 2008; Karakus et al., 2019). NO deficiency has been linked with the OAB phenotype and increased detrusor smooth muscle contraction in SCD mice and various experimental models (Khan et al., 1999; Mónica et al., 2011; Leiria et al., 2013; Leiria et al., 2014; Karakus et al., 2019; Musicki et al., 2019; Karakus et al., 2020; Lee et al., 2021). Furthermore, increased superoxide production by the NOX-2 isoform of NADPH oxidase, which acts by activating NO, also contributes to the pathophysiology of OAB in animal models (Alexandre et al., 2016; 2018; Akakpo et al., 2017; de Oliveira et al., 2022) but has not yet been evaluated in the lower urinary tract in SCD.
To date, previous studies have used SCD transgenic mice to investigate bladder alterations (Claudino et al., 2015; Karakus et al., 2019; 2020; Musicki et al., 2019). These studies reported voiding dysfunction and detrusor hypercontractility associated with reduced NO bioavailability in the bladder. However, the exclusive effects of intravascular hemolysis on the bladder have not been independently analyzed. Given the critical role of intravascular hemolysis in SCD and its potential impact on the NO signaling pathway in the bladder, we hypothesize that intravascular hemolysis contributes significantly to micturition dysfunction.
The phenylhydrazine (PHZ)-induced hemolysis model permits precise control over the onset and intensity of hemolysis (Lim et al., 1998; Dutra et al., 2014; Gotardo et al., 2023), enabling a direct correlation between hemolysis and the observed functional and molecular changes in the bladder. This model is precious when the aim is to study the exclusive effect of intravascular hemolysis, as SCD mice exhibit additional alterations beyond intravascular hemolysis. This study endeavors to fill this critical gap in knowledge, providing an in-depth understanding of the mechanisms underlying intravascular hemolysis-induced micturition dysfunction.
This study is designed to delineate the consequences of intravascular hemolysis on the micturition process and the contractile mechanisms of the detrusor smooth muscle in a mouse model of PHZ-induced hemolysis. Furthermore, we investigate the role of intravascular hemolysis in elevating ROS production in the bladder, as well as to assess changes in phosphorylated eNOS (Ser-1177), phosphorylated nNOS (Ser-1417), and phosphorylated vasodilator-stimulated phosphoprotein (p-VASP Ser-239).
2 Materials and methods
2.1 Ethical approval
All animal study protocols in this study were approved by the Ethics Committee on Animal Use of the University of San Francisco (CEUA/USF, Permit number V3:008.06.2021).
2.2 Animals and treatment
Animal procedures and experimental protocols were performed in accordance with the ethical principles in animal research adopted by the Brazilian College for Animal Experimentation and followed the Guide for the Care and Use of Laboratory Animals. All mouse strains were originally purchased from Jackson Laboratories (Bar Harbor, ME). Characterization and breeding were performed at the Multidisciplinary Center for the Investigation of Biological Science in Laboratory Animals of the University of Campinas. We used C57BL/6 male mice (control), aged 3–4 months old, housed five per cage on a 12 h light–dark cycle.
We injected PHZ at 50 mg/kg in C57BL/6 mice intraperitoneally to induce intravascular hemolysis. The mice were reinjected with 50 mg/kg 8 h later and were sacrificed 4 days after starting PHZ treatment. Control mice were treated with the saline vehicle simultaneously with the PHZ group (Henrique Silva et al., 2018).
2.3 Void spot assay
Mice were moved individually to empty mouse cages with precut qualitative filter paper (250 g) on the bottom. They were provided with food but no water. After 4 hours, the filter papers were removed and allowed to dry before being imaged using UV light transillumination and captured using the ChemiDoc MP imaging system with Image Laboratory software (Bio-Rad Laboratories, Hercules, CA). Captured images were saved in grayscale-tagged image file format (TIFF) (Figure 2A) and analyzed using ImageJ Software (National Institute of Health, Bethesda-MD, United States of America). ImageJ particle analysis was performed on >0.02 cm2 spots to reduce areas of non-specific fluorescence and artifacts that may have been created by debris and feces (Keil et al., 2016). A linear standard measurement curve was used to convert void spot areas to volumes, and total volumes were normalized to body weight. Assays were performed for each animal between 9 a.m. and 2 p.m.
2.4 Functional studies of bladder strips and concentration–response curves
Two longitudinal detrusor smooth muscle strips with intact urothelium were obtained from each bladder. The strips were mounted in 5 mL myograph organ baths (Danish Myo Technology, Aarhus, Denmark) containing Krebs–Henseleit solution composed of 117 mM NaCl, 4.7 mM KCl, 2.5 mM CaCl2, 1.2 mM MgSO4, 1.2 mM KH2PO4, 25 mM NaHCO3, and 11 mM glucose, continuously bubbled with a mixture of 95% O2 and 5% CO2 (pH 7.4) at 37°C. Changes in isometric force were recorded using a PowerLab Data Acquisition System (Software LabChart, version 7.0, ADInstruments, MA, United States of America).The resting tension was adjusted to 5 mN at the beginning of the experiments. The equilibration period was 60 min, and the bathing medium was changed every 15 min.
Cumulative concentration-response curves for the full muscarinic agonist carbachol (1 nM–30 µM) and potassium chloride (KCl; 1–300 mM) were obtained in detrusor strips. In separate experiments, electrical field stimulation (EFS)-induced contraction (20 V, 10 s of stimulation at varying frequencies, a 2-min interval between each pulse) was carried out. Non-cumulative concentration–response curves were also made for the purinergic agonist (P2X), α-β-methylene-ATP (1 μM, 3 μM, and 10 µM).
Non-linear regression analysis used GraphPad Prism (GraphPad Software, San Diego, CA, United States of America). Maximal response (Emax) data were normalized to the wet weight of the respective urinary bladder strips. Using GraphPad Prism software, EC50 values, represented as the negative logarithm (pEC50), were calculated by fitting the concentration–response relationship to a sigmoidal model (log-concentrations vs. response).
2.5 Western blot analysis
The separation of proteins from biological samples of tissue homogenates (detrusor) was performed through electrophoresis in 4%–20% polyacrylamide with 0.1% sodium sulfate (SDS-Page). Then, the protein bands were transferred electrophoretically into a submerged nitrocellulose membrane system. Non-specific protein binding to nitrocellulose was reduced by “overnight” pre-incubation of the membrane with a blocking solution (5% milk powder, 10 mm Tris, 100 mm NaCl, and 0.02% Tween 20). The bladder from each mouse was homogenized in lysis buffer and centrifuged at 12,000 g for 20 min at 4°C. Homogenates containing 70 μg of total proteins were run on 4%–20% Tris-HCl gels (Bio-Rad Laboratories, Hercules, CA, United States of America) and transferred to a nitrocellulose membrane. Non-fat dry milk (5%) (Bio-Rad) in Tris-buffered saline/Tween was used for 60 min at 24°C to block non-specific binding sites. Membranes were incubated for 15–18 h at 4°C with the following antibodies: monoclonal anti-3-nitrotyrosine (3-NT; 1:3000, Abcam), polyclonal anti-4-HNE antibody (1:3000, Abcam), anti-NOX-2 antibody (1:1000, Sigma-Aldrich), monoclonal anti-phospho(p)-eNOS (Ser-1177) antibody (1:1000, Cell Signaling), polyclonal anti-eNOS antibody (1:1000, Cell Signaling), polyclonal anti-phospho(p)-VASP (Ser-239) (1:1000, Cell Signaling), monoclonal anti-VASP antibody (1:1000, Cell Signaling), polyclonal phospho(p)-nNOS antibody (Ser-1417) (1:1000, Abcam), nNOS (1:1000, Millipore), and β-actin (1:5000, Santa Cruz Biotechnology). Densitometry was analyzed using ImageJ software (National Institute of Health, Bethesda-MD, United States of America). Quantified densitometry results were normalized to β-actin.
2.6 Drugs
Carbachol, α-β-methylene-ATP, PHZ, and KCl were purchased from Sigma-Aldrich (St Louis, MO, United States of America. All reagents were required to be of analytical grade. Deionized water was used as a solvent, and working solutions were diluted prior to use.
2.7 Statistical analysis
The GraphPad Prism Program (GraphPad Software Inc.) was used for statistical analysis. Data are expressed as the mean ± SEM of N experiments. Statistical comparisons were made using the Student’s unpaired t-test. A value of p < 0.05 was considered statistically significant.
3 Results
3.1 Hematological parameters
Mice treated with PHZ exhibited significantly reduced levels of red blood cells (Figure 1A) and total hemoglobin (Figure 1B) compared to the control group (p < 0.05). Furthermore, there was a marked increase in plasma hemoglobin concentrations in the PHZ group (p < 0.05) compared to the control (Figure 1C), confirming the occurrence of intravascular hemolysis.
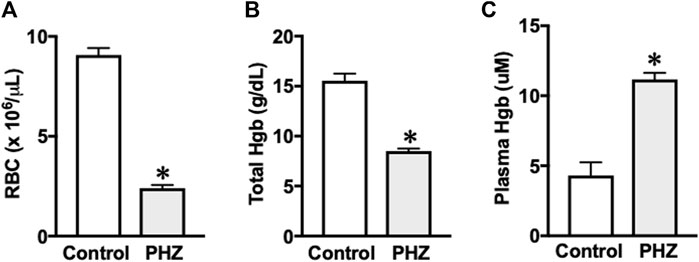
Figure 1. (A) Red blood cell, (B) hemoglobin, and (C) plasma hemoglobin. Data are shown as the mean ± SEM of 5–7 mice per group. *p < 0.05 vs. control group.
3.2 Intravascular hemolysis leads to increased urinary frequency and increased void volumes
Figure 2A presents filter paper examples from the control and PHZ-treated mice. The PHZ group showed a significant increase in urinary spots compared to the control group (p < 0.05), indicating a hyperactive voiding behavior (Figure 2B). Additionally, the total void volumes produced by the PHZ-treated mice were significantly greater than those of the control mice (p < 0.05) (Figure 2C).
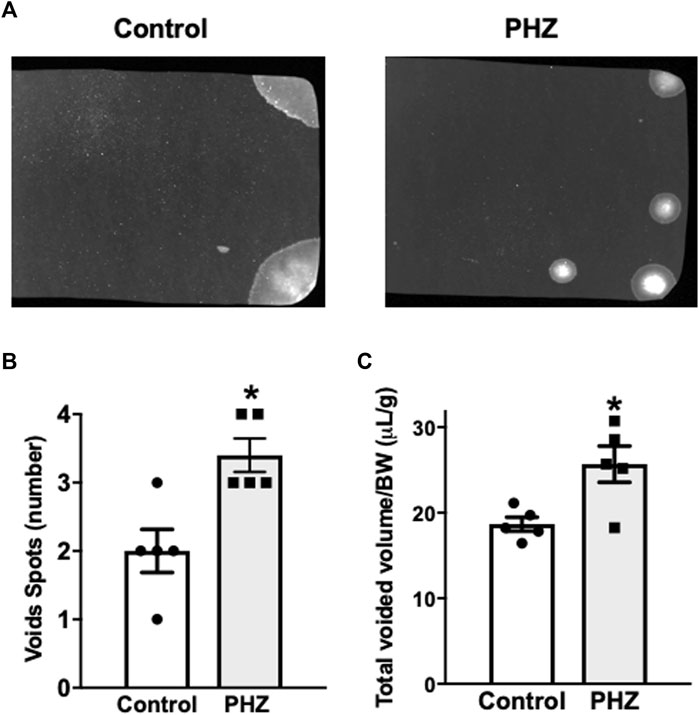
Figure 2. (A) Representative void spot assays, (B) number of spots, and (C) normalized voided volume in control and PHZ mice. Data are shown as the mean ± SEM of five mice per group. *p < 0.05 vs. control group.
3.3 Intravascular hemolysis leads to detrusor hypercontractility
EFS of 4–32 Hz induced frequency-dependent contractions in the detrusor smooth muscle in both control and PHZ-treated mice. Notably, the PHZ group exhibited significantly higher contractions at all frequencies compared to the control group (p < 0.05) (Figure 3A).
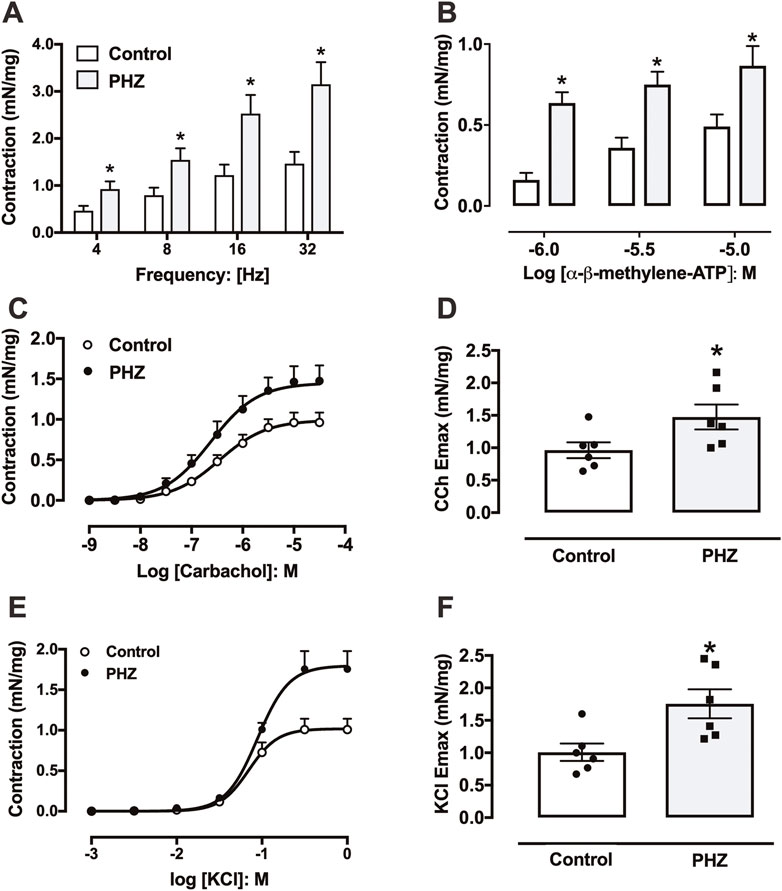
Figure 3. Contractile responses to (A) electrical field stimulation, (B) α-β-methylene-ATP, (C) carbachol, and (E) KCl in the bladder from control and PHZ mice. (D) Emax values for (D) carbachol and (F) KCl. Data are shown as the mean ± SEM of six mice per group. *p < 0.05 vs. control group.
Contractile response to α-β-methylene-ATP in the detrusor smooth muscle was assessed through non-cumulative concentration-effect curves (1 μM, 3 μM, and 10 μM) for both groups (Figure 3B). Detrusor smooth muscle from PHZ-treated mice displayed a significantly enhanced contractile response to α-β-methylene-ATP at all tested concentrations compared to the control (p < 0.05) (Figure 3B).
Contraction responses to carbachol were evaluated in detrusor smooth muscle from both PHZ and control mice through concentration-effect curves for the agonist (1 nM–30 μM) (Figure 3C). The maximal contractile response (Emax) elicited by carbachol was significantly greater in the detrusor smooth muscle of the PHZ group (p < 0.05) than that of the control group (Figure 3D), with no notable differences in potency (pEC50) between the control (6.45 ± 0.12) and PHZ-treated mice (6.62 ± 0.09). Similarly, KCl induced concentration-dependent contractions in both groups (Figure 3E). Notably, Emax to KCl was significantly greater in the PHZ group than in the control group (Figure 3F). No significant differences in potency (pEC50) for KCl were observed between the control group (1.01 ± 0.06) and the PHZ-treated group (6.62 ± 0.09).
Representative traces of responses to EFS, α-β-methylene-ATP, carbachol, and KCl are shown in Figure 4.
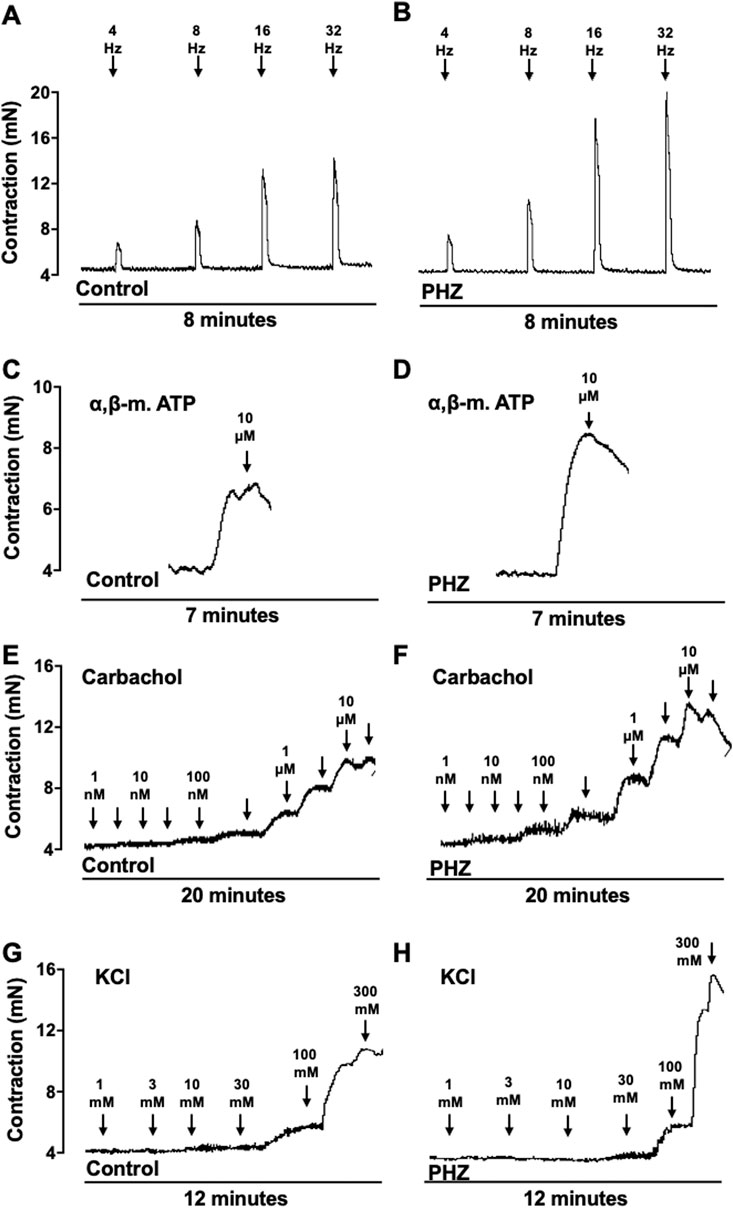
Figure 4. Representative tracings of contraction response to EFS, α-β-methylene-ATP, carbachol, and KCl from control and PHZ mice.
3.4 Intravascular hemolysis decreased protein expressions of p-eNOS (Ser-1177), p-nNOS (Ser-1417), and p-VASP (Ser-239) in the mouse bladder
Protein expression of activated (phosphorylated) forms of eNOS (p-eNOS Ser-1177), nNOS (p-nNOS Ser-1417), and VASP (p-VASP Ser-239) was investigated to understand the impact of intravascular hemolysis on signaling of nitric oxide (NO) in the bladder. These enzymes play fundamental roles in the regulation of smooth muscle tone: eNOS and nNOS are responsible for the production of NO, an important mediator of smooth muscle relaxation, while VASP is a substrate of the cGMP-protein kinase G (PKG) pathway, reflecting the activity of NO-cGMP-PKG signaling. PKG, activated by cGMP, is essential for mediating the effects of NO on smooth muscle, promoting relaxation, and directly influencing bladder function (Oelze et al., 2000; Francis et al., 2010). In the PHZ-treated mice, the activated (phosphorylated) forms of p-eNOS (Ser-1177), p-nNOS (Ser-1417), and p-VASP (Ser-239) were significantly reduced in the bladder compared to the control group (p < 0.05), as shown in Figure 5. These results suggest that intravascular hemolysis compromises NO signaling in the bladder, potentially contributing to voiding dysfunction.
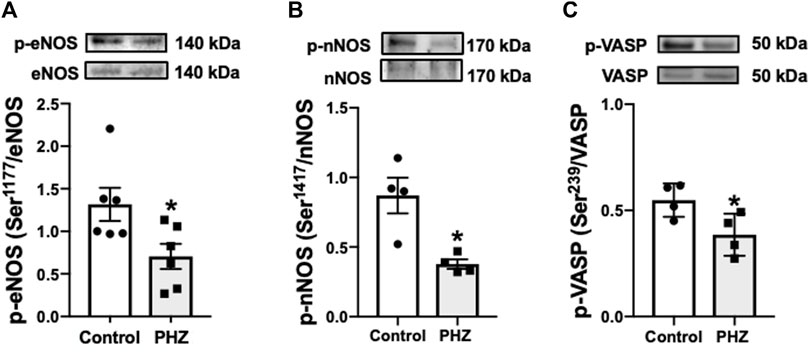
Figure 5. Representative images of Western blotting (top panels) and protein values (bottom panels) for (A) p-eNOS (ser-1177) (n = 6), (B) p-nNOS (ser-1417) (n = 4), and (C) p-VASP (Ser-239) (n = 4) in homogenates of bladder from control and PHZ mice. Data are shown as the mean ± SEM. *p < 0.05 vs. control group.
3.5 Intravascular hemolysis leads to increased oxidative stress markers in the mouse bladder
To assess the impact of intravascular hemolysis on oxidative stress in the bladder, the protein expression of oxidative stress markers, including NOX-2, 3-NT, and 4-HNE, was examined. NOX-2 is an important enzyme in ROS production, while 3-NT and 4-HNE are products of oxidative damage to proteins, serving as markers of nitrosative and oxidative stress, respectively (Pacher et al., 2007; Vermot et al., 2021). In the PHZ-treated mice, there was a significant increase in the protein expression of oxidative stress markers NOX-2, 3-NT, and 4-HNE in the bladder compared to the control group (p < 0.05), as shown in Figure 6. This increase in oxidative stress markers indicates that intravascular hemolysis promotes a pro-oxidative environment in the bladder, which may impair organ function and contribute to the development of OAB phenotypes such as those observed in SCD models.
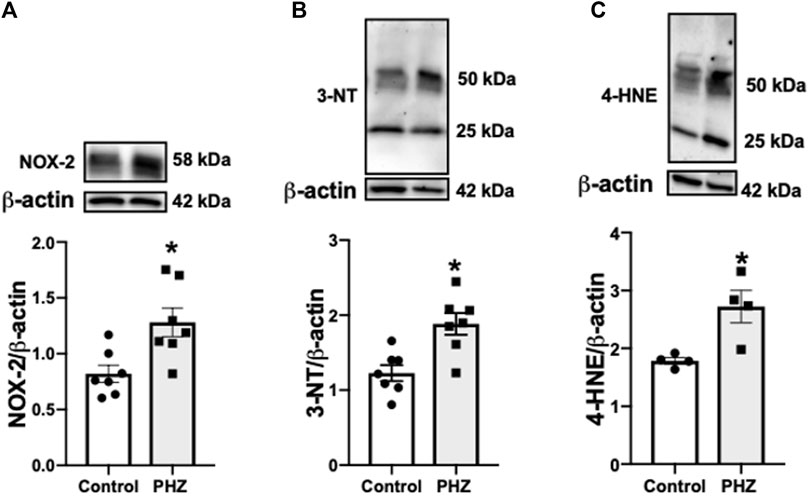
Figure 6. Representative images of Western blotting (top panels) and protein values (bottom panels) of (A) NOX-2 (n = 7), (B) 3-NT (n = 7), and (C) 4-HNE (n = 4) in homogenates of the bladder from control and PHZ mice. Data are shown as the mean ± SEM. *p < 0.05 vs. control group.
4 Discussion
In this study, PHZ-induced hemolysis in mice led to significant hematological changes, mirroring aspects of SCD. The increased urinary frequency and volume increase in PHZ-treated mice aligns with OAB symptoms, suggesting a link between intravascular hemolysis and bladder dysfunction. Notably, the enhanced contractility of the detrusor muscle in these mice indicates a direct impact of hemolysis on bladder smooth muscle activity. The decreased expression of p-eNOS (Ser-1177), nNOS (Ser-1417), and p-VASP (Ser-239) in the bladder tissue indicates a dysregulated NO signaling pathway in the impaired bladder function. Additionally, elevated oxidative stress markers in the bladders of PHZ-treated mice reinforce the role of oxidative stress in OAB phenotypes.
A central aspect of SCD is intravascular hemolysis, where red blood cell contents like hemoglobin, arginase, and other cellular components are released into the plasma (Kato et al., 2017). The PHZ-induced intravascular hemolysis model in mice is widely utilized for assessing the specific effects of intravascular hemolysis (Vannucchi et al., 2001; Henrique Silva et al., 2018; Iacopucci et al., 2022; Gotardo et al., 2023). Our study corroborates previous findings and confirms that PHZ-induced intravascular hemolysis in mice led to significant hematological changes, closely replicating the hemolytic environment seen in SCD. Free hemoglobin (HbFe2+) in the plasma or interstitial space quickly reacts with NO, leading to nitrate production and the formation of methemoglobin (HbFe3+), the oxidized form of hemoglobin (Reiter et al., 2002). This process significantly reduces NO bioavailability, contributing to tissue damage (Gladwin et al., 2012; Kato et al., 2017; Kato et al., 2018). An efficient pharmacological strategy that has been studied to limit the effects of hemoglobin involves treatment with haptoglobin. This plasma protein binds to free hemoglobin, forming a complex that is then cleared from circulation by the macrophages of the reticuloendothelial system (Buehler et al., 2020).
NO plays a crucial role in the physiology of the lower urinary tract, with its diminished bioavailability linked to micturition dysfunction. The OAB in SCD mice is associated with decreased expression of phosphorylated eNOS at its positive regulatory site Ser-1177 and phosphorylated nNOS at its positive regulatory site Ser-1412 in the bladder. Similarly, in our study, PHZ mice displayed decreased expression of p-eNOS (Ser-1177) and p-nNOS (Ser-1417), indicating lower NO production in the bladder. NO activates soluble guanylate cyclase (sGC) in smooth muscle, enhancing cGMP production. cGMP activates protein kinase G, which phosphorylates VASP at Ser-239, a reliable biomarker for monitoring the NO-stimulated cGMP-protein kinase G pathway (Oelze et al., 2000; Francis et al., 2010). In our study, protein expression for p-VASP (Ser-239) was lower in the bladder in the PHZ group, indicating decreased cGMP levels. Mice lacking nNOS exhibit bladder hypertrophy, dysfunctional urinary outlets, and increased urinary frequency (Burnett et al., 1997). Additionally, rats treated chronically with a NOS inhibitor develop an OAB phenotype (Mónica et al., 2008; Mónica et al., 2011). Altered micturition patterns have been previously reported in cGMP-dependent protein kinase I gene-deficient mice (Persson et al., 2000). PHZ-treated mice exhibited increased urinary spots and higher total void volumes. These results align with findings from animal models lacking both eNOS and nNOS, as well as SCD mice (Karakus et al., 2019; Musicki et al., 2019; Karakus et al., 2020), reinforcing the importance of NO pathways in urinary function. A prior study speculated that the augmented urine volumes observed in double-NOS (eNOS and nNOS) or triple-NOS (eNOS, nNOS, and iNOS) knockout mice could be attributed to impairments in renal function, specifically in the ability to concentrate urine, leading to polyuria (Morishita et al., 2005).
Acetylcholine, primarily through muscarinic M3 receptors, is the primary excitatory neurotransmitter in the parasympathetic nerve endings of detrusor smooth muscle (Andersson and Arner, 2004). Co-stored and co-released ATP with acetylcholine also play a significant role in nerve-mediated bladder contraction, contributing to efficient urine elimination (Burnstock, 2011). In our study, detrusor contractions induced by EFS were significantly higher in the PHZ-treated group. In parallel, the responses of detrusor smooth muscle to both muscarinic and purinergic receptor agonists (carbachol and α, β-methylene-ATP, respectively), as well as to the receptor-independent agent KCl, were also increased in PHZ-treated mice. These findings indicate that intravascular hemolysis leads to detrusor hypercontractility. The increase in detrusor muscle contraction is likely secondary to the low accumulation of cGMP in bladder tissue, a well-known secondary messenger that counteracts the contractile mechanisms of smooth muscle (Mónica and Antunes, 2018). Rats treated chronically with a non-selective inhibitor of NOS (L-NAME) show increased detrusor contraction induced by muscarinic receptor agonists (Mónica et al., 2008), as well as animal models deficient in both eNOS and nNOS (Karakus et al., 2019), highlighting the importance of the integrity of the NO pathway in bladder function.
Increased oxidative stress, characterized by elevated ROS production or reduced antioxidant capacity, is associated with the development of OAB in experimental models and participates in the pathophysiology of SCD (Alexandre et al., 2016; Silva et al., 2016; Akakpo et al., 2017; Alexandre et al., 2018; Vona et al., 2021; de Oliveira et al., 2022). NOX-2, an important NADPH oxidase isoform, catalyzes electron transfer to oxygen, generating a superoxide anion (Vermot et al., 2021). Excess superoxide reacts with NO, producing peroxynitrite, a highly toxic reactive nitrogen species (Pacher et al., 2007). Increased expression of NOX-2 has been reported in animal models with OAB (Alexandre et al., 2016; Akakpo et al., 2017; Alexandre et al., 2018; de Oliveira et al., 2022). Our study found increased NOX-2 expression in the bladder of PHZ-treated mice and elevated markers of oxidative and nitrosative stress, 4-HNE, and 3-NT. These results fit with our previous findings that demonstrate that PHZ-treated mice exhibit increased oxidative markers like 3-NT, 4-HNE, and NOX-2 in the penis (Iacopucci et al., 2022). Prior research has shown NOX-2 downregulation through NO-cGMP-dependent mechanisms (Teixeira et al., 2007). In contrast, NO inhibits NADPH oxidase-dependent superoxide anion production by a cGMP-independent mechanism without altering the protein expression of NOX-2 (Selemidis et al., 2007). In this context, this suggests that increased plasma hemoglobin may trigger oxidative stress elevation, reducing NO and cGMP bioavailability, as evidenced by reduced p-VASP (Ser-239).
In the present study, we used a PHZ-induced hemolysis model instead of transgenic mice with SCD for a few fundamental reasons. First, the PHZ-induced hemolysis model allows precise control over the onset and intensity of hemolysis, facilitating the direct correlation between hemolysis and the functional and molecular changes observed in the bladder. This precise control is important, as it allowed us to establish a direct relationship between intravascular hemolysis and the observed dysfunctions. Furthermore, this model is widely recognized for its ability to simulate key aspects of intravascular hemolysis observed in sickle cell disease, allowing specific investigation of the mechanisms underlying urinary complications. However, we recognize the value of transgenic models of SCD to study the disease in a broader context.
5 Conclusion
Our study is the first to show that intravascular hemolysis promotes voiding dysfunction correlated with alterations in the NO signaling pathway in the bladder, as evidenced by reduced levels of p-eNOS (Ser-1177), nNOS (Ser-1417), and p-VASP (Ser-239). The study also showed that intravascular hemolysis increases oxidative stress in the bladder. Our study indicates that intravascular hemolysis promotes OAB phenotypes similar to those observed in patients and mice with SCD, suggesting a potential mechanistic link. These findings suggest that pharmacologic interventions targeting intravascular hemolysis may ameliorate voiding dysfunction in SCD.
Data availability statement
The raw data supporting the conclusion of this article will be made available by the authors, without undue reservation.
Ethics statement
The animal study was approved by the Ethics Committee on Animal Use of the University of San Francisco. The study was conducted in accordance with the local legislation and institutional requirements.
Author contributions
TS: data curation, formal analysis, investigation, methodology, writing–original draft, and writing–review and editing. DlP: formal analysis, investigation, and writing–original draft. DnP: formal analysis, investigation, and writing–original draft. FBC: conceptualization and writing–review and editing. AB: conceptualization, writing–original draft, and writing–review and editing. FFC: conceptualization, writing–original draft, and writing–review and editing. FS: conceptualization, formal analysis, funding acquisition, methodology, writing–original draft, and writing–review and editing.
Funding
The author(s) declare that financial support was received for the research, authorship, and/or publication of this article. This work was supported by São Paulo Research Foundation (grant numbers: 2017/08122-9 and 2019/18886-1).
Conflict of interest
The authors declare that the research was conducted in the absence of any commercial or financial relationships that could be construed as a potential conflict of interest.
Publisher’s note
All claims expressed in this article are solely those of the authors and do not necessarily represent those of their affiliated organizations, or those of the publisher, the editors, and the reviewers. Any product that may be evaluated in this article, or claim that may be made by its manufacturer, is not guaranteed or endorsed by the publisher.
References
Akakpo W., Musicki B., Burnett A. L. (2017). cAMP-dependent regulation of RhoA/Rho-kinase attenuates detrusor overactivity in a novel mouse experimental model. BJU Int. 120, 143–151. doi:10.1111/bju.13847
Alexandre E. C., Calmasini F. B., de Oliveira M. G., Silva F. H., da Silva C. P. V., André D. M., et al. (2016). Chronic treatment with resveratrol improves overactive bladder in obese mice via antioxidant activity. Eur. J. Pharmacol. 788, 29–36. doi:10.1016/j.ejphar.2016.06.017
Alexandre E. C., Calmasini F. B., Sponton A. C. da S., de Oliveira M. G., André D. M., Silva F. H., et al. (2018). Influence of the periprostatic adipose tissue in obesity-associated mouse urethral dysfunction and oxidative stress: effect of resveratrol treatment. Eur. J. Pharmacol. 836, 25–33. doi:10.1016/j.ejphar.2018.08.010
Andersson K.-E., Arner A. (2004). Urinary bladder contraction and relaxation: physiology and pathophysiology. Physiol. Rev. 84, 935–986. doi:10.1152/physrev.00038.2003
Anele U. A., Morrison B. F., Reid M. E., Madden W., Foster S., Burnett A. L. (2015). Overactive bladder in adults with sickle cell disease. Neurourol. Urodyn. 35, 642–646. doi:10.1002/nau.22777
Buehler P. W., Humar R., Schaer D. J. (2020). Haptoglobin therapeutics and compartmentalization of cell-free hemoglobin toxicity. Trends Mol. Med. 26, 683–697. doi:10.1016/j.molmed.2020.02.004
Burnett A. L., Calvin D. C., Chamness S. L., Liu J. X., Nelson R. J., Klein S. L., et al. (1997). Urinary bladder-urethral sphincter dysfunction in mice with targeted disruption of neuronal nitric oxide synthase models idiopathic voiding disorders in humans. Nat. Med. 3, 571–574. doi:10.1038/nm0597-571
Burnstock G. (2011). Therapeutic potential of purinergic signalling for diseases of the urinary tract. BJU Int. 107, 192–204. doi:10.1111/j.1464-410X.2010.09926.x
Cita K.-C., Brureau L., Lemonne N., Billaud M., Connes P., Ferdinand S., et al. (2016). Men with sickle cell anemia and priapism exhibit increased hemolytic rate, decreased red blood cell deformability and increased red blood cell aggregate strength. PLoS ONE 11, e0154866. doi:10.1371/journal.pone.0154866
Claudino M. A., Leiria L. O. S., da Silva F. H., Alexandre E. C., Renno A., Mónica F. Z., et al. (2015). Urinary bladder dysfunction in transgenic sickle cell disease mice. PLoS ONE 10, e0133996. doi:10.1371/journal.pone.0133996
de Oliveira M. G., Monica F. Z., Passos G. R., Victorio J. A., Davel A. P., Oliveira A. L. L., et al. (2022). Selective pharmacological inhibition of NOX2 by GSK2795039 improves bladder dysfunction in cyclophosphamide-induced cystitis in mice. Antioxidants (Basel) 12, 92. doi:10.3390/antiox12010092
Dutra F. F., Alves L. S., Rodrigues D., Fernandez P. L., de Oliveira R. B., Golenbock D. T., et al. (2014). Hemolysis-induced lethality involves inflammasome activation by heme. Proc. Natl. Acad. Sci. U.S.A. 111, E4110–E4118. doi:10.1073/pnas.1405023111
Eapen R. S., Radomski S. B. (2016). Review of the epidemiology of overactive bladder. RRU 8, 71–76. doi:10.2147/RRU.S102441
Francis S. H., Busch J. L., Corbin J. D., Sibley D. (2010). cGMP-dependent protein kinases and cGMP phosphodiesterases in nitric oxide and cGMP action. Pharmacol. Rev. 62, 525–563. doi:10.1124/pr.110.002907
Gladwin M. T., Kanias T., Kim-Shapiro D. B. (2012). Hemolysis and cell-free hemoglobin drive an intrinsic mechanism for human disease. J. Clin. Investig. 122, 1205–1208. doi:10.1172/JCI62972
Gotardo É. M. F., Brito P. L., Gushiken L. F. S., Chweih H., Leonardo F. C., Costa F. F., et al. (2023). Molecular and cellular effects of in vivo chronic intravascular hemolysis and anti-inflammatory therapeutic approaches. Vasc. Pharmacol. 150, 107176. doi:10.1016/j.vph.2023.107176
Henrique Silva F., Yotsumoto Fertrin K., Costa Alexandre E., Beraldi Calmasini F., Fernanda Franco-Penteado C., Ferreira Costa F. (2018). Impairment of nitric oxide pathway by intravascular hemolysis plays a major role in mice esophageal hypercontractility: reversion by soluble guanylyl cyclase stimulator. J. Pharmacol. Exp. Ther. 367, 194–202. doi:10.1124/jpet.118.249581
Iacopucci A. P. M., da Silva Pereira P., Pereira D. A., Calmasini F. B., Pittalà V., Reis L. O., et al. (2022). Intravascular hemolysis leads to exaggerated corpus cavernosum relaxation: implication for priapism in sickle cell disease. FASEB J. 36, e22535. doi:10.1096/fj.202200867R
Karakus S., Anele U. A., Silva F. H., Musicki B., Burnett A. L. (2019). Urinary dysfunction in transgenic sickle cell mice: model of idiopathic overactive bladder syndrome. Am. J. Physiol. Ren. Physiol. 317, F540-F546–F546. doi:10.1152/ajprenal.00140.2019
Karakus S., Musicki B., Navati M. S., Friedman J. M., Davies K. P., Burnett A. L. (2020). NO-releasing nanoparticles ameliorate detrusor overactivity in transgenic sickle cell mice via restored NO/ROCK signaling. J. Pharmacol. Exp. Ther. 373, 214–219. doi:10.1124/jpet.119.264697
Kato G. J., McGowan V., Machado R. F., Little J. A., Taylor J., Morris C. R., et al. (2006). Lactate dehydrogenase as a biomarker of hemolysis-associated nitric oxide resistance, priapism, leg ulceration, pulmonary hypertension, and death in patients with sickle cell disease. Blood 107, 2279–2285. doi:10.1182/blood-2005-06-2373
Kato G. J., Piel F. B., Reid C. D., Gaston M. H., Ohene-Frempong K., Krishnamurti L., et al. (2018). Sickle cell disease. Nat. Rev. Dis. Prim. 4, 18010. doi:10.1038/nrdp.2018.10
Kato G. J., Steinberg M. H., Gladwin M. T. (2017). Intravascular hemolysis and the pathophysiology of sickle cell disease. J. Clin. Investig. 127, 750–760. doi:10.1172/JCI89741
Keil K. P., Abler L. L., Altmann H. M., Bushman W., Marker P. C., Li L., et al. (2016). Influence of animal husbandry practices on void spot assay outcomes in C57BL/6J male mice. Neurourol. Urodyn. 35, 192–198. doi:10.1002/nau.22692
Khan M. A., Dashwood M. R., Thompson C. S., Mumtaz F. H., Mikhailidis D. P., Morgan R. J. (1999). Up-regulation of endothelin (ET(A) and ET(B)) receptors and down-regulation of nitric oxide synthase in the detrusor of a rabbit model of partial bladder outlet obstruction. Urol. Res. 27, 445–453. doi:10.1007/s002400050134
Lee W.-C., Leu S., Wu K. L. H., Tain Y.-L., Chuang Y.-C., Chan J. Y. H. (2021). Tadalafil ameliorates bladder overactivity by restoring insulin-activated detrusor relaxation via the bladder mucosal IRS/PI3K/AKT/eNOS pathway in fructose-fed rats. Sci. Rep. 11, 8202. doi:10.1038/s41598-021-87505-3
Leiria L. O., Silva F. H., Davel A. P. C., Alexandre E. C., Calixto M. C., De Nucci G., et al. (2014). The soluble guanylyl cyclase activator BAY 60-2770 ameliorates overactive bladder in obese mice. J. Urol. 191, 539–547. doi:10.1016/j.juro.2013.09.020
Leiria L. O., Sollon C., Báu F. R., Mónica F. Z., D’Ancona C. L., De Nucci G., et al. (2013). Insulin relaxes bladder via PI3K/AKT/eNOS pathway activation in mucosa: unfolded protein response-dependent insulin resistance as a cause of obesity-associated overactive bladder. J. Physiol. 591, 2259–2273. doi:10.1113/jphysiol.2013.251843
Lim S. K., Kim H., Lim S. K., bin Ali A., Lim Y. K., Wang Y., et al. (1998). Increased susceptibility in Hp knockout mice during acute hemolysis. Blood 92, 1870–1877. doi:10.1182/blood.v92.6.1870
Michel M. C., Chapple C. R. (2009). Basic mechanisms of urgency: preclinical and clinical evidence. Eur. Urol. 56, 298–307. doi:10.1016/j.eururo.2009.05.028
Mónica F. Z., Antunes E. (2018). Stimulators and activators of soluble guanylate cyclase for urogenital disorders. Nat. Rev. Urol. 15, 42–54. doi:10.1038/nrurol.2017.181
Mónica F. Z., Reges R., Cohen D., Silva F. H., De Nucci G., D’Ancona C. A. L., et al. (2011). Long-term administration of BAY 41-2272 prevents bladder dysfunction in nitric oxide-deficient rats. Neurourol. Urodyn. 30, 456–460. doi:10.1002/nau.20992
Mónica F. Z. T., Bricola A. a. O., Báu F. R., Freitas L. L. L., Teixeira S. A., Muscará M. N., et al. (2008). Long-term nitric oxide deficiency causes muscarinic supersensitivity and reduces beta(3)-adrenoceptor-mediated relaxation, causing rat detrusor overactivity. Br. J. Pharmacol. 153, 1659–1668. doi:10.1038/bjp.2008.39
Morishita T., Tsutsui M., Shimokawa H., Sabanai K., Tasaki H., Suda O., et al. (2005). Nephrogenic diabetes insipidus in mice lacking all nitric oxide synthase isoforms. Proc. Natl. Acad. Sci. U. S. A. 102, 10616–10621. doi:10.1073/pnas.0502236102
Musicki B., Anele U. A., Campbell J. D., Karakus S., Shiva S., Silva F. H., et al. (2019). Dysregulated NO/PDE5 signaling in the sickle cell mouse lower urinary tract: reversal by oral nitrate therapy. Life Sci. 238, 116922. doi:10.1016/j.lfs.2019.116922
Nolan V. G., Wyszynski D. F., Farrer L. A., Steinberg M. H. (2005). Hemolysis-associated priapism in sickle cell disease. Blood 106, 3264–3267. doi:10.1182/blood-2005-04-1594
Oelze M., Mollnau H., Hoffmann N., Warnholtz A., Bodenschatz M., Smolenski A., et al. (2000). Vasodilator-stimulated phosphoprotein serine 239 phosphorylation as a sensitive monitor of defective nitric oxide/cGMP signaling and endothelial dysfunction. Circ. Res. 87, 999–1005. doi:10.1161/01.res.87.11.999
Pacher P., Beckman J. S., Liaudet L. (2007). Nitric oxide and peroxynitrite in health and disease. Physiol. Rev. 87, 315–424. doi:10.1152/physrev.00029.2006
Persson K., Pandita R. K., Aszòdi A., Ahmad M., Pfeifer A., Fässler R., et al. (2000). Functional characteristics of urinary tract smooth muscles in mice lacking cGMP protein kinase type I. Am. J. Physiol. Regul. Integr. Comp. Physiol. 279, R1112–R1120. doi:10.1152/ajpregu.2000.279.3.R1112
Portocarrero M. L., Portocarrero M. L., Sobral M. M., Lyra I., Lordêlo P., Barroso Jr. U. (2012). Prevalence of enuresis and daytime urinary incontinence in children and adolescents with sickle cell disease. J. Urology 187, 1037–1040. doi:10.1016/j.juro.2011.10.171
Reiter C. D., Wang X., Tanus-Santos J. E., Hogg N., Cannon R. O., Schechter A. N., et al. (2002). Cell-free hemoglobin limits nitric oxide bioavailability in sickle-cell disease. Nat. Med. 8, 1383–1389. doi:10.1038/nm1202-799
Selemidis S., Dusting G. J., Peshavariya H., Kemp-Harper B. K., Drummond G. R. (2007). Nitric oxide suppresses NADPH oxidase-dependent superoxide production by S-nitrosylation in human endothelial cells. Cardiovasc. Res. 75, 349–358. doi:10.1016/j.cardiores.2007.03.030
Silva F. H., Karakus S., Musicki B., Matsui H., Bivalacqua T. J., Dos Santos J. L., et al. (2016). Beneficial effect of the nitric oxide donor compound 3-(1,3-Dioxoisoindolin-2-yl)Benzyl nitrate on dysregulated phosphodiesterase 5, NADPH oxidase, and nitrosative stress in the sickle cell mouse penis: implication for priapism treatment. J. Pharmacol. Exp. Ther. 359, 230–237. doi:10.1124/jpet.116.235473
Teixeira C. E., Priviero F. B. M., Webb R. C. (2007). Effects of 5-Cyclopropyl-2-[1-(2-fluoro-benzyl)-1H-pyrazolo[3,4-b]pyridine-3-yl]pyrimidin-4-ylamine (BAY 41-2272) on smooth muscle tone, soluble guanylyl cyclase activity, and NADPH oxidase activity/expression in corpus cavernosum from wild-type, neuronal, and endothelial nitric-oxide synthase null mice. J. Pharmacol. Exp. Ther. 322, 1093–1102. doi:10.1124/jpet.107.124594
Vannucchi A. M., Bianchi L., Cellai C., Paoletti F., Carrai V., Calzolari A., et al. (2001). Accentuated response to phenylhydrazine and erythropoietin in mice genetically impaired for their GATA-1 expression (GATA-1(low) mice). Blood 97, 3040–3050. doi:10.1182/blood.v97.10.3040
Vermot A., Petit-Härtlein I., Smith S. M. E., Fieschi F. (2021). NADPH oxidases (NOX): an overview from discovery, molecular mechanisms to physiology and pathology. Antioxidants (Basel) 10, 890. doi:10.3390/antiox10060890
Keywords: cyclic guanosine monophosphate, nitric oxide, oxidative stress, NADPH oxidase, urinary dysfunction
Citation: Silveira THRe, Pereira DA, Pereira DA, Calmasini FB, Burnett AL, Costa FF and Silva FH (2024) Impact of intravascular hemolysis on functional and molecular alterations in the urinary bladder: implications for an overactive bladder in sickle cell disease. Front. Physiol. 15:1369120. doi: 10.3389/fphys.2024.1369120
Received: 11 January 2024; Accepted: 20 May 2024;
Published: 19 July 2024.
Edited by:
Russ Chess-Williams, Bond University, AustraliaReviewed by:
M. Dennis Leo, University of Tennessee Health Science Center (UTHSC), United StatesAlberto Fernando Oliveira Justo, University of São Paulo, Brazil
Copyright © 2024 Silveira, Pereira, Pereira, Calmasini, Burnett, Costa and Silva. This is an open-access article distributed under the terms of the Creative Commons Attribution License (CC BY). The use, distribution or reproduction in other forums is permitted, provided the original author(s) and the copyright owner(s) are credited and that the original publication in this journal is cited, in accordance with accepted academic practice. No use, distribution or reproduction is permitted which does not comply with these terms.
*Correspondence: Fábio Henrique Silva, ZmFiaW8uaHNpbHZhQHVzZi5lZHUuYnI=, ZmFiaW9oc2lsdmE4N0BnbWFpbC5jb20=