- 1College of Sports and Health, Shandong Sport University, Jinan, China
- 2College of Sports Human Sciences, Beijing Sport University, Beijing, China
Purpose: The correlations of postural stability with proprioception and strength may explain the recurrent sprains among individuals with functional ankle instability (FAI). This study aimed to compare anterior-posterior (AP) and medial-lateral (ML) postural stability, along with ankle proprioception and strength between individuals with and without FAI and investigated their correlations.
Methods: Forty participants with FAI and another 40 without FAI were recruited. Their postural stability, represented by time to stabilization (TTS) in the AP (TTSAP) and ML (TTSML) directions, was calculated by the ground reaction force during jumping onto a force plate. Their ankle proprioception and strength during plantarflexion/dorsiflexion and inversion/eversion were measured using a proprioception device and a strength testing system, separately.
Results: Individuals with FAI had longer TTSAP (p = 0.015) and TTSML (p = 0.006), larger ankle proprioception thresholds (p = 0.000–0.001), and less strength (p = 0.001–0.017) than those without FAI. Correlations between strength and TTSAP were detected among individuals with (ankle plantarflexion, r = −0.409, p = 0.009) and without FAI (ankle plantarflexion, r = −0.348, p = 0.028; ankle dorsiflexion, r = −0.473, p = 0.002). Correlations of proprioception (ankle inversion, r = 0.327, p = 0.040; ankle eversion, r = 0.354, p = 0.025) and strength (ankle eversion, r = −0.479, p = 0.002) with TTSML were detected among individuals without FAI but not among those with FAI.
Conclusion: Individuals with FAI have worse postural stability and proprioception and less strength. Their proprioception and strength decreased to a point where they could not provide sufficient functional assistance to the ML postural stability. Improvements in proprioception and strength may be keys to prevent recurrent ankle sprains among individuals with FAI.
1 Introduction
Ankle sprain has the highest recurrence rate among all lower-extremity musculoskeletal injuries (Fong et al., 2007; Kerr et al., 2022), with an annual economic burden of approximately $6.2 billion in the United States (Gribble et al., 2016). Following an initial sprain, 40% of individuals develop functional ankle instability (FAI), characterized by recurrent sprains, sensorimotor impairments, experiences of “giving way,” and feelings of instability at the ankle (Arnold et al., 2009; Herzog et al., 2019; Huang et al., 2021).
Postural stability deficit is one of the strongest risk factors for ankle sprain (Hertel and Corbett, 2019). Owing to the biomechanical contribution of the ankle joint on jump performance (Giustino et al., 2022), jump landing test is one of the most common functional tests to assess postural stability in the anterior-posterior (AP) and medial-lateral (ML) directions among individuals with FAI (Ross et al., 2009). Postural stability during jump landing is usually measured in terms of time to stabilization (TTS) in the AP (TTSAP) and ML (TTSML) directions, which represents the time needed to stabilize the body (McCann et al., 2018).
Maintaining postural stability involves the integration of sensory input with information from the musculoskeletal systems (Grassi et al., 2018). Proprioception and strength are two crucial contributors in maintaining postural stability and preventing sprains during sports and daily activities. Proprioceptive receptors transmit information about the position of limbs and body segments, as well as the velocity and direction of their movements, to the central nervous system to guarantee smooth and coordinated body movements during balance control (Xue et al., 2021). Sufficient torque generated by lower limb muscles ensures rapid adjustments when countered with unexpected disturbances (Park et al., 2019; Khalaj et al., 2021).
Previous studies investigated the correlations of postural stability with proprioception (Santos and Liu, 2008) and muscle strength (McCann et al., 2018). However, they did not differentiate postural stability in the AP and ML directions, and most of them focused only on individuals with FAI but not those without FAI. Humans adopt different mechanisms to maintain AP and ML postural stability (O'Connor and Kuo, 2009). Compared with AP postural stability, ML postural stability is harder to maintain and depends on motor control through active foot placement and integrated sensory feedback, such as proprioception (O'Connor and Kuo, 2009; Rankin et al., 2014). The majority of ankle sprains occur in the ML direction (primarily during ankle inversion) (Herzog et al., 2019), making it particularly important to differentiate between AP and ML postural stability. To the best of our knowledge, no studies have investigated the correlations of proprioception and strength with the AP and ML postural stability among individuals with and without FAI. Distinguishing AP and ML postural stability and investigating their correlations with proprioception and strength among individuals with and without FAI can help deepen our understanding of the causes of postural stability deficits and recurrent ankle sprains and provide guidance for the rehabilitation and prevention of ankle sprains.
This study aimed to compare AP and ML postural stability, proprioception, and strength between individuals with and without FAI and investigate the correlations of proprioception and strength with AP and ML postural stability. Addressing these issues can help select targeted interventions to enhance postural stability and develop precise ankle sprain prevention approaches among individuals with FAI. Our hypothesis are as follows: 1. Individuals with FAI have worse AP and ML postural stability, impaired proprioception, and less strength than those without FAI; 2. AP and ML postural stability are significantly correlated with proprioception and strength among individuals with and without FAI.
2 Material and methods
2.1 Design
This study has a cross-sectional design. Before participation, all participants read and signed informed consent approved by the Institutional Review Boards of Shandong Sport University (2022001).
2.2 Participants
The sample size was estimated using G*Power software (Version 3.1) based on two studies: one detected an effect size = 0.80 in TTS between people with and without FAI (with = 1.86 ± 0.67s, without = 1.44 ± 0.33s) (Ross et al., 2009), and another detected an r2 = 0.25 between muscle strength and postural stability (McCann et al., 2017). A minimum of 52 and 66 participants must be recruited to guarantee an α of 0.05 and statistical power of 0.80.
Participants were recruited from a local university from May to July 2023 through e-newsletters, e− and paper notifications, and e-mail. After the purpose and process of the study were introduced to the participants, 124 people were willing to enroll in the study, of whom 58 had self-reported FAI symptoms and 66 had no FAI symptoms. The eligible participants were screened according to the inclusion and exclusion criteria of the study. Inclusion criteria for participants with FAI were as follows: (1) at least one severe ankle sprain resulting in pain, swelling, and activity limitation for at least 1 day within at least 12 months before the start of this study; (2) age 18–25 years; (3) more than two times of ankle “giving way,” which refers to the feeling of uncontrollable or unpredictable excessive inversion of the ankle (Delahunt et al., 2010; Gribble et al., 2014), in the past 6 months; (4) persistent ankle instability and dysfunction during daily activities, which refers to the feeling of ankle instability during daily living and sporting activities (Delahunt et al., 2010) or the experience of difficulty in certain daily activities such as putting on shoes, getting in and out of a car, due to pain or joint instability (Lentell et al., 1995); (5) with a score ≤24 on the Cumberland Ankle Instability Tool (CAIT) (Gribble et al., 2014). Inclusion criteria for participants without FAI were as follows: (1) no previous ankle sprain/injury and no giving way or instability and (2) CAIT score ≥28. Exclusion criteria for all participants were as follows: (1) mechanical instability indicated by positive findings of talar tilt and anterior drawer test; (2) a fracture or surgical procedure to the lower extremity; (3) acute injury of the lower extremity within the last 3 months; (4) neurological disease, diabetes, and vestibular disorders; (5) bilateral FAI.
Following eligibility assessment, 40 individuals with FAI and another 40 without FAI were recruited. Their age, height, body mass, CAIT score, and ankle sprain and giving way experience are shown in Table 1. The number of left-dominant and right-dominant individuals (2 left and 38 right) was equal in each group. The dominant limb was defined as the limb each participant used during kicking a ball (Mitrousis et al., 2023).
2.3 Protocol
The CAIT scores, history of ankle sprain, and experience of giving way were recorded before the tests. Postural stability and proprioception were measured in a random order. Strength was assessed lastly to avoid fatigue. The individuals with FAI were tested on their affected limbs, including 26 dominant and 14 nondominant limbs. Meanwhile, 26 dominant and 14 nondominant limbs were tested among the individuals without FAI.
All participants’ CAIT scores were assessed using a paper-based questionnaire by a qualified physiotherapist with 6 years of clinical experience. The remaining three tests were conducted by three testers. For consistency, each test was conducted by the same tester. All data were collected in the Lab of Biomechanics at Shandong Sport University from May to July 2023.
2.4 Postural stability test
Postural stability was assessed by a jump landing test. The participants stood 70 cm away from the center of a force plate (AMTI, AMTI Inc., Watertown, MA, US) (Figure 1A ⅰ) and jumped forward and upward with both legs and landed onto the force plate (Gribble et al., 2010). During the jump, the participants touched the target on the vertical jump tester to ensure a jump height (Figure 1A ⅱ) and landed on their tested limbs. After landing, the participants maintained stability for 5 s (Figure 1A ⅲ). This test demonstrated good reliability (Fransz et al., 2016). Three trials were recorded, with a minimum interval of 60 s in between.
Jump height was determined by a vertical jump test before the jump landing test. Each participant stood with both feet next to a vertical jump tester (Guangzhou Gaia Sports Goods Co., ltd, China) and stretched his/her upper limbs upward as high as possible. Their standing stretching height (H1) was measured. He/she jumped as high as possible to touch a high point (H2). The jump height was calculated using the following formula:
2.5 Proprioception test
Proprioception was tested using a proprioception device (Sunny, Jinan, China) (Figure 1B) and indicated by the ankle passive detection sense, which has shown good test–retest reliability (ICC = 0.74–0.94) (Sun et al., 2015). This device comprises an operating platform and two pedals. The participants sat in a height-adjustable chair with both feet positioned on the testing pedal, hips and knees flexed at a 90° angle, and ankles in a neutral position. The participants wore eye masks and noise-canceling headphones throughout the tests to minimize distractions. During each test, one of the pedals rotated at an angular velocity of 0.4°/s, inducing ankle plantarflexion/dorsiflexion or inversion/eversion. As soon as the passive motion was perceived, the participants immediately pressed a hand-held switch to stop the pedal (Song et al., 2021). The proprioception threshold was defined as the angle of pedal rotation when the passive motion was perceived. Three trials were recorded in each direction, with a minimum interval of 60 s in between.
2.6 Strength test
Isokinetic strength during ankle plantarflexion/dorsiflexion and inversion/eversion was measured at an angular velocity of 60°/s by a strength testing system (IsoMed 2000; D. and R. Ferstl GmbH, Hemau, Germany) (Figure 1C), which showed good test–retest reliability (Gonosova et al., 2018). During the plantarflexion/dorsiflexion tests, the participants lay supine with their thighs, buttocks, and torsos fixed to the chair, lateral malleolus of the tested limbs aligned with the axis of rotation of the dynamometer arm, and started at 15° of dorsiflexion and ended at 35° of plantarflexion. During the inversion/eversion tests, the participants lay semiprone with the seat reclining at 45°, hips, knees, and ankles of the tested limbs flexed at 80°, 110°, and 10°, and started at 25° of ankle eversion and ended at 20° of ankle inversion. All the tests were conducted with consistent verbal commands and encouragement provided to all the participants. Three trials were recorded in each direction, with a minimum interval of 120 s in between.
2.7 Data processing
In the postural stability test, the ground reaction force (GRF) in AP and ML directions was recorded at 1000 Hz and then filtered using a fourth-order low-pass Butterworth filter with a cut-off frequency of 12 Hz (McCann et al., 2018). The filtered data, obtained from the time of landing (>10 N) to 5 s post-landing, were used to calculate TTS calculation through sequential average as follows (Fransz et al., 2015):
where Fx and Fy are the GRF in the AP and ML directions. TTS was defined as the time from landing to the sequential average of each component reached and remained within one-fourth of the standard deviation of the overall average (Fransz et al., 2016). In the proprioception and strength tests, the mean values in each direction of three trials were used for statistical analysis. In the strength test, the joint torques were normalized by body mass.
2.8 Statistics
Shapiro-Wilk tests were used to assess the normality of data distribution. Descriptive analysis was applied to examine outcome variables’ mean and standard deviations. Independent t-tests (normal distribution) or Mann–Whitney U tests (nonnormal distribution) were utilized to compare differences between individuals with and without FAI. Effect sizes (Cohen’s d for normal distribution, ɳ2 for nonnormal distribution) were used to evaluate the magnitude of between-group differences. Pearson (normal distribution) or Spearman correlations (nonnormal distribution) were adopted to determine the correlations of proprioception and strength to TTS while controlling for covariates (gender, age, height, and weight). Separate exploratory factor analysis was then carried out among each category of the variables of interest. Multivariable linear regression was used to explore the degree of correlation between each generated factor and TTS while controlling for the above-mentioned covariates. The thresholds for Cohen’s d were as follows: <0.20, trivial; 0.21–0.50, small; 0.51–0.80, medium; and >0.81, large. The thresholds for ɳ2 were as follows: 0.01–0.059, small; 0.06–0.14, medium; and >0.14, large. The thresholds for correlation coefficient were as follows: 0–0.1 (trivial), >0.1–0.3 (weak), >0.3–0.5 (moderate), and >0.5 (strong) (Cohen, 1988). All analyses were conducted in SPSS Version 26.0 (IBM; Armonk, NY, United States).
3 Results
Shapiro-Wilk test results showed the nonnormal distribution of all proprioception variables and strength during ankle dorsiflexion. The descriptive characteristics of the TTS are shown in Figure 2. Individuals with FAI had longer TTSAP and TTSML than those without FAI.
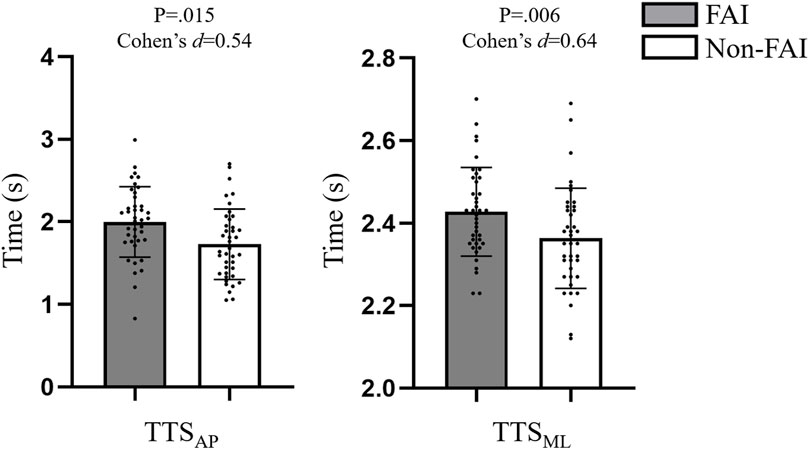
FIGURE 2. Comparisons of TTS among individuals with and without FAI. TTS: time to stabilization; AP: anterior-posterior direction; ML: medial-lateral direction.
The descriptive characteristics of proprioception and strength are shown in Table 2. Compared with individuals without FAI, those with FAI had larger proprioception thresholds and less strength during ankle plantarflexion/dorsiflexion and inversion/eversion.
The correlations of proprioception and strength to TTS are shown in Table 3. Among the individuals with FAI, the strength during ankle plantarflexion was moderately correlated with TTSAP. Among the individuals without FAI, the strength during ankle plantarflexion/dorsiflexion was moderately correlated with TTSAP, the proprioception during ankle inversion/eversion was moderately correlated with TTSML, and the strengths during ankle eversion were moderately correlated with TTSML.
The factor loadings for all the variables of proprioception and strength are shown in Table 4. Factor 1 (F1), factor 2 (F2), factor 3 (F3), and factor 4 (F4) were the summaries of proprioception during ankle plantarflexion/dorsiflexion and inversion/eversion and strength during ankle plantarflexion/dorsiflexion and inversion/eversion, with a Kaiser Meyer Olkin value of 0.760 and sphericity of <0.001.
The equation for multivariable regression among individuals with FAI is:
In Eq. 4, adjusted r2 = 0.414, pF3 = 0.014, and βF3 = 0.388.
The equations for multivariable regression among individuals without FAI are:
In Eq. 5, adjusted r2 = 0.432, pF3 = 0.008, and βF3 = 0.420.
In Eq. 6, adjusted r2 = 0.458, pF2 = 0.040, pF4 = 0.035, βF2 = 0.313, and βF4 = 0.322.
4 Discussion
This study compared postural stability, proprioception, and strength between individuals with and without FAI and investigated the correlations of proprioception and strength with AP and ML postural stability. The results partially approved hypothesis # 1 and rejected hypothesis # 2. The individuals with FAI exhibited worse postural stability and proprioception and less strength than those without FAI. Both groups demonstrated the correlations of strength with AP postural stability. The individuals without FAI demonstrated the correlations of proprioception and strength with ML postural stability, but no such correlations were detected among those with FAI.
The results demonstrated that the individuals with FAI exhibited worse postural stability and proprioception and less strength than those without FAI. The impaired postural stability among individuals with FAI aligns with previous studies (Ross et al., 2009), the reason may line in the proprioception and neuromuscular control deficits after recurrent sprains (Hertel and Corbett, 2019). Several studies (Sousa et al., 2017; Xue et al., 2021) consistently reported that individuals with FAI demonstrated worse proprioception than those without FAI. This condition may be caused by the damaged proprioceptors, such as muscle spindles, Pacinian corpuscles, and Ruffini corpuscles, due to recurrent ankle sprains (Xue et al., 2021). The finding that individuals with FAI have less strength was consistent with a previous study (Arnold et al., 2009), which stated that this condition is a result of damage or atrophy of the muscles around the ankle (Khalaj et al., 2020). Decreased proprioception may also be a potential cause of decreased muscle strength. When proprioception has deteriorated, the muscles are unable to receive accurate feedback, leading to a decrease in the precision of muscle control around the ankle, which in turn affects muscle strength (Hertel and Corbett, 2019).
Our results showed the correlations between strength and AP postural stability among individuals with and without FAI. The ankle dorsiflexors and plantarflexors could control the backward and forward movements of the body to prevent the center of mass from moving out of the posterior and anterior edges of the support base (Svoboda et al., 2019), thus helping maintain and restore AP postural stability during movements. In addition, the contraction of dorsiflexors and plantarflexors contributes to the dynamic stability at the ankle and the postural stability of the body and reduces the impact of GRF on the body (Park et al., 2019; Khalaj et al., 2021). Although their strength decreased due to recurrent ankle sprains, both groups still demonstrated the correlations between strength and AP postural stability, suggesting that strength continues to play a crucial role in AP postural stability among individuals with FAI.
One interesting finding of this study is that proprioception and strength contributed to ML postural stability among individuals without FAI but not among those with FAI. The CNS utilizes proprioceptive feedback to estimate the position of the lower limbs and then activates the appropriate muscles to facilitate rapid lateral adjustments to maintain ML postural stability (Rankin et al., 2014). Compared with those without FAI, the weaker correlation among individuals with FAI infers that they may rely less on their deteriorated proprioception and decreased strength to maintain ML postural stability. Recurrent ankle sprains could disrupt proprioception receptors and lead to muscle atrophy, which affects the perception of ankle proprioception and the development of joint force and leads to impaired neuromuscular control during joint movement (Hertel and Corbett, 2019; Hopkins et al., 2019). It can be inferred that once proprioception and strength deteriorated to a certain point, they could not provide meaningful functional assistance to the ML postural stability. Given that most ankle sprains occur in the ML direction (mostly during ankle inversion) (Herzog et al., 2019), the worse proprioception and less strength might reasonably explain the increased risk of ankle sprains among individuals with FAI. On the basis of the above inference and the confirmation of the proprioception and strength decline among individuals with FAI, it is reasonable to assume that the rehabilitation of proprioception and strength could be keys to prevent recurrent ankle sprains among individuals with FAI.
This study has several limitations. First, some potential factors, such as plantar tactile sensation, were not measured. Nevertheless, tactile sensation was transmitted by small diameter type III sensory neurons, which are slower and weaker than other sensory neurons (Li et al., 2019), so the effect of tactile sensation is limited during dynamic tasks. Second, as a cross-sectional study, the causal relationships between proprioception and strength to postural stability cannot be explained. Longitudinal studies could help deepen our understanding of how postural stability is affected by proprioception and strength.
5 Conclusion
Individuals with FAI have worse postural stability and proprioception and less strength than those without FAI. Their proprioception and strength decreased to a point where they could not provide sufficient functional assistance to the ML postural stability. Improvements in proprioception and strength may be keys to prevent recurrent ankle sprains among individuals with FAI.
Data availability statement
The original contributions presented in the study are included in the article/Supplementary material, further inquiries can be directed to the corresponding authors.
Ethics statement
The studies involving humans were approved by the Institutional Review Boards of Shandong Sport University. The studies were conducted in accordance with the local legislation and institutional requirements. The participants provided their written informed consent to participate in this study.
Author contributions
YL: Methodology, Data curation, Formal Analysis, Investigation, Writing–original draft. SD: Formal Analysis, Methodology, Writing–review and editing. QW: Formal Analysis, Methodology, Writing–review and editing. ZL: Methodology, Writing–review and editing. QS: Writing–review and editing, Conceptualization, Formal Analysis, Funding acquisition, Supervision. PS: Conceptualization, Supervision, Writing–review and editing, Methodology, Project administration.
Funding
The author(s) declare financial support was received for the research, authorship, and/or publication of this article. This research was supported by the General Administration of Sport of China (23QN009) and the National Natural Science Foundation of China (12102235).
Acknowledgments
We thank Miss Shanshan Hu, Miss Lin Zhu, Miss Yushan Miao, and Mr He Gao, graduate students at Shandong Sport University, for their assistance in data collection of this work.
Conflict of interest
The authors declare that the research was conducted in the absence of any commercial or financial relationships that could be construed as a potential conflict of interest.
Publisher’s note
All claims expressed in this article are solely those of the authors and do not necessarily represent those of their affiliated organizations, or those of the publisher, the editors and the reviewers. Any product that may be evaluated in this article, or claim that may be made by its manufacturer, is not guaranteed or endorsed by the publisher.
References
Arnold B. L., Linens S. W., de la Motte S. J., Ross S. E. (2009). Concentric evertor strength differences and functional ankle instability: a meta-analysis. J. Athl. Train. 44 (6), 653–662. doi:10.4085/1062-6050-44.6.653
Cohen J. (1988). Statistical power analysis for the behavioral sciences. Hillsdale, NJ, USA.: L. Erlbaum Associates.
Delahunt E., Coughlan G. F., Caulfield B., Nightingale E. J., Lin C. W., Hiller C. E. (2010). Inclusion criteria when investigating insufficiencies in chronic ankle instability. Med. Sci. Sports Exerc 42 (11), 2106–2121. doi:10.1249/MSS.0b013e3181de7a8a
Fong D. T., Hong Y., Chan L. K., Yung P. S., Chan K. M. (2007). A systematic review on ankle injury and ankle sprain in sports. Sports Med. 37 (1), 73–94. doi:10.2165/00007256-200737010-00006
Fransz D. P., Huurnink A., de Boode V. A., Kingma I., van Dieën J. H. (2015). Time to stabilization in single leg drop jump landings: an examination of calculation methods and assessment of differences in sample rate, filter settings and trial length on outcome values. Gait Posture 41 (1), 63–69. doi:10.1016/j.gaitpost.2014.08.018
Fransz D. P., Huurnink A., de Boode V. A., Kingma I., van Dieën J. H. (2016). The effect of the stability threshold on time to stabilization and its reliability following a single leg drop jump landing. J. Biomech. 49 (3), 496–501. doi:10.1016/j.jbiomech.2015.12.048
Giustino V., Messina G., Patti A., Padua E., Zangla D., Drid P., et al. (2022). Effects of a postural exercise program on vertical jump height in young female volleyball players with knee valgus. Int. J. Environ. Res. Public Health 19 (7), 3953. doi:10.3390/ijerph19073953
Gonosova Z., Linduska P., Bizovska L., Svoboda Z. (2018). Reliability of Ankle⁻Foot complex isokinetic strength assessment using the isomed 2000 dynamometer. Med. Kaunas. 54 (3), 43. doi:10.3390/medicina54030043
Grassi A., Alexiou K., Amendola A., Moorman C. T., Samuelsson K., Ayeni O. R., et al. (2018). Postural stability deficit could predict ankle sprains: a systematic review. Knee Surg. Sports Traumatol. Arthrosc. 26 (10), 3140–3155. doi:10.1007/s00167-017-4818-x
Gribble P. A., Bleakley C. M., Caulfield B. M., Docherty C. L., Fourchet F., Fong D. T., et al. (2016). Evidence review for the 2016 International Ankle Consortium consensus statement on the prevalence, impact and long-term consequences of lateral ankle sprains. Br. J. Sports Med. 50 (24), 1496–1505. doi:10.1136/bjsports-2016-096189
Gribble P. A., Delahunt E., Bleakley C. M., Caulfield B., Docherty C. L., Fong D. T., et al. (2014). Selection criteria for patients with chronic ankle instability in controlled research: a position statement of the International Ankle Consortium. J. Athl. Train. 49 (1), 121–127. doi:10.4085/1062-6050-49.1.14
Gribble P. A., Taylor B. L., Shinohara J. (2010). Bracing does not improve dynamic stability in chronic ankle instability subjects. Phys. Ther. Sport 11 (1), 3–7. doi:10.1016/j.ptsp.2009.11.003
Hertel J., Corbett R. O. (2019). An updated model of chronic ankle instability. J. Athl. Train. 54 (6), 572–588. doi:10.4085/1062-6050-344-18
Herzog M. M., Kerr Z. Y., Marshall S. W., Wikstrom E. A. (2019). Epidemiology of ankle sprains and chronic ankle instability. J. Athl. Train. 54 (6), 603–610. doi:10.4085/1062-6050-447-17
Hopkins J. T., Son S. J., Kim H., Page G., Seeley M. K. (2019). Characterization of multiple movement strategies in participants with chronic ankle instability. J. Athl. Train. 54 (6), 698–707. doi:10.4085/1062-6050-480-17
Huang P. Y., Jankaew A., Lin C. F. (2021). Effects of plyometric and balance training on neuromuscular control of recreational athletes with functional ankle instability: a randomized controlled laboratory study. Int. J. Environ. Res. Public Health 18 (10), 5269. doi:10.3390/ijerph18105269
Kerr Z. Y., Nedimyer A. K., Simon J. E., Kossman M. K., Corbett R. O., Chandran A. (2022). The epidemiology of ankle sprains in US high school sports, 2011-2012 to 2018-2019 academic years. J. Athl. Train. 57 (11-12), 1030–1038. doi:10.4085/1062-6050-0664.21
Khalaj N., Vicenzino B., Heales L. J., Smith M. D. (2020). Is chronic ankle instability associated with impaired muscle strength? Ankle, knee and hip muscle strength in individuals with chronic ankle instability: a systematic review with meta-analysis. Br. J. Sports Med. 54 (14), 839–847. doi:10.1136/bjsports-2018-100070
Khalaj N., Vicenzino B., Smith M. D. (2021). Hip and knee muscle torque and its relationship with dynamic balance in chronic ankle instability, copers and controls. J. Sci. Med. Sport 24 (7), 647–652. doi:10.1016/j.jsams.2021.01.009
Lentell G., Baas B., Lopez D., McGuire L., Sarrels M., Snyder P. (1995). The contributions of proprioceptive deficits, muscle function, and anatomic laxity to functional instability of the ankle. J. Orthop. Sports Phys. Ther. 21 (4), 206–215. doi:10.2519/jospt.1995.21.4.206
Li L., Zhang S., Dobson J. (2019). The contribution of small and large sensory afferents to postural control in patients with peripheral neuropathy. J. Sport Health Sci. 8 (3), 218–227. doi:10.1016/j.jshs.2018.09.010
McCann R. S., Bolding B. A., Terada M., Kosik K. B., Crossett I. D., Gribble P. A. (2018). Isometric hip strength and dynamic stability of individuals with chronic ankle instability. J. Athl. Train. 53 (7), 672–678. doi:10.4085/1062-6050-238-17
McCann R. S., Crossett I. D., Terada M., Kosik K. B., Bolding B. A., Gribble P. A. (2017). Hip strength and star excursion balance test deficits of patients with chronic ankle instability. J. Sci. Med. Sport 20 (11), 992–996. doi:10.1016/j.jsams.2017.05.005
Mitrousis I., Bourdas D. I., Kounalakis S., Bekris E., Mitrotasios M., Kostopoulos Ν., et al. (2023). The effect of a balance training program on the balance and technical skills of adolescent soccer players. J. Sports Sci. Med. 22 (4), 645–657. doi:10.52082/jssm.2023.645
O'Connor S. M., Kuo A. D. (2009). Direction-dependent control of balance during walking and standing. J. Neurophysiol. 102 (3), 1411–1419. doi:10.1152/jn.00131.2009
Park Y. H., Park S. H., Kim S. H., Choi G. W., Kim H. J. (2019). Relationship between isokinetic muscle strength and functional tests in chronic ankle instability. J. Foot Ankle Surg. 58 (6), 1187–1191. doi:10.1053/j.jfas.2019.04.005
Rankin B. L., Buffo S. K., Dean J. C. (2014). A neuromechanical strategy for mediolateral foot placement in walking humans. J. Neurophysiol. 112 (2), 374–383. doi:10.1152/jn.00138.2014
Ross S. E., Guskiewicz K. M., Gross M. T., Yu B. (2009). Balance measures for discriminating between functionally unstable and stable ankles. Med. Sci. Sports Exerc 41 (2), 399–407. doi:10.1249/MSS.0b013e3181872d89
Santos M. J., Liu W. (2008). Possible factors related to functional ankle instability. J. Orthop. Sports Phys. Ther. 38 (3), 150–157. doi:10.2519/jospt.2008.2524
Song Q., Zhang X., Mao M., Sun W., Zhang C., Chen Y., et al. (2021). Relationship of proprioception, cutaneous sensitivity, and muscle strength with the balance control among older adults. J. Sport Health Sci. 10 (5), 585–593. doi:10.1016/j.jshs.2021.07.005
Sousa A. S. P., Leite J., Costa B., Santos R. (2017). Bilateral proprioceptive evaluation in individuals with unilateral chronic ankle instability. J. Athl. Train. 52 (4), 360–367. doi:10.4085/1062-6050-52.2.08
Sun W., Song Q., Yu B., Zhang C., Mao D. (2015). Test-retest reliability of a new device for assessing ankle joint threshold to detect passive movement in healthy adults. J. Sports Sci. 33 (16), 1667–1674. doi:10.1080/02640414.2014.1003589
Svoboda Z., Bizovska L., Gonosova Z., Linduska P., Kovacikova Z., Vuillerme N. (2019). Effect of aging on the association between ankle muscle strength and the control of bipedal stance. PLoS One 14 (10), doi:10.1371/journal.pone.0223434
Keywords: ankle sprain, functional ankle instability, postural control, kinesthesia, jump landing
Citation: Liu Y, Dong S, Wang Q, Liu Z, Song Q and Shen P (2024) Deficits in proprioception and strength may contribute to the impaired postural stability among individuals with functional ankle instability. Front. Physiol. 15:1342636. doi: 10.3389/fphys.2024.1342636
Received: 22 November 2023; Accepted: 12 February 2024;
Published: 01 March 2024.
Edited by:
Qichang Mei, Ningbo University, ChinaCopyright © 2024 Liu, Dong, Wang, Liu, Song and Shen. This is an open-access article distributed under the terms of the Creative Commons Attribution License (CC BY). The use, distribution or reproduction in other forums is permitted, provided the original author(s) and the copyright owner(s) are credited and that the original publication in this journal is cited, in accordance with accepted academic practice. No use, distribution or reproduction is permitted which does not comply with these terms.
*Correspondence: Qipeng Song, songqipeng@sdpei.edu.cn; Peixin Shen, 18323022054@163.com