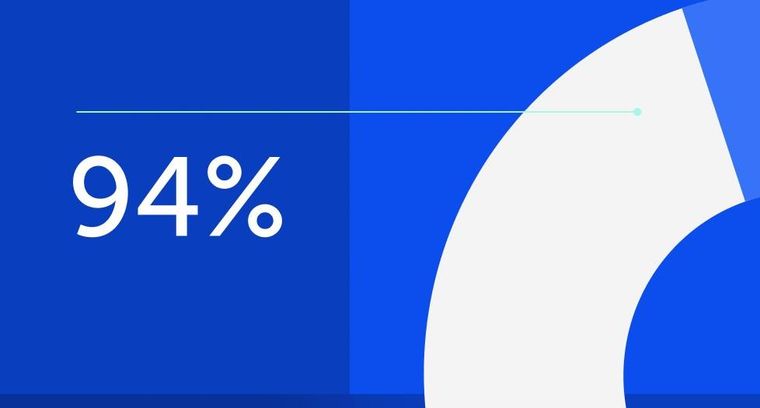
94% of researchers rate our articles as excellent or good
Learn more about the work of our research integrity team to safeguard the quality of each article we publish.
Find out more
ORIGINAL RESEARCH article
Front. Physiol., 09 May 2024
Sec. Integrative Physiology
Volume 15 - 2024 | https://doi.org/10.3389/fphys.2024.1334874
This article is part of the Research TopicIntegrative Physiological Approaches to Understand High Altitude AdaptationView all 6 articles
Background: High-altitude populations exhibit distinct cellular, respiratory, and cardiovascular phenotypes, some of which provide adaptive advantages to hypoxic conditions compared to populations with sea-level ancestry. Studies performed in populations with a history of high-altitude residence, such as Tibetans, support the idea that many of these phenotypes may be shaped by genomic features that have been positively selected for throughout generations. We hypothesize that such traits observed in Tibetans at high altitude also occur in Tibetans living at intermediate altitude, even in the absence of severe sustained hypoxia.
Methodology: We studied individuals of high-altitude ancestry (Tibetans, n = 17 females; n = 12 males) and sea-level ancestry (Han Chinese, n = 6 females; n = 10 males), both who had been living at ∼1300 m (∼4327 ft) for at least 18 months. We measured hemoglobin concentration ([Hb]), hypoxic ventilatory response (HVR), and hypoxic heart rate response (HHRR) with end-tidal CO2 (PetCO2) held constant (isocapnia) or allowed to decrease with hypoxic hyperventilation (poikilocapnia). We also quantified the contribution of CO2 on ventilation and heart rate by calculating the differences of isocapnic versus poikilocapnic hypoxic conditions (Δ
Results: Male Tibetans had lower [Hb] compared to Han Chinese males (p < 0.05), consistent with reports for individuals from these populations living at high altitude and sea level. Measurements of ventilation (resting ventilation, HVR, and PetCO2) were similar for both groups. Heart rate responses to hypoxia were similar in both groups during isocapnia; however, HHRR in poikilocapnia was reduced in the Tibetan group (p < 0.03), and the heart rate response to CO2 in hypoxia was lower in Tibetans relative to Han Chinese (p < 0.01).
Conclusion: These results suggest that Tibetans living at intermediate altitude have blunted cardiac responses in the context of hypoxia. Hence, only some of the phenotypes observed in Tibetans living at high altitude are observed in Tibetans living at intermediate altitude. Whereas blunted cardiac responses to hypoxia is revealed at intermediate altitudes, manifestation of other physiological adaptations to high altitude may require exposure to more severe levels of hypoxia.
Mammals exposed to low oxygen (O2) conditions (hypoxia) maintain O2 homeostasis through a variety of physiological responses (Lenfant, 1973; West, 2012). These include both acute responses, such as the hypoxic ventilatory response (HVR) (Teppema and Dahan, 2010; Pamenter and Powell, 2016) and increased heart rate (Richalet and Lhuissier, 2015), as well as time-dependent responses to chronic hypoxia such as ventilatory acclimatization to hypoxia (Powell et al., 1998), increased hematocrit (Beall, 2007), and changes in metabolism (Murray, 2016; O’Brien et al., 2020). Exposure to life-long hypoxia at high altitude in populations throughout many generations may exert evolutionary pressure resulting in adaptations to hypoxia by natural selection. Survival in high-altitude hypoxic environments is possible due to physiological strategies that compensate for low oxygen levels; however, physiological changes may reflect both adaptive and maladaptive responses to this environmental stress (Moore, 2001; Beall, 2006; Beall, 2007; Petousi and Robbins, 2014; Simonson, 2015; Storz and Scott, 2021).
Two populations often studied in terms of responses to hypoxia include Tibetan residents of high altitude (≥3,500 m) and Han Chinese who generally live at low altitudes (Kapoor and Kapoor, 2005; Petousi et al., 2014; Petousi and Robbins, 2014). For example, studies show that pulmonary ventilation is greater in Tibetans than Han Chinese when both are acclimatized to high altitude (Zhuang et al., 1993), and hematocrit is lower in Tibetans compared to Han Chinese living at high altitude (Zhuang et al., 1993; Garruto et al., 2003; Wu et al., 2005; Mairbäurl et al., 2020). While many Tibetans exhibit lower hemoglobin concentration ([Hb]) relative to other individuals at high altitude, many Andean residents develop excessive erythrocytosis, which is associated with chronic mountain sickness (Villafuerte and Corante, 2016; Villafuerte et al., 2022). Hence, lower [Hb] due to alterations in plasma volume (Stembridge et al., 2019) or potential limited erythrocytosis and increased red cell destruction (Tift et al., 2020; Yu et al., 2022) in Tibetans may be adaptive at high altitude.
There is evidence for positive selection in populations that have resided at high altitude for hundreds of generations, including Tibetans (Simonson, 2015; Moore, 2017; Bhandari and Cavalleri, 2019). Recent studies show that many of the same genes are subject to selection across different high-altitude populations, although the specific variants can differ between populations (Simonson, 2015; Bigham, 2016; Witt and Huerta-Sánchez, 2019; Yu et al., 2022; Lawrence et al., 2024), as can the phenotypes (Beall et al., 1997; Beall, 2006; Beall, 2007; Tift et al., 2020; Yu et al., 2022). Several studies have demonstrated strong associations between [Hb] at high altitude and selected genetic loci, e.g., EPAS1 (Beall et al., 2010; Simonson et al., 2010), although more work is needed to determine whether hemoglobin and hematocrit are the direct target of selection at high altitude or a concomitant change (Storz, 2010; Stembridge et al., 2019; Yu et al., 2022). Research that aims to identify links between [Hb] and other key phenotypes at high altitude may provide insight for future mechanistic studies.
If phenotypic differences observed between populations at high altitude have a genetic basis (Savolainen et al., 2013), it is plausible that such differences may persist independent of hypoxia exposure or demonstrate distinct gene-by-environment effects. Petousi et al. (2014) studied Tibetan individuals born at high altitude but living near sea level and compared them with Han Chinese individuals living in the same location. They found significantly lower [Hb] and higher ventilation (relative to metabolism) in Tibetans (Petousi et al., 2014), which is consistent with results collected in these groups residing at high altitude (Zhuang et al., 1993). However, in contrast to studies at high altitude comparing Tibetan to Han Chinese and other populations (Zhuang et al., 1993; Beall, 2000; Beall, 2006; Beall, 2007), they found no differences in the hypoxic ventilatory response (HVR) between Tibetans and Han Chinese. Unlike [Hb] and ventilation, the heart rate response to hypoxia has not been extensively studied in Tibetans. Blunted heart rate responses to hypoxia were observed in Tibetan individuals who, compared to participants with an ancestral history of sea-level residence, traveled from sea level to high altitude (Reeves et al., 1987; Groves et al., 1993; Bärtsch and Gibbs, 2007; Gonggalanzi et al., 2017); however, differences in acute normobaric hypoxic heart rate response in individuals with high-altitude and sea-level ancestries has not been demonstrated. Our previous research conducted on Andeans residing at an altitude of 4340 m identified an association between increased hematocrit (a measure of red blood cell concentration) and decreased hypoxic heart rate response (HHRR), suggesting a relationship between these oxygen transport components in highland populations (Heinrich et al., 2020). We extend such observations to examine individuals of Tibetan and Han Chinese ancestry living at an intermediate altitude (1,319 m) and test for differences in heart rate responses to hypoxia.
Our ultimate goals are to determine whether sensitivity to oxygen varies between individuals with high-altitude and sea-level ancestries in different environmental contexts, investigate how these phenotypes relate to other components of oxygen transport, and use this information to assess the genetic basis for phenotypic variation over a range of altitudes. We hypothesize that Tibetans living at intermediate altitude show traits of adaptation observed in high-altitude populations when compared to a Han Chinese control group. We measured [Hb], as well as ventilatory and heart rate responses to acute hypoxia, in Tibetans and Han Chinese residents of intermediate altitude and explored the relationships between these measuements.
This study was approved by the University of San Diego Human Research Protections Program (#140235). Measurements were completed at the University of Utah, School of Medicine (1,319 m above sea level).
All participants of Han Chinese (6 female and 10 male) and Tibetan (17 female and 12 male) ancestries resided in Salt Lake City, UT, for a minimum of 6 months, which exceeds current estimates of red blood cell lifespan and time needed for ventilatory de-acclimatization from chronic hypoxia exposure. The exclusion criteria included travel to altitudes above 2,500 m in the previous month, a history of cardiovascular or pulmonary disease, as well as participants with past or current history of smoking. Participants had self-reported Tibetan or Han Chinese ancestry history. Two Han Chinese and nine Tibetan participants did not provide blood samples for measurement of hemoglobin concentration. For measurements of ventilation and heart rate responses to hypoxia, six female and 10 male Han Chinese and 13 female and 10 male Tibetan performed the experiments. Two Tibetan males were excluded from the physiology portion of the study as their SpO2 did not decrease the full 10% during hypoxia required for this study. One Tibetan women did not complete ventilatory studies and another had measurements of isocapnia but not poikilocapnia. One Han Chinese and three Tibetan post-menopausal women were included in the study. All participants provided written consent to documents provided in English. Medical histories and physical examinations were performed during a preliminary screening session to verify no prior history of cardiovascular or pulmonary disease and current use of interfering medications. During this period, participants were also questioned about their history of high-altitude residence and ancestral background (self-identified ancestry and geographical location of their parents and grandparents). We measured height, weight, BMI, as well as systolic (SBP) and diastolic (DBP) blood pressure using a manual sphygmomanometer. Mean arterial blood pressure (MABP) was calculated as MABP = DBP + (1/3)*(SBP - DBP). Participants did not consume caffeine for at least 8 h and corticosteroids nor NSAIDs for at least 48 h prior to physiological tests.
[Hb] was measured via AVOXimeter 4000 (Avox Systems, Inc., San Antonio, TX) immediately following venous blood draw.
Participants completed an 8–10-min abbreviated version of the full ventilatory response protocol to allow for acclimation to the devices and to determine appropriate individual gas flows needed to reach their target oxygen saturations (SpO2) and end-tidal P CO2 (PetCO2). Participants returned after a >30-min rest period to complete the full protocol. This rest period and preparation for the next test allowed sufficient time for recovery from hypoxic ventilatory decline induced by the screening session hypoxia exposure (Easton et al., 1988). During testing, participants sat in a chair and a mask was placed over the mouth and nose (7600 V2 Oro-Nasal Mask, Hans Rudolph, Shawnee, KS); leaks were checked by having the participant inhale against a closed inspiratory valve to ensure a vacuum was produced. The mask was connected to a one-way vented breathing circuit with a non-rebreathing valve (2700 Series, Large, Hans Rudolph). A three-way valve upstream of the mask allowed either room air or gas mixtures of N2/O2/CO2 to flow into the circuit. O2 and CO2 were continuously sampled from the tube right before the mask or non-rebreathing valve directly in front of the mouth, respectively (Model 17,620 and 17515A, VacuMed, Ventura, CA, United States). Inspiratory flow was measured by a pneumotachograph (Fleisch No. 3, OEM Medical Inc., Richmond, VA, United States of America) upstream of the mask and connected to a differential pressure transducer (model CD15, Valine, Northridge, CA, United States of America). SpO2 and heart rate were measured by a pulse oximeter (Radical-7, Masimo Corporation Irvine, CA, United States) with a surface probe placed on the forehead. All analog signals were processed through a PowerLab 8/30 (ADInstruments, Colorado Springs, CO, United States) and sent digitally to a laptop computer (HP Probook, HP Inc., Palo Alto, CA, United States). Raw data was recorded in LabChart 8 (ADInstruments).
Gas mixtures were manually controlled with a three-channel rotameter flow meter (Matheson Gas Products, Montgomeryville, PA, United States) that delivered mixtures upstream of the three-way valve at flow rates sufficient to prevent rebreathing. We measured SpO2, fraction of inspired O2 (FiO2), fraction of inspired CO2 (FiCO2), tidal volume (VT), respiratory frequency (fR), minute ventilation (
Data are expressed as mean ± standard error. Statistical analyses comparing two groups were performed using Students t-tests between groups of individuals with Han Chinese and Tibetan ancestries. We performed two-way ANOVA analyses to test ancestry and sex differences within measurements and three-way ANOVA analyses to test the effects of ancestry, sex, and hypoxia exposure on the different variables. The results show significance of both ancestry and sex denoted by ψ and ɸ, respectively. Sidak post hoc test was performed when appropriate, and p < 0.05 was considered statistically significant for all tests.
We quantified significant correlations between measurements with Pearson correlation coefficients. We also determined whether slopes and intercepts were significantly different from comparisons of simple linear regressions generated for each group. For all statistical analysis, we used GraphPad Prism version 9.0.2 for Windows, GraphPad Software, San Diego, California, United States.
The analysis grouping together males and females revealed that Tibetans have significantly lower [Hb] (13.8 ± 0.29 g/dL) compared to participants of Han Chinese ancestry (15.5 ± 0.43 g/dL, Student’s t-test, p < 0.003). Since sex has an effect on [Hb] at high altitude (Beall, 2007), we performed analysis grouping by sex. We found significantly higher values of [Hb] in Han Chinese compared to Tibetan males (15.9 ± 0.34 g/dL and 14.9 ± 0.33 g/dL, respectively, p < 0.05, Figure 1A). The analysis performed in females did not show significant differences (p = 0.151, 14.53 ± 1.15 g/dL and 13.08 ± 0.31 g/dL for Han Chinese and Tibetan women, respectively, Figure 1B).
Figure 1. Hemoglobin concentration ([Hb]) in individuals with Tibetan and Han Chinese ancestry. We observed lower [Hb] in male Tibetans (red) compared to participants of Han Chinese (blue) ancestry (A), One-tailed Student’s t-test, *p < 0.05 (Han Chinese n = 10; Tibetan n = 9). Similar analysis performed in Females (B) did not show significant differences (p = 0.151 Han Chinese n = 4; Tibetan n = 15).
A summary of demographic variables and physiological measurements recorded during baseline room air conditions is provided in Table 1. The average age of participants was not different between groups. We observed a significant effect of sex on height (p < 0.0001) and weight (p < 0.0001) between groups, although sex had no effect on BMI. Post-hoc analysis revealed significantly lower values of height and weight for women compared to men within the same ancestry group (Table 1).
The two-way ANOVA showed a significant effect of ancestry on BMI and SpO2 while breathing room air (p < 0.02 for BMI and p < 0.047 for SpO2, Table 1); however, post hoc analysis did not reveal significant differences between groups (p > 0.05). We did not find significant differences for physiological features such as ventilatory parameters (VT, fR and V.i), heart rate, and blood pressure measurements in participants breathing room air (Table 1).
Figure 2 shows simultaneous example recordings used to measure the hypoxic ventilatory and heart rate responses during conditions of isocapnia and poikilocapnia.
Figure 2. Example of raw data recorded in the same participant in conditions of isocapnic (A) and poikilocapnic hypoxia (B). Simultaneous recordings of fraction of inspired oxygen (FiO2), O2 saturation (SpO2), concentration of CO2 in the mask of the participant (Mask CO2), end-tidal P CO2 (PetCO2) inspiratory volume (Ins Vol), minute ventilation (V.i), and heart rate (HR). For analysis, a minimum of 30 s of recording was averaged during baseline and stable signals during hypoxic episodes (marked in gray).
As expected, we found a significant effect of hypoxia on V.i for both isocapnic (Figure 3A, p < 0.0001) and poikilocapnic hypoxia (Figure 3B, p < 0.0001); however, ancestry was not associated with significant differences in V.i. Furthermore, we did not find significant differences in HVR between Tibetan and Han Chinese ancestry for isocapnic (Figure 3C) nor poikilocapnic hypoxia (Figure 3D). No significant effect of sex was revealed with V.i, although there was a significant interaction between ancestry, sex, and hypoxia for poikilocapnic hypoxia (p < 0.04, three-way ANOVA). We did not find a significant effect of ancestry in isocapnic or poikilocapnic conditions of HVR, although we did find a significant effect of sex (p < 0.03, two-way ANOVA) for poikilocapnic hypoxia. Post-hoc analysis of poikilocapnic hypoxia demonstrated non-significant differences in the Han Chinese group due to sex but higher values of HVR in Tibetan women (0.0026 ± 0.0003 L/min∙%) versus Tibetan men (0.0008 ± 0.0007 L/min∙%) (p < 0.05).
Figure 3. Ventilatory response to hypoxia. Measurements of minute ventilation (V.i) and hypoxic ventilatory responses (HVR) in conditions of isocapnia (A,C) and poikilocapnia (B,D) in Tibetan (red) and Han Chinese (blue) individuals. Two-way ANOVA performed for isocapnic and poikilocapnic conditions in figures (A,C) reveal a significant effect of acute hypoxia (p < 0.0001), but no effects of ancestry. Analysis of HVR in (C,D) did not show significant differences. Han Chinese n = 16 (10 males, 6 females); Tibetans n = 20 in isocapnia (8 males, 12 females) and 19 in poikilocapnia (8 males, 11 females). For (C,D) males in dark blue and red circles and female in light blue and red circles.
We found a significant effect of hypoxia on heart rate (HR) (p < 0.0001), but ancestry did not have a significant effect in isocapnic (Figure 4A) nor poikilocapnic hypoxia (Figure 4B). We identified an interaction of hypoxia and ancestry during poikilocapnic hypoxia (p < 0.03) with no sex difference.
Figure 4. Heart rate responses to hypoxia. Measurements of heart rate (HR) and hypoxic heart rate response (HHRR) in conditions of isocapnia (A,C) and poikilocapnia (B,D) in Tibetan (red) and Han Chinese (blue) individuals. Two-way ANOVA performed for isocapnic and poikilocapnic conditions for HR in figures (A,B) reveal a significant effect of acute hypoxia (p < 0.0001), but no independent effects of ancestry. There is a significant interaction between hypoxia and ancestry status for poikilocapnic hypoxia (p < 0.05). Student’s t-test analysis of HHRR in isocapnic hypoxia (C) did not show significant differences; however, we found a significant effect of ancestry in poikilocapnic hypoxia ((D), *p < 0.05). Two-way ANOVA analysis confirmed the effects of ancestry and revealed no effect of sex. Han Chinese n = 16 (10 males, 6 females); Tibetans n = 20 in isocapnia (8 males, 12 females) and 19 in poikilocapnia (8 males, 11 females). For (C,D) males in dark blue and red circles and female in light blue and red circles.
Quantification of the HHRR showed no significant differences during isocapnic hypoxia (Figure 4C). However, Tibetans have HHRR values significantly lower than those observed in the Han Chinese group during poikilocapnic hypoxia (Figure 4D; Han Chinese 1.28 ± 0.13 BPM/% SpO2 vs Tibetans 0.90 ± 0.10 BPM/% SpO2; p < 0.027), with confirmed effects of ancestry (p < 0.012, two-way ANOVA), although we did not observe significant effects of sex on HHRR.
To measure the sensitivity to changes in CO2, we quantified differences in V.i and HR between isocapnic and poikilocapnic hypoxia. The different levels of CO2 in these two conditions have a significant effect on V.i (Figure 5A) and, although sex did not have a significant effect itself, there is a significant interaction of CO2 and sex over V.i (p < 0.05). When we compared HR in these two conditions, we did not find significant effects of CO2 levels, nor ancestry (Figure 5C), although there was a significant interaction between the two factors (p < 0.006). Additional analysis to account for sex did not show significant differences.
Figure 5. Ventilatory and heart rate responses to changes of CO2 during hypoxia. Measurements of minute ventilation (V. i, (A)) and heart rate (HR, (C)) comparing values during isocapnic and poikilocapnic hypoxia. Measurements of the differences in isocapnic and poikilocapnic hypoxia for ventilation (HCVR, (B)) and heart rate (HCHRR, (D)) are shown for males (dark circles) and females (light circles) individuals of Tibetan (red) and Han Chinese (blue) ancestry. Two-way ANOVA performed for V. i (A) showed a significant effect of changes in CO2 during hypoxia (poikilocapnia versus isocapnia, p < 0.0001) but no effects of ancestry. Similar analysis of HR (C) did not show significant effects of ancestry or CO2 levels; however, there is a significant interaction of the two factors (p < 0.01). Analysis of HCVR did not show statistical differences (B); however, we found a significant effect of ancestry for HCHRR ((D), **p < 0.01). Han Chinese n = 16 (10 males, 6 females); Tibetans n = 19 (8 males, 11 females). For (B,D) males in dark colors and female in light colors.
We also measured the hypercapnic ventilatory response (hypoxic HCVR) and the hypercapnic heart rate response (hypoxic HCHRR) by comparing the values of V.i and HR between isocapnic and poikilocapnic conditions during the hypoxic stimulus. We did not find any differences in the hypoxic HCVR between Tibetan and Han Chinese groups (Figure 5B), and sex did not account for any differences. However, we found significant differences in the hypoxic HCHRR (p < 0.01) between Han Chinese and Tibetan groups. Participants with Han Chinese ancestry exhibited mean positive values of HCHRR (0.82 ± 0.31 BPM/mmHg) whereas Tibetans show a mean negative HCHRR (−0.56 ± 0.39, Figure 5D). Thus, on average, the Han Chinese group exhibited a greater heart rate response with poikilocapnia versus isocapnia, whereas the magnitude of the increase in HR induced by hypoxia is smaller in poikilocapnic compared to isocapnic conditions in the Tibetan group (Figure 5C). Analysis of HCHRR confirmed the significant effect of ancestry (p = 0.02, two-way ANOVA) but did not show a significant effect of sex.
We measured PetCO2 in isocapnic and poikilocapnic hypoxia (Figure 6). As expected, acute hypoxia produced a significant decrease in SpO2 in both isocapnic and poikilocapnic conditions (p < 0.0001 for both, data not shown) without significant effects of ancestry or sex. There was a significant effect of hypoxia on PetCO2 in poikilocapnic hypoxia (Figure 6B, p < 0.0001) but no effect of hypoxia in isocapnic conditions due to the intentional addition of CO2 to maintain baseline PetCO2 values (Figure 6A). We did not find significant differences due to ancestry in any of the conditions for levels of PetCO2.
Figure 6. End-tidal CO2 measurements (PetCO2) measured in conditions of isocapnia (A) and poikilocapnia (B) in Tibetan (red) and Han Chinese (blue), male (dark circles) and female (light circles) individuals. Analysis of PetCO2 in isocapnic hypoxia (A) did not show significant differences, and we found a significant effect of acute hypoxia (p < 0.0001) in poikilocapnic hypoxia (B) with no effects of ancestry. Han Chinese n = 16; Tibetans n = 20 in isocapnia and 19 in poikilocapnia.
To test if hypoxia produced a coordinated ventilatory and heart rate response, and for possible interactions between ventilatory and heart rate responses during hypoxia, we examined the relationships between HVR and HHRR under isocapnic and/or poikilocapnic conditions. We did not find significant correlations between HVR and HHRR for Han Chinese nor Tibetan groups during isocapnic hypoxia, and the linear correlations derived from those measurements were not different between groups (Figure 7A). We observed a significant positive correlation of HVR and HHRR for the Tibetan group during poikilocapnic conditions (p < 0.006) (Figure 6B), and the slopes of the linear correlations between HVR and HHRR were significantly different when we compared Han Chinese and Tibetans (p < 0.002) during poikilocapnic hypoxia (Figure 7B). When we explored the associations of HVR and HHRR separating by sex, we found Han Chinese males exhibited a significant correlation in isocapnic hypoxia (p < 0.03), which was not observed in Han Chinese females nor in Tibetan males and females. In poikilocapnic hypoxia, we found a significant correlation between HVR and HHRR in Tibetan males (p < 0.04) but not for Han Chinese males nor any of the groups of females. We found significantly different slopes of HVR versus HHRR in both Han Chinese and Tibetan males (p < 0.02) and females (p < 0.02) during poikilocapnic hypoxia.
Figure 7. Hypoxic ventilatory response (HVR) versus hypoxic heart rate response (HHRR). Relationships between HVR and HHRR during isocapnic (A) and poikilocapnic hypoxia (B) in Tibetan (red) and Han Chinese (blue), male (dark circles) and female (light circles) individuals. * Significant correlation for Tibetans (red asterisk); π p < 0.05 comparing slopes for linear regression of Tibetan versus Han Chinese groups. Han Chinese n = 16 (10 males, 6 females); Tibetans n = 20 in isocapnia (8 males, 12 females) and 19 in poikilocapnia (8 males, 11 females).
To study potential relationships of ventilatory and cardiac responses to hypoxia with [Hb], we examined relationships between HVR and HHRR with [Hb] for both isocapnic and poikilocapnic hypoxia. We did not find significant correlations between HVR and [Hb] during isocapnic nor poikilocapnic hypoxia (Figures 8A, B). However, we found significant correlations between HHRR and [Hb] during isocapnic (p < 0.05) and poikilocapnic (p < 0.005) hypoxia for Han Chinese but not Tibetans (Figures 8C, D); moreover, the slopes of the linear regressions were significantly different between Han Chinese and Tibetans in poikilocapnic hypoxia (Figure 8D; p < 0.03). When we performed separated analysis for male and females, we confirmed that relationships between HVR and [Hb] were not significant in male and females, neither for isocapnic nor poikilocapnic hypoxia. The analysis of HHRR versus [Hb] separated by sex showed a significant correlation for male Han Chinese during poikilocapnic hypoxia (p < 0.02), with no significant correlation for Tibetan males, and slopes were significantly different (p < 0.02) between groups.
Figure 8. Hemoglobin concentration ([Hb]) versus hypoxic ventilatory response (HVR) or hypoxic heart rate response (HHRR) in isocapnic (A,C) and poikilocapnic conditions (B,D). Tibetan (red) and Han Chinese (blue), male (dark circles) and female (light circles) individuals. * Significant correlation for Han Chinese (blue asterisk); π p < 0.05 comparing slopes for linear regression of Tibetan versus. Han Chinese groups. Han Chinese n = 16 (10 males, 6 females); Tibetans n = 20 in isocapnia (8 males, 12 females) and 19 in poikilocapnia (8 males, 11 females).
Our results show Tibetan males exhibit relatively lower [Hb] than Han Chinese males at intermediate altitude, consistent with studies at low and high altitudes, no differences in HVR in contrast to previous findings at high altitude, and novel hypoxia-induced heart rate response differences under poikilocapnic conditions between these groups. Our analyses also revealed differences in the relationship between HVR and HHRR in poikilocapnia and between [Hb] with HHRR in both isocapnic and poikilocapnic conditions.
A hallmark phenotype in Tibetans is relatively lower [Hb], which is suggested to be the result of a blunted hematological response to hypoxia (Beall et al., 1998; Petousi and Robbins, 2014; Gassmann et al., 2019), greater plasma volume (Stembridge et al., 2019), or potentially increased red cell destruction (Tift et al., 2020) in this population. Many Tibetans living at high altitude have [Hb] similar to that observed in sea-level lowland residents (Beall et al., 1998) and have lower [Hb] concentrations compared to many adult Han Chinese or Andeans living at similar high altitudes (Garruto et al., 2003; Wu et al., 2005; Beall, 2007). Some high-altitude residents increase [Hb] to pathological levels that are linked to chronic mountain sickness, which has a notably lower prevalence in Tibetans relative to other populations who have lived at high altitude for hundreds of generations (Villafuerte and Corante, 2016; Villafuerte et al., 2022). We found that participants of this study at intermediate altitude exhibited [Hb] within a range similar (Figure 1) to that of lowland residents and Tibetans at high altitude (Beall et al., 1998), which is further consistent with lower [Hb] observed in Tibetans males relative to Han Chinese males living at sea level (Petousi et al., 2014) or Tibetan males and females residing at high altitude (Beall, 2007). Petousi et al. (2014) also measured plasma erythropoietin in Tibetan volunteers exposed to 8 h of hypoxia in a hypobaric chamber and reported the “major Tibetan” allele of EPAS1 and EGLN1 genes were associated with a blunted hypoxia-induced increase in plasma erythropoietin when compared to participants with the minor “Tibetan” allele. However, it is also possible that differences in plasma volume (Stembridge et al., 2019) or red blood cell degradation (Tift et al., 2020) may have an effect on different levels of [Hb] measured at low altitude, as has been observed or suggested at high altitude. Thus, independent of the mechanism, it is plausible that differences in [Hb] we observed at intermediate altitude are driven by genetic variants present in the Tibetan population. This is particularly important given that differences in [Hb] vary based on ancestry at different altitudes (Mairbäurl et al., 2020) and sex (Beall, 2007; Gassmann et al., 2019; Mairbäurl et al., 2020); however, most of the studies comparing [Hb] measurements between Han Chinese and Tibetans have been performed in male participants or report values of [Hb] in females at high altitude (Zhuang et al., 1993; Beall, 2007; Gassmann et al., 2019; Mairbäurl et al., 2020). In this study, we report values of [Hb] in women of Tibetan ancestry living at intermediate altitude. We found [Hb] in Tibetan women in this study is similar to those observed in Tibetan women at high altitude (Beall, 2007); however, we did not find significant differences in [Hb] between Tibetan and Han Chinese women. We acknowledge the low number of female individuals studied and that four of these female participants are post-menopausal. Therefore, with this evidence, it is clear that more studies need to be performed in women at sea level and intermediate altitude to determine potential [Hb] differences in based on ancestry, menopausal status, and hormone profile.
We did not find differences in ventilatory parameters during baseline nor acute-hypoxic conditions between Han Chinese and Tibetans. The values of VT, fR, and V.i in participants breathing room air as well as the HVRs (both isocapnic and poikilocapnic), and the ventilatory responses to changes in CO2 during hypoxia (HCVR), did not show a significant effect of ancestry at intermediate altitude. These findings are different from those reported for Han Chinese and Tibetans residing at high altitude (Zhuang et al., 1993; Curran et al., 1997), although they agree partially with data from Sherpas compared to “Westerners” at intermediate and high altitude (Hackett et al., 1980) and Tibetans versus Han Chinese at sea level (Petousi et al., 2014). Zhuang et al. compared lifelong Tibetan residents with acclimatized Han Chinese newcomers at 3,658 m and described increased minute ventilation breathing room air and increased hypoxic sensitivity (parameter A from the HVR, with hyperbolic adjustment) for the Tibetan group under isocapnic conditions. However, they did not find differences between groups when HVR values of delta V. i over delta SpO2 were compared. Despite differences in hypoxic sensitivity, the authors compared their measurements with others published previously and found that hypoxic sensitivity in high-altitude Tibetans was similar to low-altitude residents or acclimatized newcomers, demonstrating that Tibetans do not exhibit a blunted HVR and, on the contrary, they maintain increased ventilation as if they just arrived to high-altitude (Zhuang et al., 1993). Petousi et al. (2014) studied Han Chinese and Tibetan participants residing at sea level and found that Tibetans had lower End-tidal PetCO2 values than Han Chinese (indicating increased baseline ventilation for a given metabolic rate), but the authors also found similar values of ventilation in normoxia and acute hypoxic ventilatory sensitivity during isocapnia for Han Chinese and Tibetans (Petousi et al., 2014). Hence, differences in End-tidal PetCO2 must reflect metabolic differences, as well as potential differences in CO2 regulation. A study performed in Sherpas and “Westerners” at low and high altitude demonstrated higher values of resting ventilation in the Sherpa group, although they found that HVR values were not different between groups when corrected by body size (Hackett et al., 1980).
Thus, our results at intermediate altitude agree with previous literature observing similar acute ventilatory responses between Han Chinese and Tibetans at sea level and high altitude, although we did not find differences in baseline ventilation based on analyses of both male and female participants. Although our analysis did not reveal sex and/or ancestry effects for End-tidal PetCO2 nor HVR, it will be necessary to perform a large-scale analysis regarding sex differences in these populations. It is plausible that the severity of hypoxia is not enough to produce significant differences in resting ventilation between Han Chinese and Tibetans or that such differences are not present in the absence of long-term high-altitude exposure.
Exposure to acute hypoxia produced the expected increase in heart rate, which stabilized during 5 minutes of the stimulus. We found the HHRR was similar during isocapnic hypoxia between ancestry groups; however, during poikilocapnic hypoxia, the increase of HR was smaller in the Tibetans compared to the average Han Chinese response (Figure 4D). Moreover, when we quantified the heart rate responses to changes in CO2 during hypoxia (or HCHRR), we found a significant difference with an average negative value in Tibetans contrasting the positive average value for the Han Chinese group (Figure 5D). These results highlight an inhibitory effect of lower values of PetCO2 (due to hyperventilation when we do not control CO2) on heart rate that is present in the Tibetan group but not in the Han Chinese. To our knowledge, this is the first time that population-related differences in HR response to hypoxia are found in people not living in extreme high-altitude conditions. The range of values of HHRR that we observed for Han Chinese (0.9–1.28) is comparable to those reported before for individuals from sea-level populations after 5 min of isocapnic and poikilocapnic hypoxia in healthy men (Simon et al., 1995; Ainslie and Poulin, 2004; Steinback and Poulin, 2008). Experiments studying the mechanisms of HVR establish that the acute ventilatory response is mainly attributed to activation of the peripheral chemoreflex starting in the carotid bodies, the cardiac and vascular changes induced by hypoxia are influenced by more than one process. The cardiovascular responses to hypoxia occur by activation of several mechanism including: stimulation of central nervous system and arterial chemoreceptors, direct effects of hypoxia and hypocapnia on heart and vasculature, modulation of the nervous system by secondary mechanisms such as pulmonary stretch afferents, alkalosis produced by hyperventilation, and increased secretion of catecholamine from the adrenal glands (de Burgh Daly, 1997; Joyce and Wang, 2022). In particular, the changes of heart rate observed in acute hypoxia are the result of activation of sympathetic and vagal cardiac efferent activities that can be triggered by the secondary inputs mentioned (de Burgh Daly, 1997). Studies performed in dogs and humans concluded that the primary HR response resulting from isolated activation of the carotid bodies is bradycardia (Downing et al., 1962; de Burgh Daly, 1997; de Burgh Daly et al., 2000; Burgh Daly, 2011), and patients with resected carotid bodies show tachycardia with breath-holding in an oxygen-tension-dependent way (Gross et al., 1976). With this, we initially speculated that Tibetans may have increased arterial chemoreceptor activity resulting in smaller HR responses during hypoxia. However, the fact that we did not find differences in ventilatory parameters (Figure 3) nor in Dejour’s test (data not shown) associated with ancestry suggests that the blunted heart rate responses observed in Tibetans in poikilocapnic conditions (Figure 4D; Figure 5D; Figure 7B) are regulated by differential effects to CO2 sensitivity more than O2-derived mechanisms. Our finding of blunted heart rate responses in Tibetans during poikilocapnia, but not isocapnia, reflects a realistic situation of hypoxia exposure where hyperventilation produces a decrease in PetCO2 and highlights the role central CO2 chemoreceptors may have in the differential cardiovascular responses to hypoxia in populations historically living at high altitude. When we compared the HR response in poikilocapnic versus isocapnic hypoxia, Han Chinese showed a positive value of HCHRR, reflecting that the average HR in poikilocapnic hypoxia is higher than in isocapnic hypoxia (Figures 5C, D). Early reports found similar results, with smaller heart rate responses to hypoxia in conditions of isocapnia when compared to poikilocapnic hypoxia (Richardson et al., 1966). However, we found for the first time that Tibetans have different HR responses with an average negative HCHRR value, meaning that, on average, Tibetans have lower HR during poikilocapnic hypoxia compared to isocapnic hypoxia (Figures 5C, D). This finding in Tibetans who are not exposed to high altitude suggests that genetic factors, such as those previously reported, including those in the hypoxia inducible factor pathway (Simonson, 2015; Yu et al., 2022; Lawrence et al., 2024), may preserve high-altitude features in these populations even when living closer to normoxic conditions.
Exposure to acute hypoxia produces an increase of HR concomitant to an increased sympathetic activity (Halliwill et al., 2003; Hanada et al., 2003), and in high-altitude hypoxia, the increase in heart rate is related to an increase in sympathetic activity and vagal withdrawal (Koller et al., 1988; Hansen and Sander, 2003; Bärtsch and Gibbs, 2007). Thus, it is also possible that genetic factors determining different autonomic responses to hypoxia in human populations explain different HR responses that we observed at intermediate altitude, with lower HHRR and HCHRR in Tibetans compared to Han Chinese groups. In fact, increased sympathetic activity is observed in both lowlanders and Andeans exposed to high altitude (Hansen and Sander, 2003; Lundby et al., 2018; Simpson et al., 2021a; Simpson et al., 2021b), whereas Sherpa highlanders show lower muscle sympathetic nerve activity at high altitude (Simpson et al., 2019; Simpson et al., 2021b). The differences in the autonomic responses between lowlanders and/or Andeans and Sherpa populations could also be explained by genetic factors contributing to the differential responses observed in Tibetans. Zhou et al. studied Tibetans born and raised at high altitude (3700 m) who migrated to sea level for four years and compared them with a lowland Han Chinese group. The authors exposed both groups to acute hypoxia in a hypobaric chamber (2 h, simulating 3700 m) and found that acute hypoxia induced a significant increase of HR in the Han Chinese but not in the Tibetan population. They attribute these differences to changes in sympathetic stimulation during acute hypoxia (based on heart rate variability parameters) that occur in Han Chinese but were not observed in the Tibetan group (Zhou et al., 2008). These responses may manifest also at lower altitudes in individuals with high-altitude ancestry. For example, Petousi et al. found blunted pulmonary vascular responses to acute isocapnic hypoxia in a Tibetan population living at sea level when compared to a Han Chinese population residing in the same location (Petousi et al., 2014). Another argument to explain the observed differences in HHRR may be distinct values of stroke volume in Tibetan and Han Chinese populations that compensate for differences in heart rate during hypoxia. Reports studying Tibetan and Han Chinese individuals have shown similar stroke volume and cardiac output in resting conditions at more than 3417 m of altitude (Ge et al., 1995; Chen et al., 1997; Simonson et al., 2015), at sea level, and during maximum exercise (Simonson et al., 2015). Petousi et al. did not find significant differences in the cardiac output of Tibetan and Han Chinese in resting conditions, either during normoxia nor during isocapnic hypoxia (Petousi et al., 2014), but the changes of cardiac output during poikilocapnic hypoxia have not been studied. Thus, more studies are required to establish the responses to acute hypoxia in these different populations and which high-altitude phenotypes are maintained under sea-level conditions.
In order to characterize phenotypic differences between Tibetan and Han Chinese groups during hypoxia, we studied the correlations between HVR and HHRR and also between these parameters and [Hb]. We acknowledge that correlations are not a mechanistic approach to study causal mechanisms of the potential genetic or CO2-dependent contributors to the responses to hypoxia. However, these observations in human populations may provide insight into potential mechanisms that could be studied in animal or cellular models that help to elucidate relevance in evolutionary contexts and/or hypoxia-related pathological conditions.
The mechanisms involved in control of breathing and heart rate are considered independent, although these two variables can interact, for example, during respiratory sinus arrhythmia (Perry et al., 2019; Hayano et al., 1996). A high level of correlation is described between minute ventilation and HR, particularly during aerobic work (Vai et al., 1988; Treese et al., 1993; Mermier et al., 1993) with both V.i and HR positively correlated with oxygen uptake (Gastinger et al., 2010). Richalet and Lhuissier proposed that during moderate exercise, HVR and HHRR exhibit a negative association in sojourners exposed to high altitude (Richalet and Lhuissier, 2015) and therefore poikilocapnic hypoxia. Our study is the first to show these correlations in Han Chinese and Tibetan groups. We found that Tibetans have a positive correlation of HVR and HHRR in poikilocapnic hypoxia, with a slope significantly different than the Han Chinese group. The Han Chinese group shows a negative slope although the correlation of HVR and HHRR was not significant in our results. Future studies with a larger number of participants need to be performed to determine if people with Han Chinese ancestry show negative correlations of HVR and HHRR.
The correlation between ventilatory and heart rate responses to hypoxia also varies across species (Burgh Daly, 2011). Stimulation of arterial chemoreceptors with hypoxic blood produced increased ventilation in various species but produced bradycardia in seals and cats (Macleod and Scott, 1964; Elsner et al., 1977), a mixed bradycardic and tachycardic response in dogs (de Burgh Daly and Scott, 1958), and complete tachycardia in monkeys (de Burgh Daly et al., 1978; Burgh Daly, 2011). The correlation between ventilation and heart rate response induced in hypoxia in humans is similar to the one found in primates, with tachycardia observed concomitant to increased ventilation, although a significant correlation of HVR versus HHRR has not been found in conditions of isocapnic hypoxia (Slutsky and Rebuck, 1978; Simon et al., 1995). Our results agree with these results showing that, in isocapnic hypoxia, there is no correlation between HVR and HHRR, and we did not find differences between linear regressions of Tibetans and Han Chinese (Figure 7A). However, during poikilocapnic hypoxia, we found a positive significant correlation in Tibetans that was not observed in the Han Chinese group, and the slopes of the Tibetan and Han Chinese group were significantly different (Figure 7B). Thus, there is an effect of lower CO2 due to hyperventilation that is affecting Tibetan and Han Chinese populations differently. This effect must be attributed to CO2 since these dissimilar relationships are not observed in isocapnic conditions, thus affecting this correlation due to different HR responses in poikilocapnia.
We found negative associations between [Hb] and HHRR in Han Chinese in both hypoxic isocapnic and poikilocapnic conditions (Figures 8C, D). A similar negative correlation is also observed in individuals with excessive erythrocytosis and blunted HHRR in isocapnic hypoxia in a study that included Andean participants with chronic mountain sickness (Heinrich et al., 2020). We found these negative [Hb] versus HHRR associations even when none of our Han Chinese participants showed levels of [Hb] outside the range expected at sea level. On the other hand, we did not find significant correlations of [Hb] versus HHRR in our Tibetan group (Figure 8). These profiles of correlations between [Hb] and HHRR for Han Chinese and Tibetans are independent of the isocapnic or poikilocapnic status; therefore, these differences are attributed to ancestry but not to an inhibitory effect of CO2. Recent studies indicate [Hb] increases consistently with altitude across several human populations, but the increase in [Hb] with altitude is greater only among individuals of native South American ancestry (Gassmann et al., 2019) versus a reference group. In the same manuscript, in Tibetans, the correlation between [Hb] and altitude is significant, but not different from the reference group, highlighting the potential for distinct physiological outcomes such as the prevalence of chronic mountain sickness, which is much less common among Tibetans (Gassman et al., 2019). Whether excessive erythrocytosis and potential deleterious effects of increased blood viscosity are absent in Tibetans or if there are different mechanisms modulating vascular contractility to reduce vascular resistance during hypoxia remains to be determined.
The main limitation of this study is a limited number of participants, which are similar to other studies of ventilatory responses to hypoxia (Zhang and Robbins, 2000; Brutsaert et al., 2005). However, in this study, we examine ventilatory and heart rate responses in both males and females, four of whom were post-menopausal. Future studies that aim to understand sex differences in physiological responses to hypoxia and the contribution of hormones and menopausal status to ventilatory responses to hypoxia are needed in populations with a history of high-altitude residence.
The average lower [Hb] observed in Tibetan males confirm a phenotype associated (directly or indirectly) with high-altitude adaptation that is maintained at intermediate altitude. The hypoxic ventilatory responses in Tibetans living at intermediate altitude were not different from Han Chinese living in the same location during isocapnic or poikilocapnic conditions. Compared to Han Chinese, Tibetans exhibited attenuated heart rate responses to hypoxia in poikilocapnic conditions, whereas isocapnic responses were not different. Also, the relationship between HVR and HHRR was different during poikilocapnia but not in isocapnia, highlighting the differential effect of CO2 on the coordinated breathing and heart rate responses to hypoxia in people with Tibetan ancestry. The potential contribution of molecular signals to high-altitude hypoxic adaptation as well as the physiological responses driven by these pathways when living at different altitudes require further investigation.
The raw data supporting the conclusion of this article will be made available by the authors, without undue reservation.
The studies involving humans were approved by University of San Diego Human Research Protections Program (#140235). The studies were conducted in accordance with the local legislation and institutional requirements. The participants provided their written informed consent to participate in this study.
EM: Conceptualization, Data curation, Formal Analysis, Investigation, Methodology, Project administration, Supervision, Validation, Visualization, Writing–original draft, Writing–review and editing. JY: Data curation, Formal Analysis, Investigation, Methodology, Supervision, Validation, Writing–review and editing. SB: Investigation, Methodology, Project administration, Writing–review and editing. WG: Investigation, Writing–review and editing. EL: Project administration, Writing–review and editing. RC: Methodology, Project administration, Writing–review and editing. AB: Methodology, Project administration, Writing–review and editing. WW: Methodology, Project administration, Writing–review and editing. KA: Project administration, Writing–review and editing. SM: Investigation, Methodology, Project administration, Resources, Supervision, Writing–review and editing. FP: Conceptualization, Investigation, Supervision, Validation, Writing–review and editing. TS: Conceptualization, Formal Analysis, Funding acquisition, Investigation, Project administration, Resources, Supervision, Validation, Writing–review and editing.
The author(s) declare that financial support was received for the research, authorship, and/or publication of this article. This research was supported by National Institutes of Health R01HL145470, the National Geographic Society Explorer Award, and the John B. West Endowed Chair in Respiratory Physiology to TS.
We are grateful to all participants involved in this study. We also thank Dr. Lynn B. Jorde and W. Scott Watkins for assistance with sample storage.
The authors declare that the research was conducted in the absence of any commercial or financial relationships that could be construed as a potential conflict of interest.
All claims expressed in this article are solely those of the authors and do not necessarily represent those of their affiliated organizations, or those of the publisher, the editors and the reviewers. Any product that may be evaluated in this article, or claim that may be made by its manufacturer, is not guaranteed or endorsed by the publisher.
Ainslie P. N., Poulin M. J. (2004). Ventilatory, cerebrovascular, and cardiovascular interactions in acute hypoxia: regulation by carbon dioxide. J. Appl. Physiol. 97, 149–159. doi:10.1152/japplphysiol.01385.2003
Bärtsch P., Gibbs J. S. R. (2007). Effect of altitude on the heart and the lungs. Circulation 116, 2191–2202. doi:10.1161/CIRCULATIONAHA.106.650796
Beall C. M. (2000). Tibetan and Andean patterns of adaptation to high-altitude hypoxia. Hum. Biol. 72, 201–228.
Beall C. M. (2006). Andean, Tibetan, and Ethiopian patterns of adaptation to high-altitude hypoxia. Integr. Comp. Biol. 46, 18–24. doi:10.1093/icb/icj004
Beall C. M. (2007). Two routes to functional adaptation: Tibetan and Andean high-altitude natives. Proc. Natl. Acad. Sci. U. S. A. 104 (Suppl. 1), 8655–8660. doi:10.1073/pnas.0701985104
Beall C. M., Brittenham G. M., Strohl K. P., Blangero J., Williams-Blangero S., Goldstein M. C., et al. (1998). Hemoglobin concentration of high-altitude Tibetans and Bolivian Aymara. Am. J. Phys. Anthropol. 106, 385–400. doi:10.1002/(SICI)1096-8644(199807)106:3<385::AID-AJPA10>3.0.CO;2-X
Beall C. M., Cavalleri G. L., Deng L., Elston R. C., Gao Y., Knight J., et al. (2010). Natural selection on EPAS1 (HIF2alpha) associated with low hemoglobin concentration in Tibetan highlanders. Proc. Natl. Acad. Sci. U. S. A. 107, 11459–11464. doi:10.1073/pnas.1002443107
Beall C. M., Strohl K. P., Blangero J., Williams-Blangero S., Almasy L. A., Decker M. J., et al. (1997). Ventilation and hypoxic ventilatory response of Tibetan and Aymara high altitude natives. Am. J. Phys. Anthropol. 104, 427–447. doi:10.1002/(SICI)1096-8644(199712)104:4<427::AID-AJPA1>3.0.CO;2-P
Bhandari S., Cavalleri G. L. (2019). Population history and altitude-related adaptation in the Sherpa. Front. Physiol. 10, 1116. doi:10.3389/fphys.2019.01116
Bigham A. W. (2016). Genetics of human origin and evolution: high-altitude adaptations. Curr. Opin. Genet. Dev. 41, 8–13. doi:10.1016/j.gde.2016.06.018
Brutsaert T. D., Parra E. J., Shriver M. D., Gamboa A., Rivera-Ch M., León-Velarde F. (2005). Ancestry explains the blunted ventilatory response to sustained hypoxia and lower exercise ventilation of Quechua altitude natives. Am. J. Physiol. Regul. Integr. Comp. Physiol. 289, R225–R234. doi:10.1152/ajpregu.00105.2005
Burgh Daly M. (2011). “Interactions between respiration and circulation,” in Comprehensive physiology. Editor R. Terjung (Wiley), 529–594.
Chen Q. H., Ge R. L., Wang X. Z., Chen H. X., Wu T. Y., Kobayashi T., et al. (1997). Exercise performance of Tibetan and Han adolescents at altitudes of 3,417 and 4,300 m. J. Appl. Physiol. 83, 661–667. doi:10.1152/jappl.1997.83.2.661
Curran L. S., Zhuang J., Sun S. F., Moore L. G. (1997). Ventilation and hypoxic ventilatory responsiveness in Chinese-Tibetan residents at 3,658 m. J. Appl. Physiol. 83, 2098–2104. doi:10.1152/jappl.1997.83.6.2098
de Burgh Daly M. (1997). Peripheral arterial chemoreceptors and respiratory-cardiovascular integration. Clarendon Press. Available at: https://play.google.com/store/books/details?id=GO1qAAAAMAAJ.
de Burgh Daly M., Korner P. I., Angell-James J. E., Oliver J. A. (1978). Cardiovascular and respiratory effects of carotid body stimulation in the monkey. Clin. Exp. Pharmacol. Physiol. 5, 511–524. doi:10.1111/j.1440-1681.1978.tb00704.x
de Burgh Daly M., Scott M. J. (1958). The effects of stimulation of the carotid body chemoreceptors on heart rate in the dog. J. Physiol. 144, 148–166. doi:10.1113/jphysiol.1958.sp006092
de Burgh Daly M., Wood L. M., Ward J. (2000). Cardiovascular responses to carotid chemoreceptor stimulation in the dog: their modulation by urinary bladder distension. J. Physiol. 524 (Pt 3), 903–917. doi:10.1111/j.1469-7793.2000.00903.x
Downing S. E., Remensnyder J. P., Mitchell J. H. (1962). Cardiovascular responses to hypoxic stimulation of the carotid bodies. Circ. Res. 10, 676–685. doi:10.1161/01.res.10.4.676
Easton P. A., Slykerman L. J., Anthonisen N. R. (1988). Recovery of the ventilatory response to hypoxia in normal adults. J. Appl. Physiol. 64, 521–528. doi:10.1152/jappl.1988.64.2.521
Elsner R., Angell-James J. E., de Burgh Daly M. (1977). Carotid body chemoreceptor reflexes and their interactions in the seal. Am. J. Physiol. 232, H517–H525. doi:10.1152/ajpheart.1977.232.5.H517
Garruto R. M., Chin C.-T., Weitz C. A., Liu J.-C., Liu R.-L., He X. (2003). Hematological differences during growth among Tibetans and Han Chinese born and raised at high altitude in Qinghai, China. Am. J. Phys. Anthropol. 122, 171–183. doi:10.1002/ajpa.10283
Gassmann M., Mairbäurl H., Livshits L., Seide S., Hackbusch M., Malczyk M., et al. (2019). The increase in hemoglobin concentration with altitude varies among human populations. Ann. N. Y. Acad. Sci. 1450, 204–220. doi:10.1111/nyas.14136
Gastinger S., Sorel A., Nicolas G., Gratas-Delamarche A., Prioux J. (2010). A comparison between ventilation and heart rate as indicator of oxygen uptake during different intensities of exercise. J. Sports Sci. Med. 9, 110–118.
Ge R.-L., Lun G.-W. H., Chen Q.-H., Li H.-L., Gen D., Kubo K., et al. (1995). Comparisons of oxygen transport between Tibetan and Han residents at moderate altitude. Wilderness Environ. Med. 6, 391–400. doi:10.1580/1080-6032(1995)006[0391:cootbt]2.3.co;2
Gonggalanzi L., Bjertness E., Wu T., Stigum H., Nafstad P. (2017). Acute mountain sickness, arterial oxygen saturation and heart rate among Tibetan students who reascend to Lhasa after 7 years at low altitude: a prospective cohort study. BMJ Open 7, e016460. doi:10.1136/bmjopen-2017-016460
Gross P. M., Whipp B. J., Davidson J. T., Koyal S. N., Wasserman K. (1976). Role of the carotid bodies in the heart rate response to breath holding in man. J. Appl. Physiol. 41, 336–340. doi:10.1152/jappl.1976.41.3.336
Groves B. M., Droma T., Sutton J. R., McCullough R. G., McCullough R. E., Zhuang J., et al. (1993). Minimal hypoxic pulmonary hypertension in normal Tibetans at 3,658 m. J. Appl. Physiol. 74, 312–318. doi:10.1152/jappl.1993.74.1.312
Hackett P. H., Reeves J. T., Reeves C. D., Grover R. F., Rennie D. (1980). Control of breathing in Sherpas at low and high altitude. J. Appl. Physiol. 49, 374–379. doi:10.1152/jappl.1980.49.3.374
Halliwill J. R., Morgan B. J., Charkoudian N. (2003). Peripheral chemoreflex and baroreflex interactions in cardiovascular regulation in humans. J. Physiol. 552, 295–302. doi:10.1113/jphysiol.2003.050708
Hanada A., Sander M., González-Alonso J. (2003). Human skeletal muscle sympathetic nerve activity, heart rate and limb haemodynamics with reduced blood oxygenation and exercise. J. Physiol. 551, 635–647. doi:10.1113/jphysiol.2003.044024
Hayano J., Yasuma F., Okada A., Mukai S., Fujinami T. (1996). Respiratory sinus arrhythmia. A phenomenon improving pulmonary gas exchange and circulatory efficiency. Circulation 94, 842–847.
Hansen J., Sander M. (2003). Sympathetic neural overactivity in healthy humans after prolonged exposure to hypobaric hypoxia. J. Physiol. 546, 921–929. doi:10.1113/jphysiol.2002.031765
Heinrich E. C., Orr J. E., Gilbertson D., Anza-Ramirez C., DeYoung P. N., Djokic M. A., et al. (2020). Relationships between chemoreflex responses, sleep quality, and hematocrit in andean men and women. Front. Physiol. 11, 437. doi:10.3389/fphys.2020.00437
Joyce W., Wang T. (2022). Regulation of heart rate in vertebrates during hypoxia: a comparative overview. Acta Physiol. 234, e13779. doi:10.1111/apha.13779
Kapoor S., Kapoor A. K. (2005). Body structure and respiratory efficiency among high altitude Himalayan populations. Coll. Antropol. 29, 37–43.
Koller E. A., Drechsel S., Hess T., Macherel P., Boutellier U. (1988). Effects of atropine and propranolol on the respiratory, circulatory, and ECG responses to high altitude in man. Eur. J. Appl. Physiol. Occup. Physiol. 57, 163–172. doi:10.1007/BF00640657
Lawrence E. S., Gu W., Bohlender R. J., Anza-Ramirez C., Cole A. M., Yu J. J., et al. (2024). Functional EPAS1/HIF2A missense variant is associated with hematocrit in Andean highlanders. Sci. Adv. 10, eadj5661. doi:10.1126/sciadv.adj5661
Lenfant C. (1973). High altitude adaptation in mammals. Am. Zool. 13, 447–456. doi:10.1093/icb/13.2.447
Lundby C., Calbet J., van Hall G., Saltin B., Sander M. (2018). Sustained sympathetic activity in altitude acclimatizing lowlanders and high-altitude natives. Scand. J. Med. Sci. Sports 28, 854–861. doi:10.1111/sms.12976
Macleod R. D., Scott M. J. (1964). The heart rate responses to carotid body chemoreceptor stimulation in the cat. J. Physiol. 175, 193–202. doi:10.1113/jphysiol.1964.sp007511
Mairbäurl H., Gassmann M., Muckenthaler M. U. (2020). Geographical ancestry affects normal hemoglobin values in high-altitude residents. J. Appl. Physiol. 129, 1451–1459. doi:10.1152/japplphysiol.00025.2020
Mermier C. M., Samet J. M., Lambert W. E., Chick T. W. (1993). Evaluation of the relationship between heart rate and ventilation for epidemiologic studies. Arch. Environ. Health 48, 263–269.
Moore L. G. (2001). Human genetic adaptation to high altitude. High. Alt. Med. Biol. 2, 257–279. doi:10.1089/152702901750265341
Moore L. G. (2017). Measuring high-altitude adaptation. J. Appl. Physiol. 123, 1371–1385. doi:10.1152/japplphysiol.00321.2017
Murray A. J. (2016). Energy metabolism and the high-altitude environment. Exp. Physiol. 101, 23–27. doi:10.1113/EP085317
O’Brien K., Simonson T., Murray A. (2020). Metabolic adaptation to high altitude. Curr. Opin. Endocr. Metabolic Res. 11, 33–41. doi:10.1016/j.coemr.2019.12.002
Pamenter M. E., Powell F. L. (2016). Time domains of the hypoxic ventilatory response and their molecular basis. Compr. Physiol. 6, 1345–1385. doi:10.1002/cphy.c150026
Perry S., Khovanova N. A., Khovanov I. A. (2019). Control of heart rate through guided high-rate breathing. Sci. Rep. 9, 1545
Petousi N., Croft Q. P. P., Cavalleri G. L., Cheng H.-Y., Formenti F., Ishida K., et al. (2014). Tibetans living at sea level have a hyporesponsive hypoxia-inducible factor system and blunted physiological responses to hypoxia. J. Appl. Physiol. 116, 893–904. doi:10.1152/japplphysiol.00535.2013
Petousi N., Robbins P. A. (2014). Human adaptation to the hypoxia of high altitude: the Tibetan paradigm from the pregenomic to the postgenomic era. J. Appl. Physiol. 116, 875–884. doi:10.1152/japplphysiol.00605.2013
Powell F. L., Milsom W. K., Mitchell G. S. (1998). Time domains of the hypoxic ventilatory response. Respir. Physiol. 112, 123–134. doi:10.1016/s0034-5687(98)00026-7
Reeves J. T., Groves B. M., Sutton J. R., Wagner P. D., Cymerman A., Malconian M. K., et al. (1987). Operation Everest II: preservation of cardiac function at extreme altitude. J. Appl. Physiol. 63, 531–539. doi:10.1152/jappl.1987.63.2.531
Richalet J.-P., Lhuissier F. J. (2015). Aging, tolerance to high altitude, and cardiorespiratory response to hypoxia. High. Alt. Med. Biol. 16, 117–124. doi:10.1089/ham.2015.0030
Richardson D. W., Kontos H. A., Shapiro W., Patterson J. L. (1966). Role of hypocapnia in the circulatory responses to acute hypoxia in man. J. Appl. Physiol. 21, 22–26.
Savolainen O., Lascoux M., Merilä J. (2013). Ecological genomics of local adaptation. Nat. Rev. Genet. 14, 807–820. doi:10.1038/nrg3522
Simon P. M., Taha B. H., Dempsey J. A., Skatrud J. B., Iber C. (1995). Role of vagal feedback from the lung in hypoxic-induced tachycardia in humans. J. Appl. Physiol. 78, 1522–1530. doi:10.1152/jappl.1995.78.4.1522
Simonson T. S. (2015). Altitude adaptation: a glimpse through various lenses. High. Alt. Med. Biol. 16, 125–137. doi:10.1089/ham.2015.0033
Simonson T. S., Wei G., Wagner H. E., Wuren T., Qin G., Yan M., et al. (2015). Low haemoglobin concentration in Tibetan males is associated with greater high-altitude exercise capacity. J. Physiol. 593, 3207–3218. doi:10.1113/JP270518
Simonson T. S., Yang Y., Huff C. D., Yun H., Qin G., Witherspoon D. J., et al. (2010). Genetic evidence for high-altitude adaptation in tibet. Science 329, 72–75. doi:10.1126/science.1189406
Simpson L. L., Busch S. A., Oliver S. J., Ainslie P. N., Stembridge M., Steinback C. D., et al. (2019). Baroreflex control of sympathetic vasomotor activity and resting arterial pressure at high altitude: insight from Lowlanders and Sherpa. J. Physiol. 597, 2379–2390. doi:10.1113/JP277663
Simpson L. L., Meah V. L., Steele A. R., Gasho C., Howe C. A., Dawkins T. G., et al. (2021a). Global REACH 2018: Andean highlanders, chronic mountain sickness and the integrative regulation of resting blood pressure. Exp. Physiol. 106, 104–116. doi:10.1113/EP088473
Simpson L. L., Steinback C. D., Stembridge M., Moore J. P. (2021b). A sympathetic view of blood pressure control at high altitude: new insights from microneurographic studies. Exp. Physiol. 106, 377–384. doi:10.1113/EP089194
Slutsky A. S., Rebuck A. S. (1978). Heart rate response to isocapnic hypoxia in conscious man. Am. J. Physiol. 234, H129–H132. doi:10.1152/ajpheart.1978.234.2.H129
Steinback C. D., Poulin M. J. (2008). Cardiovascular and cerebrovascular responses to acute isocapnic and poikilocapnic hypoxia in humans. J. Appl. Physiol. 104, 482–489. doi:10.1152/japplphysiol.00553.2007
Stembridge M., Williams A. M., Gasho C., Dawkins T. G., Drane A., Villafuerte F. C., et al. (2019). The overlooked significance of plasma volume for successful adaptation to high altitude in Sherpa and Andean natives. Proc. Natl. Acad. Sci. U. S. A. 116, 16177–16179. doi:10.1073/pnas.1909002116
Storz J. F. (2010). Evolution. Genes for high altitudes. Science 329, 40–41. doi:10.1126/science.1192481
Storz J. F., Scott G. R. (2021). Phenotypic plasticity, genetic assimilation, and genetic compensation in hypoxia adaptation of high-altitude vertebrates. Comp. Biochem. Physiol. A Mol. Integr. Physiol. 253, 110865. doi:10.1016/j.cbpa.2020.110865
Teppema L. J., Dahan A. (2010). The ventilatory response to hypoxia in mammals: mechanisms, measurement, and analysis. Physiol. Rev. 90, 675–754. doi:10.1152/physrev.00012.2009
Tift M. S., Alves de Souza R. W., Weber J., Heinrich E. C., Villafuerte F. C., Malhotra A., et al. (2020). Adaptive potential of the heme oxygenase/carbon monoxide pathway during hypoxia. Front. Physiol. 11, 886. doi:10.3389/fphys.2020.00886
Treese N., MacCarter D., Akbulut O., Coutinho M., Baez M., Liebrich A., et al. (1993). Ventilation and heart rate response during exercise in normals: relevance for rate variable pacing. Pacing Clin. Electrophysiol. 16, 1693–1700.
Vai F., Bonnet J. L., Ritter P., Janes G. (1988). Relationship between heart rate and minute ventilation, tidal volume and respiratory rate during brief and low level exercise. Pacing Clin. Electrophysiol. 11, 1860–1865.
Villafuerte F. C., Corante N. (2016). Chronic Mountain sickness: clinical aspects, etiology, management, and treatment. High. Alt. Med. Biol. 17, 61–69. doi:10.1089/ham.2016.0031
Villafuerte F. C., Simonson T. S., Bermudez D., León-Velarde F. (2022). High-altitude erythrocytosis: mechanisms of adaptive and maladaptive responses. Physiology 37, 0. doi:10.1152/physiol.00029.2021
West J. B. (2012). High-altitude medicine. Am. J. Respir. Crit. Care Med. 186, 1229–1237. doi:10.1164/rccm.201207-1323CI
Witt K. E., Huerta-Sánchez E. (2019). Convergent evolution in human and domesticate adaptation to high-altitude environments. Philos. Trans. R. Soc. Lond B Biol. Sci. 374, 20180235. doi:10.1098/rstb.2018.0235
Wu T., Wang X., Wei C., Cheng H., Wang X., Li Y., et al. (2005). Hemoglobin levels in Qinghai-Tibet: different effects of gender for Tibetans vs. Han. J. Appl. Physiol. 98, 598–604. doi:10.1152/japplphysiol.01034.2002
Yu J., Non A. L., Heinrich E. C., Gu W., Alcock J., Moya E. A., et al. (2022). Time domains of hypoxia responses and -omics insights. Front. Physiol. 13, 885295. doi:10.3389/fphys.2022.885295
Zhang S., Robbins P. A. (2000). Methodological and physiological variability within the ventilatory response to hypoxia in humans. J. Appl. Physiol. 88, 1924–1932. doi:10.1152/jappl.2000.88.5.1924
Zhou Z.-N., Zhuang J.-G., Wu X.-F., Zhang Y., Cherdrungsi P. (2008). Tibetans retained innate ability resistance to acute hypoxia after long period of residing at sea level. J. Physiol. Sci. 58, 167–172. doi:10.2170/physiolsci.RP009207
Keywords: high altitude, Tibetan, heart rate, hemoglobin, control of breathing
Citation: Moya EA, Yu JJ, Brown S, Gu W, Lawrence ES, Carlson R, Brandes A, Wegeng W, Amann K, McIntosh SE, Powell FL and Simonson TS (2024) Tibetans exhibit lower hemoglobin concentration and decreased heart response to hypoxia during poikilocapnia at intermediate altitude relative to Han Chinese. Front. Physiol. 15:1334874. doi: 10.3389/fphys.2024.1334874
Received: 07 November 2023; Accepted: 09 April 2024;
Published: 09 May 2024.
Edited by:
Esteban Ortiz-Prado, University of the Americas, EcuadorReviewed by:
Heimo Mairbäurl, Heidelberg University Hospital, GermanyCopyright © 2024 Moya, Yu, Brown, Gu, Lawrence, Carlson, Brandes, Wegeng, Amann, McIntosh, Powell and Simonson. This is an open-access article distributed under the terms of the Creative Commons Attribution License (CC BY). The use, distribution or reproduction in other forums is permitted, provided the original author(s) and the copyright owner(s) are credited and that the original publication in this journal is cited, in accordance with accepted academic practice. No use, distribution or reproduction is permitted which does not comply with these terms.
*Correspondence: E. A. Moya, ZWFtb3lhQHVjc2QuZWR1; T. S. Simonson, dHNpbW9uc29uQGhlYWx0aC51Y3NkLmVkdQ==
Disclaimer: All claims expressed in this article are solely those of the authors and do not necessarily represent those of their affiliated organizations, or those of the publisher, the editors and the reviewers. Any product that may be evaluated in this article or claim that may be made by its manufacturer is not guaranteed or endorsed by the publisher.
Research integrity at Frontiers
Learn more about the work of our research integrity team to safeguard the quality of each article we publish.