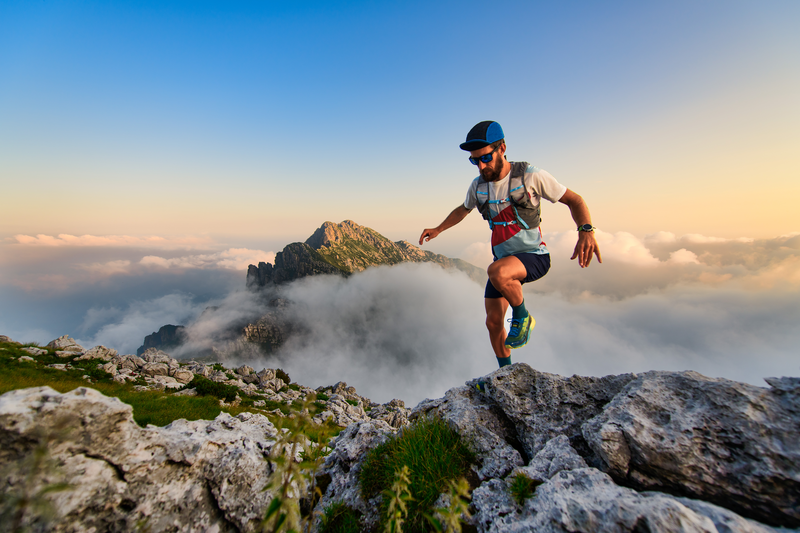
94% of researchers rate our articles as excellent or good
Learn more about the work of our research integrity team to safeguard the quality of each article we publish.
Find out more
EDITORIAL article
Front. Physiol. , 13 November 2023
Sec. Computational Physiology and Medicine
Volume 14 - 2023 | https://doi.org/10.3389/fphys.2023.1322077
This article is part of the Research Topic Recent Advances in the Design and Preclinical Evaluation of Ventricular Assist Devices View all 9 articles
Editorial on the Research Topic
Recent advances in the design and preclinical evaluation of ventricular assist devices
In recent years, ventricular assist devices (VADs, also known as blood pumps) have gradually replaced heart transplantation to provide circulatory support for patients with heart failure in clinical practice (Kormos et al., 2019). However, after the implantation of these VADs, adverse events related to mechanical blood damage, were often reported and have become a major concern during the development of VADs, limiting their clinical and economic benefits (Birschmann et al., 2014; Gurbel et al., 2018). Further efforts are necessitated to improve the performance of VADs by novel designs and evaluation strategies.
Design of VADs must continue to be improved, to optimize the blood-device interaction to reduce blood damage. Various preclinical evaluation approaches have been employed: in silico simulations are flexible and inexpensive; during in vitro testing, physical devices can be tested and iteratively improved; in vivo animal trials can evaluate device-body interactions. Evaluation strategies, both separately and in combination should continue to be optimized and implemented to maximize efficacy of testing. Thus, this Research Topic aims at collecting recent advances in the design techniques and evaluation strategies of VADs. We are delighted to summarize the contributions from the eight publications in this Editorial Article:
In recent years, many efforts have been devoted to the optimization of blood pumps (Zhu et al., 2010; Chen et al., 2013; Wiegmann et al., 2018; Wu et al., 2021). Nonetheless, most studies focused on the design of the impeller of conventional centrifugal and axial blood pumps. The percutaneous ventricular assist devices (pVADs) are advanced treatment with the advantages of rapid implantation, less invasive, low rates of complications, and easy nursing. Yun et al. optimized the outflow structures of a pVAD using experiments and CFD, and proposed four outflow structures considering features such as blades, shape of cage bridges. The results showed that outflow structure with curved bridges and bladeless diffuser has an overall lower level of shear stress and hemolysis.
In vitro hemocompatibility testing is a critical step before clinical trials of blood pumps. Li et al. investigated the hemocompatibility of five extracorporeal blood pumps with heparinized porcine blood at nominal operating conditions (5 L/min, 160 mmHg) and extreme conditions (1 L/min, 290 mmHg). Hemolysis and the degradation of von Willebrand factor (VWF) were evaluated. It was found that blood damage was significantly more severe at extreme conditions. The results also demonstrated superior hemocompatibility of two maglev pumps over blood pumps with mechanical bearings.
In vitro bench testing for evaluating hydraulic and blood damage performance of VADs, and their physiological interactions with cardiovascular system is vital during the development of VADs. The mock circulatory loop (MCL) is an in vitro experimental system that can provide continuous pulsatile flows and simulate different behaviors of human circulation system. Xu et al. reviewed the applications of MCL for testing prosthetic heart valve, VAD, total artificial heart and intra-aortic balloon pump, as well as the pediatric MCLs. This review also pointed out that MCL should be flexibly modularized and adjusted for testing different types of mechanical circulatory support devices, in combination with more advanced measurement methods. Suction is one of the adverse events during the clinical application of VADs which may cause damage to both the patients and medical device. Rocchi et al. validated a suction module (SM) as a test bench for LVAD suction detection and speed control algorithms. The SM was implemented into a hybrid in vitro-in silico cardiovascular simulator. Results showed that the simulator could reproduce most of the pump waveforms observed in vivo, and can be used to investigate suction in different conditions.
CFD has become an important tool for the design, optimization and evaluation of blood pumps (Huo et al., 2021; Wu et al., 2022). Gao et al. investigated the effect of VADs on the hemodynamics in the ascending aorta. Results showed that vorticity intensity under LVAD was significantly higher than that patients without the support of LVAD and the hemodynamics in the ascending aorta were similar to that of a healthy condition. These findings contribute to a more comprehensive understanding of the clinical efficacy of LVAD. Huang et al. investigated the hemolysis performance of a VAD under different wave speed modulation profiles using CFD, coupled with a cardiovascular lumped parameter model. The results reveal that speed modulations slightly affect the pump hemolysis, and the hemolysis differences between the different speed modulation profiles were insignificant. This study suggests that speed modulations could be a feasible way to improve the flow pulsatility of blood pumps while not increasing hemolysis.
Typical hemolysis models consider hemolysis as a power-law function of equivalent stress and exposure time (Heuser and Opitz, 1980; Giersiepen et al., 1990; Zhang et al., 2011; Wu et al., 2019). Nonetheless, stress-based models cannot account for blood damage at cellular level. Thus, hemolysis models accounting for effects at cellular or mesoscopic level are necessitated. Xu et al. employed the coarse-grained hemolysis model at the mesoscopic scale based on the transport dissipative particle dynamics method, to predict hemolysis in an axial blood pump. Results showed that the rate of shear stress is the most critical factor in the hemolysis occurring in the rotor region. Wang et al. proposed a hemolysis model considering the aging of blood cells. The critical value of shear stress for the damage of red blood cells was determined through simulating the movement of a single RBC in a shear flow field at the mesoscale. The model was verified against experimental hemolysis results. The results showed that compared with conventional stress-based hemolysis models, the proposed hemolysis model reduced the error of the hemolysis predictions.
PW: Writing–original draft, Writing–review and editing. ZW: Writing–review and editing. HC: Writing–review and editing. ZC: Writing–review and editing. XZ: Writing–review and editing. MY: Writing–review and editing.
The authors declare financial support was received for the research, authorship, and/or publication of this article. This work was supported by the National Natural Science Foundation of China (Grant No. 12072216), and the Mobility Program of the Sino-German Center for Research Promotion (Grant No. M-0231).
The authors declare that the research was conducted in the absence of any commercial or financial relationships that could be construed as a potential conflict of interest.
All claims expressed in this article are solely those of the authors and do not necessarily represent those of their affiliated organizations, or those of the publisher, the editors and the reviewers. Any product that may be evaluated in this article, or claim that may be made by its manufacturer, is not guaranteed or endorsed by the publisher.
Birschmann I., Dittrich M., Eller T., Wiegmann B., Reininger A. J., Budde U., et al. (2014). Ambient hemolysis and activation of coagulation is different between HeartMate II and HeartWare left ventricular assist devices. J. Heart Lung Transplant. 33 (1), 80–87. doi:10.1016/j.healun.2013.11.010
Chen Z. S., Yao Z. H., Zhu L. L., Zhang X. W. (2013). Hemolysis analysis of axial blood pumps with various structure impellers. J. Mech. Med. Biol. 13 (4), UNSP1350054. doi:10.1142/s0219519413500541
Giersiepen M., Wurzinger L. J., Opitz R., Reul H. (1990). Estimation of shear stress-related blood damage in heart valve prostheses-in vitro comparison of 25 aortic valves. Int. J. Artif. organs 13 (5), 300–306. doi:10.1177/039139889001300507
Gurbel P., Shah P., Desai S., Tantry U. (2018). Antithrombotic strategies and device thrombosis. Cardiol. Clin. 36 (4), 541–550. doi:10.1016/j.ccl.2018.06.008
Heuser G., Opitz R. A. (1980). A Couette viscometer for short-time shearing of blood. Biorheology 17, 17–24. doi:10.3233/BIR-1980-171-205
Huo J. D., Wu P., Zhang L. D., Wu W. T. (2021). Large eddy simulation as a fast and accurate engineering approach for the simulation of rotary blood pumps. Int. J. Artif. Organs 44 (11), 887–899. doi:10.1177/03913988211041636
Kormos R. L., Cowger J., Pagani F. D., Teuteberg J. J., Goldstein D. J., Jacobs J. P., et al. (2019). The Society of Thoracic Surgeons Intermacs database annual report: evolving indications, outcomes, and scientific partnerships. J. Heart Lung Transplant. 38 (2), 114–126. doi:10.1016/j.healun.2018.11.013
Wiegmann L., Boës S., de Zélicourt D., Thamsen B., Schmid Daners M., Meboldt M., et al. (2018). Blood pump design variations and their influence on hydraulic performance and indicators of hemocompatibility. Ann. Biomed. Eng. 46, 417–428. doi:10.1007/s10439-017-1951-0
Wu P., Gao Q., Hsu P. L. (2019). On the representation of effective stress for computing hemolysis. Biomech. Model Mechanobiol. 18 (3), 665–679. doi:10.1007/s10237-018-01108-y
Wu P., Huo J. D., Dai W. F., Wu W. T., Yin C. K., Li S. (2021). On the optimization of a centrifugal maglev blood pump through design variations. Front. Physio 12, 699891. doi:10.3389/fphys.2021.699891
Wu P., Huo J. D., Zhang Z. J., Wang C. J. (2022). The influence of non-conformal grid interfaces on the results of large eddy simulation of centrifugal blood pumps. Artif. Organs 46 (9), 1804–1816. doi:10.1111/aor.14263
Zhang T., Taskin M. E., Fang H. B., Pampori A., Jarvik R., Griffith B. P., et al. (2011). Study of flow-induced hemolysis using novel Couette-type blood shearing devices. Artif. organs 35 (12), 1180–1186. doi:10.1111/j.1525-1594.2011.01243.x
Keywords: ventricular assist devices, blood pumps, blood damage, CFD, in-vitro testing
Citation: Wu P, Wu ZJ, Chen H, Chen Z, Zhang X and Yang M (2023) Editorial: Recent advances in the design and preclinical evaluation of ventricular assist devices. Front. Physiol. 14:1322077. doi: 10.3389/fphys.2023.1322077
Received: 15 October 2023; Accepted: 16 October 2023;
Published: 13 November 2023.
Edited and reviewed by:
Raimond L. Winslow, Northeastern University, United StatesCopyright © 2023 Wu, Wu, Chen, Chen, Zhang and Yang. This is an open-access article distributed under the terms of the Creative Commons Attribution License (CC BY). The use, distribution or reproduction in other forums is permitted, provided the original author(s) and the copyright owner(s) are credited and that the original publication in this journal is cited, in accordance with accepted academic practice. No use, distribution or reproduction is permitted which does not comply with these terms.
*Correspondence: Peng Wu, cGVuZy53dTA3QGdtYWlsLmNvbQ==
Disclaimer: All claims expressed in this article are solely those of the authors and do not necessarily represent those of their affiliated organizations, or those of the publisher, the editors and the reviewers. Any product that may be evaluated in this article or claim that may be made by its manufacturer is not guaranteed or endorsed by the publisher.
Research integrity at Frontiers
Learn more about the work of our research integrity team to safeguard the quality of each article we publish.