- Food Biotechnology Research Team, National Center for Genetic Engineering and Biotechnology, Pathum Thani, Thailand
1 Introduction
Chicken meat, particularly the breast portion, offers high-quality protein, providing adequate amounts of all of the essential amino acids with a Protein Digestibility Corrected Amino Acid Score value ranging between 0.91 and 0.95 (Boye et al., 2012). Among land animal meats, chicken breast is fairly low in lipid and collagen which is more favorable for protein digestibility (Barrón-Hoyos et al., 2013; Marangoni et al., 2015). Global demand for poultry meat has been steadily rising and it has been projected at 145 tons by 2029, with chicken expected to account for 50% of the meat consumed (USDA, 2021). A steady increase in chicken breast meat consumption within the next decade has been predicted although alternative proteins, e.g., insect and plant-based proteins, has gained increasing attention. The main reason behind its high demand is its affordable price for all classes of consumers. In addition, for the past decades, meat consumption has shifted from predominantly red meat to white meat as a healthier choice.
For the past decades, commercial broilers have been intensively selected through a breeding selection for production efficiency to meet high consumer demand (Barbut and Leishman, 2022). The success in breeding selection, however, has coincided with increased abnormalities among broilers, including growth-related myopathies, namely, White striping (WS), Wooden breast (WB) and Spaghetti meat (Barbut, 2019; Petracci et al., 2019; Soglia et al., 2019; Barbut and Leishman, 2022). These myopathies can be found together or individually in all broiler chicken breeds with a large variation in occurrence and severity across global regions (Lorenzi et al., 2014; Malila et al., 2018; Barbut, 2019; Soglia et al., 2019; Che et al., 2022).
The issue of growth-related myopathies in broilers are globally recognized among poultry community. The industry has found an increasing prevalence of those abnormalities in the past decade with mild WS as the “new norm” of chicken breast meat. Originally found only in the breast (Pectoralis major), occurrence of WS and WB has now been observed in other cuts, including chicken filet (Pectoralis minor) and thigh (Petracci et al., 2019). A number of studies previous investigated the approaches, including selecting the hybrids with slower growth rate (Gratta et al., 2019), slowing the growth with manipulating the amino acids in the feed (Meloche et al., 2018; Zampiga et al., 2019; Lackner et al., 2022), and terminating the birds at the younger ages (Abreu et al., 2022) with an attempt to reduce the prevalence of the myopathies. Although the previous studies addressed the experimental reduction of the myopathies, the issue at the industrial scale does still exist.
2 An association between growth-related myopathies in broilers and occurrence of in vivo oxidative stress
The actual etiology of the myopathy is still under investigation. Yet, previous histological studies consistently show chronic muscle fiber damage as shown by accumulated macrophages, large-rimmed vacuoles, nuclei internalization, deposition of adipocytes, thickened endomysium and perimysium, inconsistent size of rounded myofibers, infiltration of lymphocytes and macrophages, and necrosis in the affected muscles (Kuttappan et al., 2012; Sihvo et al., 2014; Papah et al., 2017; Malila et al., 2018; Salles et al., 2019; Hosotani et al., 2020; Praud et al., 2020). The occurrence of fiber necrosis, fibrosis and adipose tissue filtration increased as the severity degree of the myopathies increased (Praud et al., 2020). The muscle fiber damage has been hypothesized as an adverse consequence when the muscle fibers were outgrown their supportive systems, particularly vascularization (Mutryn et al., 2015; Alnahhas et al., 2016; Kindlein et al., 2017; Papah et al., 2017; Sihvo et al., 2018; Lake and Abasht, 2020). In male Cobb 500 broilers, an increased intercapillary distances together with reduced ratio of capillary to muscle fibers were correlated with WB severity level (Kindlein et al., 2017). The early pathogenesis of WB was likely associated with endothelial cell dysfunction, particularly in the capillaries and venous ends of the vasculature (Abasht et al., 2021). In the breast muscle of commercial broilers, multifocal perivascular and perivenous aggregates of lipid-laden macrophages were observed at 1 week of age prior to the development of myopathic lesions at 2 weeks of age (Papah et al., 2017). Limited oxygenation (Kindlein et al., 2017) in combination with the sequellae of phlebitis and impaired venous drainage (Papah et al., 2017; Abasht et al., 2021) may lead to local accumulation of metabolic waste and reactive oxygen species (ROS), triggering muscle fiber degeneration. In addition, lipid accumulation in the affected Pectoralis major, resemblance to type 2 diabetes, may exert cellular stress to the cells and further suppress glycolysis and gluconeogenesis in the affected birds (Lake and Abasht, 2020). Accumulated lipids can enhance oxidative stress through the lipid peroxidation of fatty acids (Li et al., 2022). In addition, ROS can readily react with other biomolecules, particularly lipids, proteins and DNA (Min and Ahn, 2005). Malondialdehyde, a product of lipid oxidation, has been shown to be responsible for secondary carbonylation of myoglobin and myofibrillar proteins along with cross-linking of myofibrillar proteins from rabbit skeletal muscle (Wang et al., 2020; Yin et al., 2022).
Differential gene expression patterns associated with development of growth-related myopathies suggested alteration of several biological processes, including metabolisms of nutrients, programmed cell death, to muscle regeneration (Mutryn et al., 2015; Zambonelli et al., 2016; Malila et al., 2020). Among the key stress-related transcription factors, transcript abundance of hypoxia-inducible factor 1 (HIF-1), particularly alpha subunit (HIF1A), along with antioxidant enzymes, particularly superoxide dismutase isoform 2 and 3, were increased in the myopathic muscles (Malila et al., 2019; Marchesri et al., 2019; Malila et al., 2022). The findings implied molecular activities against cellular oxidative stress. However, chronic hypoxia within the affected breast muscle appeared to weaken HIF signaling and disrupt the processes of autophagy and mitophagy (Hosotani et al., 2020). In turn, such pathological condition attenuated adaptability of the muscle to hypoxia. The stress environment might trigger aberrant activity of fibro-adipogenic progenitors, resulting in fibrosis (Malila et al., 2022). Metabolic intermediates, i.e., fumarate, and malate, from tricarboxylic acid cycle, were accumulated in the affected breast muscles, suggesting the back flux of oxaloacetate converted into malate and fumarate under limited oxygenation condition (Boerboom et al., 2018). An increased conversion of L-arginine into citrulline was observed in WS breast muscle, presumably to produce nitric oxide (Boerboom et al., 2018). When the level of nitric oxide was elevated, tyrosine residue on polypeptide chains could undergo oxidation, resulting in nitrotyrosine which was associated with inflammation diseases (Cai and Yan, 2013).
3 Potential effects of consuming oxidized lipids and proteins
Although whether consumption of oxidized lipids and proteins would cause any chronic diseases in human is still inconclusive, a growing evidence demonstrated that diets containing excessive oxidative products showed potential to disturb in vivo cell redox status (Estévez and Luna, 2017; Estévez and Xiong, 2019; Hu et al., 2021). The chronic oxidative stress is not only responsible for the virulence and severity of the disease but also the oxidative DNA damage of the epithelial cells (Hardbower et al., 2013). Damages of DNA may interrupt transcription leading to the aberrant cellular response to the oxidative stress or the obtain of erroneous protein structure and functions (Huang and Anh, 2019). Unless the damage to DNA is repaired, it can induce long-term physiological conditions, including inflammation, atherosclerosis, aging and cancer (Huang and Anh, 2019). The intake of high-fat diets and oxidized lipids has been known to be associated with pathological conditions (Estévez and Xiong, 2019). Previous studies in animal models suggested that consumption of diet containing oxidized oils elevated the risk of cellular oxidative stress (Dalle-Donne et al., 2006). An association between 4-hydroxyhexenal, a lipid peroxidation product, and the progression of Alzheimer’s disease was addressed (Bradley et al., 2012).
In contrast to oxidized lipids, the investigation regarding impacts of consuming dietary oxidized proteins have recently gained attention (Estévez and Xiong, 2019). As reviewed by Soladoye et al. (2015), Estévez and Xiong, (2019), and Domínguez et al. (2022), an accumulation of oxidized proteins and their products (e.g., heterocyclic aromatic amines or advanced-glycation end products) was linked with pathological conditions of certain diseases (e.g., Parkinson’s, Alzheimer’s, type II diabetes, and renal failure). Protein carbonylation, an irreversible modification associated with oxidative damage, has been widely used as a biomarker for protein oxidation (Cai and Yan, 2013). The modification occurs on multiple amino acid residues on selected protein targets, including arginine, histidine, lysine, proline, threonine and cysteine (Soladoye et al., 2015). Hence, quantity and quality of essential amino acids were reduced (Soladoye et al., 2015). Gut proteases may not recognize the target sites on the oxidized proteins, leading to reduced protein digestibility and bioavailability (Soladoye et al., 2015). Protein carbonyls may induce polymerization among the oxidized proteins or between their derivatives and other polypeptide chains. On the contrary, in severe condition, the carbonyls can also attack the peptide backbone, resulting in breakdown of the polypeptides into several carbonyl-containing peptides (Stadtman and Levine, 2003). In addition, lipid-derived protein carbonyls can promote a pro-oxidative environment in the muscle tissue. An example was the role of 4-hydroxynonenal in the formation of formation of protein adducts in heart, liver and skeletal muscle of rats (Keller et al., 2020).
4 Health consequences of consuming growth-related myopathies in chicken breast meat: should it be concerned?
Previous studies consistently demonstrated a decreased proportion of protein and increased fat in raw chicken breast meat severely affected with WS and WB condition (Petracci et al., 2014; Soglia et al., 2016; Baldi et al., 2018; Malila et al., 2018; Soglia et al., 2018; Adabi and Suncu, 2019; Mudalal, 2019; Carvalho et al., 2021). The deviation of chemical composition can shift energy distribution of the affected chicken breast meat towards energy contribution from fat (Petracci et al., 2014). The change was more pronounced as the severity elevated. Profile of essential amino acids were also altered in the myopathic chicken meat (Adabi and Suncu, 2019; Soglia et al., 2019; Dalle Zotte et al., 2020; Thanatsang et al., 2020). Proteins might undergo degradation into free amino acids in the affected breast (Soglia et al., 2019) which might be potentially lost with dripping and purging fluids during storage and cooking, respectively. In addition, antemortem oxidative stress condition would result in increased ROS and free radicals promoting oxidation of lipids and proteins in food (Estévez and Xiong, 2019). Given that growth-related myopathies were associated with oxidative stress (Thanatsang et al., 2020; Li et al., 2022), one may assume that when chicken meat with high severity of WS and WB abnormalities was consumed, consumers might increase risk of an expose to oxidized lipids and proteins (Figure 1). However, no investigation has been conducted on such aspect.
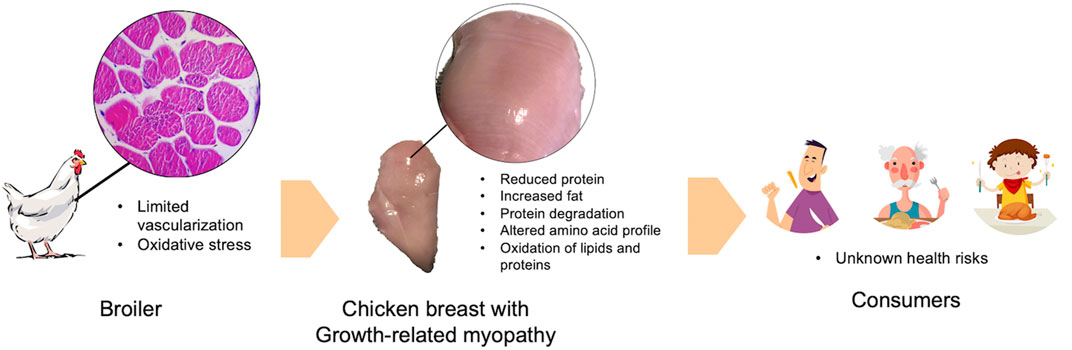
FIGURE 1. A schematic diagram depicts an association between growth-related myopathies and oxidative stress. Impacts of growth-related myopathies and the assumption of health consequence are included.
So far, because the issue of growth-related myopathies has been emerged for about a decade, the published research has been emphasized on the impacts on technological properties and underlying their etiology with the best attempt to establish effective solutions. Apart from those aforementioned altered macronutrients, other health consequences due to consumption of those growth-related myopathies have not been investigated. Whether such altered macronutrients would significantly exert health impacts remains unclear. It is worth noting that in most countries, the severe WB are rejected and only focal mild cases are utilized as human food. Additionally, muscle damages were widely detected on the superficial part of the affected breast and the lesions were less pronounced at the deeper regions (Sihvo et al., 2014; Soglia et al., 2017; Baldi et al., 2018); hence, it is reasonable to hypothesize that consumption of the whole breast meat may less likely exert any adverse health consequences. However, media and internet began to criticize chicken breasts with growth-related myopathies. Such information may someday gain wide attention and eventually exert any negative perception and fear towards chicken meat and poultry industry. Therefore, it is essential that scientific community begins to gather the reliable scientific evidence regarding the influence of growth-related myopathies on protein quality, protein bioavailability and health impact particularly among susceptible consumers (e.g., elderlies and patients) at a long-term exposure.
Author contributions
The author confirms being the sole contributor of this work and has approved it for publication.
Funding
The author(s) declare financial support was received for the research, authorship, and/or publication of this article. This project was funded by the National Research Council of Thailand (NRCT) and National Center for Genetic Engineering and Biotechnology (BIOTEC, Thailand), National Science and Technology Development Agency (NSTDA, Thailand) with project number of N42A660312.
Conflict of interest
The author declares that the research was conducted in the absence of any commercial or financial relationships that could be construed as a potential conflict of interest.
Publisher’s note
All claims expressed in this article are solely those of the authors and do not necessarily represent those of their affiliated organizations, or those of the publisher, the editors and the reviewers. Any product that may be evaluated in this article, or claim that may be made by its manufacturer, is not guaranteed or endorsed by the publisher.
References
Abasht B., Papah M. B., Qiu J. (2021). Evidence of vascular endothelial dysfunction in Wooden Breast disorder in chickens: insights through gene expression analysis, ultra-structural evaluation and supervised machine learning methods. PLoS One 16 (1), e0243983. doi:10.1371/journal.pone.0243983
Abreu A. R. C., Araújo I. C. S., Vaz D. P., Saldanha M. M., Fontes D. O., Leão P. A., et al. (2022). Performance, nutrient digestibility, and muscular evaluation of female broiler chickens fed different dietary protein levels and slaughtered at 38 or 46 days. Rev. Bras. Zootec. 51, e20210151. doi:10.37496/rbz5120210151
Adabi S. G., Soncu E. D. (2019). White striping prevalence and its effect on meat quality of broiler breast fillets under commercial conditions. J. Anim. Physiol. Anim. Nutr. 103 (4), 1060–1069. doi:10.1111/jpn.13092
Alnahhas N., Berri C., Chabault M., Chartrin P., Boulay M., Bourin M. C., et al. (2016). Genetic parameters of white striping in relation to body weight, carcass composition, and meat quality traits in two broiler lines divergently selected for the ultimate pH of the pectoralis major muscle. BMC Genet. 17, 61. doi:10.1186/s12863-016-0369-2
Baldi G., Soglia F., Mazzoni M., Sirri F., Canonico L., Babini E., et al. (2018). Implications of white striping and spaghetti meat abnormalities on meat quality and histological features in broilers. Animal 12 (1), 164–173. doi:10.1017/S1751731117001069
Barbut S. (2019). Recent myopathies in broiler's breast meat fillets. Poult. Sci. J. 75, 559–582. doi:10.1017/S0043933919000436
Barbut S., Leishman E. M. (2022). Quality and processability of modern poultry meat. Animals 12 (20), 2766. doi:10.3390/ani12202766
Barrón-Hoyos J. M., Archuleta A. R., Refugio F. M., Canett-Romero R., Cinco-Moroyoqui F. J., Romero-Barancini A. L., et al. (2013). Protein Quality Evaluation of Animal Food Proteins by <i&gt;In-Vitro&lt;/i&gt; Methodologies. Nutr. Food Sci. 4, 376–384. doi:10.4236/fns.2013.44048
Boerboom G., van Kempen T., Navarro-Villa A., Pérez-Bonilla A. (2018). Unraveling the cause of white striping in broilers using metabolomics. Poult. Sci. 97 (11), 3977–3986. doi:10.3382/ps/pey266
Boye J., Wijesinha-Bettoni R., Burlingame B. (2012). Protein quality evaluation twenty years after the introduction of the protein digestibility corrected amino acid score method. Br. J. Nutr. 108, S183–S211. doi:10.1017/S0007114512002309
Bradley M. A., Xiong-Fister S., Markesbery W. R., Lovell M. A. (2012). Elevated 4-hydroxyhexenal in Alzheimer's disease (AD) progression. Neurobiol. Aging. 33 (6), 1034–1044. doi:10.1016/j.neurobiolaging.2010.08.016
Cai Z., Yan L. J. (2013). Protein oxidative modifications: beneficial roles in disease and health. J. Biochem. Pharmacol. Res. 1 (1), 15–26.
Carvalho L. T., Owens C. M., Giampietro-Ganeco A., Malagoli de Mello J. L., Ferrari F. B., de Carvalho F. A. L., et al. (2021). Quality of turkeys breast meat affected by white striping myopathy. Poult. Sci. 100 (4), 101022. doi:10.1016/j.psj.2021.101022
Che S., Wang C., Varga C., Barbut S., Susta L. (2022). Prevalence of breast muscle myopathies (spaghetti meat, woody breast, white striping) and associated risk factors in broiler chickens from Ontario Canada. PLoS One 17, e0267019. doi:10.1371/journal.pone.0267019
Dalle-Donne I., Aldini G., Carini M., Colombo R., Rossi R., Milzani A. (2006). Protein carbonylation, cellular dysfunction, and disease progression. J. Cell Mol. Med. 10 (2), 389–406. doi:10.1111/j.1582-4934.2006.tb00407.x
Dalle Zotte A., Ricci R., Cullere M., Serva L., Tenti S., Marchesini G. (2020). Research Note: effect of chicken genotype and white striping–wooden breast condition on breast meat proximate composition and amino acid profile. Poult. Sci. 99 (3), 1797–1803. doi:10.1016/j.psj.2019.10.066
Domínguez R., Pateiro M., Munekata P. E. S., Zhang W., Garcia-Oliveira P., Carpena M., et al. (2022). Protein oxidation in muscle foods: a comprehensive review. Antioxidants 11, 60. doi:10.3390/antiox11010060
Estévez M., Luna C. (2017). Dietary protein oxidation: a silent threat to human health? Crit. Rev. Food Sci. Nutr. 57 (17), 3781–3793. doi:10.1080/10408398.2016.1165182
Estévez M., Xiong Y. (2019). Intake of oxidized proteins and amino acids and causative oxidative stress and disease: recent scientific evidences and hypotheses. J. Food Sci. 84 (3), 387–396. doi:10.1111/1750-3841.14460
Gratta F., Birolo M., Sacchetto R., Radaelli G., Xiccato G., Ballarin C., et al. (2019). Effect of feed restriction timing on live performance, breast myopathy occurrence, and muscle fiber degeneration in 2 broiler chicken genetic lines. Poult. Sci. 98 (11), 5465–5476. doi:10.3382/ps/pez352
Hardbower D. M., de Sablet T., Chaturvedi R., Wilson K. T. (2013). Chronic inflammation and oxidative stress: the smoking gun for Helicobacter pylori-induced gastric cancer? Gut Microbes 4 (6), 475–481. doi:10.4161/gmic.25583
Hosotani M., Kawasaki T., Hasegawa Y., Wakasa Y., Hoshino M., Takahashi N., et al. (2020). Physiological and pathological mitochondrial clearance is related to pectoralis major muscle pathogenesis in broilers with wooden breast syndrome. Front. Physiol. 11, 579. doi:10.3389/fphys.2020.00579
Hu Y., Zhao G., Zhang M., Zhou D., Zhu B. (2021). Potential adverse health effects of dietary lipid oxidation products. J. Food Bioact. 15. doi:10.31665/JFB.2021.15282
Huang X., Anh D. U. (2019). Lipid oxidation and its implications to meat quality and human health. Food Sci. Biotechnol. 28 (5), 1275–1285. doi:10.1007/s10068-019-00631-7
Keller J., Chevolleau S., Noguer-Meireles M. H., Pujos-Guillot E., Delosière M., Chantelauze C., et al. (2020). Heme-iron-induced production of 4-hydroxynonenal in intestinal lumen may have extra-intestinal consequences through protein-adduct formation. Antioxidants (Basel) 9 (12), 1293. doi:10.3390/antiox9121293
Kindlein L., Ferreira T. Z., Driemeier D., Nascimento V. P., Vieira S. L., Moraes L. E., et al. (2017). Occurrence and severity of white striping in broilers until 50d of age fed with high and low-energy diets: body weight, histopathological changes and meat quality. J. Vet. Sci. Technol. 8, 478. doi:10.4172/2157-7579.1000478
Kuttappan V. A., Brewer V. B., Apple J. K., Waldroup P. W., Owens C. M. (2012). Influence of growth rate on the occurrence of white striping in broiler breast fillets. Poult. Sci. 91 (10), 2677–2685. doi:10.3382/ps.2012-02259
Lackner J., Hess V., Stef L., Sauerwein H. (2022). Effects of feeding different histidine to lysine ratios on performance, meat quality, and the occurrence of breast myopathies in broiler chickens. Poult. Sci. 101, 101568. doi:10.1016/j.psj.2021.101568
Lake J. A., Abasht B. (2020). Glucolipotoxicity: a proposed etiology for wooden breast and related myopathies in commercial broiler chickens. Front. Physiol. 11, 169. doi:10.3389/fphys.2020.00169
Li B., Dong X., Puolanne E., Ertbjerg P. (2022). Effect of wooden breast degree on lipid and protein oxidation and citrate synthase activity of chicken pectoralis major muscle. LWT 154, 112884. doi:10.1016/j.lwt.2021.112884
Lorenzi M., Mudalal S., Cavani C., Petracci M. (2014). Incidence of white striping under commercial conditions in medium and heavy broiler chickens in Italy. J. Appl. Poult. Res. 23, 754–758. doi:10.3382/japr.2014-00968
Malila Y., Thanatsang K., Arayamethakorn S., Uengwetwanit T., Srimarut Y., Petracci M., et al. (2019). Absolute expressions of hypoxia-inducible factor-1 alpha (HIF1A) transcript and the associated genes in chicken skeletal muscle with white striping and wooden breast myopathies. PLoS One 14 (8), e0220904. doi:10.1371/journal.pone.0220904
Malila Y., Thanatsang K. V., Sanpinit P., Arayamethakorn S., Soglia F., Zappaterra H., et al. (2022). Differential expression patterns of genes associated with metabolisms, muscle growth and repair in Pectoralis major muscles of fast- and medium-growing chickens. PLoS One 17 (10), e0275160. doi:10.1371/journal.pone.0275160
Malila Y., U-chupaj J., Srimarut Y., Chaiwiwattrakul P., Uengwetwanit T., Arayamethakorn S., et al. (2018). Monitoring of white striping and wooden breast cases and impacts on quality of breast meat collected from commercial broilers (Gallus gallus). Asian-Australas. J. Anim. Sci. 31 (11), 1807–1817. doi:10.5713/ajas.18.0355
Malila Y., Uengwetwanit T., Arayamethakorn S., Srimarut Y., Thanatsang K. V., Soglia F., et al. (2020). Transcriptional profiles of skeletal muscle associated with increasing severity of white striping in commercial broilers. Front. Physiol. 11, 580. doi:10.3389/fphys.2020.00580
Marangoni F., Corsello G., Cricelli C., Ferrara N., Ghiselli A., Lucchin L., et al. (2015). Role of poultry meat in a balanced diet aimed at maintaining health and wellbeing: an Italian consensus document. Food Nutr. Res. 59, 27606. doi:10.3402/fnr.v59.27606
Marchesi J. A. P., Ibelli A. M. G., Peixoto J. O., Cantão M. E., Pandolfi J. R. C., Marciano C. M. M., et al. (2019). Whole transcriptome analysis of the pectoralis major muscle reveals molecular mechanisms involved with white striping in broiler chickens. Poult. Sci. 98 (2), 590–601. doi:10.3382/ps/pey429
Meloche K. J., Fancher B. I., Emmerson D. A., Bilgili S. F., Dozier W. A. (2018). Effects of reduced dietary energy and amino acid density on Pectoralis major myopathies in broiler chickens at 36 and 49 days of age. Poult. Sci. 97, 1794–1807. doi:10.3382/ps/pex454
Min B., Ahn D. (2005). Mechanism of lipid peroxidation in meat and meat products -a review. Food Sci. Biotechnol. 14, 152–163.
Mudalal S. (2019). Incidence of white striping and its effect on the quality traits of raw and processed Turkey breast meat. Food Sci. Anim. Resour. 39 (3), 410–417. doi:10.5851/kosfa.2019.e35
Mutryn M. F., Brannick E. M., Fu W., Lee W. R., Abasht B. (2015). Characterization of a novel chicken muscle disorder through differential gene expression and pathway analysis using RNA-sequencing. BMC Genomics 16, 399. doi:10.1186/s12864-015-1623-0
Papah M. B., Brannick E. M., Schmidt C. J., Abasht B. (2017). Evidence and role of phlebitis and lipid infiltration in the onset and pathogenesis of Wooden Breast Disease in modern broiler chickens. Avian Pathol. 46, 623–643. doi:10.1080/03079457.2017.1339346
Petracci M., Mudalal S., Babini E., Cavani C. (2014). Effect of white striping on chemical composition and nutritional value of chicken breast meat. Ital. J. Anim. Sci. 13 (1), 3138–3183. doi:10.4081/ijas.2014.3138
Petracci M., Soglia F., Madruga M., Carvalho L., Ida E., Estévez M. (2019). Wooden-breast, white striping, and spaghetti meat: causes, consequences and consumer perception of emerging broiler meat abnormalities. Compr. Rev. Food Sci. Food Saf. 18, 565–583. doi:10.1111/1541-4337.12431
Praud C., Jimenez J., Pampouille E., Couroussé N., Godet E., Le Bihan-Duval E., et al. (2020). Molecular phenotyping of white striping and wooden breast myopathies in chicken. Front. Physiol. 11, 633. doi:10.3389/fphys.2020.00633
Salles G. B. C., Boiago M. M., Silva A. D., Morsch V. M., Gris A., Mendes R. E., et al. (2019). Lipid peroxidation and protein oxidation in broiler breast fillets with white striping myopathy. J. Food Biochem. 43 (4), e12792. doi:10.1111/jfbc.12792
Sihvo H. K., Airas N., Lindén J., Puolanne E. (2018). Pectoral vessel density and early ultrastructural changes in broiler chicken wooden breast myopathy. J. Comp. Pathol. 161, 1–10. doi:10.1016/j.jcpa.2018.04.002
Sihvo H. K., Immonen K., Puolanne E. (2014). Myodegeneration with fibrosis and regeneration in the pectoralis major muscle of broilers. Vet. Pathol. 51, 619–623. doi:10.1177/0300985813497488
Soglia F., Baldi G., Laghi L., Mudalal S., Cavani C., Petracci M. (2018). Effect of white striping on Turkey breast meat quality. Animal 12 (10), 2198–2204. doi:10.1017/S1751731117003469
Soglia F., Gao J., Mazzoni M., Puolanne E., Cavani C., Petracci M., et al. (2017). Superficial and deep changes of histology, texture and particle size distribution in broiler wooden breast muscle during refrigerated storage. Poult. Sci. 96, 3465–3472. doi:10.3382/ps/pex115
Soglia F., Mudalal S., Babini E., Di Nunzio M., Mazzoni M., Sirri F., et al. (2016). Histology, composition, and quality traits of chicken Pectoralis major muscle affected by wooden breast abnormality. Poult. Sci. 95, 651–659. doi:10.3382/ps/pev353
Soglia F., Silva A. K., Lião L. M., Laghi L., Petracci M. (2019). Effect of broiler breast abnormality and freezing on meat quality and metabolites assessed by 1H-NMR spectroscopy. Poult. Sci. 98 (12), 7139–7150. doi:10.3382/ps/pez514
Soladoye O. P., Juarez M. L., Aalhus J. L., Shand P., Estévez M. (2015). Protein oxidation in processed meat: mechanisms and potential implications on human health. Compr. Rev. Food Sci. Food Saf. 14, 106–122. doi:10.1111/1541-4337.12127
Stadtman E., Levine R. (2003). Free radical-mediated oxidation of free amino acids and amino acid residues in proteins. Amino Acids 25, 207–218. doi:10.1007/s00726-003-0011-2
Thanatsang K. V., Malila Y., Arayamethakorn S., Srimarut Y., Tatiyaborworntham N., Uengwetwanit T., et al. (2020). Nutritional properties and oxidative indices of broiler breast meat affected by wooden breast abnormality. Animals 10 (12), 2272. doi:10.3390/ani10122272
USDA (2021). Livestock and poultry: world markets and trade. Available at: https://apps.fas.usda.gov/psdonline/circulars/livestock_poultry.PDF (Accessed April 13, 2021).
Wang J., Clark D. L., Jacobi S. K., Velleman S. G. (2020). Effect of vitamin E and omega-3 fatty acids early posthatch supplementation on reducing the severity of wooden breast myopathy in broilers. Poult. Sci. 99 (4), 2108–2119. doi:10.1016/j.psj.2019.12.033
Yin Y., Zhou L., Cai J., Feng F., Xing L., Zhang W. (2022). Effect of malondialdehyde on the digestibility of beef myofibrillar protein: potential mechanisms from structure to modification site. Foods 11, 2176. doi:10.3390/foods11152176
Zambonelli P., Zappaterra M., Soglia F., Petracci M., Sirri F., Cavani C., et al. (2016). Detection of differentially expressed genes in broiler pectoralis major muscle affected by White Striping – wooden Breast myopathies. Poult. Sci. 95, 2771–2785. doi:10.3382/ps/pew268
Keywords: broilers, chicken meat, myopathy, protein oxidation, oxidative stress
Citation: Malila Y (2023) In vivo oxidative stress associated with growth-related myopathies in chicken and potential health impact: an opinion paper. Front. Physiol. 14:1291323. doi: 10.3389/fphys.2023.1291323
Received: 09 September 2023; Accepted: 24 October 2023;
Published: 02 November 2023.
Edited by:
Kent M. Reed, University of Minnesota Twin Cities, United StatesReviewed by:
Eero Puolanne, University of Helsinki, FinlandCopyright © 2023 Malila. This is an open-access article distributed under the terms of the Creative Commons Attribution License (CC BY). The use, distribution or reproduction in other forums is permitted, provided the original author(s) and the copyright owner(s) are credited and that the original publication in this journal is cited, in accordance with accepted academic practice. No use, distribution or reproduction is permitted which does not comply with these terms.
*Correspondence: Yuwares Malila, eXV3YXJlcy5tYWxAYmlvdGVjLm9yLnRo