- 1Clinical Physiology, Department of Clinical Sciences Lund, Skåne University Hospital, Lund University, Lund, Sweden
- 2Department of Clinical Physiology, Skåne University Hospital, Lund, Sweden
- 3Wallenberg Centre for Molecular Medicine, Lund University, Lund, Sweden
Volume loading of the right ventricle (RV) in patients with atrial septal defect (ASD) and patients with repaired Tetralogy of Fallot (rToF) affects the pumping mechanics of the left ventricle (LV). Intervention of the lesion will relieve the RV volume load however quantifiable impact on exercise capacity, arrhytmias or death are limited. A possible explanation could be remaining effects on the function of the LV. The aim of this study was therefore to investigate if hemodynamics of the LV differs between patients with RV volume load due to ASD or rToF and healthy controls and if they change after intervention. Eighteen patients with ASD, 17 patients with rToF and 16 healthy controls underwent cardiac magnetic resonance imaging (CMR) and maximal exercise test with continuous gas analysis. Reexamination was performed 13 ± 2 months after closure of the ASD in 13 of the patients and 10 ± 4 months after pulmonary valve replacement (PVR) in 9 of the patients with rToF. Non-invasive PV-loops from CMR and brachial pressures were analyzed. Stroke work (SW) and potential energy (PE) increased after ASD closure but not in ToF patients after valve repair. Patients with ASD or rToF had higher contractility and arterial elastance than controls. No major effects were seen in LV energetics or in peak VO2 after ASD closure or PVR. Peak VO2 correlated positively with SW and PE in patients with ASD (r = 0.54, p < 0.05; r = 0.61, p < 0.01) and controls (r = 0.72, p < 0.01; r = 0.53, p < 0.05) to approximately the same degree as peak VO2 and end-diastolic volume (EDV) or end-systolic volume (ESV). In ToF patients there was no correlation between PV loop parameters and peak VO2 even if correlation was found between peak VO2 and EDV or ESV. In conclusion, the LV seems to adapt its pumping according to anatomic circumstances without losing efficiency, however there are indications of persistent vascular dysfunction, expressed as high arterial elastance, which might have impact on exercise performance and prognosis. Future studies might elucidate if the duration of RV volume load and decreased LV filling have any impact on the ability of the vascular function to normalize after ASD closure or PVR.
Introduction
Volume load of the right ventricle (RV) is a common feature in congenital heart disease, primarily in patients with atrial septal defect (ASD) and repaired Tetralogy of Fallot (rToF) with pulmonary regurgitation. In ASD patients there is a left-to-right shunt across the atrial septum and in rToF the pulmonary regurgitation leads to volume overload. The volume-loaded RV secondarily affects the pumping mechanics of the left ventricle (LV) (Broberg et al., 2011; Stephensen et al., 2014; Sjoberg et al., 2018a; Sjoberg et al., 2018b; Stephensen et al., 2018; Sjoberg et al., 2020). Interventional treatment of the underlying lesion, i.e., closing the ASD or repairing the pulmonary valve will in most cases result in a decrease in RV volumes but not all patients will experience increased exercise capacity and the impact on arrhythmias, or death is limited (Suchon et al., 2005; Geva et al., 2014; Ho et al., 2015; Bokma et al., 2018). A possible reason is the effect on the LV hemodynamics from a prolonged dilated RV. The knowledge in this field is limited. Pressure-volume (PV) loops are the “gold standard” for assessment of ventricular function (Suga and Shoukat, 1973), but they are rarely used in clinical routine due to the invasive procedure needed. Also, anatomical variations can make it difficult to correctly assess the ventricular volume in congenital heart disease. We have developed and validated a novel noninvasive technique for PV loops using cardiac magnetic resonance imaging (CMR) and brachial cuff pressure (Seemann et al., 2019; Sjoberg et al., 2021; Arvidsson et al., 2023). Using this non-invasive PV-loop algorithm we recently showed that the LV of patients with ASD and volume-loaded RV have normal ventricular efficiency in contrast to other patient groups with decreased cardiac output such as ischemic heart disease or dilated cardiomyopathy. The method has also been shown to be useful in assessing patients with rToF (Binka et al., 2022). The aim of this study was to determine if LV hemodynamics from PV loop analysis differs in patients with RV volume load due to ASD or rToF, if they change after ASD closure or pulmonary valve replacement (PVR), and if they are related to exercise capacity with the purpose to increase the understanding of heart failure in these patients.
Material and methods
Study design
Patients with rToF with a clinical referral for CMR were prospectively included as previously reported (Sjoberg et al., 2018a; Stephensen et al., 2019). Patients who had progressive right ventricular dilatation with end-diastolic volume >150 mL/m2, and/or symptoms and signs of heart failure (shortness of breath, fatigue, lightheadedness, chest discomfort or pain, high heart rate or arrythmia) underwent surgical PVR and were reexamined 10 ± 4 months postoperatively. Patients with pulmonary stenosis were not considered for the study. Patients with secundum ASD and Qp/Qs > 1.5 based on echocardiography or CMR were examined before transcutaneous ASD closure and 13 ± 2 months postoperatively. Healthy volunteers from a previously reported study (Stephensen et al., 2019) with normal ECG and blood pressure <140/90 mmHg, no cardiovascular medication, and no medical history of cardiovascular or other systemic disease acted as controls. All subjects had brachial cuff pressure measurements at rest and all subjects underwent a cardiopulmonary exercise test with continuous gas analysis for determination of exercise capacity. The study is performed in accordance with the tenets of the Helsinki Declaration (as revised in 2013) and has been approved by the authors’ National Review Board (Nr.: 213–436 and 2010–55).
Cardiac magnetic resonance imaging
Cardiac magnetic resonance imaging was performed in the supine position with retrospective ECG gating using a 1.5 T Achieva (Philips Healthcare, Best, the Netherlands). Seven patients with rToF were examined using a 1.5 T Magnetom Aera (Siemens Healthcare, Erlangen, Germany). For assessment of ventricular volumes, a short-axis balanced steady-state free-precession (bSSFP) cine images covering the entire heart was acquired. Typical imaging parameters were: acquired temporal resolution 45 ms reconstructed to 30 time phases per heart beat; TE/TR 1.4/3 ms; flip angle 60°, slice thickness 8 mm with no gap. Two-dimensional free-breathing, through-plane phase-contrast (PC) flow measurements were performed in the ascending aorta and pulmonary artery to assess effective stroke volume, shunt and pulmonary regurgitation.
Image analysis
Left ventricular endocardial borders were delineated in all timeframes and analyzed using Segment software (http://segment.heiberg.se) with an in-house developed method for PV loop computation (Seemann et al., 2019; Sjoberg et al., 2021). The parameters derived from PV loops are explained in Figure 1.
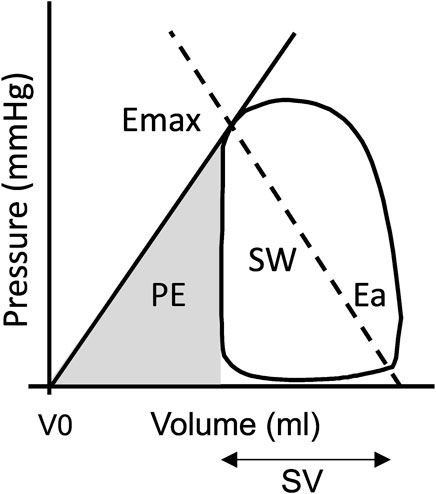
FIGURE 1. A: Schematic pressure-volume (PV) diagram. The PV loop area represents stroke work (SW), the energy produced by the left ventricle (LV) to eject the stroke volume (SV). Mean external power that the LV delivers is calculated as SW*(heart rate/60). Potential energy (PE) is obtained from the triangular area under the end-systolic PV relations curve and to the left of the PV loop. The energy consumption of a heart beat is thus SW + PE, which has been shown to be proportional to LV oxygen consumption [15]. The energy used per ejected volume is calculated as (SW + PE)/SV. SW as a fraction of total energy consumption, SW/(SW + PE), is a measure of ventricular energy efficiency (VE). Myocardial contractility (Emax) can be expressed as the slope of the relationship of end-systolic volume to end-systolic pressure. Arterial elastance (Ea) is the negative slope of the line from maximal ventricular elastance to the end-diastolic volume.
Haemodynamic variables
The hemodynamic variables that can be derived from PV-loop analysis are explained in detail in Figure 1. Stroke work is defined as the area within the PV loop and reflects the energy produced by the left ventricle to eject the stroke volume. The energy stored in the ventricle at the end of systole, and thus “wasted” energy that will be converted to heat, is the potential energy. Stroke work and potential energy adds up to the total energy consumption of a heart beat and has been shown to be proportional to oxygen consumption (Suga, 1979). The total energy consumption divided by the stroke volume shows how much energy is needed to eject a certain volume and stroke work as a fraction of total energy consumption is a measure of the ventricular efficiency. Contractility was defined as the slope between the point of maximal ventricular elastance and the volume at zero pressure, which was fixed to 0, (Sunagawa et al., 1985; Westerhof et al., 2010; Seemann et al., 2019; Sjoberg et al., 2021), and arterial elastance as the negative slope of the line between the point of maximal ventricular elastance on the PV loop and the end-diastolic volume.
Cardiopulmonary exercise test
All participants performed a maximal exercise test with continuous gas analysis (Carefusion, Oxycon Pro, Jaeger, Würzburg, Germany) on a cycle ergometer (939E, Monark, Vansbro, Sweden). The patient performs the test with a face mask applied and the system analyzes oxygen uptake, carbon dioxide elimination, breathing frequency and tidal volumes, using breath by breath technique. The breathing gases are analyzed continuously during the test. The workload was increased until exhaustion, at which point peak oxygen uptake (VO2) was registered. The participants were encouraged to continue until the respiratory exchange ratio was 1.1 or more to ensure maximal exertion. Twelve-lead ECG was continuously recorded, and blood pressure was measured at rest and every minute during exercise. Peak VO2 was presented as milliliters per minute per kilogram, and as percentage of predicted value according to reference values for peak VO2 from the Study of Health in West Pomerania (SHIP) (Gläser 2010).
Statistical analysis
GraphPad (La Jolla, CA; United States) was used for statistics. Continuous variables are presented as mean ± SD or median and interquartile range [IQR] and categorical variables as absolute numbers and percentages. Student’s t-test was used to evaluate differences between subjects. Paired t-tests were used to assess differences in patients before and after PVR or ASD closure. Results with a p-value below 0.05 were considered statistically significant.
Results
Patients’ characteristics are shown in Table 1. Patients with ASD were older than patients with rToF and controls and had a higher proportion of women. Eight patients with ASD (44%) were on medication; 5 on betablockers (28%), 3 on diuretics (17%), one had ACE inhibitor (5%) and one was treated with Flecainide (5%). None of the patients with rToF had any medication due to their heart condition. Both patient groups had lower left ventricular end-diastolic volumes and stroke volumes, higher right ventricular volumes and lower peak oxygen uptake than controls. Patients with rToF had decreased right ventricular ejection fraction in contrast to patients with ASD who had normal ejection fraction.
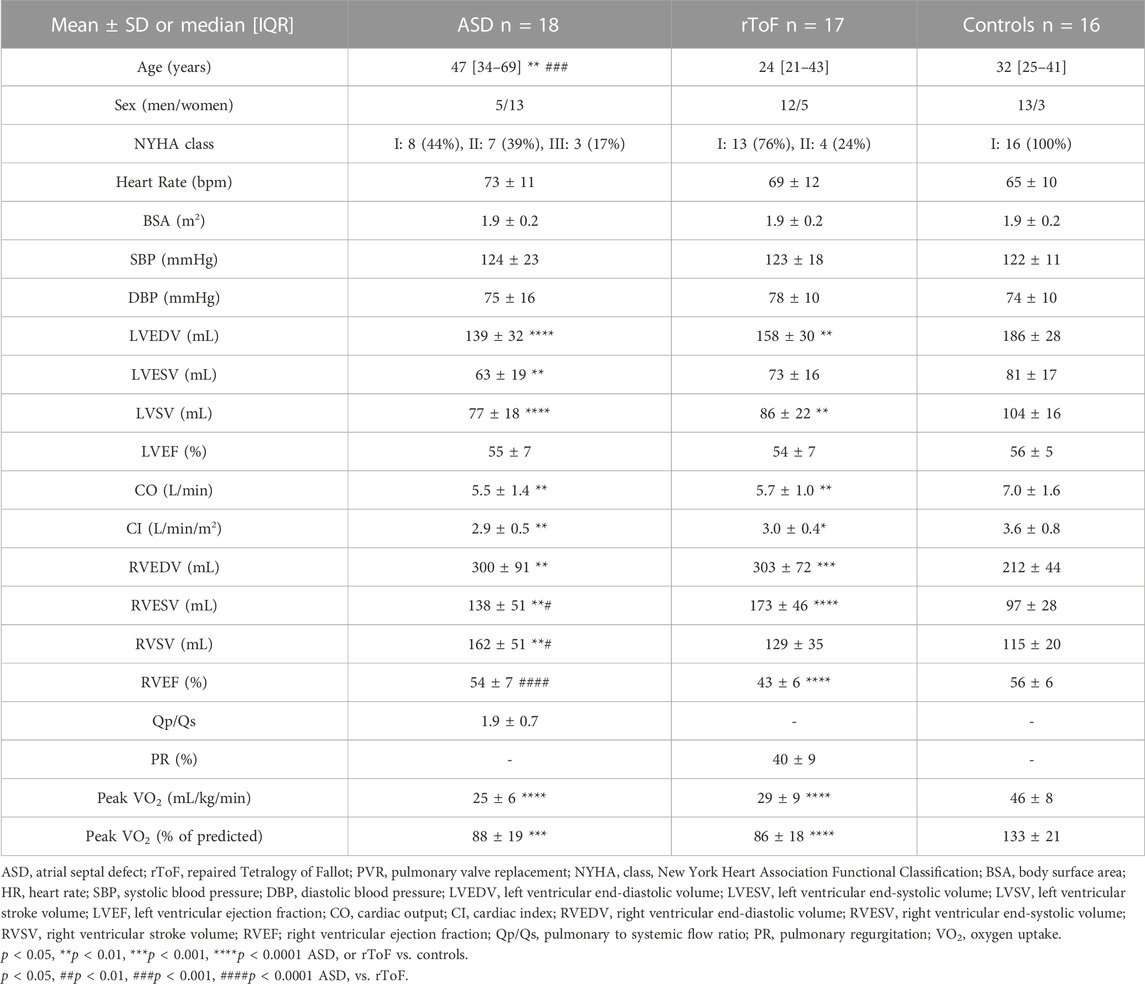
TABLE 1. Characteristics of patients with atrial septal defect and patients with tetralogy of Fallot before transcatheter closure or pulmonary valve replacement and controls.
Table 2 shows the characteristics of patients who received ASD closure or PVR. Patients with ASD increased their left ventricular volumes and decreased their right ventricular volumes after closure of the defect. Patients with rToF had no change in left ventricular volumes after PVR, however right ventricular volumes decreased.
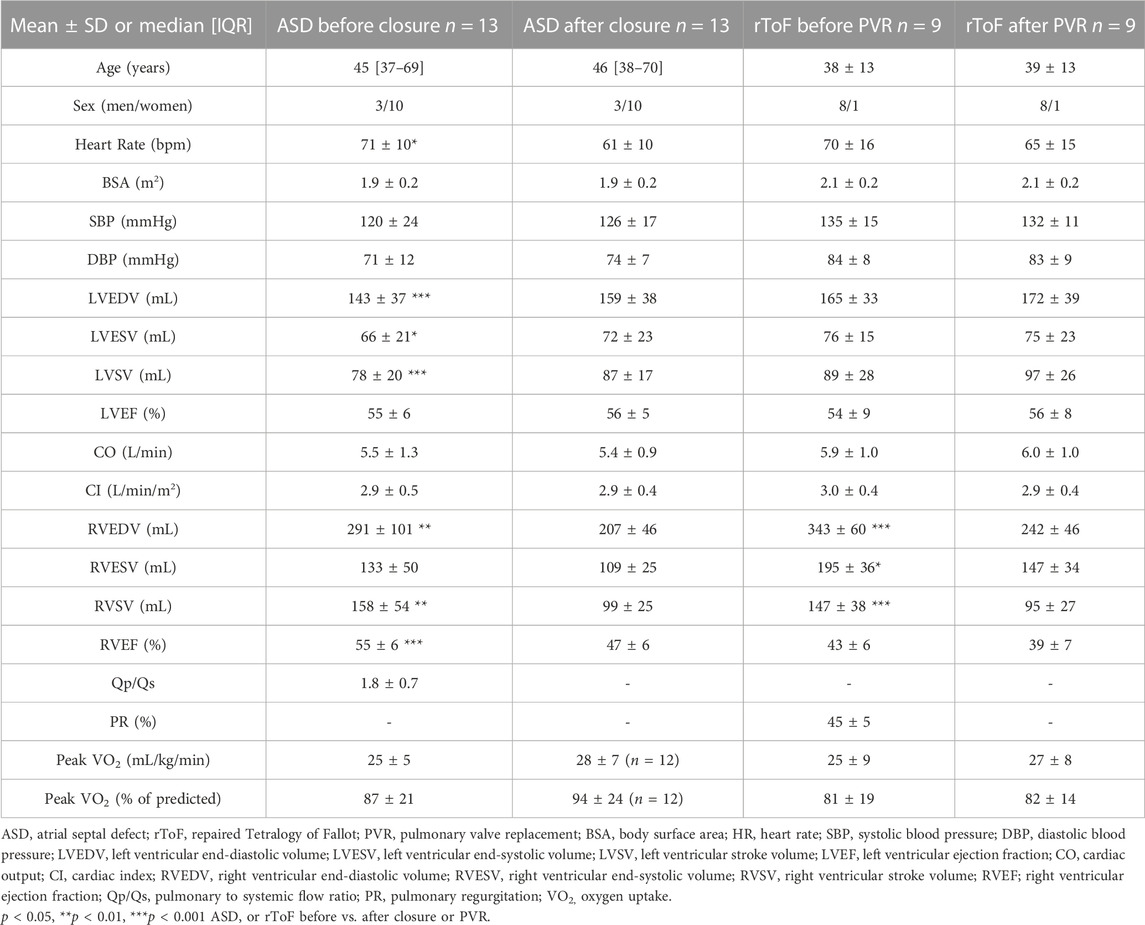
TABLE 2. Characteristics of patients with atrial septal defect and patients with tetralogy of Fallot before and after transcatheter closure or pulmonary valve replacement.
Peak oxygen uptake did not change after ASD closure or PVR. Left ventricular end-diastolic and end-systolic volume correlated with peak VO2 in both patients with ASD and rToF before intervention, as well as in controls, Figure 2.
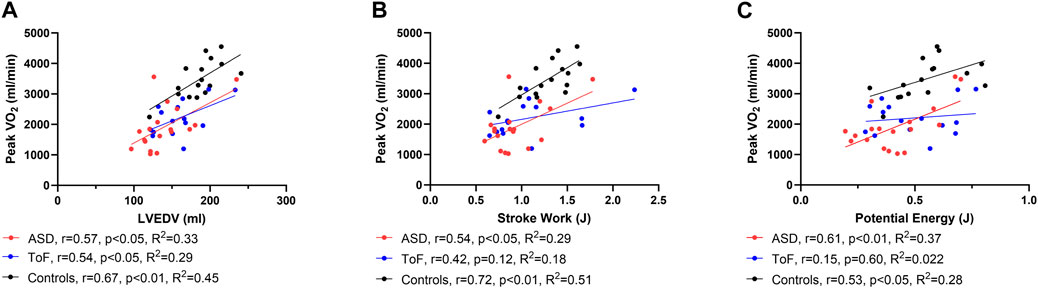
FIGURE 2. Scatter plot showing correlation between left ventricular end-diastolic volume (LVEDV) (A), stroke work (B) and potential energy (C), and peak oxygen uptake (VO2) in patients with atrial septal defect (ASD) and Tetralogy of Fallot (ToF) as well as controls.
Examples of PV loops for patients before and after intervention and controls are shown in Figure 3. The derived hemodynamic parameter values are shown in Tables 3, 4. Patients with ASD had lower stroke work (0.9 ± 0.3 J, p < 0.001) and potential energy (0.4 ± 0.2 J, p = 0.02) than controls (1.3 ± 0.3 J; 0.5 ± 0.1 J) before closure of the defect, and the values increased after closure (1.1 ± 0.3 J, p = 0.02; 0.5 ± 0.2 J, p = 0.04).
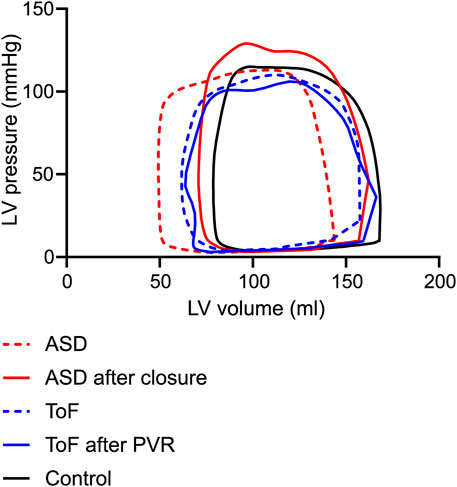
FIGURE 3. Example of PV loops from controls (black), patients with atrial septal defect (ASD) (red) and patients with repaired Tetralogy of Fallot (rToF) (blue). PV loops before ASD closure or pulmonary valve replacement (PVR) are shown with broken line and after with solid line.
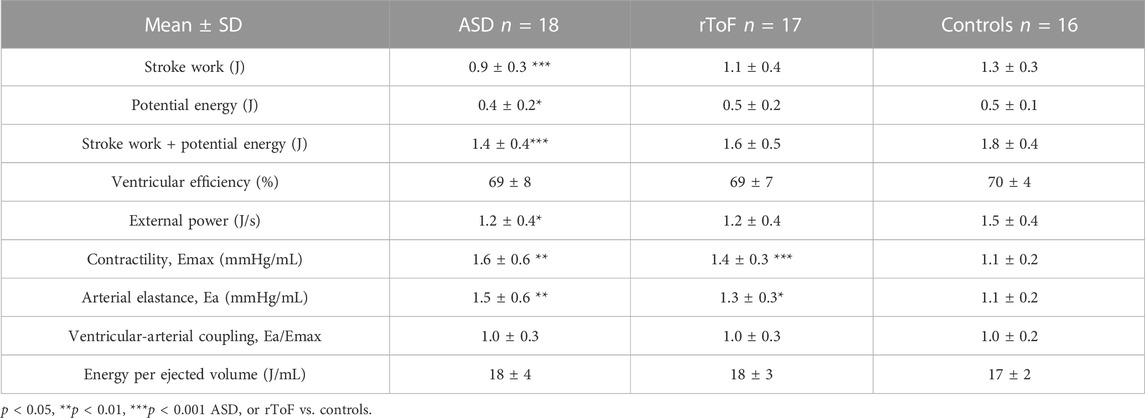
TABLE 3. Hemodynamic parameters in patients with atrial septal defect and patients with tetralogy of Fallot before transcatheter closure or pulmonary valve replacement and controls.
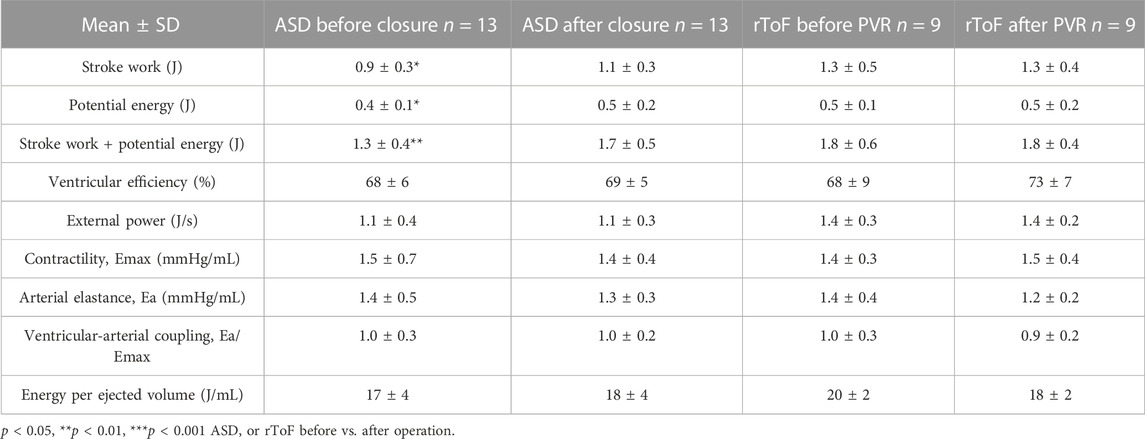
TABLE 4. Hemodynamic parameters in patients with atrial septal defect and patients with tetralogy of Fallot before and after transcatheter closure or pulmonary valve replacement.
Both patients with ASD and rToF had higher contractility (1.6 ± 0.6 mmHg/mL, p = 0.003; 1.4 ± 0.3 mmH/mL, p = 0.021) and arterial elastance (1.5 ± 0.6 mmHg/mL, p = 0.005; 1.3 ± 0.3 mmHg/mL, p = 0.031) before intervention than controls (1.1 ± 0.2 mmHg/mL; 1.1 ± 0.2 mmHg/mL). There was no change in contractility after volume unloading of the RV in either group (ASD: 1.4 ± 0.5 mmHg/mL, rToF: 1.5 ± 0.4). Also, arterial elastance remained unchanged in both patient groups (ASD: 1.3 ± 0.3 mmHg/mL, rToF: 1.2 ± 0.2 mmHg/mL).
There was no difference in energy per ejected volume between patients with ASD or rToF and controls, and no change was noted after ASD closure or PVR.
Left ventricular end-diastolic volume showed a positive correlation with SW, r of 0.56, 0.83 and 0.81 for ASD, rToF and controls respectively. The corresponding determination coefficients (r2) were 0.32, 0.65 and 0.69.
Stroke work and potential energy correlated with peak VO2 in patients with ASD and in controls but not in patients with rToF, Figure 2. There were no correlations between ventricular efficiency, contractility, or energy per ejected volume and peak VO2 in neither patients nor controls.
Discussion
We have shown how LV hemodynamics from pressure volume loops differ between patients with ASD and rTOF and how these parameters are affected by ASD-closure and PVR respectively. The main findings are that 1) patients with ASD as well as rToF patients had normal energy consumption per ejected volume both before and after relieving the volume load of the right ventricle 2) patients with ASD or rToF had increased contractility and arterial elastance, possibly due to increased sympathetic tone and these parameters remained increased 1 year after intervention indicating lasting vascular dysfunction 3) PV loop analysis showed poorer relationship with exercise capacity compared to standard LV volumes.
Ventricular volumes
Closure of the ASD or PVR resulted in a decrease in right ventricular volumes, LV volumes increased after ASD closure but remained unchanged after PVR as earlier reported (Stephensen et al., 2019; Sjoberg et al., 2020). Patients with ASD also showed a decrease in LV ejection fraction, which was normal before intervention, in line with Umemoto et al. (2020). Patients with ASD had lower left ventricular end-diastolic volume, end-systolic volume, stroke volume and cardiac index than controls, because of the left-to-right shunt across the atrial septum causing low left ventricular filling. The larger left ventricular volumes after ASD closure did however not lead to an increased cardiac output, since heart rate decreased. These results are in line with other studies (Teo et al., 2008; Yoshiba et al., 2021). The reason for lower left ventricular end-diastolic volume and stroke volume in patients with rToF compared to controls might be explained by reduced transpulmonary flow caused by the pulmonary regurgitation leading to low left ventricular filling (Kopic et al., 2017; Ylitalo et al., 2018). The LV volumes were however within normal range and the control groups ventricular volumes were towards the upper limit, which might exaggerate the difference. The lack of change in LV volumes after PVR is consistent with Heng et al. (2017).
Energy consumption
The lower energy consumption per heartbeat in patients with ASD is explained by the lower ventricular volumes and stroke volume since patients did not differ from controls in energy per ejected volume. The higher contractility and arterial elastance indicate that patients with ASD likely have an increased inotropic drive at rest compared to controls to preserve cardiac output. After ASD closure, the heart rate decreased, stroke volume increased as did left ventricular energy consumption per heartbeat indicating a lowered sympathetic drive. Energy per ejected volume was however unchanged, likely because of a lower heart rate after ASD closure compared to before as well as an unchanged systolic blood pressure.
Patients with rToF did not differ significantly in left ventricular energy consumption from controls and no change was seen after PVR. Although the number of patients with rToF who underwent PVR is limited the results indicate similar response to decreased filling of the LV as in patients with ASD.
Although the total energy consumption of the left ventricle correlates strongly with myocardial oxygen consumption in studies performed on excised heart ex vivo (Suga, 1979), there are more variability in this relation when assessing human hearts in vivo (Chirinos, 2013). Thus, this must be taken in consideration why it is difficult to draw any clear conclusions of how myocardial oxygen consumption change in the studied patient populations after intervention.
Ventricular-arterial coupling
Contractility, as defined in PV loop analysis, is the slope between V0 and the point on the PV loop where the elastance is maximal (just before the end-systolic volume). The PV loops were displaced more to the left in both patients with ASD and patients with rToF than in controls. Thus, contractility was higher, possibly due to increased inotropy that compensates for low left ventricular filling in these patients. Earlier studies using cardiac catheterization have shown similar results (Senzaki et al., 2001).
The increased inotropy is likely also the reason for the higher arterial elastance, Ea. The ratio between Ea and Emax is used to assess ventricular-arterial coupling (VAC) and is potentially a prognostic marker (Ky et al., 2013; Godfrey et al., 2018). A ratio around or below 1 is thought to be most favorable for the energy expenditure of the heart (Chantler et al., 2008; Chirinos, 2013). The VAC in the patients with volume-loaded RV and, to various extent, underfilled LV in this study was normal. This suggests that the systolic function of the left ventricle is sufficient to compensate for the lower preload thereby upholding sufficient cardiac output, balanced by an increased afterload. This in contrast to patients with acquired heart failure with reduced ejection fraction, where the VAC is high and is prognostic for adverse clinical outcomes (Ky et al., 2013; Ikonomidis et al., 2019).
A speculation is that long lasting increased inotropic state to maintain cardiac output might have long term effects on the arterial endothelium predisposing these patients to cardiovascular disease. In this study we have no direct measure of sympathetic tone and the decrease in heart rate in ASD patients after ASD closure indicates that the sympathetic drive is lower after intervention. However, increased blood pressure, contractility and arterial elastance suggest that the sympathetic tone still remains increased 1 year after intervention. High sympathetic activity reduces arterial distensibility and affects the endothelial function so there is an imbalance between endothelium-derived vasocontriction and vasodilatation (Quarti-Trevano et al., 2021). Studies have shown that adults with rToF or ASD have diminished endothelial function (Scicchitano et al., 2019; Goeder et al., 2022). Patients after heart-transplantation also have increased arterial elastance which has been proposed to play a role in late graft failure (Latus et al., 2023) but the prognostic value has not to our knowledge been studied in patients with ASD or rToF. It is however shown that patients with non-complex CHD have an increased risk of cardiovascular disease, disproportionate to the burden of other known risk factors (Saha et al., 2019). Arterial distensibility and endothelial function is important to tissue perfusion and correlates with exercise performance (Buscemi et al., 2013). This might be a factor adding to why the patients did not improve their exercise capacity after intervention. Studies have shown that the endothelial function can improve after ASD closure (Scicchitano et al., 2019), aortic valve replacement in aortic stenosis patients (Takata et al., 2015) and cardiac resynchronization therapy in heart failure (Santini et al., 2013). Further studies are needed to elucidate if early intervention and thus shorter duration with high sympathetic tone might decrease the risk of cardiovascular disease.
Cardiopulmonary exercise test
Peak VO2 in patients with ASD did not change 12 months after ASD closure in contrast to what has been reported by other groups (Yoshiba et al., 2021). This could be explained by peak VO2 before intervention already being within the normal range of what was expected in contrast to earlier reports that have shown mildly impaired peak oxygen uptake in patients with ASD (Kempny et al., 2012).
Patients with rToF had peak VO2 in the lower normal range expected for their age, however higher compared to other reports rToF (Kempny et al., 2012; Sabate Rotes et al., 2015). Peak VO2 did not increase after surgery, in line with earlier studies (Babu-Narayan et al., 2014).
Peak VO2 in patients with ASD or rToF correlated to a similar degree with left ventricular volumes as in controls but with lower oxygen uptake for the same ventricular size. After intervention with ASD closure or PVR, left ventricular volumes increased in ASD but not in ToF, and regardless of the differences in changes of volumes, peak oxygen uptake did not increase significantly in any group.
Limitations
The method for PV loop estimation requires a non-restrictive pathway from the brachial artery to the LV. None of the participants in the study had any significant aortic stenosis, why this is not judged to affect the results. The volume at the pressure 0 mmHg in the LV is set to be 0 (V0), although it most likely should be a small positive value in a healthy LV. The method has however been validated and shown good agreement with in vivo measurements (Seemann et al., 2019). The V0 could possibly be higher in patients, but in patients with volume-loaded right ventricle it is reasonable to believe that the approximation is still valid. The ventricular end-diastolic pressure also needs to be estimated, but the validation of the method showed low influence on the derived parameters within a range of 0–15 mmHg, and therefore this will probably not affect the results in this study. Controls and patients were not matched for age and sex, and this is acknowledged as a limitation. However, blood pressure, which one could expect to be higher in older persons, was not higher in the ASD group compared to controls and will therefore not affect the PV loop parameters. Finally, since the number of patients is limited, statistical evaluation is difficult. However, the results are congruent with expected physiological response to right ventricular volume load.
Conclusion
The LV seems to adapt its pumping according to the anatomic circumstances in ASD and rToF without losing efficiency. However, there are signs of persistent vascular dysfunction, expressed as high arterial elastance, which might have impact on exercise performance and prognosis after treatment. Future studies might elucidate if the duration of RV volume load and decreased LV filling have any impact on the ability of the vascular function do normalize after ASD closure or PVR.
Scope statement
There is a need to be better at defining when and how to perform intervention in patients with congenital heart disease to improve outcome and wellbeing patients. A prerequisite is to increase the understanding of the pathophysiology. Symptoms in patients with volume-loaded right ventricles due to atrial septal defect or tetralogy of Fallot do not always improve after operation which might be explained by remaining effects on left ventricular function. We thus investigated the hemodynamic response to pulmonary valve replacement or ASD closure by means of non-invasive Pressure-volume loops with cardiac magnetic resonance imaging. We found that the left ventricle seems to adapt its pumping according to anatomic circumstances without losing efficiency, however there are indications of persistent vascular dysfunction, expressed as high arterial elastance, which might have impact on exercise performance and prognosis.
Data availability statement
The datasets presented in this article are not readily available because patient data cannot be made available due to data privacy concerns. Other data will be made available upon reasonable request. Requests to access the datasets should be directed to pia.sjoberg@med.lu.se.
Ethics statement
The studies involving humans were approved by the Regional Ethical Review Board in Lund, Sweden. The studies were conducted in accordance with the local legislation and institutional requirements. The participants provided their written informed consent to participate in this study.
Author contributions
PS: Conceptualization, Data curation, Formal Analysis, Funding acquisition, Investigation, Methodology, Project administration, Visualization, Writing–original draft, Writing–review and editing. SS: Data curation, Writing–review and editing. HA: Investigation, Resources, Writing–review and editing. EH: Funding acquisition, Methodology, Software, Validation, Writing–review and editing. MC: Conceptualization, Funding acquisition, Investigation, Supervision, Writing–review and editing.
Funding
This work was funded by Svenska Läkaresällskapet, Grant/Award Number: 961559; Lunds Universitet; Vetenskapsrådet, Grant/Award Numbers: 2021-04855, 2016-01617; Region Skåne; Hjärt-Lungfonden, Grant/Award Numbers: 20200830, 20210399, 20200653, 20220369; Skånes universitetssjukhus; Marcus och Amalia Wallenbergs minnesfond; Knut och Alice Wallenbergs Stiftelse; Maggie Stephens Stiftelse; Kungliga Fysiografiska Sällskapet i Lund.
Conflict of interest
EH is the founder of Medviso AB, Lund, Sweden, the company that produces the Segment software.
The remaining authors declare that the research was conducted in the absence of any commercial or financial relationships that could be construed as a potential conflict of interest.
Publisher’s note
All claims expressed in this article are solely those of the authors and do not necessarily represent those of their affiliated organizations, or those of the publisher, the editors and the reviewers. Any product that may be evaluated in this article, or claim that may be made by its manufacturer, is not guaranteed or endorsed by the publisher.
References
Arvidsson P. M., Green P. G., Watson W. D., Shanmuganathan M., Heiberg E., De Maria G. L., et al. (2023). Non-invasive left ventricular pressure-volume loops from cardiovascular magnetic resonance imaging and brachial blood pressure: validation using pressure catheter measurements. Eur. Heart J. - Imaging Methods Pract. 1, qyad035–10. doi:10.1093/ehjimp/qyad035
Babu-Narayan S. V., Diller G. P., Gheta R. R., Bastin A. J., Karonis T., Li W., et al. (2014). Clinical outcomes of surgical pulmonary valve replacement after repair of tetralogy of Fallot and potential prognostic value of preoperative cardiopulmonary exercise testing. Circulation 129, 18–27. doi:10.1161/CIRCULATIONAHA.113.001485
Binka E., Zhang J., Seemann F., Jani V., Barnes B., Gaur L., et al. (2022). Biventricular pressure-volume loop assessment before and after pulmonary valve replacement in tetralogy of fallot. J. Thorac. Imaging 37, W70–w71. doi:10.1097/rti.0000000000000665
Bokma J. P., Geva T., Sleeper L. A., Babu Narayan S. V., Wald R., Hickey K., et al. (2018). A propensity score-adjusted analysis of clinical outcomes after pulmonary valve replacement in tetralogy of Fallot. Heart 104, 738–744. doi:10.1136/heartjnl-2017-312048
Broberg C. S., Aboulhosn J., Mongeon F. P., Kay J., Valente A. M., Khairy P., et al. (2011). Prevalence of left ventricular systolic dysfunction in adults with repaired tetralogy of fallot. Am. J. Cardiol. 107, 1215–1220. doi:10.1016/j.amjcard.2010.12.026
Buscemi S., Canino B., Batsis J. A., Buscemi C., Calandrino V., Mattina A., et al. (2013). Relationships between maximal oxygen uptake and endothelial function in healthy male adults: a preliminary study. Acta Diabetol. 50, 135–141. doi:10.1007/s00592-010-0229-x
Chantler P. D., Lakatta E. G., Najjar S. S. (2008). Arterial-ventricular coupling: mechanistic insights into cardiovascular performance at rest and during exercise. J. Appl. Physiol. (1985) 105, 1342–1351. doi:10.1152/japplphysiol.90600.2008
Chirinos J. A. (2013). Ventricular-arterial coupling: invasive and non-invasive assessment. Artery Res. 7, 2. doi:10.1016/j.artres.2012.12.002
Geva T., Martins J. D., Wald R. M. (2014). Atrial septal defects. Lancet 383, 1921–1932. doi:10.1016/s0140-6736(13)62145-5
Godfrey M. E., Rathod R. H., Keenan E., Gauvreau K., Powell A. J., Geva T., et al. (2018). Inefficient ventriculoarterial coupling in fontan patients: a cardiac magnetic resonance study. Pediatr. Cardiol. 39, 763–773. doi:10.1007/s00246-018-1819-6
Goeder D., Oberhoffer-Fritz R., Brudy L., Willinger L., Meyer M., Ewert P., et al. (2022). Diminished endothelial function but normal vascular structure in adults with tetralogy of fallot. J. Clin. Med. 11, 493. doi:10.3390/jcm11030493
Heng E. L., Gatzoulis M. A., Uebing A., Sethia B., Uemura H., Smith G. C., et al. (2017). Immediate and midterm cardiac remodeling after surgical pulmonary valve replacement in adults with repaired tetralogy of fallot: a prospective cardiovascular magnetic resonance and clinical study. Circulation 136, 1703–1713. doi:10.1161/CIRCULATIONAHA.117.027402
Ho J. G., Schamberger M. S., Hurwitz R. A., Johnson T. R., Sterrett L. E., Ebenroth E. S. (2015). The effects of pulmonary valve replacement for severe pulmonary regurgitation on exercise capacity and cardiac function. Pediatr. Cardiol. 36, 1194–1203. doi:10.1007/s00246-015-1143-3
Ikonomidis I., Katsanos S., Triantafyllidi H., Parissis J., Tzortzis S., Pavlidis G., et al. (2019). Pulse wave velocity to global longitudinal strain ratio in hypertension. Eur. J. Clin. Invest. 49, e13049. doi:10.1111/eci.13049
Kempny A., Dimopoulos K., Uebing A., Moceri P., Swan L., Gatzoulis M. A., et al. (2012). Reference values for exercise limitations among adults with congenital heart disease. Relation to activities of daily life--single centre experience and review of published data. Eur. Heart J. 33, 1386–1396. doi:10.1093/eurheartj/ehr461
Kopic S., Stephensen S. S., Heiberg E., Arheden H., Bonhoeffer P., Ersboll M., et al. (2017). Isolated pulmonary regurgitation causes decreased right ventricular longitudinal function and compensatory increased septal pumping in a porcine model. Acta Physiol. (Oxf) 221, 163–173. doi:10.1111/apha.12904
Ky B., French B., May Khan A., Plappert T., Wang A., Chirinos J. A., et al. (2013). Ventricular-arterial coupling, remodeling, and prognosis in chronic heart failure. J. Am. Coll. Cardiol. 62, 1165–1172. doi:10.1016/j.jacc.2013.03.085
Latus H., Raap R., Klingel K., Happel C., Moysich A., Khalil M., et al. (2023). Left ventricular Physiology and ventricular-vascular interactions in young patients after heart transplantation. J. Am. Heart Assoc. 12, e028766. doi:10.1161/jaha.122.028766
Quarti-Trevano F., Seravalle G., Grassi G. (2021). Clinical relevance of the sympathetic-vascular interactions in Health and disease. Biomedicines 9, 1007. doi:10.3390/biomedicines9081007
Sabate Rotes A., Johnson J. N., Burkhart H. M., Eidem B. W., Allison T. G., Driscoll D. J. (2015). Cardiorespiratory response to exercise before and after pulmonary valve replacement in patients with repaired tetralogy of fallot: a retrospective study and systematic review of the literature. Congenit. Heart Dis. 10, 263–270. doi:10.1111/chd.12207
Saha P., Potiny P., Rigdon J., Morello M., Tcheandjieu C., Romfh A., et al. (2019). Substantial cardiovascular morbidity in adults with lower-complexity congenital heart disease. Circulation 139, 1889–1899. doi:10.1161/circulationaha.118.037064
Santini L., Capria A., Di Molfetta A., Mahfouz K., Panattoni G., Minni V., et al. (2013). Endothelial dysfunction is a marker of systemic response to the cardiac resynchronization therapy in heart failure. J. Card. Fail 19, 419–425. doi:10.1016/j.cardfail.2013.05.001
Scicchitano P., Gesualdo M., Cortese F., Acquaviva T., De Cillis E., Bortone A. S., et al. (2019). Atrial septal defect and patent foramen ovale: early and long-term effects on endothelial function after percutaneous occlusion procedure. Heart Vessels 34, 1499–1508. doi:10.1007/s00380-019-01385-2
Seemann F., Arvidsson P., Nordlund D., Kopic S., Carlsson M., Arheden H., et al. (2019). Noninvasive quantification of pressure-volume loops from brachial pressure and cardiovascular magnetic resonance. Circ. Cardiovasc Imaging 12, e008493. doi:10.1161/CIRCIMAGING.118.008493
Senzaki H., Chen C. H., Masutani S., Taketazu M., Kobayashi J., Kobayashi T., et al. (2001). Assessment of cardiovascular dynamics by pressure-area relations in pediatric patients with congenital heart disease. J. Thorac. Cardiovasc Surg. 122, 535–547. doi:10.1067/mtc.2001.115424
Sjoberg P., Bidhult S., Bock J., Heiberg E., Arheden H., Gustafsson R., et al. (2018a). Disturbed left and right ventricular kinetic energy in patients with repaired tetralogy of Fallot: pathophysiological insights using 4D-flow MRI. Eur. Radiol. 28, 4066–4076. doi:10.1007/s00330-018-5385-3
Sjoberg P., Ostenfeld E., Hedstrom E., Arheden H., Gustafsson R., Nozohoor S., et al. (2020). Changes in left and right ventricular longitudinal function after pulmonary valve replacement in patients with Tetralogy of Fallot. Am. J. Physiol. Heart Circ. Physiol. 318, H345–H353. doi:10.1152/ajpheart.00417.2019
Sjoberg P., Seemann F., Arheden H., Heiberg E. (2021). Non-invasive quantification of pressure-volume loops from cardiovascular magnetic resonance at rest and during dobutamine stress. Clin. Physiol. Funct. Imaging 41, 467–470. doi:10.1111/cpf.12718
Sjoberg P., Toger J., Hedstrom E., Arvidsson P., Heiberg E., Arheden H., et al. (2018b). Altered biventricular hemodynamic forces in patients with repaired tetralogy of Fallot and right ventricular volume overload because of pulmonary regurgitation. Am. J. Physiol. Heart Circ. Physiol. 315, H1691–H1702. doi:10.1152/ajpheart.00330.2018
Stephensen S., Steding-Ehrenborg K., Munkhammar P., Heiberg E., Arheden H., Carlsson M. (2014). The relationship between longitudinal, lateral, and septal contribution to stroke volume in patients with pulmonary regurgitation and healthy volunteers. Am. J. Physiol. Heart Circ. Physiol. 306, H895–H903. doi:10.1152/ajpheart.00483.2013
Stephensen S. S., Ostenfeld E., Kutty S., Steding-Ehrenborg K., Arheden H., Thilen U., et al. (2019). Transcatheter closure of atrial septal defect in adults: time-course of atrial and ventricular remodeling and effects on exercise capacity. Int. J. Cardiovasc Imaging 35, 2077–2084. doi:10.1007/s10554-019-01647-0
Stephensen S. S., Ostenfeld E., Steding-Ehrenborg K., Thilen U., Heiberg E., Arheden H., et al. (2018). Alterations in ventricular pumping in patients with atrial septal defect at rest, during dobutamine stress and after defect closure. Clin. Physiol. Funct. Imaging 38, 830–839. doi:10.1111/cpf.12491
Suchon E., Tracz W., Podolec P., Sadowski J. (2005). Atrial septal defect in adults: echocardiography and cardiopulmonary exercise capacity associated with hemodynamics before and after surgical closure. Interact. Cardiovasc Thorac. Surg. 4, 488–492. doi:10.1510/icvts.2004.101451
Suga H. (1979). Total mechanical energy of a ventricle model and cardiac oxygen consumption. Am. J. Physiol. 236, H498–H505. doi:10.1152/ajpheart.1979.236.3.H498
Suga S. K., Shoukat a A., Shoukas A. A. (1973). Load independence of the instantaneous pressure-volume ratio of the canine left ventricle and effects of epinephrine and heart rate on the ratio. Circulation Res. 32, 314–322. doi:10.1161/01.res.32.3.314
Sunagawa K., Maughan W. L., Sagawa K. (1985). Optimal arterial resistance for the maximal stroke work studied in isolated canine left ventricle. Circ. Res. 56, 586–595. doi:10.1161/01.res.56.4.586
Takata M., Amiya E., Watanabe M., Ozeki A., Watanabe A., Kawarasaki S., et al. (2015). Brachial artery diameter has a predictive value in the improvement of flow-mediated dilation after aortic valve replacement for aortic stenosis. Heart Vessels 30, 218–226. doi:10.1007/s00380-014-0475-x
Teo K. S., Dundon B. K., Molaee P., Williams K. F., Carbone A., Brown M. A., et al. (2008). Percutaneous closure of atrial septal defects leads to normalisation of atrial and ventricular volumes. J. Cardiovasc Magn. Reson 10, 55. doi:10.1186/1532-429x-10-55
Umemoto S., Sakamoto I., Abe K., Ishikita A., Yamasaki Y., Hiasa K. I., et al. (2020). Preoperative threshold for normalizing right ventricular volume after transcatheter closure of adult atrial septal defect. Circ. J. 84, 1312–1319. doi:10.1253/circj.CJ-20-0136
Ylitalo P., Jokinen E., Lauerma K., Holmstrom M., Pitkanen-Argillander O. M. (2018). Additional mechanism for left ventricular dysfunction: chronic pulmonary regurgitation decreases left ventricular preload in patients with tetralogy of Fallot. Cardiol. Young 28, 208–213. doi:10.1017/S1047951117001457
Keywords: congenital heart disease, magnetic resonance imaging (MRI), atrial septal defect (ASD), tetralogy of fallot, arterial elastance (Ea), contractility
Citation: Sjöberg P, Stephensen S, Arheden H, Heiberg E and Carlsson M (2023) Patients with volume-loaded right ventricle - quantification of left ventricular hemodynamic response to intervention measured by noninvasive pressure-volume loops. Front. Physiol. 14:1291119. doi: 10.3389/fphys.2023.1291119
Received: 20 September 2023; Accepted: 24 November 2023;
Published: 06 December 2023.
Edited by:
Marcello Chinali, Bambino Gesù Children’s Hospital (IRCCS), ItalyReviewed by:
Per Lav Madsen, University of Copenhagen, DenmarkRoberta Iacobelli, Bambino Gesù Children’s Hospital (IRCCS), Italy
Copyright © 2023 Sjöberg, Stephensen, Arheden, Heiberg and Carlsson. This is an open-access article distributed under the terms of the Creative Commons Attribution License (CC BY). The use, distribution or reproduction in other forums is permitted, provided the original author(s) and the copyright owner(s) are credited and that the original publication in this journal is cited, in accordance with accepted academic practice. No use, distribution or reproduction is permitted which does not comply with these terms.
*Correspondence: Pia Sjöberg, pia.sjoberg@med.lu.se