- 1Department of Physiology, Medical College of Wisconsin, Milwaukee, WI, United States
- 2Department of Physical Therapy, Marquette University, Milwaukee, WI, United States
- 3Departments of Pediatrics and Pharmacology, Case Western Reserve University, Cleveland, OH, United States
- 4Zablocki Veterans Affairs Medical Center, Milwaukee, WI, United States
- 5Neuroscience Research Center, Medical College of Wisconsin, Milwaukee, WI, United States
Synthetic opioids like fentanyl have improved the standard of care for many patients in the clinical setting, but their abuse leads to tens of thousands of overdose deaths annually. The current opioid epidemic underscores a critical need for insights into the physiological effects of fentanyl on vital functions. High doses of opioids in small mammals cause opioid-induced respiratory depression (OIRD) leading to hypoventilation, hypoxemia, and hypercapnia. In addition, opioids can also increase the alveolar to arterial oxygen (A-a) gradient and airway dysfunction. However, little is known about the physiologic effects of sub-lethal doses of opioids in large mammals. Here we report the effects of a sub-lethal dose range of fentanyl (25–125 μg/kg; IV) on vital physiologic functions over 90 min (min) and withdrawal-like behaviors over the subsequent 4 h (h) in adult female goats (n = 13). Fentanyl induced decreases in breathing frequency in the first few min post-injection, but then led to a sustained increase in tidal volume, total ventilation, and blood pressure with a reduced heart rate for ≥90 min. These ventilatory changes resulted in time-dependent arterial hypocapnia and hypoxemia and an increased alveolar to arterial oxygen gradient ∼30 min post-injection indicative of impaired gas exchange in the lung. The predominant effects of fentanyl on breathing were stimulatory, underscored by an increased rate of rise of the diaphragm muscle activity and increased activation of upper airway, intercostal and abdominal muscles. Beginning 90 min post-injection we also quantified withdrawal-like behaviors over 4 h, demonstrating dose- and time-dependent increases in locomotor, biting, itching, and pawing behaviors. We conclude that fentanyl at sublethal doses induces multiple physiologic and behavior changes that emerge along different time courses suggesting multiple independent mechanisms underlying effects of opioids.
Introduction
The development of synthetic opioids like fentanyl has improved the standard of care for patients in the clinical setting. However, the use and abuse of fentanyl has skyrocketed leading to tens of thousands of overdose deaths annually, which has stimulated studies to gain insight into the life-threating physiologic effects of opioids. Current data suggest fentanyl impairs ventilatory function by at least three mechanisms to elicit life-threatening hypoxemia. One major effect is opioid depression of CNS-driven ventilatory output termed Opioid-induced Respiratory Depression (OIRD). The neural circuitry that generates respiratory rhythm and motor patterns largely reside in the brainstem and includes regions like the pre-Bötzinger Complex (pre-BötC), the post-inspiratory complex (PiCO), and rostral pontine nuclei (Smith et al., 1991; St John, 1999; Anderson et al., 2016). Several studies indicate neurons in this key respiratory control circuit may be uniquely sensitive to opioid inhibition and underlie hypopnea/hypoventilation under physiologic conditions (Montandon and Horner, 2014; Anderson et al., 2016). A second ventilatory impairment elicited by opioids is skeletal muscle rigidity, including accessory respiratory muscles in the chest wall to create what is clinically known as “wooden chest syndrome” (WCS) (Marty and Desmonts, 1981; Drummond, 1983; Scamman, 1983; Weinger et al., 1988; Neidhart et al., 1989; Weinger and Bednarczyk, 1994; Lui et al., 1995; Bowdle, 1998). Synchronous activation of normally reciprocal respiratory muscles (diaphragm and abdominal muscles, internal and external intercostals) and upper airway muscles results from what appears to be an extreme simultaneous stimulation of respiratory pump and airway muscles (Scamman, 1983; Abrams et al., 1996; Bennett et al., 1997). A third respiratory impairment induced by opioids is pulmonary reductions in oxygen (O2) exchange leading to an increase in the alveolar to arterial (A-a) O2 gradient (Meyer et al., 2010; Getsy et al., 2022), which may have as great of an effect as apneas to reduce PaO2 after opioid administration. High dose opioids impair alveolar-capillary gas exchange presumably due to ventilation-perfusion mismatch within the lung.
Each of the effects of opioids alone or in combination could be life-threatening and may depend upon the timing of their occurrence. Decreases in ventilatory frequency presumably via inhibition of respiratory rhythm generation suggests that opioids can be life threatening because of a progressive CNS-mediated frequency decline leading to cessation of diaphragmatic activity and airflow (central apnea; CA). However, cessation of respiratory airflow also occurs even when respiratory rhythm generation is sustained but the airway is closed to induce an obstructive apnea (OA). The mechanism underlying opioid-induced OA is likely part of the WCS phenomenon via tonic activation of expiratory airway muscle and/or active vocal cord closure (Torralva and Janowsky, 2019). WCS and OA often emerge in less than 2 min after opioid intravenous (IV) injections, usually last up to 15 min, and presumably could cause death with a more accelerated time course than CA (Streisand et al., 1993). The mechanism(s) and timing of opioid-induced increases in the A-a O2 gradient are less clear but have been shown to develop with a slower time course than OIRD (Meyer et al., 2010; Getsy et al., 2022). Withdrawal symptoms manifest for hours after fentanyl use, and include symptoms such as hyperactivity, anxiety, and repetitive behaviors among others (Varshneya et al., 2019; Digranes et al., 2023). Accordingly, there is a need for a better understanding of the timing and magnitude of fentanyl on multiple vital physiological functions and potential withdrawal-like behaviors, particularly in a large animal model and across a dose range of fentanyl that does not cause sustained apnea or death.
Accordingly, herein we studied healthy, intact, freely-behaving adult goats to gain insight into time- and dose-dependent physiological effects and withdrawal-like behaviors following intravenous (IV) administration of fentanyl (2 min) over a range of doses that cause minimal or no OA. This design allowed for the characterization of the temporal pattern of each potential cause of fentanyl-induced hypoxemia. We tested the hypotheses that sublethal doses of opioids will induce both dose- and time-dependent dysfunction in multiple physiologic functions, including breathing frequency suppression (OIRD), increased tonic (or phasic) activation of chest wall and upper airway respiratory muscles (WCS) leading to a delayed or lack of inspiratory flow, arterial hypertension, reduced heart rate, and reductions of reflexes such as sighs and swallows. We further hypothesize that fentanyl will also increase acute withdrawal-like behaviors in adult goats like those seen in small mammals and humans (Varshneya et al., 2019; Digranes et al., 2023). Data from sublethal fentanyl doses will be important to clinicians in decisions regarding use of opioids as an analgesic after surgery and in chronic conditions of persistent pain.
Methods
All protocols were reviewed and approved by The Medical College of Wisconsin (MCW) Institutional Animal Care and Use Committee (IACUC). This institution has an Animal Welfare Assurance on file with the Office of Laboratory Animal Welfare. The Assurance Number is D16-00064 (A3102-01).
Adult female (>2y) goats (n = 13) of various breeds (i.e., Alpine, Toggenburg, Saanen) weighing 49.1 ± 2.9 kg were obtained from a local outbred herd. Goats were reared, transported, housed, and cared for according to USDA regulations. Upon arrival to the lab blood samples were drawn (jugular puncture) for complete blood count panels and pregnancy testing. Deworming conditioning (Ivermectin, 0.2 mg/kg, SQ); Fenbendazole, 5 mg/kg, PO) also occurred upon arrival and was repeated 2 weeks later.
Animal preparation (surgical instrumentation and recovery): As described previously (Feroah et al., 2002; Feroah et al., 2003), the goats underwent aseptic surgery for subcutaneous relocation of the carotid arteries bilaterally (for blood sampling and BP/HR monitoring) and implantation of EMG electrodes into airway (genioglossus and thyropharyngeus) and respiratory pump (diaphragm, intercostal, and transverse abdominus) muscles. Goats were anaesthetized (ketamine; 15 mg/kg, IV) for intubation and mechanically ventilated under 2%–3% isoflurane anesthesia (in 100% O2). Post-surgical health assessments (body temperature, food/water intake, surgical incision healing, etc.) were completed daily by trained staff, and goats received Banamine or Flunixin Meglumine (2 mg/kg, IM) and Naxcel (4 mg/kg, IM) for 3 and 7 days post-op, respectively. Unilateral carotid catheterization was performed ∼14 days post-op after which Naxcel (4 mg/kg, IM) was administered daily. Indwelling catheters were flushed daily with heparinized saline (0.1% heparin) followed by heparin lock solution.
Study preparation: Studies began ≥2 weeks post-surgery and following a week of training to familiarize the goats to the equipment. Goats were tethered with ropes and a harness in a stanchion and fitted with a custom ventilatory mask and one-way breathing valve adhered to the snout. The inspiratory and the expiratory ports of the valve were each connected to separate hoses and the inspired hose connected to a calibrated pneumotachometer whereas the expiratory hose was connected to a Tissot gasometer for collection of expired gases. An IV catheter was placed and secured unilaterally in the jugular vein daily prior to study (<30 min prior to injections). Muscle electromyographic (EMG) wires were clipped to cables and an arterial catheter line attached to a manifold and pressure transducer to allow for arterial blood sampling and measurement of blood pressure/heart rate. Rectal temperatures were recorded at regular intervals.
Study protocol: Physiologic measurements were recorded for 30 min before and for 90 min following IV injections of saline (0.9% NaCl) or fentanyl (25, 50, 75, 100, or 125 μg/kg; Hospira or West-Ward (50 μg/mL solution). Fentanyl dose order was randomized and distributed over approximately 4–5 weeks (≥48 h between studies). The dose range selected includes clinical intraoperative doses used in humans (20–50 μg/kg) to a level well below doses considered as “high” doses in rodents (<300 μg/kg) (Fujii et al., 2019). Arterial blood was sampled at regular intervals (every 15 min during pre-injection period; every 1 min for up to 5 min post-injection; every 15 min thereafter; Figure 1A). Study equipment (i.e., mask, valve, EMG cables, catheter lines) was removed after completion of the 120 min study and then the goats were fitted with a wearable Bioharness (Biopac Systems Inc, Goleta, CA, United States) and placed in a clear plexiglass pen (3.5′ x 4′ x 6’) equipped with an HD video camera (Logitech Webcam C930e) for recording withdrawal-like behaviors (Figure 1B) during which time the goat did not have access to food or water (4 h). Goats had access to food/water ad libitum other than pre-surgical fasting or during studies. After completion of all studies the goats were euthanized with IV ketamine/xylazine (2.5 mL; 24:1) and IV phenytoin/phenobarbital (B-euthanasia, 97.5 mg/kg) and the brains extracted (<10 min), rapidly frozen (2-methylbutane and dry ice) and stored at −80 °C for future studies.
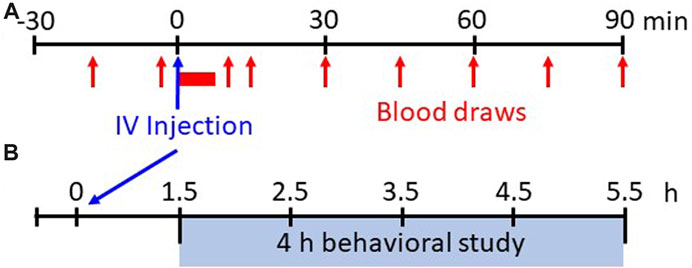
FIGURE 1. Experimental protocols. Shown in (A) is the experimental protocol for physiological measures for 30 min before and up to 90 min after IV injections of saline or various doses of fentanyl. Breathing, blood pressure/heart rate, and EMG activity are measured continuously whereas arterial blood samples are drawn at 15 min intervals during the pre-injection period and beginning 15 min post-injection (serial blood samples taken from 1–5 min post-injection initiation). Shown in (B) is the experimental protocol for withdrawal-like behavior monitoring. Following 90 min post-injection the animals are placed in a custom pen wearing a Bluetooth bio-harness and video recordings (HD webcam) are captured for offline analyses of locomotor behaviors beginning 1.5 h–5.5 h (4 h total) post-injection.
Data Acquisition and Analysis: Powerlab and LabChart software (ADInstruments, Colorado Springs, CO, United States) was used for the continuous recording of inspiratory flow, blood pressure/heart rate, and respiratory muscle (EMG) activity (Figure 2A). A gas analyzer (OxiGraf, Sunnyvale, CA, United States) was utilized for measurement of mixed expired gas composition, i.e., expired O2 (FEO2) and CO2 (FECO2) collected in a Tissot gasometer (5 min collections) from which oxygen consumption (
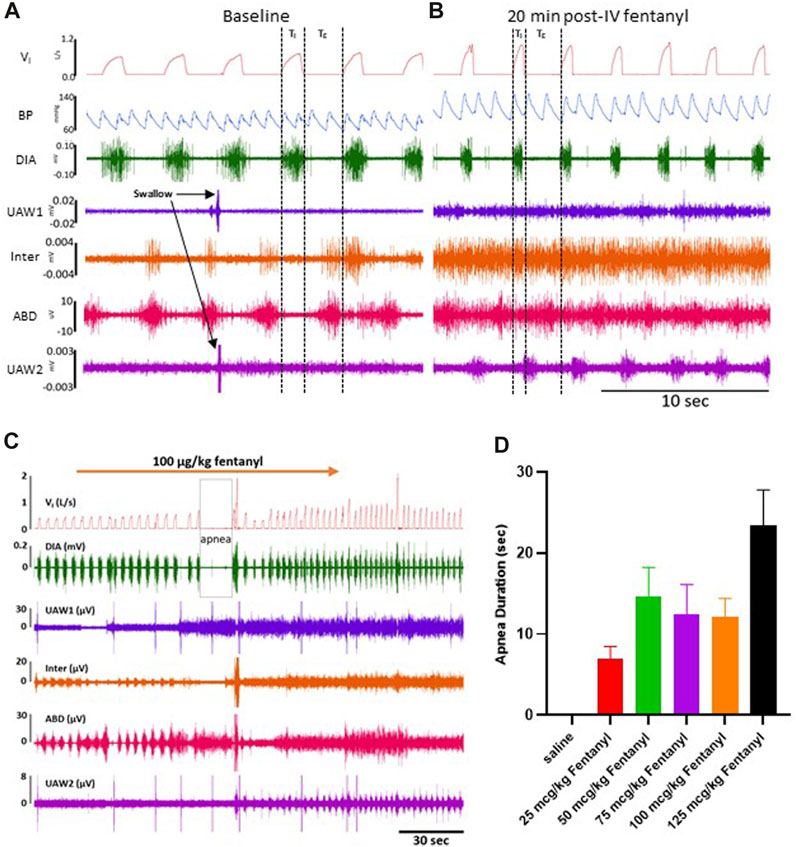
FIGURE 2. Representative tracing during baseline and 50 μg/kg fentanyl. Inspiratory flow, arterial pulse pressure, and muscle activity is measured during a control period (A) followed by an intravenous (IV) injection (2 min) of 50 μg/kg fentanyl.
Statistical analyses were completed using One- or Two-way RM ANOVA or Mixed-effects Analysis (if there were missing data) with Dunnett’s or another appropriate post hoc test (Time and Dose factors; GraphPad Prism 8 Software), and p < 0.05 except wherever noted. All statistical tests (and confidence intervals) used are reported in the figure legends or Results section.
Results
All fentanyl injections (2 min; IV) caused all goats to lay supine from a standing posture during or shortly after completing the injection of fentanyl while animals given IV saline injections remained standing (n = 13). The time from initiation of the injection to laying with 25 μg/kg fentanyl was 3.04 ± 0.39 min which decreased to 1.85 ± 0.22 min (50 μg/kg), 1.59 ± 0.19 min (75 μg/kg), 1.43 ± 0.11 min (100 μg/kg) and 1.58 ± 0.16 min (125 μg/kg) with increasing doses (p ≤ 0.014; Supplementary Figure S2A). Once supine, the animals appeared sedated and largely immobilized with the exceptions of voluntary repositioning side to side, grinding their teeth and/or occasional bouts of coughing. Periods of these events were deleted from the recordings before quantitation of physiologic responses. The time animals remained in the laying position was dose-dependent, where they voluntarily stood after 55.2 ± 7.7 min, 70.4 ± 6.3 min, 81.8 ± 4.0 min, 82.7 ± 3.7 min, or 89.3 ± 0.3 min after 25, 50, 75, 100 or 125 μg/kg fentanyl, respectively, where the time to stand was longer for all doses >75 μg/kg compared to 25 μg/kg (p ≤ 0.005; Supplementary Figure S2B). It is noteworthy that the time to stand for the higher doses was affected by the removal of instrumentation from the animal at the end of the 90 min experiment, and at times the goats would remain immobilized requiring assistance to be transferred to the behavioral chamber.
Due to their immobilization with fentanyl, we assessed potential changes in protective behaviors (sighs and swallows) along with obstructive apneas (OAs) during baseline conditions and up to 90 min post-injection (Supplementary Figure S2C-E). Swallows occurred frequently during baseline conditions (∼2/min) and continued without change after saline injection but decreased for 90 min post-injection of all doses of fentanyl (p < 0.05; Supplementary Figure S2C). Sighs, which occur regularly to reinflate alveoli and prevent atelectasis, did not differ during baseline conditions but generally increased at various timepoints for all doses of fentanyl (p < 0.05; Supplementary Figure S2D). OAs (defined as TE> 2x baseline) were very infrequent across all conditions and timepoints and were only increased initially after 100 μg/kg fentanyl in the first 30 min after injection (p = 0.0096; Supplementary Figure S2E).
The majority of goats responded to fentanyl with transient decreases in FB (via increased TE) and sustained increases in VT and VI (representative data shown in Figure 2). In addition, we also typically observed increases in tonic activation of the upper airway (UAW), intercostal and abdominal muscles after fentanyl injections (Figure 2). Furthermore, heart rate (HR) decreased and arterial blood pressures were increased with IV fentanyl (Figure 2). However, the effects of fentanyl on breathing frequency (FB), tidal volume (VT) and minute ventilation (
Due to the transient nature of the effects, breathing metrics were calculated in 1 s intervals, binned (30 s intervals) and normalized to each animals’ respective baseline (control; averaged across 5 min) value (Figures 3A–F). Across all animals, IV saline had no significant effect on
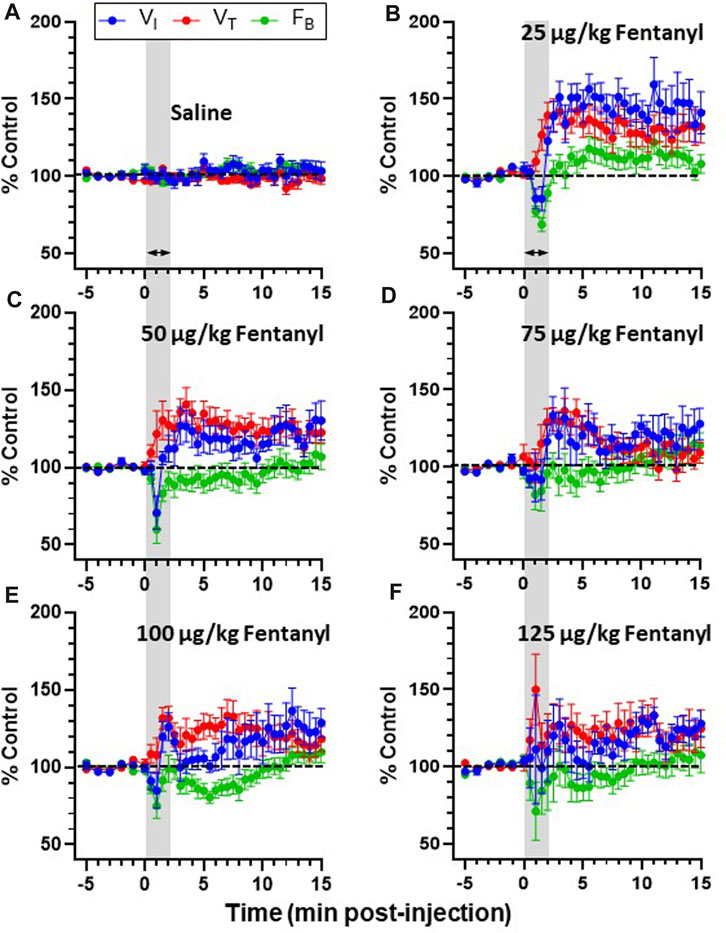
FIGURE 3. Effects of fentanyl on breathing measures in adult goats. Minute ventilation (
Inspiratory and expiratory time (TI and TE, respectively) and the ratio of VT/TI (an index of respiratory drive) was calculated during baseline conditions and for up to 90 min post-injection (Figures 4A–C). TI was ∼1.1 s during baseline conditions for all goats and remained >1.0 s for up to 90 min following IV saline (Figure 4A) but decreased in a dose-independent manner after IV fentanyl such that by 30–90 min post-injection TI was reduced at all doses tested (p ≤ 0.046). Consistent with the transient decrease in FB, TE increased slightly within the first 5 min post-fentanyl injection with higher doses but then returned to baseline levels thereafter (Figure 4B) similar to the effects on ventilatory drive (Figure 4C). The decreased TI and increased
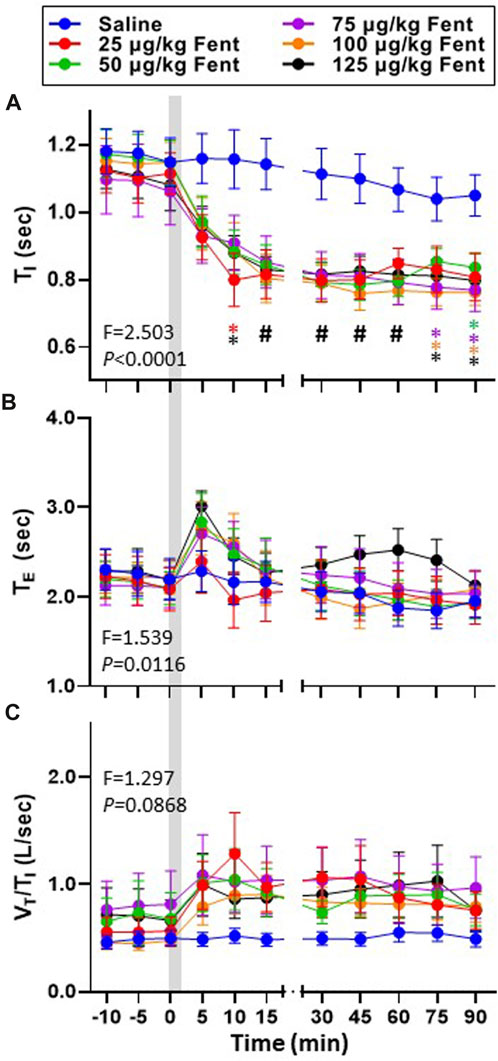
FIGURE 4. Effects of fentanyl on breath cycle timing and ventilatory drive. Inspiratory time (TI (sec); (A), expiratory time (TE (sec); (B) and an index of ventilatory drive (VT/TI (L/breath/sec)); (C) before and up to 90 min post-injection of IV saline (blue) or 25 (red), 50 (green), 75 (purple), 100 (orange) or 125 (black) µg/kg fentanyl. Two-way RM ANOVA (Time and Dose factors; interaction terms in figure) #p < 0.05 all doses vs. saline; *p < 0.05 individual dose vs. saline (n = 10–13). Grey bar indicated injection (2 min). The figure shows that fentanyl decreased inspiratory time and increased expiratory time and at most doses and increased ventilatory drive.
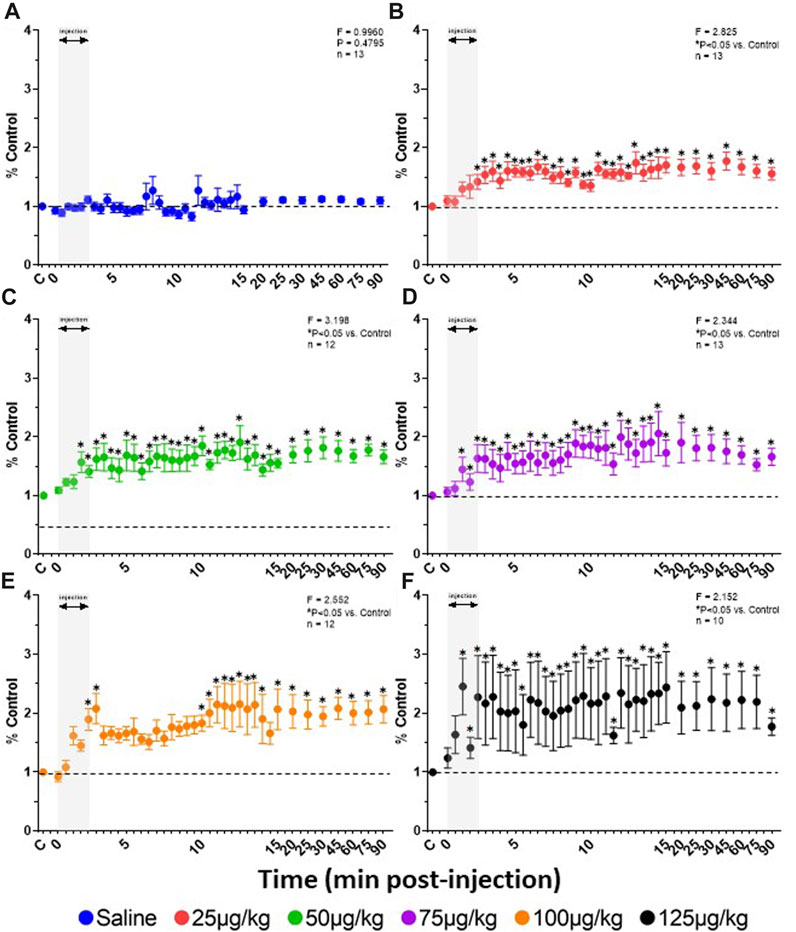
FIGURE 5. Effect of fentanyl on diaphragmatic activation. Rate of rise (mV/sec) calculations from the integrated DIA EMG (expressed as a percent of control (baseline)) during and up to 90 min after IV injections of saline (blue; (A) or 25 (red; (B), 50 (green; (C), 75 (purple; (D), 100 (orange; (E) or 125 (black; (F) µg/kg fentanyl. Grey indicates injection period and dashed line control (baseline) values. Two-way RM ANOVA (Time and Dose factors; interaction terms included in figure) and Dunnett’s post hoc. * indicates p < 0.05 vs. control. This figure shows that all doses of fentanyl increased rate of rise of the diaphragm EMG indicating an excitatory effect during 90 min after the injection.
Arterial blood gases measured during baseline conditions were consistent across animals and did not vary between studies over the ∼3 weeks required to complete the sum of all experiments (Supplementary Table S1) indicating no lasting effects of the injections. Assessments of arterial pH, PCO2 and PO2 were made during the injection period and immediately following (1–4.5 min) and then at 10, 15, 30, 45, 60, 75 and 90 min post-injection. IV injections of saline had no major effects on arterial blood gases throughout the post-injection period, expressed either in absolute values (Supplementary Table S1) or as a change from baseline (Figures 6A–F). Arterial pH was unchanged with all doses of fentanyl during and immediately after injections but tended to increase with the highest dose (Figure 6A, B) but was consistently increased thereafter likely due to corresponding decreases in PaCO2 at the same timepoints (Figure 6C, D). Despite the observed hyperventilation, PaO2 levels were lower immediately after fentanyl injections and persisted for up to 45 min post-injection (Figure 6E, F). The slight hypoxemia shown in Figure 6 may contribute to the post fentanyl hyperpnea shown in Figure 3. However, any contribution would be minimal due to the small or lack of increase in carotid chemoreceptor activity and ventilatory stimulation with the minor changes from normal in oxygenation (Nielsen et al., 1985). We also noted minor fluctuations in blood electrolytes, with the most significant changes observed in blood glucose levels after low dose fentanyl persisting up to 90 min post-injection (Supplementary Table S1). Differences between alveolar (A) and arterial (a) PO2 increased following fentanyl injections at multiple doses, either expressed in absolute terms (Figure 7A) or as the change from baseline conditions (Figure 7B). Together these data suggest that at sub-lethal doses fentanyl led to sustained hyperventilation, and are highly suggestive of increases in pulmonary ventilation to perfusion (
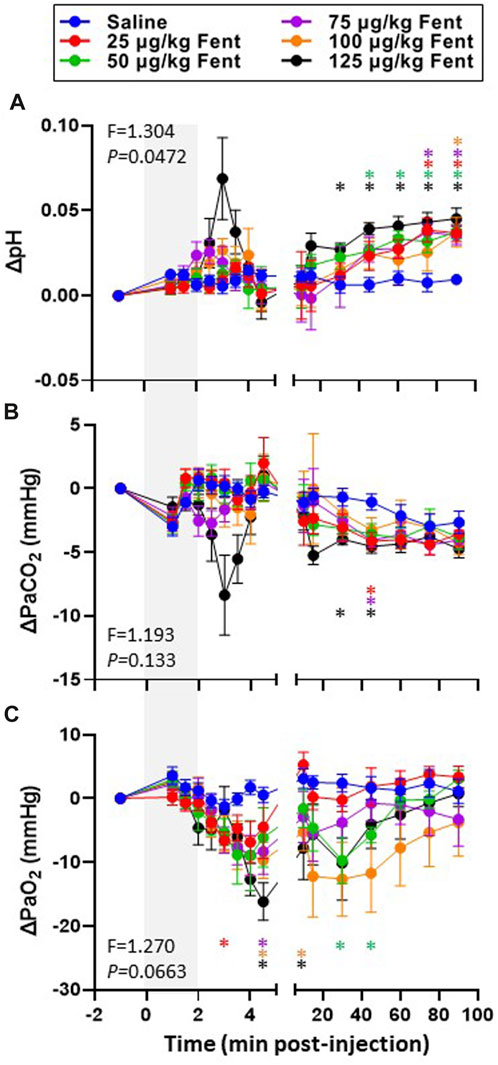
FIGURE 6. Changes in arterial blood gases with IV fentanyl injections. Shown are the changes from baseline in arterial pH (A; pH units), PaCO2 (mmHg; (B) and PaO2 (C; mmHg) before, during and after IV injections of saline (blue) or 25 (red), 50 (green), 75 (purple), 100 (orange) or 125 (black) µg/kg fentanyl. Mixed-effects Model (REML) with Dunnett’s Multiple Comparisons (Time and Dose as factors; Interaction terms shown in figure). * indicates p < 0.05 vs. saline. Note that injection of saline did not alter blood gases and pH whereas fentanyl caused transient hypercapnia and acidosis initially but alkalosis and hypocapnia over most of the post injection period. In addition, fentanyl decreased PaO2 for most of the post injection period.
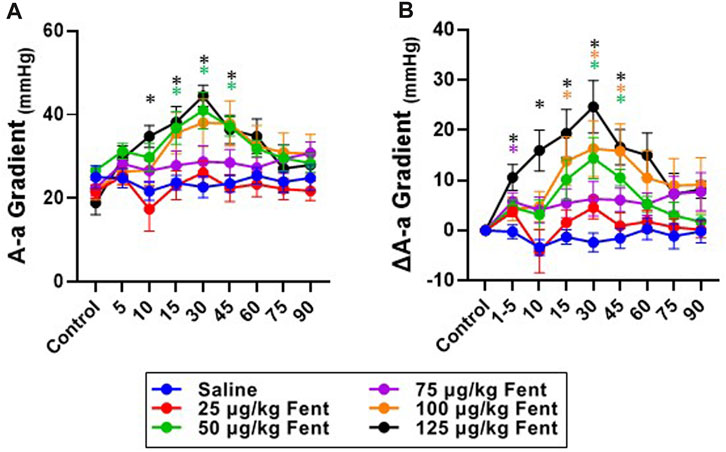
FIGURE 7. Absolute and changes in the alveolar to arterial (A–A) O2 gradient with fentanyl. Absolute values (A) or the change from baseline values (delta; (B) of A-a O2 gradients during control (baseline) conditions for up to 90 min post IV injection of saline (blue; n = 9) or 25 (red; n = 9), 50 (green; n = 11), 75 (purple; n = 13), 100 (orange; n = 12) or 125 (black; n = 8) µg/kg fentanyl. Mixed-effects Model (REML) with Dunnett’s Multiple Comparisons (Time and Dose as factors). *indicates p < 0.05 vs. saline for individual dose. Note that there was a dose- and time-dependent fentanyl-induced increase in the A-a O2 difference.
Heart rate, mean arterial blood pressure, and systolic and diastolic blood pressures were generally unchanged after IV saline injections (Figures 8A–D). However, IV fentanyl transiently decreased heart rate at lower doses but had a sustained depressant effect with 125 μg/kg fentanyl (Figure 8A). In contrast, IV fentanyl increased mean (Figure 8B), systolic (Figure 8C) and diastolic (Figure 8D) blood pressures within the first 10 min and for up to 90 min post-injection, although it appeared that these values were near baseline at the end of the study period with the 25 μg/kg dose. These effects were independent of changes in O2 consumption (
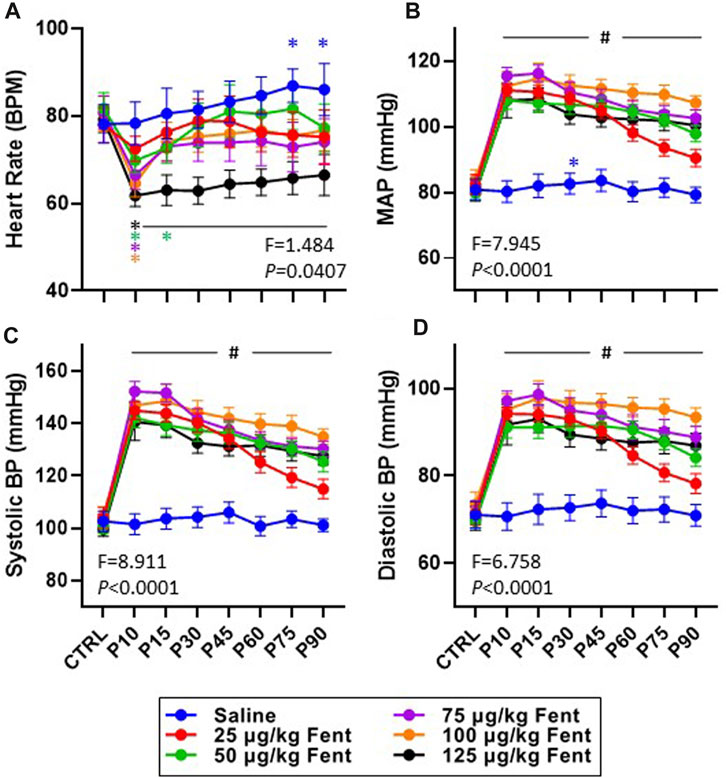
FIGURE 8. Effects of fentanyl on heart rate and blood pressures. Heart rate (beats/min; (A) and mean arterial (mmHg; (B), systolic (mmHg; (C) and diastolic (mmHg; (D) blood pressures during control (baseline) conditions and up to 90 min post-injection (P) of saline (blue; n = 10) or 25 (red; n = 10), 50 (green; n = 13), 75 (purple; n = 13), 100 (orange; n = 12) or 125 (black; n = 10) µg/kg fentanyl. Mixed-effects Model (REML) with Dunnett’s Multiple Comparisons (Time and Dose as factors; interaction terms shown in figure). #indicates p < 0.05 at all doses vs. baseline; * indicates p < 0.05 for individual dose vs. baseline. Note also that fentanyl increased arterial blood pressure with very little time dependent recovery.
The effects of fentanyl on cardiovascular, metabolic rate, and body temperature responses within 90 min after injections were complicated by shifts in posture while laying and occasional shivering which varied in intensity and duration. Due to potential of affecting ventilatory responses. we did not implement methods to adjust for periods of shivering or changes in posture.
In addition to the physiological effects of sub-lethal doses of fentanyl described above, we also quantified withdrawal-like behaviors over a 4 h period beginning 90 min post-injection. The withdrawal-like behaviors quantified (in 30 min intervals) included general increases in movement in the behavioral chamber measured with a Bioharness tracking device (hyperactivity; vector magnitude; Figure 9A), and videographic observations of numbers of biting, pawing and itching (Figures 9B–D, respectively). All animals had some level of activity in the behavioral chamber following saline injections, but activity was far greater following fentanyl injections (Figure 9A). The increased activity was dose-dependent as by 3 h their overall activity was not different from control after 25 or 50 μg/kg fentanyl but remained increased up to 4 h (5.5 h post-injection) after the 125 μg/kg dose. Similarly, the occurrences of biting (Figure 9B), pawing (Figure 9C) and itching (Figure 9D) were generally increased at various timepoints across the 4 h monitoring period after fentanyl injections compared to saline. Collectively, there was a measurable increase in withdrawal-like behaviors across a 4 h monitoring period beginning 90 min post-injection.
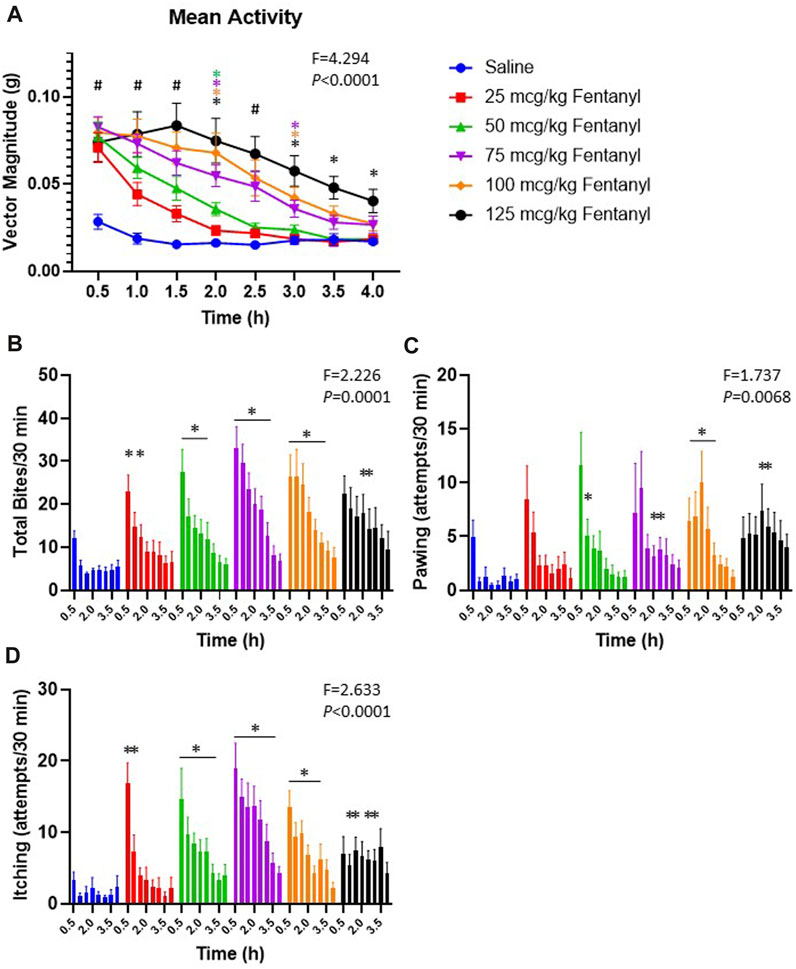
FIGURE 9. Withdrawal-like behaviors increase after fentanyl injections. Shown in (A) is the average bodily movement measured as vector magnitude (G) in 30 min intervals over 4 h beginning 1.5 h post-injection (time 0.5 is from 90–120 min post-injection). Mixed Effects analysis with multiple comparisons (Factors Time and Dose; F and p values shown are Interaction term). # indicates differences for all doses vs. saline; * indicates difference vs. saline with a specific dose (color-coded). Shown in (B-D) is the occurrences of biting, pawing and itching in each 30-min interval of the 4 h observation period. * indicates difference from saline at that timepoint (*p < 0.10). Mixed Effects analysis with multiple comparisons (Factors Time and Dose; F and p values shown are Interaction term).
Discussion
With the rise in opioid-related overdose fatalities there is a growing need to better understand the integrative physiological effects of synthetic opioids like fentanyl. Here we tested the hypothesis that sublethal doses of IV fentanyl in intact, awake, adult goats would lead to significant dysfunction in ventilation, pump and upper airway muscles, and the cardiovascular system in a time- and dose-dependent manner. We further tested the hypothesis that adult goats may also experience withdrawal-like behaviors in the hours after fentanyl administration, and thus be a good model for studying acute withdrawal. The major findings of the present study were that sub-lethal doses of fentanyl had a time- and dose-dependent stimulatory effect on many physiologic functions including ventilation, pump and airway muscle activity, and arterial blood pressure in addition to increases in the alveolar-arterial O2 gradient. Moreover, the fentanyl injections induced behavioral excitation which continued after physiologic effects had subsided. Collectively, these data suggest that fentanyl can be stimulatory to multiple vital functions in large animals across the dose range tested.
Opioids have long been known to affect breathing in a dose-dependent manner, where the response to fentanyl in humans is typically described as “deep and slow” breathing across a clinically-relevant dose range (Bouillon et al., 2003). Whether or not patients or animals develop a hypopnea (reduced minute ventilation) or hypoventilation (increased PaCO2) largely depends upon how much fentanyl they receive or their “sensitivity” to the drug. As the dose of fentanyl increases, ventilatory suppression becomes more pronounced leading to the life-threatening opioid-induced respiratory depression (OIRD). However, fentanyl has also been shown to elicit additional respiratory-related dysfunction through increased upper airway (UAW) resistance, chest wall rigidity (“Wood Chest Syndrome”; WCS), and by worsening ventilation/perfusion ratios to increase the alveolar to arterial oxygen gradient (A-a O2 gradient). Here we tested a dose range of IV fentanyl in a large animal species sufficient to induce sedation (immobilization) without sustained obstructive or central apneas and determine if OIRD, increased airway and chest wall muscle activity, and/or an increased A-a O2 gradient are present.
The overall effects of fentanyl (25–125 μg/kg) on ventilation in goats was largely stimulatory. Within seconds to minutes after fentanyl injections we noted transient periods with extremely long TE and suppression of FB. However, VT immediately increased and remained higher for up to 90 min post-injection. The relative increase in VT was greater than the decrease in FB such that
Perhaps most important among the many effects of fentanyl documented herein are the effects on airway and pump muscle activity. General muscle rigidity following opioid administration has been well-documented in animals (Hamilton and Cullen, 1953; Freye and Kuschinsky, 1976; Wells et al., 1994; Elakkumanan et al., 2008; Coruh et al., 2013; Phua et al., 2017) but reports of this phenomenon in humans are less common. WCS likely is caused by increased simultaneous activation of inspiratory and expiratory chest wall muscles to reduce compliance which could impair ventilation. However, studies on patients in which the airway was bypassed (intubation or tracheostomy) suggested mechanical ventilation was effective and thus that airway compromise may contribute more to ventilatory dysfunction with opioids than previously recognized (Scamman, 1983; Abrams et al., 1996; Bennett et al., 1997). Here we showed that tonic muscle activation in the genioglossus and thyropharyngeus was increased 2 to 3-fold for up to 30 min post-injection. In addition, fentanyl-induced increases in tonic activities of the intercostal and abdominal muscles would also support the concept that chest wall compliance and/or the mechanics of pulmonary ventilation are dysfunctional and limited. Changes in body position, from standing to supine, could affect activity of pump and airway muscles and thereby have a wooden chest like effect on breathing. We also found that the frequency of swallows was reduced by ∼50%, which indicates a decrease in airway protective reflexes. Together, these data strongly suggest fentanyl-induced increases in inspiratory airway resistance, a suppression of protective airway reflexes (swallowing), and potentially decreased chest wall compliance.
Fentanyl can also decrease arterial PO2 through impairment of O2 exchange in the lung, presumably due to increase
Discontinuation of opioid use leads to acute withdrawal symptoms in humans and pre-clinical rodent models, which include hyperalgesia, anxiety, hyperactivity, rearing vocalization, piloerection and itching (Kosten and Baxter, 2019; Pergolizzi et al., 2020; Ozdemir et al., 2023). Here we extended our studies to include observations of some withdrawal-like behaviors in a large animal model. During a 4 h observations period (beginning 90 min post-injection) we noted significant increases in locomotor behavior in addition to biting, itching and pawing. Each of these behaviors were greatest at the beginning of the observation period and waned by 3–4h, where the duration of the increases in each behavior was dose-dependent. These behaviors are similar to that observed in rodents and pigs following abstinence (Varshneya et al., 2019; Digranes et al., 2023) or naloxone-precipitated withdrawal from fentanyl (Uddin et al., 2021), suggesting that the adult goat may also be a useful model to study mechanisms or other unique aspects of withdrawal from fentanyl and its relevance to human withdrawal symptomatology.
Most clinical data on the use of opioids in patients report fairly uniform ventilatory responses of “slow and deep” breathing patterns. Less well recognized are reports that ventilatory and behavioral responses to fentanyl can vary appreciably among patients (Bouillon et al., 2003; Ikeda et al., 2005; Nishizawa et al., 2023). Moreover, most humans receiving opioids experience a euphoria (positive feeling), but ∼25% of patients instead experience dysphoria (strong negative feeling) accompanied by agitation (hoehe, 2007). The variation in human responses to opioids was the basis for a systematic analysis of variance in the human µ opioid receptor gene which revealed “abundant DNA sequence diversity suggesting numerous individual forms of the gene” (hoehe, 2007; Hoehe, 1988; Hoehe et al., 1988). Another feature of our data in goats was the unexpected, individual-dependent ventilatory responses to systemic fentanyl (Supplementary Figure S3). Most adult female goats respond over the first few minutes after fentanyl injection by decreasing breathing FB and increasing VT (Figure 2) which is representative of the average effect. However, two of the thirteen goats studied (∼15%) had highly stimulatory responses to fentanyl at multiple doses which lead to us prematurely stopping the experiment and removing the breathing valve and mask even with these relatively low doses of fentanyl. Only one of 13 goats required “rescue” with IV naloxone due to extremely low PaO2 (and high PaCO2) levels 30–40 min post-injection of 125 μg/kg fentanyl, and all other goats at all doses (64 of 65 experiments) did not require naloxone reversal. The hypoxemia observed in this goat was due to an above normal A-a O2 gradient without any measurable OIRD. Despite individual variation in physiological responses to fentanyl at a given dose, repeat experiments with 50 μg/kg fentanyl within each goat were highly reproducible in acute physiologic effects and 4 h behavioral studies (Supplementary Figure S4A-E) suggesting that randomization of dose order and a 48 h minimum between experiments had no measurable “desensitizing” effect. Irrespective of the mechanism leading to inter-animal variation in response to opioids, our data indicate that adult female goats have a human-like ventilatory response to systemic opioids suggesting goats are an appropriate model for mechanistic studies. This response differs in some respects to the response to opioids of other species. Comparisons between species are of value to gain insight into multiple factors such as drug distribution, metabolism, and drug interactions with other substances that affect the physiologic and behavioral responses to opioids. Accordingly, one goal of our future studies is to compare the effects of other opioid reversal substances shown to be effective or ineffective in other species.
In summary, herein we present findings at sublethal doses of fentanyl indicating there are time dependent changes in respiratory rhythm generation, wooden chest syndrome, alveolar-capillary gas exchange and withdrawal like symptoms that compromise normal physiologic functions and behavior. One fentanyl effect is transient depression of the respiratory rhythm generating mechanisms in the brain which was evident herein by the reduction in breathing frequency and increased duration of expiration only over the first few minutes after fentanyl injection. The dominate effect observed herein was increased breathing frequency and excitation of respiratory muscles for 30–90 min post-injection. A second fentanyl effect known as the “wooden chest syndrome” is simultaneous contraction of inspiratory and expiratory pump and airway muscles which begins a few minutes after fentanyl injection and is intermittent for at least 90 min after fentanyl injection. This effect was manifested herein by a delay in inspiratory airflow after diaphragm contraction indicative of airway constriction. A third fentanyl effect is reduced exchange of gas between alveoli and pulmonary capillaries. This effect is caused by a mismatch in delivery of air and blood to individual exchange units. The time course of each of these three effects differ, strongly suggesting that there are independent mechanisms for each fentanyl effect (neuro-respiratory output, tonic muscle activation and alveolar-capillary gas exchange). Fourth, impediment to normal physiologic function were withdrawal like symptoms characterized by random postural movements indicative of a prolonged excitatory state that persist for up to 3 hours after fentanyl injection. The present study is important in utilizing a design to avoid or minimize lethal fentanyl doses and thereby elucidate the distinct timing of emergence of fentanyl-induced physiologic and behavioral dysfunction. We conclude that sub-lethal doses of fentanyl elicit multiple compromised physiological functions independent of OIRD which may independently or in combination lead to severe outcomes.
Data availability statement
The original contributions presented in the study are included in the article/Supplementary Material, further inquiries can be directed to the corresponding author.
Ethics statement
The animal study was approved by the All protocols were reviewed and approved by The Medical College of Wisconsin (MCW) Institutional Animal Care and Use Committee (IACUC). This institution has an Animal Welfare Assurance on file with the Office of Laboratory Animal Welfare. The study was conducted in accordance with the local legislation and institutional requirements.
Author contributions
SN: Conceptualization, Data curation, Formal Analysis, Investigation, Methodology, Supervision, Writing–original draft, Writing–review and editing, Validation. NB: Data curation, Formal Analysis, Investigation, Methodology, Validation, Writing–review and editing. GH: Data curation, Formal Analysis, Investigation, Methodology, Validation, Writing–review and editing. KG: Conceptualization, Data curation, Methodology, Writing–review and editing, Formal Analysis, Investigation, Supervision, Validation, Writing–original draft. RT: Writing–review and editing, Data curation, Methodology, Formal Analysis, Validation. JD: Data curation, Formal Analysis, Methodology, Validation, Writing–review and editing. GH: Formal Analysis, Validation, Writing–review and editing. MMH: Formal Analysis, Validation, Writing–review and editing. LP: Conceptualization, Data curation, Investigation, Methodology, Supervision, Writing–review and editing. SL: Conceptualization, Investigation, Methodology, Validation, Writing–review and editing. HF: Conceptualization, Data curation, Formal Analysis, Funding acquisition, Investigation, Methodology, Project administration, Resources, Software, Supervision, Validation, Visualization, Writing–original draft, Writing–review and editing. MRH: Conceptualization, Data curation, Formal Analysis, Funding acquisition, Investigation, Methodology, Project administration, Resources, Software, Supervision, Validation, Visualization, Writing–original draft, Writing–review and editing.
Funding
The author(s) declare financial support was received for the research, authorship, and/or publication of this article. This work was funded by NIH DA050571 (MH and HF).
Conflict of interest
The authors declare that the research was conducted in the absence of any commercial or financial relationships that could be construed as a potential conflict of interest.
The author(s) declared that they were an editorial board member of Frontiers, at the time of submission. This had no impact on the peer review process and the final decision.
Publisher’s note
All claims expressed in this article are solely those of the authors and do not necessarily represent those of their affiliated organizations, or those of the publisher, the editors and the reviewers. Any product that may be evaluated in this article, or claim that may be made by its manufacturer, is not guaranteed or endorsed by the publisher.
Supplementary material
The Supplementary Material for this article can be found online at: https://www.frontiersin.org/articles/10.3389/fphys.2023.1277601/full#supplementary-material
References
Abrams J. T., Horrow J. C., Bennett J. A., Van Riper D. F., Storella R. J. (1996). Upper airway closure: A primary source of difficult ventilation with sufentanil induction of anesthesia. Anesth. Analg. 83 (3), 629–632. doi:10.1097/00000539-199609000-00034
Anderson T. M., Garcia A. J., Baertsch N. A., Pollak J., Bloom J. C., Wei A. D., et al. (2016). A novel excitatory network for the control of breathing. Nature 536 (7614), 76–80. doi:10.1038/nature18944
Bennett J. A., Abrams J. T., Van Riper D. F., Horrow J. C. (1997). Difficult or impossible ventilation after sufentanil-induced anesthesia is caused primarily by vocal cord closure. Anesthesiology 87 (5), 1070–1074. doi:10.1097/00000542-199711000-00010
Bouillon T., Bruhn J., Roepcke H., Hoeft A. (2003). Opioid-induced respiratory depression is associated with increased tidal volume variability. Eur. J. Anaesthesiol. 20 (2), 127–133. doi:10.1017/s0265021503000243
Bowdle T. A. (1998). Adverse effects of opioid agonists and agonist-antagonists in anaesthesia. Drug Saf. 19 (3), 173–189. doi:10.2165/00002018-199819030-00002
Burgraff N. J., Neumueller S. E., Buchholz K., Langer T. M., Hodges M. R., Pan L., et al. (2018). Ventilatory and integrated physiological responses to chronic hypercapnia in goats. J. Physiol. 596 (22), 5343–5363. doi:10.1113/JP276666
Coruh B., Tonelli M. R., Park D. R. (2013). Fentanyl-induced chest wall rigidity. Chest 143 (4), 1145–1146. doi:10.1378/chest.12-2131
Digranes N., Haga H. A., Nordgreen J. (2023). High and hyper: fentanyl induces psychomotor side-effects in healthy pigs. Anim. (Basel) 13 (10), 1671. doi:10.3390/ani13101671
Drummond G. B. (1983). Comparison of decreases in ventilation caused by enflurane and fentanyl during anaesthesia. Br. J. Anaesth. 55 (9), 825–835. doi:10.1093/bja/55.9.825
Elakkumanan L. B., Punj J., Talwar P., Rajaraman P., Pandey R., Darlong V. (2008). An atypical presentation of fentanyl rigidity following administration of low dose fentanyl in a child during intraoperative period. Paediatr. Anaesth. 18 (11), 1115–1117. doi:10.1111/j.1460-9592.2008.02602.x
Feroah T. R., Forster H. V., Fuentes C. G., Lang I. M., Beste D., Martino P., et al. (2002). Effects of spontaneous swallows on breathing in awake goats. J. Appl. Physiol. 92 (5), 1923–1935. doi:10.1152/japplphysiol.01079.2000
Feroah T. R., Forster H. V., Fuentes C. G., Martino P., Hodges M., Wenninger J., et al. (2003). Perturbations in three medullary nuclei enhance fractionated breathing in awake goats. J. Appl. Physiol. 94 (4), 1508–1518. doi:10.1152/japplphysiol.00837.2002
Freye E., Kuschinsky K. (1976). Effects of fentanyl and droperidol on the dopamine metabolism of the rat striatum. Pharmacology 14 (1), 1–7. doi:10.1159/000136573
Fujii K., Koshidaka Y., Adachi M., Takao K. (2019). Effects of chronic fentanyl administration on behavioral characteristics of mice. Neuropsychopharmacol. Rep. 39 (1), 17–35. doi:10.1002/npr2.12040
Getsy P. M., Baby S. M., May W. J., Young A. P., Gaston B., Hodges M. R., et al. (2022). D-cysteine ethyl ester reverses the deleterious effects of morphine on breathing and arterial blood-gas chemistry in freely-moving rats. Front. Pharmacol. 13, 883329. doi:10.3389/fphar.2022.883329
Hamilton W. K., Cullen S. C. (1953). Effect of levallorphan tartrate upon opiate induced respiratory depression. Anesthesiology 14 (6), 550–554. doi:10.1097/00000542-195311000-00002
Hoehe M., Duka T., Doenicke A. (1988). Human studies on the mu opiate receptor agonist fentanyl: neuroendocrine and behavioral responses. Psychoneuroendocrinology 13 (5), 397–408. doi:10.1016/0306-4530(88)90046-7
Hoehe M. (1988). Influence of the menstrual cycle on neuroendocrine and behavioral responses to an opiate agonist in humans: preliminary results. Psychoneuroendocrinology 13 (4), 339–344. doi:10.1016/0306-4530(88)90059-5
hoehe M. R. (2007). Individual variation in response to u opiate receptor challenge - past, present and future: A "personal" history of investigation. dialogues Clin. Neurosci. 9, 471–475.
Ikeda K., Ide S., Han W., Hayashida M., Uhl G. R., Sora I. (2005). How individual sensitivity to opiates can be predicted by gene analyses. Trends Pharmacol. Sci. 26 (6), 311–317. doi:10.1016/j.tips.2005.04.001
Kosten T. R., Baxter L. E. (2019). Review article: effective management of opioid withdrawal symptoms: a gateway to opioid dependence treatment. Am. J. Addict. 28 (2), 55–62. doi:10.1111/ajad.12862
Langer T. M., Neumueller S. E., Crumley E., Burgraff N. J., Talwar S., Hodges M. R., et al. (2017b). Effects on breathing of agonists to mu-opioid or GABAA receptors dialyzed into the ventral respiratory column of awake and sleeping goats. Respir. Physiol. Neurobiol. 239, 10–25. doi:10.1016/j.resp.2017.01.007
Langer T. M., Neumueller S. E., Crumley E., Burgraff N. J., Talwar S., Hodges M. R., et al. (2017a). Ventilation and neurochemical changes during µ-opioid receptor activation or blockade of excitatory receptors in the hypoglossal motor nucleus of goats. J. Appl. Physiol. 123 (6), 1532–1544. doi:10.1152/japplphysiol.00592.2017
Lui P. W., Tsen L. Y., Fu M. J., Yeh C. P., Lee T. Y., Chan S. H. (1995). Inhibition by intrathecal prazosin but not yohimbine of fentanyl-induced muscular rigidity in the rat. Neurosci. Lett. 201 (2), 167–170. doi:10.1016/0304-3940(95)12162-5
Marty J., Desmonts J. M. (1981). Effects of fentanyl on respiratory pressure-volume relationship in supine anesthetized children. Acta Anaesthesiol. Scand. 25 (3), 293–296. doi:10.1111/j.1399-6576.1981.tb01654.x
Meyer L. C., Hetem R. S., Fick L. G., Mitchell D., Fuller A. (2010). Effects of serotonin agonists and doxapram on respiratory depression and hypoxemia in etorphine-immobilized impala (Aepyceros melampus). J. Wildl. Dis. 46 (2), 514–524. doi:10.7589/0090-3558-46.2.514
Meyer L. C., Hetem R. S., Mitchell D., Fuller A. (2015). Hypoxia following etorphine administration in goats (Capra hircus) results more from pulmonary hypertension than from hypoventilation. BMC Vet. Res. 11, 18. doi:10.1186/s12917-015-0337-5
Montandon G., Horner R. (2014). CrossTalk proposal: the preBotzinger complex is essential for the respiratory depression following systemic administration of opioid analgesics. J. Physiol. 592 (6), 1159–1162. doi:10.1113/jphysiol.2013.261974
Neidhart P., Burgener M. C., Schwieger I., Suter P. M. (1989). Chest wall rigidity during fentanyl- and midazolam-fentanyl induction: ventilatory and haemodynamic effects. Acta Anaesthesiol. Scand. 33 (1), 1–5. doi:10.1111/j.1399-6576.1989.tb02849.x
Nielsen A. M., Bisgard G. E., Vidruk E. H. (1985). Carotid chemoreceptor activity during acute and sustained hypoxia in goats. J. Appl. Physiol. 65, 1796–1802. doi:10.1152/jappl.1988.65.4.1796
Nishizawa D., Mieda T., Tsujita M., Nakagawa H., Yamaguchi S., Kasai S., et al. (2023). Genome-wide association study identifies genetic polymorphisms associated with estimated minimum effective concentration of fentanyl in patients undergoing laparoscopic-assisted colectomy. Int. J. Mol. Sci. 24 (9), 8421. doi:10.3390/ijms24098421
Ozdemir D., Allain F., Kieffer B. L., Darcq E. (2023). Advances in the characterization of negative affect caused by acute and protracted opioid withdrawal using animal models. Neuropharmacology 232, 109524. doi:10.1016/j.neuropharm.2023.109524
Pergolizzi J. V., Raffa R. B., Rosenblatt M. H. (2020). Opioid withdrawal symptoms, a consequence of chronic opioid use and opioid use disorder: current understanding and approaches to management. J. Clin. Pharm. Ther. 45 (5), 892–903. doi:10.1111/jcpt.13114
Phua C. K., Wee A., Lim A., Abisheganaden J., Verma A. (2017). Fentanyl-induced chest wall rigidity syndrome in a routine bronchoscopy. Respir. Med. Case Rep. 20, 205–207. doi:10.1016/j.rmcr.2017.02.012
Scamman F. L. (1983). Fentanyl-O2-N2O rigidity and pulmonary compliance. Anesth. Analg. 62 (3), 332–334. doi:10.1213/00000539-198303000-00008
Smith J. C., Ellenberger H. H., Ballanyi K., Richter D. W., Feldman J. L. (1991). Pre-botzinger complex: A brainstem region that may generate respiratory rhythm in mammals. Science 254 (5032), 726–729. doi:10.1126/science.1683005
St John W. M. (1999). Rostral medullary respiratory neuronal activities of decerebrate cats in eupnea, apneusis and gasping. Respir. Physiol. 116 (1), 47–65. doi:10.1016/s0034-5687(99)00030-4
Streisand J. B., Bailey P. L., LeMaire L., Ashburn M. A., Tarver S. D., Varvel J., et al. (1993). Fentanyl-induced rigidity and unconsciousness in human volunteers. Incidence, duration, and plasma concentrations. Anesthesiology 78 (4), 629–634. doi:10.1097/00000542-199304000-00003
Torralva R., Janowsky A. (2019). Noradrenergic mechanisms in fentanyl-mediated rapid death explain failure of naloxone in the opioid crisis. J. Pharmacol. Exp. Ther. 371 (2), 453–475. doi:10.1124/jpet.119.258566
Uddin O., Jenne C., Fox M. E., Arakawa K., Keller A., Cramer N. (2021). Divergent profiles of fentanyl withdrawal and associated pain in mice and rats. Pharmacol. Biochem. Behav. 200, 173077. doi:10.1016/j.pbb.2020.173077
Varshneya N. B., Walentiny D. M., Moisa L. T., Walker T. D., Akinfiresoye L. R., Beardsley P. M. (2019). Opioid-like antinociceptive and locomotor effects of emerging fentanyl-related substances. Neuropharmacology 151, 171–179. doi:10.1016/j.neuropharm.2019.03.023
Weinger M. B., Bednarczyk J. M. (1994). Atipamezole, an alpha 2 antagonist, augments opiate-induced muscle rigidity in the rat. Pharmacol. Biochem. Behav. 49 (3), 523–529. doi:10.1016/0091-3057(94)90064-7
Weinger M. B., Cline E. J., Smith N. T., Blasco T. A., Koob G. F. (1988). Localization of brainstem sites which mediate alfentanil-induced muscle rigidity in the rat. Pharmacol. Biochem. Behav. 29 (3), 573–580. doi:10.1016/0091-3057(88)90022-6
Keywords: OIRD, control of breathing, fentanyl, ventilation, EMG
Citation: Neumueller SE, Buiter N, Hilbert G, Grams K, Taylor R, Desalvo J, Hodges GL, Hodges MM, Pan LG, Lewis SJ, Forster HV and Hodges MR (2023) Effects of sub-lethal doses of fentanyl on vital physiologic functions and withdrawal-like behaviors in adult goats. Front. Physiol. 14:1277601. doi: 10.3389/fphys.2023.1277601
Received: 14 August 2023; Accepted: 26 September 2023;
Published: 11 October 2023.
Edited by:
Gaspard Montandon, University of Toronto, CanadaReviewed by:
Nathan Andrew Baertsch, Seattle Children’s Research Institute, United StatesAdrienn G. Varga, University of Florida, United States
Copyright © 2023 Neumueller, Buiter, Hilbert, Grams, Taylor, Desalvo, Hodges, Hodges, Pan, Lewis, Forster and Hodges. This is an open-access article distributed under the terms of the Creative Commons Attribution License (CC BY). The use, distribution or reproduction in other forums is permitted, provided the original author(s) and the copyright owner(s) are credited and that the original publication in this journal is cited, in accordance with accepted academic practice. No use, distribution or reproduction is permitted which does not comply with these terms.
*Correspondence: Matthew R. Hodges, mhodges@mcw.edu