- 1Department of Endocrinology and Metabolism, The Affiliated Hospital of Qinghai University, Xining, China
- 2Department of Traumatology and Orthopaedics, The Affiliated Hospital of Qinghai University, Xining, China
Angiopoietin-like protein 8 (ANGPTL8) is a secreted protein predominantly expressed in liver and adipose tissue. ANGPTL8 modulates the clearance of triglycerides (TGs) by suppressing the activity of lipoprotein lipase (LPL) within the plasma. Previous studies found that circulating ANGPTL8 levels were significantly increased in metabolic disorder-related diseases, such as type 2 diabetes mellitus (T2DM), obesity, metabolic syndrome and nonalcoholic fatty liver disease (NAFLD). Whether ANGPTL8 has a direct pathogenic role in these diseases remains to be determined. In this review, we summarize the emerging roles of ANGPTL8 in the regulation of inflammation, tumours, circulatory system-related diseases, and ectopic lipid deposition, which may provide new insights into the diverse functions of ANGPTL8 in various diseases beyond its well-established functions in glucose and lipid metabolism.
1 Introduction
Angiopoietin-like protein 8 (ANGPTL8), also known as betatrophin, TD26, and lipasin, is released from the liver and adipose tissue (Guo et al., 2021). ANGPTL8 is a novel but atypical member of the ANGPTL family, as it lacks the typical fibrinogen-like domain. However, its N-terminal domain shares homology with ANGPTL3 and ANGPTL4 (Zhang, 2016; Guo et al., 2021). Previous studies have demonstrated the role of the ANGPTL3-4-8 model in inhibiting the activity of lipoprotein lipase (LPL), which is a rate-limiting enzyme for triglyceride (TG) hydrolysis and plasma TG clearance (Zhang, 2012; Zhang, 2016). Feeding induces ANGPTL8 expression and inhibits ANGPTL4 expression. Then, the ANGPTL8–ANGPTL3 pathway is activated and inhibits LPL in cardiac and skeletal muscles, allowing circulating TG to be taken up by white adipose tissue (WAT) to store energy. Conversely, ANGPTL8 levels are reduced during fasting, while ANGPTL4 levels are increased. Thus, TGs are directed towards the muscles to provide energy (Zhang, 2012; Zhang, 2016). The expression of ANGPTL8 is regulated by nutrition (Zhang, 2012), insulin (Lu et al., 2017) and thyroid hormone (Tseng et al., 2014). ANGPTL8 is closely associated with metabolic disorder-related diseases, such as type 2 diabetes mellitus (T2DM) (Zou et al., 2020a; Zou et al., 2020b), nonalcoholic fatty liver disease (NAFLD) (von Loeffelholz et al., 2017), obesity and metabolic syndrome (Mele et al., 2017; Wang et al., 2016). However, the precise roles of ANGPTL8 in these diseases are not known (Abu-Farha et al., 2020). Whether ANGPTL8 is involved in other pathophysiological processes beyond glucose and lipid metabolism remains to be explored. With the deepening of research, evolving implications of ANGPTL8 actions in the regulation of inflammation, tumours, circulatory system-related diseases, and ectopic lipid deposition have been reported. Therefore, in this work we review recent findings related to ANGPTL8 and aim to provide new insights into the diverse functions of ANGPTL8 in various diseases.
2 ANGPTL8 and inflammation
Previous studies have found that circulating ANGPTL8 levels are elevated in inflammation-related diseases, such as systemic inflammatory response syndrome (SIRS) (Zhang et al., 2017), T2DM (Chen et al., 2015), atherosclerosis (Jiao et al., 2018), and NAFLD (Vatner et al., 2018), and are associated with disease severity. However, the precise roles of ANGPTL8 in these diseases are not known. Zhang et al. (2017) found an increase in ANGPTL8 levels in patients with severe sepsis. Furthermore, they observed a notable connection between circulating ANGPTL8 levels and the acute inflammatory response induced by lipopolysaccharide (LPS) in mice (Zhang et al., 2017). Liao et al. (2019) demonstrated the detrimental role of ANGPTL8 expression in the pathogenesis of intervertebral disc degeneration (IDD), and knockdown of ANGPTL8 ameliorated IDD progression. (Figure 1) (Liao et al., 2019). They found that tumour necrosis factor-α (TNF-α) stimulated the expression of ANGPTL8 in bone marrow cells. Then, ANGPTL8 increased the production of matrix metalloproteinases (MMPs) and inflammatory cytokines while reducing the level of type II collagen in bone marrow cells through inhibition of NF-κB signalling activation. A previous study showed that fatty acids (FAs) upregulate ANGPTL8 expression within the liver, consequently triggering the expression and secretion of transforming growth factor-beta1 (TGFβ1) (Zhang et al., 2023). Subsequently, secreted TGFβ1 further stimulates ANGPTL8 expression. ANGPTL8 combines with the leukocyte immunoglobulin-like receptor B (LILRB2) receptor and then acts as a proinflammatory factor that stimulates hepatic stellate cells (HSCs). This then induces extracellular signal-regulated kinase (ERK) signalling and upregulates genes associated with liver fibrosis (Figure 1) (Zhang et al., 2023). However, another study demonstrated that ANGPTL8 bound with paired immunoglobulin-like receptor B (PirB)/LILRB2 on macrophages, but not HSCs, during nonalcoholic steatohepatitis (NASH) (Li et al., 2023). This PirB/LILRB2-ANGPTL8 interaction facilitated the conversion of hepatic macrophages into a proinflammatory phenotype by intensifying phosphorylation signals of P38, protein kinase B (AKT), and P65, thereby exacerbating hepatocyte lipid accumulation and propelling the progression from simple hepatic steatosis to steatohepatitis (Figure 1) (Li et al., 2023). Based on the above findings, ANGPTL8 may act as a proinflammatory factor.
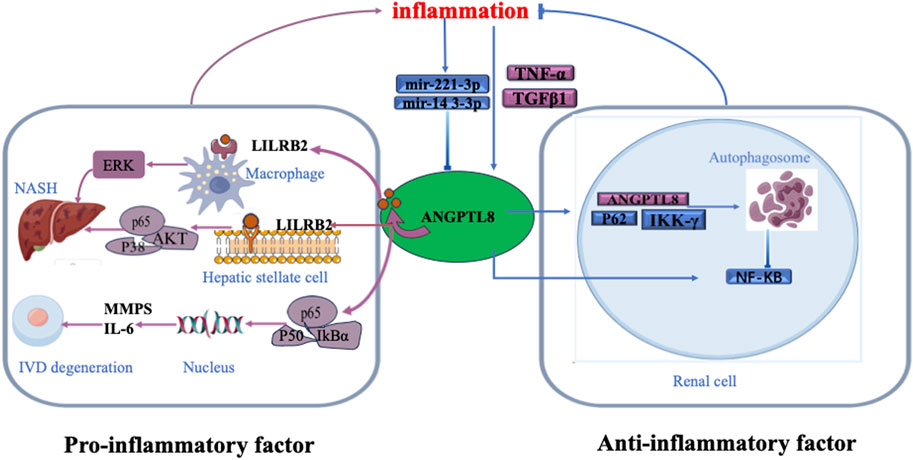
FIGURE 1. The role of ANGPTL8 in inflammation. Bladder urothelial carcinoma (BLCA), breast invasive carcinoma (BRCA), clear cell renal cell carcinoma (ccRcc), cholangiocarcinoma (CHOL), colon adenocarcinoma (COAD), glioblastoma (GBM), hepatocellular carcinoma (HCC), kidney chromophobe (KICH), kidney renal clear cell carcinoma (KIRC), lung adenocarcinoma (LUAD), lung squamous cell carcinoma (LUSC), pancreatic adenocarcinoma (PAAD), pheochromocytoma and paraganglioma (PCPG), rectum adenocarcinoma (READ, sarcoma (SARC), stomach adenocarcinoma (STAD), thymoma (THYM), uterine corpus endometrial carcinoma (UCEC), uterine carcinosarcoma (UCS), and uveal melanoma (UVM).
However, several other studies have suggested that ANGPTL8 may be a negative regulator of inflammation. ANGPTL8 was reported to exert a negative regulatory influence on nuclear factor kappa-B (NF-κB) by facilitating the autophagic degradation of inhibitory nuclear factor kinase κB-γ (IKKγ) within the cellular context (Zhang et al., 2017). Notably, NF-κB functions as a pivotal transcription factor within the intricate framework of inflammatory signalling (Zhang et al., 2017). Moreover, the well-established inducer of NF-κB activation, TNF-α, was observed to elevate ANGPTL8 expression in human hepatocytes (HepG2). ANGPTL8, through its interaction with sequestosome1 (p62) and autophagy receptors, facilitates targeted autophagic breakdown of IKKγ, thereby resulting in dampened NF-κB activity (Figure 1) (Zhang et al., 2017; Zhang et al., 2018). MicroRNAs (miRNAs) are small noncoding RNA molecules that regulate gene expression and have emerged as important components in the regulation of inflammation. MiR-221-3p is upregulated under inflammatory conditions, but there is a negative correlation between miR-221-3p in subcutaneous adipose tissue biopsy samples and plasma ANGPTL8 levels (Figure 1) (Mysore et al., 2017). Another miRNA, MiR-143-3p, has also been shown to be modulated by inflammation and downregulate ANGPTL8 in hepatocytes (Figure 1) (DiStefano, 2019). These studies indicated that ANGPTL8 may serve as a “brake” in certain inflammatory pathways. As shown in Figure 1, the dual roles of ANGPTL8 in inflammation suggest potential divergence in its intracellular function compared to its role as a secreted hormone in other physiological contexts, such as blood vessels (Abu-Farha et al., 2020).
3 ANGPTL8 and cancer
Increasing evidence suggests that ANGPTL8 is associated with cancer development and prognosis. However, ANGPLT8 levels and the association between ANGPTL8 and prognosis also differ among various cancers.
3.1 Liver cancer
ANGPTL8 is secreted by the liver and acts as an inflammatory factor in the pathophysiology of liver cancer. Initial investigations have established an association between increased serum concentrations of ANGPTL8 and NAFLD and its severity (von Loeffelholz et al., 2017; Lee et al., 2016; Cengiz et al., 2016). Furthermore, ANGPTL8 expression levels are also elevated in the livers of NAFLD mice (Lee et al., 2016), which is potentially attributable to its involvement in glucose and lipid metabolic pathways. NAFLD can further progress to liver fibrosis and liver cancer. Clinical investigations have identified elevated serum ANGPTL8 levels in individuals with liver fibrosis and liver cancer (Vatner et al., 2018; Pazgan-Simon et al., 2020; Wang et al., 2018). In the study by Wang et al. (2018), ANGPTL8 was highly expressed in hepatocellular carcinoma (HCC) tissues when compared to matched normal tissues, emerging as an independent predictor of overall survival and recurrence-free survival among HCC patients. ANGPTL8 significantly augments lipid synthesis within HCC cells and promotes tumour growth and metastasis by modulating the immune response within the tumour microenvironment; therefore, it was named “tumour-derived factor 26” (TD26) (Wang et al., 2018). Zhang et al. (2023) also found that the serum concentrations of ANGPTL8 in patients with liver cirrhosis and HCC increased by 1.57-fold and 2.14-fold, respectively. As discussed above, their findings indicated that ANGPTL8 expedites hepatic fibrosis through the LILRB2/ERK signalling pathways in HSCs. According to Gao et al. (2023), ANGPTL8 plays a dual role in hepatocarcinogenesis by promoting both tumour cell proliferation and immune evasion: 1) In hepatocytes, ANGPTL8-triggered LILRB2/PIRB activation regulates the ROS/ERK pathway, upregulating autophagy and promoting HCC cell growth; 2) In macrophages, the LILRB2/PIRB-ANGPTL8 interaction drives polarization towards the M2 phenotype, leading to suppression of the antitumour immune response within the tumour microenvironment, ultimately allowing tumorigenic HCC cells to evade immune surveillance (Gao et al., 2023). Nevertheless, Li et al. (2023) investigated PirB expression across various cell types in liver tissue (hepatocytes, HSCs, endothelial cells, and hepatic immunocytes) and determined that PirB is predominantly expressed in hepatic macrophages. Therefore, the effect of the PirB/LILRB2-ANGPTL8 interaction in different cell types in liver tissue remains to be further investigated.
However, another study suggested that the elevated expression of ANGPTL8 in HCC cells may inhibit cell proliferation by affecting the Wnt signalling pathway, upregulating the tumour suppressor gene WNT inhibitory factor-1 (WIF1) and downregulating β-catenin (Monzavi et al., 2019). In their opinion, ANGPTL8 may act as a moderate suppressor of human liver carcinoma cells.
3.2 Renal cell carcinoma
Our prospective study found that serum levels of ANGPTL8 are associated with renal dysfunction (Zou et al., 2021), consistent with other observational studies (Maurer et al., 2017). It has been suggested that ANGPTL8 may be an advanced endocrine regulatory factor involved in the development of diabetic kidney disease (Chen et al., 2016), which suggests that ANGPTL8 is closely associated with renal disease.
Renal cell carcinoma (RCC) is the most common type of kidney cancer, and clear cell renal cell carcinoma (ccRCC) constitutes approximately 70% of all renal cancer cases and correlates with chronic inflammation (Fox et al., 2013). ANGPTL8 gene expression was observed to be significantly increased in ccRCC tissues (Matsukawa et al., 2023). They found that ANGPTL8 was induced by inflammation and then sustained the generation of chemokines via the NF-κB signalling pathway (Matsukawa et al., 2023). Consequently, these chemokines attract immune suppressor cells to the tumour microenvironment and maintain the undifferentiated status of ccRCC cells. These results indicate that ANGPTL8 can promote tumour progression by establishing the tumour microenvironment. It was reported that patients with high ANGPTL8 expression were associated with a lower 5-year survival rate of kidney renal clear cell carcinoma (KIRC)/ccRCC (Xu et al., 2021). Based on the above, ANGPTL8 may serve as a novel unfavourable prognostic marker in renal cancer. However, the protein expression of ANGPTL8 displayed opposite results in ccRCC/KIRC samples from patients in different cancer stages, years old age groups and weight categories (Xu et al., 2021). The apparently contradictory results in gene and protein expression of ANGPTL8/betatrophin in ccRCC/KIRC must be further validated.
3.3 Other cancers
As shown in Table 1, analysis of various databases has shown significantly decreased expression of the ANGPTL8 gene in lung squamous cell carcinoma (LUSC), lung adenocarcinoma (LUAD), breast invasive carcinoma (BRCA), glioblastoma (GBM), cholangiocarcinoma (CHOL), uterine corpus endometrial carcinoma (UCEC), bladder urothelial carcinoma (BLCA), and kidney chromophobe (KICH) compared to their respective normal samples (Xu et al., 2021; Iqbal et al., 2023). Conversely, ANGPTL8 levels are significantly increased in HCC (Wang et al., 2018), KIRC/ccRCC (Matsukawa et al., 2023; Xu et al., 2021), colon adenocarcinoma (COAD), rectum adenocarcinoma (READ), and stomach adenocarcinoma (STAD) (Xu et al., 2021). However, the gene expression of ANGPTL8 was decreased in CHOL, BRCA, LUAD, LUSC, UCEC, BLCA KICH, GBM, and UCEC (Table 1) (Xu et al., 2021; Iqbal et al., 2023). It has also been found that ANGPTL8 levels are elevated in pancreatic adenocarcinoma (PAAD)-associated diabetes patients (Susanto et al., 2016). Furthermore, ANGPTL8 levels were associated with poor prognosis in KIRC, UCEC, and LUSC patients (Xu et al., 2021) but with longer overall survival or disease-free survival time in CHOL, GBM, sarcoma (SARC), uveal melanoma (UVM), BLCA, BRCA, pheochromocytoma and paraganglioma (PCPG), thymoma (THYM), uterine carcinosarcoma (UCS) patients (Xu et al., 2021; Iqbal et al., 2023) and PAAD (Taherkhani et al., 2020) (Table 1). An integrated computational analysis revealed that 4 SNPs in ANGPTL8 were predicted to be involved in large intestine, breast, and liver cancer (Iqbal et al., 2023).
The potential mechanism of ANGPTL8 in cancers has not yet reached a consensus. ANGPTL8 may be induced by STAT3 signalling (Matsukawa et al., 2023), FFAs (Liu et al., 2018; Zhang et al., 2023) or other proinflammatory cytokines, such as TNF-α (Zhang et al., 2017) and TGFβ1, in the tumour microenvironment, and it may affect the differentiation and development of tumours through the following pathways. First, ANGPTL8 may inhibit cell proliferation and promote apoptosis by affecting the Wnt signalling pathway, increasing the expression of the tumour suppressor gene WIF1 and downregulating the antiapoptotic gene Bcl2 (Monzavi et al., 2019; Taherkhani et al., 2020). Second, the interaction of the C-terminus of ANGPTL8 with sterol regulatory element binding protein 1 (SREBP1) inhibits protein kinase activated by adenosine 5′-monophosphate (AMP)-activated protein kinases (AMPK) (Navaeian et al., 2021; Lee et al., 2015); therefore, they increase lipogenesis and tumour growth and enhance tumour progression (Navaeian et al., 2021). Third, as mentioned earlier, ANGPTL8 negatively regulates inflammation and autophagy by inhibiting NF-κB activation, which was confirmed to be crucial to the progression of cancer (Perkins, 2012; DiDonato et al., 2012). Fourth, the ANGPTL protein family is also associated with the regulation of stem cell activity (Endo, 2019; Zheng et al., 2012; Santulli, 2014). Therefore, ANGPTL8 may affect cell proliferation by modulating stem cell activity. Fifth, ANGPTL8 was demonstrated to engage with 24 amino acid residues within RASSF5 proteins. These proteins play vital roles in various cellular processes, including the regulation of the cell cycle, cell proliferation, and differentiation (Iqbal et al., 2023). Furthermore, the deactivation of RASSF5 has been associated with the initiation of oncogenic processes (Iqbal et al., 2023). In summary, the potential role of ANGPTL8 in tumours may be complicated and involve multiple pathways.
4 ANGPTL8 and the circulatory system
ANGPTL8, a factor involved in regulating lipid metabolism, serves as a potential biomarker for metabolic diseases, which are closely associated with cardiovascular diseases. Recently, it was also reported to be involved in the pathophysiological processes of circulatory system-related diseases as an inflammatory factor.
Atherosclerosis is a major cause of cardiovascular disease (Brandsma et al., 2019). Atherosclerosis has been extensively linked to lipid infiltration and inflammation in numerous investigations (Libby, 2021). Notably, studies have revealed elevated serum ANGPTL8 levels in coronary heart disease patients relative to their healthy counterparts, and a positive correlation was observed between ANGPTL8 levels and disease severity (Jiao et al., 2018). This connection has spurred researchers to investigate the role of ANGPTL8 in atherosclerosis progression. Findings have indicated an upregulation of ANGPTL8 expression in atherosclerotic mice, and the improvement of atherosclerosis was observed after knocking out ANGPTL8 (Zheng et al., 2018). Jiao et al. (2018) investigated the location of ANGPTL8 in atherosclerosis using immunofluorescence and found that ANGPTL8 is expressed in macrophages within atherosclerotic lesions. Overexpression of ANGPTL8 in macrophages led to foam cell formation and extensive cholesterol deposition, promoting the development of atherosclerosis (Jiao et al., 2020); this suggests that the influence of ANGPTL8 on blood vessels is not solely through the regulation of triglyceride levels. Additionally, studies have shown that inhibiting ANGPTL8 using second-generation 2′-O-methoxyethyl (ASO) can prevent cardiovascular disease (Morelli et al., 2020). However, contrary to the mainstream notion, a prospective study of 553 coronary heart disease patients followed for 8 years indicated that elevated plasma levels of ANGPTL8 have a protective effect on the heart and are associated with a lower risk of cardiovascular events and mortality (Leiherer et al., 2020). The specific mechanisms require further investigation.
4.1 Hypertension
Circulating ANGPTL8 levels were significantly higher in hypertensive patients, as well as in mice and rats (Abu-Farha et al., 2018; Xu et al., 2023; Jiao et al., 2023). It is generally believed that this association is due to its role in LPL inhibition. Jiao et al. (2023) reported that targeted removal of ANGPTL8 in vascular smooth muscle cells (VSMCs) mitigated AngII-induced hypertension and hypertensive cardiovascular remodelling. They demonstrated that ANGPTL8 regulates hypertension and that arterial remodelling involves accelerated constriction, proliferation, and migration of VSMCs through the promotion of PI3K-AkT pathway activation.
4.2 Cardiomyopathy
Serum ANGPTL8 levels were elevated in patients with hypertensive left ventricular hypertrophy (Hu et al., 2022). ANGPTL8, as an endocrine protein, is not expressed in cardiomyocytes but is mainly expressed in primary mouse liver cells and HepG2 cells. In mice with angiotensin II (Ang II)-induced cardiac hypertrophy or transverse aorta constriction (TAC)-induced cardiac hypertrophy, serum ANGPTL8 levels were also significantly increased (Su et al., 2021). Importantly, these mice do not exhibit lipid metabolism disorders, suggesting that the impact of ANGPTL8 on the heart is not mediated through the regulation of blood lipids but may involve direct effects on cardiomyocytes. Hu et al. (2022) found that ANGPTL8 can bind to LILRB3 and block downstream signalling pathways, such as those of AKT and glycogen synthase kinase-3β (GSK3β), thereby improving pathological cardiac hypertrophy. ANGPTL8 deficiency accelerates Ang II-induced cardiac hypertrophy and fibrosis (Su et al., 2021). Wang et al. (2022) also discovered a negative correlation between ANGPTL8 and left ventricular mass index (LVMI), indicating a protective effect of ANGPTL8 on cardiac remodelling. Thus, cardiac hypertrophy may upregulate the expression of ANGPTL8 through certain mechanisms, and circulating ANGPTL8 can directly act on cardiomyocytes to exert anti-ventricular remodelling effects.
5 ANGPTL8 and ectopic fat deposition
Mesenchymal stem cells (MSCs) are multipotent stem cells with self-renewal and multilineage differentiation potential, including differentiation into adipocytes (Fu et al., 2019). When body fat exceeds the normal threshold, MSCs differentiate into adipocytes to meet the body’s energy storage needs. Tang et al. (2022) demonstrated that ANGPTL8 promotes adipogenic differentiation of MSCs in liver, kidney, and heart tissues by inhibiting the Wnt/β-catenin signalling pathway. This upregulates the expression of peroxisome proliferator-activated receptor γ (PPARγ) and c/EBPα and leads to bone marrow fat deposition (Sun et al., 2017). Ectopic bone marrow fat deposition is closely related to chronic metabolic diseases, such as T2DM, NAFLD, and obesity (Tang et al., 2022).
6 Conclusion
In summary, ANGPTL8 is primarily secreted by the liver and adipocytes. Previous studies have mainly focused on improving lipid profiles in individuals with metabolic risk factors by creating antibodies targeting ANGPTL3, ANGPTL4, and ANGPTL8. In recent years, it has been discovered that ANGPTL8 exerts different biological effects in different locations (including within and outside blood vessels and target cells) and participates in various pathological processes, such as inflammation, tumour processes, ventricular remodelling, and ectopic fat deposition. These findings suggest that ANGPTL8 may be involved in metabolism or inflammation across multiple organ systems. Further research is needed to investigate the effects of ANGPTL8 in various disease states, its cellular and extracellular actions, and its precise molecular mechanisms.
Author contributions
HY: Data curation, Investigation, Methodology, Writing–original draft, Writing–review and editing. QZ: Conceptualization, Data curation, Formal Analysis, Methodology, Validation, Writing–original draft, Writing–review and editing. RZ: Conceptualization, Investigation, Supervision, Writing–review and editing. HZ: Conceptualization, Formal Analysis, Funding acquisition, Investigation, Methodology, Project administration, Supervision, Writing–review and editing.
Funding
The author(s) declare financial support was received for the research, authorship, and/or publication of this article. This work was supported by the National Natural Science Foundation of China (82260172), the Natural Science Foundation of the Department of Science and Technology of Qinghai Province (2023-ZJ-959Q), and grants from the Qinghai University Youth Scientific Research Fund Project (2022-QYY-2).
Conflict of interest
The authors declare that the research was conducted in the absence of any commercial or financial relationships that could be construed as a potential conflict of interest.
Publisher’s note
All claims expressed in this article are solely those of the authors and do not necessarily represent those of their affiliated organizations, or those of the publisher, the editors and the reviewers. Any product that may be evaluated in this article, or claim that may be made by its manufacturer, is not guaranteed or endorsed by the publisher.
References
Abu-Farha M., Cherian P., Qaddoumi M. G., AlKhairi I., Sriraman D., Alanbaei M., et al. (2018). Increased plasma and adipose tissue levels of ANGPTL8/Betatrophin and ANGPTL4 in people with hypertension. Lipids health Dis. 17 (1), 35. doi:10.1186/s12944-018-0681-0
Abu-Farha M., Ghosh A., Al-Khairi I., Madiraju S. R. M., Abubaker J., Prentki M. (2020). The multi-faces of Angptl8 in health and disease: novel functions beyond lipoprotein lipase modulation. Prog. lipid Res. 80, 101067. doi:10.1016/j.plipres.2020.101067
Brandsma E., Kloosterhuis N. J., Koster M., Dekker D. C., Gijbels M. J. J., van der Velden S., et al. (2019). A proinflammatory gut microbiota increases systemic inflammation and accelerates atherosclerosis. Circulation Res. 124 (1), 94–100. doi:10.1161/CIRCRESAHA.118.313234
Cengiz M., Ozenirler S., Kocabiyik M. (2016). Serum β-trophin level as a new marker for noninvasive assessment of nonalcoholic fatty liver disease and liver fibrosis. Eur. J. gastroenterology hepatology 28 (1), 57–63. doi:10.1097/MEG.0000000000000502
Chen C. C., Susanto H., Chuang W. H., Liu T. Y., Wang C. H. (2016). Higher serum betatrophin level in type 2 diabetes subjects is associated with urinary albumin excretion and renal function. Cardiovasc. Diabetol. 15, 3. doi:10.1186/s12933-015-0326-9
Chen X., Lu P., He W., Zhang J., Liu L., Yang Y., et al. (2015). Circulating betatrophin levels are increased in patients with type 2 diabetes and associated with insulin resistance. J. Clin. Endocrinol. metabolism 100 (1), E96–E100. doi:10.1210/jc.2014-2300
DiDonato J. A., Mercurio F., Karin M. (2012). NF-κB and the link between inflammation and cancer. Immunol. Rev. 246 (1), 379–400. doi:10.1111/j.1600-065X.2012.01099.x
DiStefano J. K. (2019). Angiopoietin-like 8 (ANGPTL8) expression is regulated by miR-143-3p in human hepatocytes. Gene 681, 1–6. doi:10.1016/j.gene.2018.09.041
Endo M. (2019). The roles of ANGPTL families in cancer progression. J. UOEH 41 (3), 317–325. doi:10.7888/juoeh.41.317
Fox P., Hudson M., Brown C., Lord S., Gebski V., De Souza P., et al. (2013). Markers of systemic inflammation predict survival in patients with advanced renal cell cancer. Br. J. cancer 109 (1), 147–153. doi:10.1038/bjc.2013.300
Fu X., Liu G., Halim A., Ju Y., Luo Q., Song A. G., et al. (2019). Mesenchymal stem cell migration and tissue repair. Cells 8 (8), 784. doi:10.3390/cells8080784
Gao Y., Yuan Y., Wen S., Chen Y., Zhang Z., Feng Y., et al. (2023). Dual role of ANGPTL8 in promoting tumor cell proliferation and immune escape during hepatocarcinogenesis. Oncogenesis 12 (1), 26. doi:10.1038/s41389-023-00473-3
Guo C., Wang C., Deng X., He J., Yang L., Yuan G. (2021). ANGPTL8 in metabolic homeostasis: more friend than foe? Open Biol. 11 (9), 210106. doi:10.1098/rsob.210106
Hu L., Wei J., Zhang Y., Wang Z., Tang J., Tang J., et al. (2022). ANGPTL8 is a negative regulator in pathological cardiac hypertrophy. Cell death Dis. 13 (7), 621. doi:10.1038/s41419-022-05029-8
Iqbal S., Begum F., Nyamai D. W., Jalal N., Shaw P. (2023). An integrated computational analysis of high-risk SNPs in angiopoietin-like proteins (ANGPTL3 and ANGPTL8) reveals perturbed protein dynamics associated with cancer. Mol. (Basel, Switz. 28 (12), 4648. doi:10.3390/molecules28124648
Jiao X., He J., Yang Y., Yang S., Li J., Qin Y. (2018). Associations between circulating full-length angiopoietin-like protein 8 levels and severity of coronary artery disease in Chinese non-diabetic patients: a case-control study. Cardiovasc. Diabetol. 17 (1), 92. doi:10.1186/s12933-018-0736-6
Jiao X., Yang Y., Li L., Yu H., Yang Y., Li J., et al. (2020). Angiopoietin-like protein 8 accelerates atherosclerosis in ApoE−/− mice. Atherosclerosis 307, 63–71. doi:10.1016/j.atherosclerosis.2020.06.014
Jiao X., Yu H., Du Z., Li L., Hu C., Du Y., et al. (2023). Vascular smooth muscle cells specific deletion of angiopoietin-like protein 8 prevents angiotensin II-promoted hypertension and cardiovascular hypertrophy. Cardiovasc. Res. 119 (9), 1856–1868. doi:10.1093/cvr/cvad089
Lee J., Hong S. W., Park S. E., Rhee E. J., Park C. Y., Oh K. W., et al. (2015). AMP-activated protein kinase suppresses the expression of LXR/SREBP-1 signaling-induced ANGPTL8 in HepG2 cells. Mol. Cell. Endocrinol. 414, 148–155. doi:10.1016/j.mce.2015.07.031
Lee Y. H., Lee S. G., Lee C. J., Kim S. H., Song Y. M., Yoon M. R., et al. (2016). Association between betatrophin/ANGPTL8 and non-alcoholic fatty liver disease: animal and human studies. Sci. Rep. 6, 24013. doi:10.1038/srep24013
Leiherer A., Ebner J., Muendlein A., Brandtner E. M., Zach C., Geiger K., et al. (2020). High betatrophin in coronary patients protects from cardiovascular events. Atherosclerosis 293, 62–68. doi:10.1016/j.atherosclerosis.2019.11.011
Li D. P., Huang L., Kan R. R., Meng X. Y., Wang S. Y., Zou H. J., et al. (2023). LILRB2/PirB mediates macrophage recruitment in fibrogenesis of nonalcoholic steatohepatitis. Nat. Commun. 14 (1), 4436. doi:10.1038/s41467-023-40183-3
Liao Z., Wu X., Song Y., Luo R., Yin H., Zhan S., et al. (2019). Angiopoietin-like protein 8 expression and association with extracellular matrix metabolism and inflammation during intervertebral disc degeneration. J. Cell. Mol. Med. 23 (8), 5737–5750. doi:10.1111/jcmm.14488
Libby P. (2021). The changing landscape of atherosclerosis. Nature 592 (7855), 524–533. doi:10.1038/s41586-021-03392-8
Liu X., Liang Y., Song R., Yang G., Han J., Lan Y., et al. (2018). Long non-coding RNA NEAT1-modulated abnormal lipolysis via ATGL drives hepatocellular carcinoma proliferation. Mol. cancer 17 (1), 90. doi:10.1186/s12943-018-0838-5
Lu P., Chen X., Zhang Z., Zhang J., Yang Y., Liu Z., et al. (2017). Insulin upregulates betatrophin expression via PI3K/Akt pathway. Sci. Rep. 7 (1), 5594. doi:10.1038/s41598-017-06052-y
Matsukawa T., Doi T., Obayashi K., Sumida K., Fujimoto N., Endo M. (2023). ANGPTL8 links inflammation and poor differentiation, which are characteristics of malignant renal cell carcinoma. Cancer Sci. 114 (4), 1410–1422. doi:10.1111/cas.15700
Maurer L., Schwarz F., Fischer-Rosinsky A., Schlueter N., Brachs S., Möhlig M., et al. (2017). Renal function is independently associated with circulating betatrophin. PloS one 12 (3), e0173197. doi:10.1371/journal.pone.0173197
Mele C., Grugni G., Mai S., Vietti R., Aimaretti G., Scacchi M., et al. (2017). Circulating angiopoietin-like 8 (ANGPTL8) is a marker of liver steatosis and is negatively regulated by Prader-Willi Syndrome. Sci. Rep. 7 (1), 3186. doi:10.1038/s41598-017-03538-7
Monzavi N., Zargar S. J., Gheibi N., Azad M., Rahmani B. (2019). Angiopoietin-like protein 8 (betatrophin) may inhibit hepatocellular carcinoma through suppressing of the Wnt signaling pathway. Iran. J. basic Med. Sci. 22 (10), 1166–1171. doi:10.22038/ijbms.2019.36612.8764
Morelli M. B., Chavez C., Santulli G. (2020). Angiopoietin-like proteins as therapeutic targets for cardiovascular disease: focus on lipid disorders. Expert Opin. Ther. targets 24 (1), 79–88. doi:10.1080/14728222.2020.1707806
Mysore R., Ortega F. J., Latorre J., Ahonen M., Savolainen-Peltonen H., Fischer-Posovszky P., et al. (2017). MicroRNA-221-3p regulates angiopoietin-like 8 (ANGPTL8) expression in adipocytes. J. Clin. Endocrinol. metabolism 102 (11), 4001–4012. doi:10.1210/jc.2017-00453
Navaeian M., Asadian S., Ahmadpour Yazdi H., Gheibi N. (2021). ANGPTL8 roles in proliferation, metabolic diseases, hypothyroidism, polycystic ovary syndrome, and signaling pathways. Mol. Biol. Rep. 48 (4), 3719–3731. doi:10.1007/s11033-021-06270-8
Pazgan-Simon M., Zuwala-Jagiello J., Menzyk T., Bator M., Derra A., Lekstan A., et al. (2020). Serum betatrophin and irisin levels in hepatocellular carcinoma. J. physiology Pharmacol. 71 (1), 10–26402. doi:10.26402/jpp.2020.1.11
Perkins N. D. (2012). The diverse and complex roles of NF-κB subunits in cancer. Nat. Rev. Cancer 12 (2), 121–132. doi:10.1038/nrc3204
Santulli G. (2014). Angiopoietin-like proteins: a comprehensive look. Front. Endocrinol. 5, 4. doi:10.3389/fendo.2014.00004
Su X., Cheng Y., Wang B. (2021). ANGPTL8 in cardio-metabolic diseases. Clin. chimica acta; Int. J. Clin. Chem. 519, 260–266. doi:10.1016/j.cca.2021.05.017
Sun L. L., Liu T. J., Li L., Tang W., Zou J. J., Chen X. F., et al. (2017). Transplantation of betatrophin-expressing adipose-derived mesenchymal stem cells induces β-cell proliferation in diabetic mice. Int. J. Mol. Med. 39 (4), 936–948. doi:10.3892/ijmm.2017.2914
Susanto H., Liu T. Y., Chen C. C., Purnomo J. D., Chen S. F., Wang C. H. (2016). Increased serum levels of betatrophin in pancreatic cancer-associated diabetes. Oncotarget 7 (27), 42330–42339. doi:10.18632/oncotarget.9815
Taherkhani F., Hosseini K. M., Zebardast S., Chegini K. G., Gheibi N. (2020). Anti proliferative and apoptotic effects on pancreatic cancer cell lines indicate new roles for ANGPTL8 (Betatrophin). Genet. Mol. Biol. 43 (3), e20190196. doi:10.1590/1678-4685-GMB-2019-0196
Tang J., Ma S., Gao Y., Zeng F., Feng Y., Guo C., et al. (2022). ANGPTL8 promotes adipogenic differentiation of mesenchymal stem cells: potential role in ectopic lipid deposition. Front. Endocrinol. 13, 927763. doi:10.3389/fendo.2022.927763
Tseng Y. H., Ke P. Y., Liao C. J., Wu S. M., Chi H. C., Tsai C. Y., et al. (2014). Chromosome 19 open reading frame 80 is upregulated by thyroid hormone and modulates autophagy and lipid metabolism. Autophagy 10 (1), 20–31. doi:10.4161/auto.26126
Vatner D. F., Goedeke L., Camporez J. G., Lyu K., Nasiri A. R., Zhang D., et al. (2018). Angptl8 antisense oligonucleotide improves adipose lipid metabolism and prevents diet-induced NAFLD and hepatic insulin resistance in rodents. Diabetologia 61 (6), 1435–1446. doi:10.1007/s00125-018-4579-1
von Loeffelholz C., Pfeiffer A. F. H., Lock J. F., Lieske S., Döcke S., Murahovschi V., et al. (2017). ANGPTL8 (betatrophin) is expressed in visceral adipose tissue and relates to human hepatic steatosis in two independent clinical collectives. Hormone metabolic Res. 49 (5), 343–349. doi:10.1055/s-0043-102950
Wang C., Tong Y., Wen Y., Cai J., Guo H., Huang L., et al. (2018). Hepatocellular carcinoma-associated protein TD26 interacts and enhances sterol regulatory element-binding protein 1 activity to promote tumor cell proliferation and growth. Hepatol. Baltim. Md) 68 (5), 1833–1850. doi:10.1002/hep.30030
Wang D., Feng D., Wang Y., Dong P., Wang Y., Zhong L., et al. (2022). Angiopoietin-like protein 8/leptin crosstalk influences cardiac mass in youths with cardiometabolic risk: the BCAMS study. Front. Endocrinol. 12, 788549. doi:10.3389/fendo.2021.788549
Wang H., Lai Y., Han C., Liu A., Fan C., Wang H., et al. (2016). The effects of serum ANGPTL8/betatrophin on the risk of developing the metabolic syndrome - a prospective study. Sci. Rep. 6, 28431. doi:10.1038/srep28431
Xu F., Shen L., Yang Y., Kong L., Zu W., Tian D., et al. (2023). Association between plasma levels of ANGPTL3, 4, 8 and the most common additional cardiovascular risk factors in patients with hypertension. Diabetes, metabolic syndrome Obes. targets Ther. 16, 1647–1655. doi:10.2147/DMSO.S411483
Xu F., Tian D., Shi X., Sun K., Chen Y. (2021). Analysis of the expression and prognostic potential of a novel metabolic regulator ANGPTL8/betatrophin in human cancers. Pathology Oncol. Res. POR 27, 1609914. doi:10.3389/pore.2021.1609914
Zhang R. (2012). Lipasin, a novel nutritionally-regulated liver-enriched factor that regulates serum triglyceride levels. Biochem. biophysical Res. Commun. 424 (4), 786–792. doi:10.1016/j.bbrc.2012.07.038
Zhang R. (2016). The ANGPTL3-4-8 model, a molecular mechanism for triglyceride trafficking. Open Biol. 6 (4), 150272. doi:10.1098/rsob.150272
Zhang Y., Guo X., Yan W., Chen Y., Ke M., Cheng C., et al. (2017). ANGPTL8 negatively regulates NF-κB activation by facilitating selective autophagic degradation of IKKγ. Nat. Commun. 8 (1), 2164. doi:10.1038/s41467-017-02355-w
Zhang Y., Zheng L., Huang K. (2018). A new way to regulate inflammation: selective autophagic degradation of IKKγ mediated by ANGPTL8. Cell stress 2 (3), 66–68. doi:10.15698/cst2018.03.128
Zhang Z., Yuan Y., Hu L., Tang J., Meng Z., Dai L., et al. (2023). ANGPTL8 accelerates liver fibrosis mediated by HFD-induced inflammatory activity via LILRB2/ERK signaling pathways. J. Adv. Res. 47, 41–56. doi:10.1016/j.jare.2022.08.006
Zheng J., Umikawa M., Cui C., Li J., Chen X., Zhang C., et al. (2012). Inhibitory receptors bind ANGPTLs and support blood stem cells and leukaemia development. Nature 485 (7400), 656–660. doi:10.1038/nature11095
Zheng T., Ge B., Liu H., Chen B., Qin L., Xiao L., et al. (2018). Triglyceride-mediated influence of serum angiopoietin-like protein 8 on subclinical atherosclerosis in type 2 diabetic patients: results from the GDMD study in China. Cardiovasc. Diabetol. 17 (1), 84. doi:10.1186/s12933-018-0687-y
Zou H., Duan W., Zhang Z., Chen X., Lu P., Yu X. (2020a). The circulating ANGPTL8 levels show differences among novel subgroups of adult patients with diabetes and are associated with mortality in the subsequent 5 years. Sci. Rep. 10 (1), 12859. doi:10.1038/s41598-020-69091-y
Zou H., Xu Y., Chen X., Yin P., Li D., Li W., et al. (2020b). Predictive values of ANGPTL8 on risk of all-cause mortality in diabetic patients: results from the REACTION Study. Cardiovasc. Diabetol. 19 (1), 121. doi:10.1186/s12933-020-01103-7
Keywords: ANGPTL8, inflammation, tumour, circulatory system, ectopic lipid deposition
Citation: Ye H, Zong Q, Zou H and Zhang R (2023) Emerging insights into the roles of ANGPTL8 beyond glucose and lipid metabolism. Front. Physiol. 14:1275485. doi: 10.3389/fphys.2023.1275485
Received: 13 August 2023; Accepted: 15 November 2023;
Published: 01 December 2023.
Edited by:
Dmitri Samovski, Washington University in St. Louis, United StatesReviewed by:
Weiqin Chen, Augusta University, United StatesLing Yang, Temple University, United States
Copyright © 2023 Ye, Zong, Zou and Zhang. This is an open-access article distributed under the terms of the Creative Commons Attribution License (CC BY). The use, distribution or reproduction in other forums is permitted, provided the original author(s) and the copyright owner(s) are credited and that the original publication in this journal is cited, in accordance with accepted academic practice. No use, distribution or reproduction is permitted which does not comply with these terms.
*Correspondence: Huajie Zou, hjzou0406@163.com; Ruixia Zhang, qhzrx@126.com
†These authors have contributed equally to this work and share first authorship