- 1Department of Cardiology, Yanan Hospital Affiliated to Kunming Medical University, Kunming, Yunnan, China
- 2Department of Cardiology, Zhongshan Hospital Wusong Branch, Fudan University, Shanghai, China
- 3PKU-HKUST Shenzhen-Hong Kong Institution, Shenzhen, Guangdong, China
- 4Institute of Mechanobiology and Medical Engineering, School of Life Sciences and Biotechnology, Shanghai Jiao Tong University, Shanghai, China
Myocardial infarction (MI) impaired both cardiac functions and peripheral arteries. The changes in normal and shear stresses in the peripheral artery wall are of importance for understanding the progression of MI-induced heart failure (HF). The aim of the study is to investigate the corresponding changes of normal and shear stresses. The coronary artery ligation was used to induce the MI in Wistar rats. The analysis of wall mechanics and hemodynamics was performed based on in vivo and in vitro measurements. Myocardial infarction increased wall stiffness in elastic carotid and muscular femoral arteries significantly albeit different changes occurred between the two vessels from 3 to 6 weeks postoperatively. Moreover, the hemodynamic analysis showed the gradually deteriorated wall shear stress, oscillatory shear index and relative residence time in the two arteries. This study probably shed light on understanding the interaction between abnormal systemic circulation and peripheral mechanics and hemodynamics during the development of MI-induced HF.
1 Introduction
Myocardial infarction (MI) induces ischemia that could further cause heart failure (HF) with reduced ejection fraction (HFrEF) (Humeres and Frangogiannis, 2019). Mechanical property of vessel wall and hemodynamics in peripheral arteries are important elements that affected the occurrence and progression of MI-induced HFrEF (Kassab, 2006; Huo and Li, 2022). Poelzl et al. showed that severe HF was associated with brachial artery remodeling, characterized by morphological, mechanical, and functional changes in the vessel wall (Poelzl et al., 2005). Wang et al. showed that MI resulted in the high peripheral resistance, pulse wave velocity (PWV) and augmentation index (AIx) and the low total compliance as well as a significant increase of myocardial fibers and peripheral artery wall collagens (Wang et al., 2022).
The changes in normal and shear stresses in the peripheral artery wall should be taken into account to investigate basic mechanisms for the occurrence and progression of MI-induced HFrEF (Miyoshi and Ito, 2021). Mechanical tests have been used to characterize the stress-tension response of arteries and provide sufficient data for mathematical modeling of their behavior (Humphrey and Canham, 2000; Humphrey, 2002; 2008; Huo et al., 2012; Huo et al., 2013; Lu et al., 2017; Feng et al., 2021). The Womersley model has also been applied to the analysis of pulsatile flow velocity profile, wall shear stress (WSS), oscillatory shear index (OSI) and relative residence time (RRT) in aorta and peripheral arteries (Bing et al., 2020; Li et al., 2021). Hence, the objective of the study is to investigate the changes in normal and shear stresses in peripheral artery wall during the development of MI-induced HFrEF.
Myocardial infarction was created through the ligation of the left anterior descending artery (LAD) in Wistar rats. Hemodynamic measurement and mechanical test were carried out in carotid arteries (CA) and femoral arteries (FA) in vivo and in vitro, based on which the mathematical analysis was demonstrated to compute normal and shear stresses. The significance and limitation were discussed for understanding the progression of heart failure caused by myocardial infarction.
2 Methods
2.1 Experimental measurements
2.1.1 Animal preparation
Six-week-old Wistar male rats (Beijing Vital River Laboratory Animal Technology Co., Ltd.) were used in the study. As shown in Figure 1A, the experimental protocol has been described in details in a previous study (Wang et al., 2022), which was approved by the Animal Care and Use Committee of Peking University, China. Here, 15 rats underwent ligation of the left anterior descending (LAD) artery of the heart as the MI group and 11 rats as the sham group. Briefly, A 7-0 suture line was placed at ∼1 mm below the left auricle appendage to close the LAD artery, which led to pale LV anterior wall and apex region. Alternatively, the suture was placed but removed in sham-operated animals. After the chest was closed, all animals were housed at standard SPF laboratory for 3 or 6 weeks after operation and free access to standard rodent chow and water. The sham and MI groups has the survival rate of 91% (n = 10) and 67% (n = 10), respectively, which were included in the following experimental measurements. The animals of MI were divided into two groups of MI-3 (n = 5) and MI-6 (n = 5) according to postoperative times (i.e., 3, 6 weeks) after the LAD ligation. Accordingly, the shams were divided into two groups of SHAM-3 (n = 5) and SHAM-6 (n = 5). All experiments were performed in accordance with the Chinese National and Peking University ethical guidelines regarding the use of animals in research, consistent with the NIH guidelines (Guide for the care and use of laboratory animals) on the protection of animals used for scientific purposes.
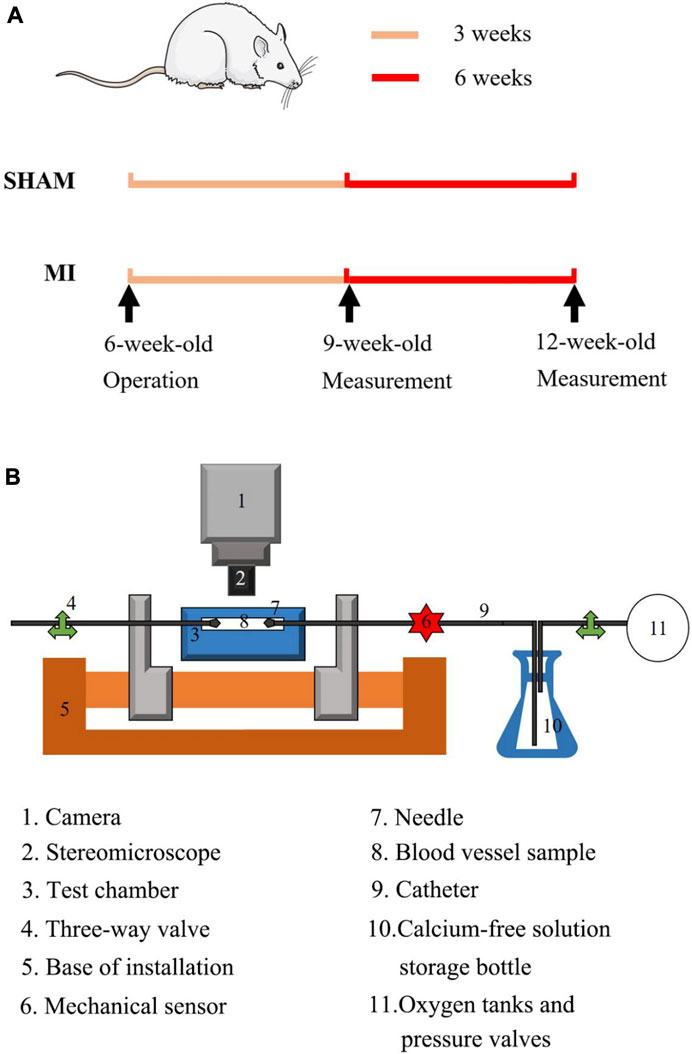
FIGURE 1. (A) Schematic representative of experimental protocol: echocardiographic and hemodynamic measurements were demonstrated at postoperative 3 and 6 weeks; (B) Overview of the utilized extension-inflation setup with inserted artery. 1. Camera; 2. Stereomicroscope; 3. Test chamber; 4. Three-way valve; 5. Base of installation; 6. Mechanical sensor; 7. Needle; 8. Blood vessel sample; 9. Catheter; 10. Calcium-free solution; 11. Oxygen tanks and pressure valves.
2.1.2 Hemodynamic measurements
There is a distinct difference in microstructural and biomechanical properties between elastic and muscular arteries (Feng et al., 2021). There are packaged differences of VSMCs and elastic lamellae between elastic and muscular arteries. The media layer of elastic artery incudes many concentric elastic laminae between internal and external elastic laminae while the muscular artery only has an internal lamina. Moreover, elastic and muscular arteries have different ratios of constitutions, e.g., elastic and collagen fibers and VSMCs. Similar to previous studies (Bing et al., 2020; Li et al., 2021; Wang et al., 2022), the right elastic CA and right muscular FA were dissected in anesthetized animals before termination at postoperative 3 and 6 weeks. Perivascular flow probes (Transonic Systems Inc.; relative error of ± 2%) were mounted on the two arteries to measure flow waves. A 1.4F micromanometer-tipped catheter (Millar Instruments) was calibrated for zero pressure in 37°C saline and inserted into the two arteries to record pressure waves over 10 cardiac cycles. All data were monitored with a BIOPAC MP150.
2.1.3 Mechanical tests
We carried out mechanical tests in the left CA and FA, similar to previous studies (Huo et al., 2012; Huo et al., 2013; Feng et al., 2021). Here, we chose the axial stretch ratio of 1.4 and 1.2 for the passive test using the myograph in Figure 1B At the axial stretch ratio of 1.4, the intravascular pressure increased to 15 mmHg gradually and the vessel was equilibrated for 45 min in the HEPES PSS aerated with 95% O2-5% CO2 in room temperature. Subsequently, PSS in the arteries and baths was replaced with the 37°C Ca2+-free Krebs solution. After equilibrating the vessels for 15 min to have full vasodilation, the transmural pressure increased from 20 to 180 mmHg by an increment of 20 mmHg. Vessel images were recorded by a CCD camera (DS-Ri2, Nikon, Resolution: 4,908 pixels
2.2 Mathematical models
2.2.1 Womersley analysis
Similar to a previous study (Bing et al., 2020), the equation for the pulsatile flow velocity profile across the lumen,
where r is the radial coordinate, R is the radius of artery,
The viscosity (μ) and density (ρ) were assumed to be 4.0 cp and 1.06 g/cm3, respectively. Moreover, RRT reflects the residence time of flow particles near the wall and is recommended as a single metric of low oscillating shear stress, which is expressed as follows:
2.2.2 Strain energy function
The 2D passive model (Feng et al., 2021) were selected to characterize mechanical properties of the CA and FA, which can be written as:
where
2.3 Data analysis
The mean ± SD (standard deviation) values of different parameters were determined (averaged over all carotid and femoral arteries in the four groups). Two-way ANOVA (SigmaStat 3.5) was used to compare wall mechanics and hemodynamic parameters. If indicated statistically significant effect, groups were compared by the Bonferroni test for a comparison of parameters, where p-value < 0.05 represented the statistically significant difference.
3 Results
Figure 1 shows schematic representative of experimental protocol. Figures 2A, B show the circumferential and axial first PK stresses, respectively, obtained from mechanical tests in the elastic CA of SHAM-3, SHAM-6, MI-3, and MI-6 groups. Accordingly, Figure 2B shows the first PK stresses in the muscular FA of the four groups. The first PK stress in the circumferential direction is higher than that in the axial direction (
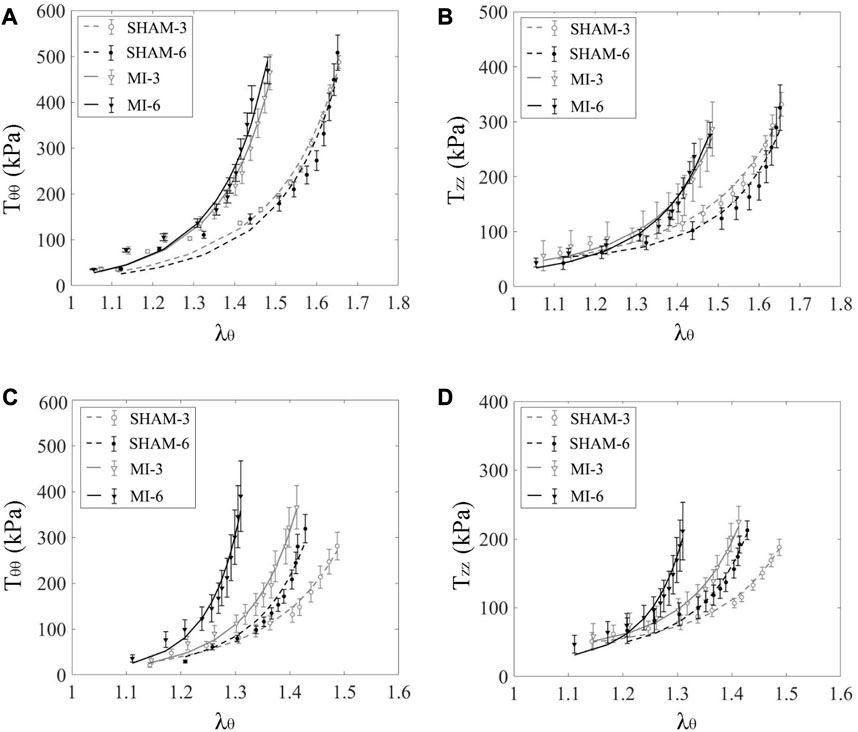
FIGURE 2. Circumferential (A) and axial (B) first PK stresses (
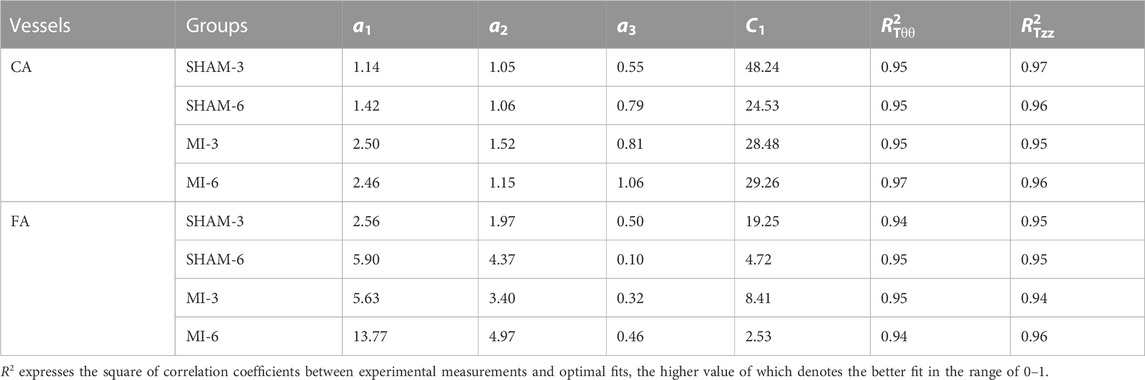
TABLE 1. Material constants by the optimal fit of all measured data in the carotid artery and femoral artery of SHAM-3, SHAM-6, MI-3 and MI-6 groups.
Figure 3 shows flow velocity profiles in CA and FA of SHAM-3, SHAM-6, MI-3, and MI-6 groups at time instances of trough and peak flows. Figure 4 shows transient WSS waves (unit: dynes/cm2) in CA and FA of the 4 groups. The transient WSS waves significantly decreases in the MI-3 group and has the lowest time-averaged value and amplitude in the MI-6 group. The trough flow of CA in MI-6 group shows the strong reversal flow near the wall. Accordingly, Table 2 lists mean ± SD values of TAWSS, OSI, and RRT, which show no statistical difference between SHAM-3 and SHAM-6 groups. Myocardial infarction causes low TAWSS and high OSI and RRT. The MI-6 group has the lowest TAWSS and the highest OSI and RRT than other groups.
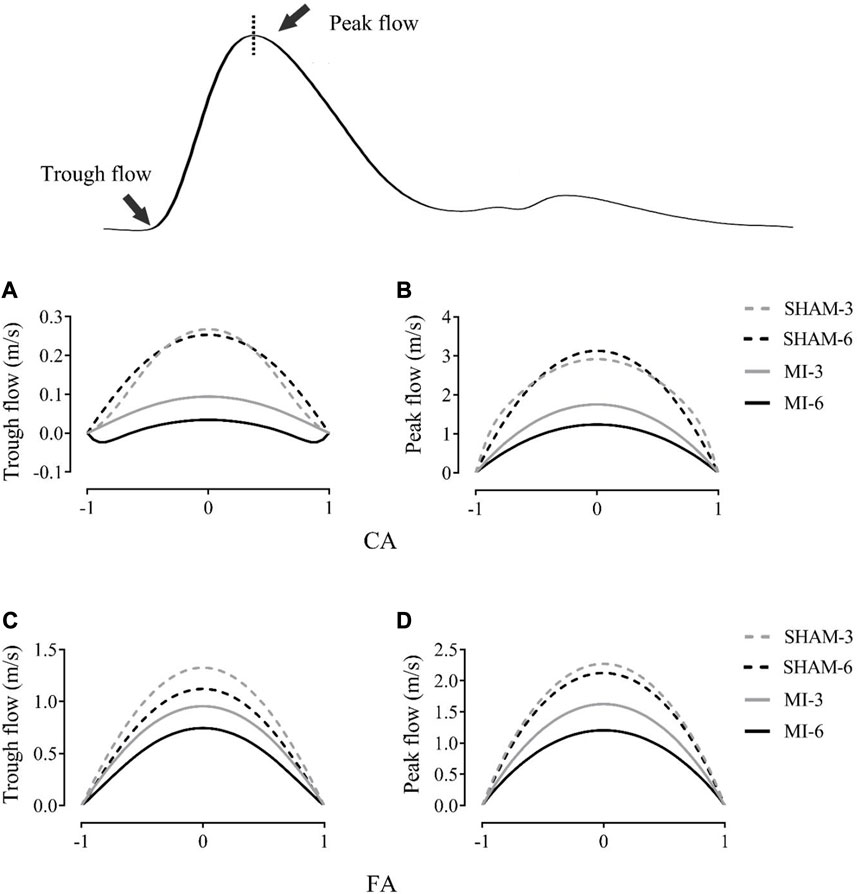
FIGURE 3. Flow velocity profiles (m/s) in the carotid artery at time instances of trough (A) and peak (B) flows. (C, D) show values in femoral arteries corresponding to (A, B).
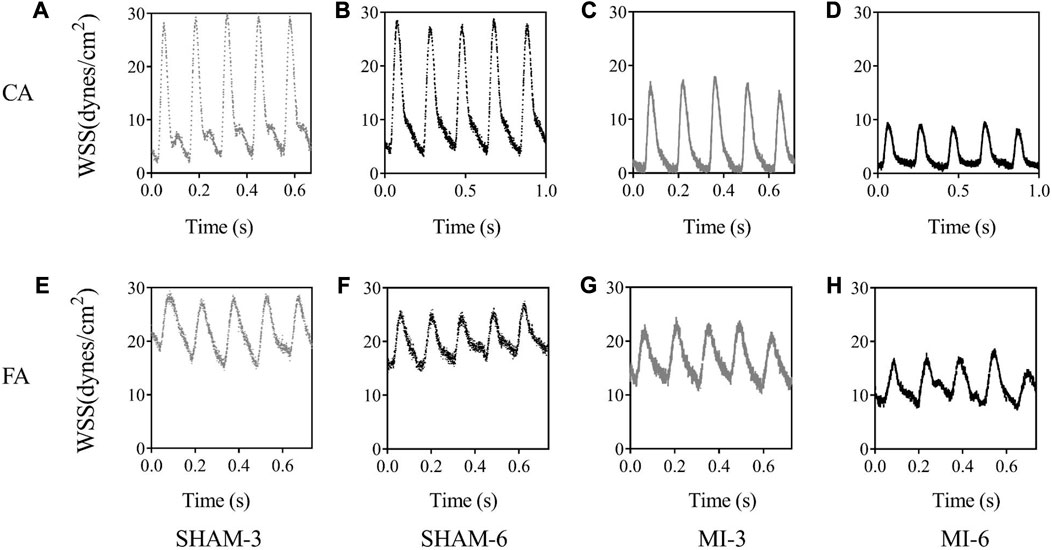
FIGURE 4. Transient WSS waves (unit: dynes/cm2) in the carotid artery of SHAM-3 (A), SHAM-6 (B), MI-3 (C) and MI-6 (D). (E–H) show values in femoral arteries corresponding to (A–D).
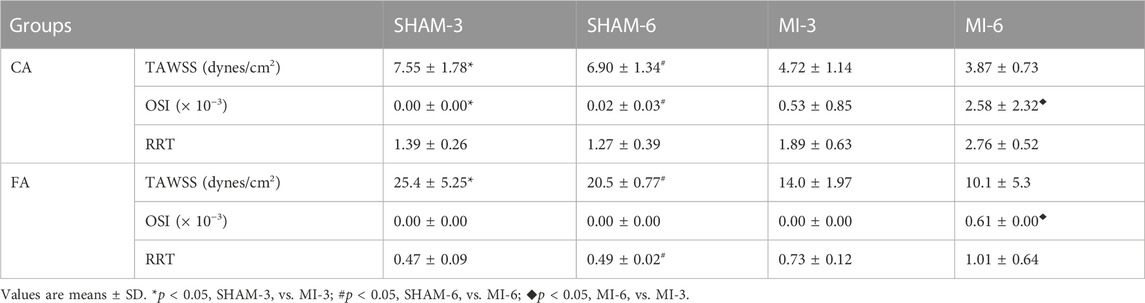
TABLE 2. TAWSS, OSI, and RRT in the carotid artery and femoral artery of SHAM-3, SHAM-6, MI-3 and MI-6 groups.
4 Discussion
This study determined the first PK stresses (i.e.,
Myocardial infarction impaired the LV systolic function (Thygesen et al., 2007; Lu et al., 2020; Jenca et al., 2021), which induced a gradual decrease of cardiac output (CO) and mean aortic pressure (MAP) with time (Wang et al., 2022). The reduced CO and MAP deteriorated the systemic circulation, e.g., an increase of total peripheral resistance and a decrease of total compliance. Here, we demonstrated the analysis of wall mechanics and hemodynamics in peripheral arteries. In both elastic CA and muscular FA, MI resulted in a significant increase of wall stiffness, consistent with previous studies (Hirai et al., 1989; Wang et al., 2022). There was no statistical difference of wall stiffness in the CA between SHAM-3 and SHAM-6 groups and between MI-3 and MI-6 groups. There was, however, a significant increase of wall stiffness in the FA of sham or MI groups from 3 to 6 weeks postoperatively. We have shown the relatively unchanged wall stiffness in healthy carotid arteries between juvenile and adult rats, but an increase of femoral arterial stiffness in the adult rats (Feng et al., 2021), consistent with the present observations in the sham groups. On the other hand, we showed an increase of collagen type III and I in both CA and FA of MI rats from 3 to 6 weeks postoperatively (Wang et al., 2022). It is known that a distinct difference in microstructural and biomechanical properties occurred between muscular and elastic arteries (Wagner and Humphrey, 2011; Feng et al., 2021; Murtada et al., 2021; Wang et al., 2022). The carotid artery had internal and external elastic laminae plus many concentric elastic laminae within the media (Feng et al., 2021), which cut down the effect of the increased collagen. Hence, there was the relatively unchanged carotid arterial stiffness between MI-6 and MI-3 groups. On the other hand, the increased collagen within the media of the MI-6 group induced a significant increase of wall stiffness than the MI-3 group because the femoral artery had a prominent internal elastic lamina only.
The abnormal hemodynamic parameters, i.e., low WSS and high OSI and RRT, were known to impair the endothelial cells of blood vessels (Chen et al., 2016; Huang et al., 2016; Fan et al., 2019). The Womersley analysis showed that TAWSS in CA and FA of the MI groups was >35% lower and RRT was >35% higher than the sham groups. There was a significant increase of OSI in the MI groups from 3 to 6 weeks postoperatively despite negligible OSI in the sham groups. Myocardial infarction gradually worsened the hemodynamic environment in both CA and FA, such as the reduction of WSS, the increase of OSI, and the prolongation of RRT. On the other hand, the Womersley analysis showed the velocity profiles in CA and FA at time instances of trough and peak flows. Myocardial infarction changed parabolic profiles to blunt profiles as well as induced reversal flows near the vessel wall in the MI-6 group. The MI-induced abnormal changes can result in the endothelial dysfunction, monocyte deposition, microemboli formation, SMC proliferation in peripheral arteries (Davies, 2009; Chiu and Chien, 2011; Green et al., 2017; Han et al., 2018), which requires further investigations.
The interplay of cardiac dysfunctions and total impairments in systemic circulation was known to accelerate the progression of MI-induced HF (Wang et al., 2022). Here, the analysis of vessel wall mechanics and hemodynamics demonstrates that the increased normal stress and the decreased shear stress as well as other abnormal parameters impaired vessel wall, which contributed to the development of MI-induced HF. Hence, the detrimental changes in peripheral arteries should be considered to study the MI-induced HF.
5 Critiques of the study
This study only carried out passive mechanical tests in both CA and FA during the development of MI-induced HF. The active mechanical tests should be performed for understanding the interplay of MI and peripheral vessel wall mechanics. A three-dimensional computational fluid dynamic model, coupled with morphometric data of peripheral arteries, should be used to investigate abnormal hemodynamic parameters in details. The relevant molecular and cellular mechanisms should be demonstrated in the following studies.
6 Conclusion
The analysis of wall mechanics showed an increase of MI-induced wall stiffness, which had different changes with time between elastic CA and muscular FA. The hemodynamic analysis demonstrated the gradually worsened WSS, OSI and RRT in peripheral arteries during the progression of MI-induced HF. Both normal and shear stresses are of importance for understanding the MI-induced HF.
Data availability statement
The raw data supporting the conclusion of this article will be made available by the authors, without undue reservation.
Ethics statement
The animal study was approved by Peking University ethical guidelines. The study was conducted in accordance with the local legislation and institutional requirements.
Author contributions
QX: Formal Analysis, Methodology, Writing–review and editing. HS: Formal Analysis, Methodology, Writing–review and editing. LL: Methodology, Writing–review and editing. QJ: Methodology, Validation, Writing–review and editing. XW: Investigation, Methodology, Software, Validation, Writing–original draft. YH: Conceptualization, Writing–review and editing, Funding acquisition.
Funding
The author(s) declare financial support was received for the research, authorship, and/or publication of this article. This work is supported by the National Key Research and Development Program of China 2021YFA1000200 and 2021YFA1000203 (YH), the Shenzhen Science and Technology R&D Grant KQTD20180411143400981 (YH).
Acknowledgments
We thank all participants of the study in The Peking University and The Shanghai Jiao Tong University.
Conflict of interest
The authors declare that the research was conducted in the absence of any commercial or financial relationships that could be construed as a potential conflict of interest.
The author(s) declared that they were an editorial board member of Frontiers, at the time of submission. This had no impact on the peer review process and the final decision.
Publisher’s note
All claims expressed in this article are solely those of the authors and do not necessarily represent those of their affiliated organizations, or those of the publisher, the editors and the reviewers. Any product that may be evaluated in this article, or claim that may be made by its manufacturer, is not guaranteed or endorsed by the publisher.
References
Bing, F., Wang, X., Shen, W., Li, L., Niu, P., Chen, Y., et al. (2020). Inhalation of ultrafine zinc particles impaired cardiovascular functions in hypertension-induced heart failure rats with preserved ejection fraction. Front. Bioeng. Biotechnol. 8, 13. doi:10.3389/fbioe.2020.00013
Chen, X., Gao, Y., Lu, B., Jia, X., Zhong, L., Kassab, G. S., et al. (2016). Hemodynamics in coronary arterial tree of serial stenoses. PLoS One 11, e0163715. doi:10.1371/journal.pone.0163715
Chiu, J. J., and Chien, S. (2011). Effects of disturbed flow on vascular endothelium: pathophysiological basis and clinical perspectives. Physiol. Rev. 91, 327–387. doi:10.1152/physrev.00047.2009
Davies, P. F. (2009). Hemodynamic shear stress and the endothelium in cardiovascular pathophysiology. Nat. Clin. Pract. Cardiovasc. Med. 6, 16–26. doi:10.1038/ncpcardio1397
Fan, T., Zhou, Z., Fang, W., Wang, W., Xu, L., and Huo, Y. (2019). Morphometry and hemodynamics of coronary artery aneurysms caused by atherosclerosis. Atherosclerosis 284, 187–193. doi:10.1016/j.atherosclerosis.2019.03.001
Feng, Y. D., Wang, X., Zhao, Y. Y., Li, L., Niu, P., Huang, Y. F., et al. (2021). A comparison of passive and active wall mechanics between elastic and muscular arteries of juvenile and adult rats. J. Biomechanics 126, 110642. doi:10.1016/j.jbiomech.2021.110642
Green, D. J., Hopman, M. T., Padilla, J., Laughlin, M. H., and Thijssen, D. H. (2017). Vascular adaptation to exercise in humans: role of hemodynamic stimuli. Physiol. Rev. 97, 495–528. doi:10.1152/physrev.00014.2016
Han, Y., Huang, K., Yao, Q. P., and Jiang, Z. L. (2018). Mechanobiology in vascular remodeling. Natl. Sci. Rev. 5, 933–946. doi:10.1093/nsr/nwx153
Hirai, T., Sasayama, S., Kawasaki, T., and Yagi, S. (1989). Stiffness of systemic arteries in patients with myocardial infarction. A noninvasive method to predict severity of coronary atherosclerosis. Circulation 80, 78–86. doi:10.1161/01.cir.80.1.78
Huang, X., Yin, X., Xu, Y., Jia, X., Li, J., Niu, P., et al. (2016). Morphometric and hemodynamic analysis of atherosclerotic progression in human carotid artery bifurcations. Am. J. Physiol. Heart Circ. Physiol. 310, H639–H647. doi:10.1152/ajpheart.00464.2015
Humeres, C., and Frangogiannis, N. G. (2019). Fibroblasts in the infarcted, remodeling, and failing heart. JACC. Basic Transl. Sci. 4, 449–467. doi:10.1016/j.jacbts.2019.02.006
Humphrey, J. D., and Canham, P. B. (2000). Structure, mechanical properties, and mechanics of intracranial saccular aneurysms. J. Elast. 61, 49–81. doi:10.1023/a:1010989418250
Humphrey, J. D. (2008). Mechanisms of arterial remodeling in hypertension: coupled roles of wall shear and intramural stress. Hypertension 52, 195–200. doi:10.1161/HYPERTENSIONAHA.107.103440
Huo, Y., Cheng, Y., Zhao, X., Lu, X., and Kassab, G. S. (2012). Biaxial vasoactivity of porcine coronary artery. Am. J. Physiol. Heart Circ. Physiol. 302, H2058–H2063. doi:10.1152/ajpheart.00758.2011
Huo, Y., and Li, L. (2022). Long-term inhalation of ultrafine zinc particles deteriorated cardiac and cardiovascular functions in rats of myocardial infarction. Front. physiology 13, 921764. doi:10.3389/fphys.2022.921764
Huo, Y., Zhao, X., Cheng, Y., Lu, X., and Kassab, G. S. (2013). Two-layer model of coronary artery vasoactivity. J. Appl. Physiol. 114, 1451–1459. doi:10.1152/japplphysiol.01237.2012
Jenca, D., Melenovsky, V., Stehlik, J., Stanek, V., Kettner, J., Kautzner, J., et al. (2021). Heart failure after myocardial infarction: incidence and predictors. Esc. Heart Fail 8, 222–237. doi:10.1002/ehf2.13144
Kassab, G. S. (2006). Biomechanics of the cardiovascular system: the aorta as an illustratory example. J. R. Soc. Interface 3, 719–740. doi:10.1098/rsif.2006.0138
Li, L., Niu, P., Wang, X., Bing, F., Tan, W., and Huo, Y. (2021). Short-term inhalation of ultrafine zinc particles could alleviate cardiac dysfunctions in rats of myocardial infarction. Front. Bioeng. Biotechnol. 9, 646533. doi:10.3389/fbioe.2021.646533
Lu, Q., Liu, P., Huo, J. H., Wang, Y. N., Ma, A. Q., Yuan, Z. Y., et al. (2020). Cardiac rupture complicating acute myocardial infarction: the clinical features from an observational study and animal experiment. BMC Cardiovasc Disord. 20, 409. doi:10.1186/s12872-020-01683-y
Lu, Y., Wu, H., Li, J., Gong, Y., Ma, J., Kassab, G. S., et al. (2017). Passive and active triaxial wall mechanics in a two-layer model of porcine coronary artery. Sci. Rep. 7, 13911. doi:10.1038/s41598-017-14276-1
Miyoshi, T., and Ito, H. (2021). Arterial stiffness in health and disease: the role of cardio-ankle vascular index. J. Cardiol. 78, 493–501. doi:10.1016/j.jjcc.2021.07.011
Murtada, S. I., Kawamura, Y., Weiss, D., and Humphrey, J. D. (2021). Differential biomechanical responses of elastic and muscular arteries to angiotensin II-induced hypertension. J. Biomech. 119, 110297. doi:10.1016/j.jbiomech.2021.110297
Poelzl, G., Frick, M., Huegel, H., Lackner, B., Alber, H. F., Mair, J., et al. (2005). Chronic heart failure is associated with vascular remodeling of the brachial artery. Eur. J. Heart Fail. 7, 43–48. doi:10.1016/j.ejheart.2004.04.008
Thygesen, K., Alpert, J. S., White, H. D., Joint, E. S. C. A. A. H. A. W. H. F. T. F. f. t. R. o. M. I., Jaffe, A. S., Apple, F. S., et al. (2007). Universal definition of myocardial infarction. Circulation 116, 2634–2653. doi:10.1161/CIRCULATIONAHA.107.187397
Wagner, H. P., and Humphrey, J. D. (2011). Differential passive and active biaxial mechanical behaviors of muscular and elastic arteries: basilar versus common carotid. J. Biomech. Eng. 133, 051009. doi:10.1115/1.4003873
Keywords: myocardial infarction, wall mechanics, hemodynamics, Womersley model, peripheral artery
Citation: Xue Q, Shi H, Li L, Jin Q, Wang X and Huo Y (2023) Myocardial infarction impaired wall mechanics and hemodynamics in peripheral arteries. Front. Physiol. 14:1266568. doi: 10.3389/fphys.2023.1266568
Received: 25 July 2023; Accepted: 16 August 2023;
Published: 29 August 2023.
Edited by:
Dalin Tang, Worcester Polytechnic Institute, United StatesReviewed by:
Bao Li, Beijing University of Technology, ChinaYonggang Lv, Wuhan Textile University, China
Copyright © 2023 Xue, Shi, Li, Jin, Wang and Huo. This is an open-access article distributed under the terms of the Creative Commons Attribution License (CC BY). The use, distribution or reproduction in other forums is permitted, provided the original author(s) and the copyright owner(s) are credited and that the original publication in this journal is cited, in accordance with accepted academic practice. No use, distribution or reproduction is permitted which does not comply with these terms.
*Correspondence: Xuan Wang, paige56@163.com; Yunlong Huo, huoyunlong@sjtu.edu.cn
†These authors have contributed equally to this work