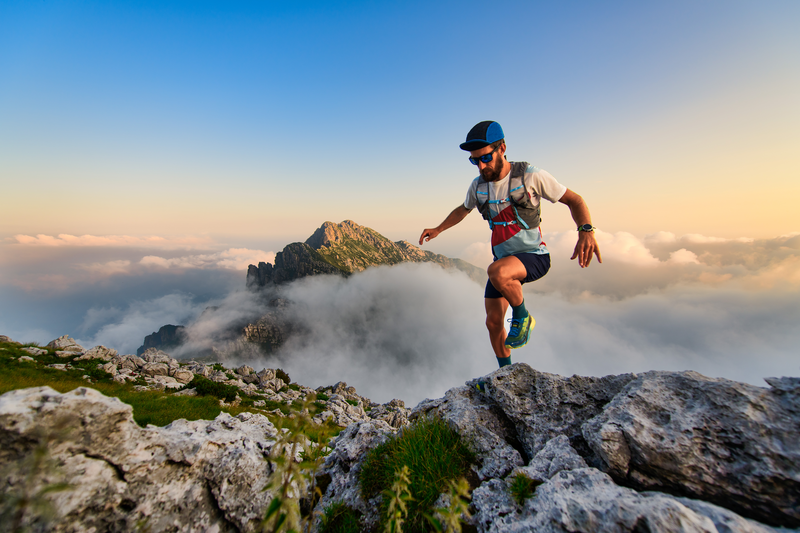
95% of researchers rate our articles as excellent or good
Learn more about the work of our research integrity team to safeguard the quality of each article we publish.
Find out more
REVIEW article
Front. Physiol. , 28 November 2023
Sec. Gastrointestinal Sciences
Volume 14 - 2023 | https://doi.org/10.3389/fphys.2023.1264570
Aquaporin-3 (AQP3) is a predominant water channel protein expressed in the intestine, and plays important roles in the gut physiology and pathophysiology due to its permeability to water, glycerol and hydrogen peroxide. In this review, we systematically summarized the current understanding of the expression of AQP3 in the intestine of different species, and focused on the potential roles of AQP3 in water transport, different types of diarrhea and constipation, intestinal inflammation, intestinal barrier function, oxidative stress, and autophagy. These updated findings have supported that AQP3 may function as an important target in maintaining gut health of human and animals.
Aquaporins (AQPs) belong to a highly conserved group of major intrinsic proteins present in the cell membrane. Nowadays, at least 13 AQPs (AQP0 through AQP12) have been identified in the mammalians, which can be subdivided into three groups including classical aquaporins (AQP0, AQP1, AQP2, AQP4, AQP5, AQP6, and AQP8), aquaglyceroporins (AQP3, AQP7, AQP9, and AQP10) and unorthodox aquaporins (AQP11 and AQP12). Within the digestive tract, several AQPs including AQP1, AQP3, AQP4, AQP5, AQP6, AQP7, AQP8, AQP9, AQP10, and AQP11 have been demonstrated to be expressed abundantly in the stomach and intestines (Laforenza et al., 2005; Zhu et al., 2016; Cao et al., 2018; Zhu et al., 2023). Of these, AQP3 represents a predominant isoform distributed in the gastrointestinal tract which could selectively transport water, glycerol, and hydrogen peroxide (H2O2) (Yde et al., 2016). In recent decade, AQP3 has received tremendous attention due to its important roles in maintaining water transport, cell volume regulation, intestinal permeability, fluid secretion and absorption homeostasis (Zhu et al., 2016; Ikarashi et al., 2019a). Increasing data has indicated that the expression or alterations of AQP3 in the gut may be associated with several intestinal disorders, such as inflammatory bowel disease, diarrhea, intestinal barrier injury, irritable bowel syndrome, intestinal oxidative stress, and autophagy (Meli et al., 2018). Notably, previous study has indicated that AQPs including AQP3 represent important targets for dietary nutrients to regulate intestinal health (Lv et al., 2022). Thus, this review mainly summarized the updated progress concerning the role and regulation of AQP3 in the gut of human and animals, which are important for enhancing our understanding on this research field.
Nowadays, AQP3 has been demonstrated to be expressed in the intestines of many mammalian species (Table 1), including human (Hamabata et al., 2002), rats (Ramírez-Lorca et al., 1999; Zhao et al., 2016), mice (Zhang et al., 2017), pigs (Zhu et al., 2017; He et al., 2017), and desert hare (Zhang et al., 2019). For example, the AQP3 mRNA was detected in the distal ileum and colon while the localization of AQP3 protein was confirmed at the intestinal mucosal epithelium of patients with inflammatory bowel diseases by immunofluorescence confocal microscopy (Ricanek al., 2015). Previous study via human tissue microarrays also indicated that AQP3 was selectively targeted to the basolateral membrane in human intestine (Cohly et al., 2008), particularly with strong expression in the basolateral membranes of distal colon (Mobasheri et al., 2005). However, another study showed that AQP3 protein was restricted to the villus epithelial cells of human colon, with more intense in the apical than in the basolateral membranes (Silberstein et al., 1999). In vitro study also confirmed the presences of AQP3 mRNA and protein (Itoh et al., 2003) as well as the immunocytochemical localization of AQP3 in HT-29 human colon epithelial cell line (Li et al., 2015).
In rats, the AQP3 mRNA was found in the middle portion of small intestine, ileum, proximal colon and distal colon segments, while AQP3 protein was detected abundantly in the basolateral plasma membrane of colon surface columnar epithelial cells (Koyama et al., 1999; Ramírez-Lorca et al., 1999; Yde et al., 2016; Zhao et al., 2016). Another study has also indicated that the expression of AQP3, AQP4, and AQP8 in superficial colonocytes was higher than the lower crypt cells of rat proximal colon (Laforenza et al., 2005). In fact, the immunofluorescence analysis clearly showed that AQP3 expressed at both the apical and basal sides of mucosal epithelial cells in rat colon (Ikarashi et al., 2016), confirming that AQP3 was the dominant AQP subtype in the luminal side of rat colon mucosa compared to AQP4 and AQP8 (Ikarashi et al., 2011a). Moreover, the AQP3 mRNA expression was downregulated in jejunum tissue samples of rats treated by heat stroke, while AQP3 localization was present from the lamina propria to the crypt following heat stroke (Wang et al., 2015). However, the expression of AQP3 in the rat residual ileum and colon was upregulated during the adaptation after an 80% small bowel resection on postoperative day 1 and 7 (Tsujikawa et al., 2003).
In mice, AQP3 was mainly localized to the jejunum villi epithelial cells of mice rather than the duodenum or ileum (Zhang et al., 2017). Moreover, AQP3 has also been found to be localized at the apical side of mice colonic epithelial cells (Wang et al., 2017). Notably, the AQP3 localization shifted from its normal location along cell membranes to the cell cytoplasm during bacterial infection in C57BL/6 mice (Guttman et al., 2007).
In pig, the AQP3 was widely distributed in different sections of small intestine of piglets, with more abundant expression in the duodenum than jejunum and ileum (He et al., 2017). However, another study showed that AQP3, AQP7, and AQP10 expression was greatest in the jejunum of growing pigs (Krone et al., 2019). We previously also found that AQP3 was expressed in the duodenum, jejunum, and ileum of weaned piglets (Zhu et al., 2017).
Interestingly, AQP3 was found to be located in the small intestinal villus epithelium and colonic epithelium of a desert hare, Lepus yarkandensis (Zhang et al., 2019). To date, though limited research was conducted on poultry regarding the AQPs distribution and expression, the transcripts of AQP1, AQP3, and AQP9 were evident in the jejunum of broilers (Ross 308) (Alhotan et al., 2021).
The gastrointestinal epithelium plays a major role in the control of fluid and ion balance (Turvill and Farthing, 1999; Laforenza, 2012). For the water transport and fluid absorption in gut epithelium, it often involves both the paracellular route and the transcellular route (Laforenza, 2012). The paracellular route is mainly mediated by the space between cell tight junctions and is quite limited in small intestine with leaky epithelium (Masyuk et al., 2002). Increasing evidence has indicated that there are several potential mechanisms to mediate the transcellular route of water transport in gut epithelium, which include 1) the passive diffusion through the phospholipid bilayer (Benga, 2009), 2) the cotransporter with ions and nutrients (Wright and Loo, 2000; Zeuthen, 2010), and 3) the osmotically-driven transmembrane water movements through AQPs (Ma and Verkman, 1999; Benga, 2009; Mollajew et al., 2010; Laforenza, 2012; Ye et al., 2023). Thus, the expression of AQP3 in gastrointestinal tract of different species may suggest an involvement in water transport through a transcellular route. Numerous studies have suggested the important role of AQP3 in water transport and fluid absorption of gut, and the alterations of AQP3 expression in the gut may be associated with intestinal orders such as diarrhea or constipation (Camilleri et al., 2019; Yde et al., 2021). For example, the inhibition of AQP3 function in the colon using HgCl2 or CuSO4 would contribute to increased fecal water contents and develop severe diarrhea in rats (Ikarashi et al., 2012a). Similar results have showed that mercury exposure would induce significant reductions of protein expression of AQP3 in the stomach, jejunum and colon of rats (Bottino et al., 2016). Another study also reported that both AQP3 mRNA and protein expression was decreased in patients with or without bile acid malaabsorption (Camilleri et al., 2019). Based on these findings, the altered expression of AQP3 in the intestine might cause disorder of water transfer and fluid metabolism, which indicated the potential role of AQP3 in influencing the fluid homeostasis and gut health (Koyama et al., 1999; Zhu et al., 2017). However, the precise assessment of the functional role of AQP3 under physiological and pathophysiological conditions still requires to be fully defined in future studies (Yde et al., 2016).
Irritable bowel syndrome (IBS) is a chronic functional gastrointestinal disorder that affects 9%–23% of the population across the world (Saha, 2014; Radziszewska et al., 2023). Abdominal pain associated with defecation disorder and fluid balance disturbance, was the predominant bowel dysfunction in patients with IBS-D (Camilleri, 2015). Previous study has showed that the AQP3 expression at both mRNA and protein levels was decreased in rectosigmoid mucosal biopsies of patients with IBS-D (Camilleri et al., 2019). Further study showed that the levels of long noncoding RNA H19, AQP1, and AQP3 were all reduced in the colonic mucosa of IBS-D patients, and the reduced AQP1 and AQP3 protein expression in Caco-2 cells was positively correlated with the level of long noncoding RNA H19 (Chao et al., 2020). However, previous study in rats showed that atractylodes oils could alleviate the predominant IBS-D and reduce intestinal inflammation by partially increasing the expression of AQP3 in the colon (Xie et al., 2021).
ETEC is well-recognized as a major cause of diarrhea in neonates, children and travelers in lower-income countries (Zhang et al., 2022), and ETEC-induced diarrhea also represents an important problem in swine production especially during the weaning period (Kim et al., 2022). Increasing data has indicated the important role of AQP3 in ETEC-induced diarrhea. For example, we previously demonstrated that ETEC K88 induced significant changes of ion transporter and water channel proteins, with AQP3 expression in the intestine of weaned piglets significantly downregulated by ETEC challenge (Zhu et al., 2017). Similarly, Zhang et al. (2017) also found that the AQP3 expression was gradually decreased after ETEC administration for 1, 3, 5, or 7 days compared to the unchanged control group. Moreover, the protein expression of AQP3 in colonic mucosa of piglets was significantly decreased when challenged with either recombinant Escherichia coli expressing heat-stable enterotoxin or ETEC K88 (Lv et al., 2018). Besides, both AQP2 and AQP3 localizations would be altered from cell membranes to cell cytoplasm after bacterial infection depending on the bacterial type III effector proteins EspF and EspG (Guttman et al., 2007). This was true since the altered localizations of AQPs may represent an important contributing factor to diarrhea during bacterial infection (Guttman et al., 2007). Increasing data has suggested that AQP3 has an important role in the reduction of ETEC-induced diarrhea for potential dietary interventions. For example, previous study has showed that Lactobacillus plantarum strains FCQNA30M6 and CCFM1143 alleviated ETEC-induced diarrhea and increased the serum concentration of AQP3 in mice (Yue et al., 2020). Another study also found that immunohistochemistry scores of AQP3 in colon cancer patients were positively correlated with serum AQP3 concentrations (Hong et al., 2020). A recent study has also shown that dietary berberine supplementation significantly upregulated the mRNA expression of aquaporins (AQP1, AQP3, AQP4, AQP7, and AQP10) and Na+/H+ exchanger 3 in ETEC K88-challenged piglets (Zhu et al., 2023). However, other ETEC strains (SEC470, SEC 298; SEC 817 or C197) had little effect on the protein abundance of AQP3 in the jejunum of mice at 24 h post ETEC infection (Wu and Su, 2018).
Zinc oxide (ZnO) has been well demonstrated to alleviate the postweaning diarrhea of piglets. Peng et al. (2020) found that dietary supplementation with either pharmacologic dose (3,000 mg/kg) of conventional ZnO or porous ZnO at 750 or 1,500 mg/kg could reduce the mRNA expression of AQP3 in the jejunal mucosa, and alleviate the incidence of diarrhea of weaned piglets. In contrast to ZnO, dietary supplementation with an amino acid blend (glutamate: glutamine: glycine: arginine: N-acetylcysteine = 5: 2: 2: 1: 0.5) could significantly upregulate the mRNA expression of AQP3, AQP8, and AQP10 as well as the protein expression of AQP3 and AQP4 in the jejunum, and reduce the incidence of diarrhea with an enhanced intestinal function and growth performance in weaned piglets (Yi et al., 2018). The discrepancy of these results may be related to the differences in experimental design, sampling time, management, and the potential interactions of AQP3 with other AQPs. Thus, further investigations concerning the possible mechanism of different nutritional interventions on diarrhea through AQP3 regulation in weaned piglets.
In summary, most of these findings indicated that the inhibition or reduced AQP3 expression was closely associated with regulation of water transport and absorption and fluid homeostasis in the gastrointestinal tract. AQP3 may represent an important target for the nutritional regulation of neonates and piglets during the critical weaning period when they are vulnerable to ETEC infection.
Porcine epidemic diarrhea virus (PEDV) causes a highly contagious intestinal disease in neonatal and weaned piglets with the symptoms of acute diarrhea, vomiting, and severe dehydration porcine epidemic diarrhea (Wang et al., 2022). It has been demonstrated that AQP3 expression was significantly decreased in the small intestine of PEDV-infected piglets as well as in Sp1-inhibited porcine intestinal epithelial cells (IPEC-J2), and showed a negative correlation with the increased methylation levels of mC-20 site of CpG1 and mC-10 site of CpG2 in the AQP3 promoter region (Wu et al., 2020). In line with this, downregulation of AQP3 by shRNA silencing in IPEC-J2 cells increased the genome copies and viral titers of PEDV, which proved that AQP3 could inhibit the PEDV infection in IPEC-J2 cells (Wang et al., 2022). Moreover, in a mouse model of rotavirus diarrhea, the AQP3 expression in the colon was significantly upregulated, while AQP1, AQP4, while AQP8 expression was downregulated by rotavirus challenge (Cao et al., 2014).
In traditional Chinese medicine, the spleen-deficiency diarrhea is regarded as a prevalent gastrointestinal condition with diarrhea as the primary symptom (Fan et al., 2023). The AQP3, AQP4, and AQP8 expression of colon was significantly decreased in rats with spleen-deficiency diarrhea, while their expression was significantly increased by administration with Pingwei San or Shenling Baizhu San (Fan et al., 2023). Similarly, treatment with a high dose of Atractylodis Rhizoma extract for consistent 10 days help prevent the spleen deficiency-induced diarrhea and reduce the pathological changes in colon tissue of mice, which might be associated with the enhanced expressions of both AQP3 and AQP8 in the colon (Shi et al., 2019). Moreover, as reported in a recent study, the treatment with ethanol extract of deep-fried Atractylodes lancea Rhizome significantly improved the symptoms of mice with spleen-deficiency diarrhea by partially promoting the expression of AQP3, AQP4, and AQP8 and tight junction markers (Liu et al., 2023). Another study showed that oral administration of both low dose and high dose of Ershen pill extract could prevented body weight loss and inhibited diarrhea after 2-week treatment (Pan et al., 2019). The anti-diarrhea mechanism of Ershen pill extract might be associated with the regulation of AQP3, since high dose of Ershen pill extract significantly improved the AQP3 positive staining intensity as well as the AQP3 protein synthesis in the colon tissue of rats with spleen deficiency-induced diarrhea (Pan et al., 2019).
Antibiotic-related diarrhea is known as a common side-effect during antibiotic treatment inducing dysbacteriosis of gut microbiota, with Clostridium difficile infection being the most common causative agent of the disease (Li et al., 2023). The incidence of antibiotic-related diarrhea remains relatively high especially in hospitalized children and older adults (Kaya et al., 2023; Zhao et al., 2023). The effectiveness of probiotic strains in the prevention and treatment of antibiotic-associated diarrhea has been well-summarized in recent systematic literature reviews (Doar and Samuthiram, 2023; Yang et al., 2023). However, few studies could be found concerning probiotic regulation on antibiotic-associated diarrhea by targeting AQPs. There was such one study showing that Bacteroides fragilis strain ZY-312 could alleviate the antibiotic-associated diarrhea in rats by enhancing the colonic AQP3 and AQP8 expression and modulating intestinal defenses (Zhang et al., 2018).
Bile acid-induced diarrhea is usually caused by an excess of bile acids in the colon due to bile acid malabsorption (Yde et al., 2016), which is very common in patients with inflammatory diseases, such as ileal resection, Crohn’s disease, and diarrhea-predominant irritable bowel syndrome (Duan et al., 2019). An earlier study indicated that the mechanism of bile acid-induced diarrhea might be associated with an increase in mucosal permeability to ion and water and mucosal damage (Argenzio and Whipp, 1983). These authors found that the ion transport and net water absorption in the pig colon were abolished by deoxycholic acid, accompanied with an increased mucosal permeability (Argenzio and Whipp, 1983). Previous study in rats fed with cholic acid also revealed that a reduced AQP3 protein levels in colonic epithelia and increased fecal water contents, indicating its involvement in the pathophysiology of bile acid-induced diarrhea (Yde et al., 2016).
Irinotecan is a broad-spectrum cytotoxic anticancer agent with a series of toxic side-effects (Yue et al., 2021). A recent systematic review and meta-analysis study has demonstrated that Chinese herbal medicine represented an effective complementary and alternative prevention and therapy for irinotecan induced diarrhea (Lin et al., 2023). Indeed, the herbal medicines and their derived phytocompounds might be effective complementary treatments for irinotecan induced diarrhea compared to the generally recommended treatments with high-dose loperamide and octreotide of controversial effects (Tang et al., 2014). In contrast, another study suggested a lack of benefit of administered probiotic formula containing Bifidobacterium and Lactobacillus rhamnosus for the prevention of irinotecan-induced diarrhea in colorectal cancer patients (Mego et al., 2023). Interestingly, the incidence of severe irinotecan-induced diarrhea in patients with advanced colorectal cancer has been significantly reduced by the application of activated charcoal (Michael et al., 2004). By inducing severe diarrhea, irinotecan has been demonstrated to cause a significant decrease of AQP3 expression in the colon of rats, while anti-inflammatory drugs celecoxib could prevent irinotecan-induced delayed diarrhea by suppressing the reduction of AQP3 expression (Kon et al., 2018a).
Castor oil is mainly used to treat elderly or patients with long-term constipation due to its laxative property (Arslan and Eşer, 2011). However, excess intake of castor oil might induce diarrhea characterized by disordered water and ion balance. Previous study has found that the fruit extracts of Capsicum annum L. abundant in Pakistan could significantly inhibit the number of defecations in castor oil-induced diarrhea by increasing the urinary excretion of water and electrolytes (Mazhar et al., 2022). Similarly, Camelina sativa oil treatment could relieve the castor oil-induced diarrhea by modulating gut microbiota composition and the production of short-chain fatty acids and reducing serum proinflammatory indices in mice (Zhu et al., 2022). Nevertheless, other investigators showed that castor oil increased the mRNA expression of AQP3, but the anti-diarrhea effect of Chinese herb medicine FengLiao was associated with a decreased AQP3 expression in the jejunum of mice with castor oil-induced diarrhea (Chen et al., 2020).
Senna and its main component sennosides are recognized as effective laxative drugs for treating intestinal constipation (Cao et al., 2018). However, clinical side-effects of diarrhea and toxicity on the kidneys and livers should be cautioned (Cao et al., 2018). These authors demonstrated that high doses of both senna extract and sennosides treatments resulted in a significant diarrhea grade, with colonic AQP3 mRNA expression upregulated in all diarrhea groups (Cao et al., 2018). In a mice model of senna-induced diarrhea, the transdermal administration of Renzhu ointment has been shown to significantly reduce the frequency of loose stools, diarrhea rate and index, and fecal moisture content (Zhong et al., 2023). Moreover, the BALB/c mice developed acute diarrhea via senna extract solution in accompany with a decreased expression of AQP3 in the jejunum, while interventions of Malus pumila leaf flavonoids at either 25 mg/kg or 50 mg/kg by gavage displayed antidiarrheal effects by increasing AQP3 expression (Yi et al., 2020).
cAMP is known as the second messenger in the body. Accumulating evidence indicated that AQP3 expression could be regulated by cAMP pathway mainly through protein kinase A (PKA) and its downstream effectors such as cAMP-responsive element binding protein (CREB). This might be due to the fact that the 5′-flanking region of AQP3 gene harbors promoter activity and two binding sites of the transcription factor CERB (Yde et al., 2016). Previous study has found that cholera toxin treatment negatively regulated osmotic water permeability of AQP3 and AQP4 by increasing intracellular cAMP concentration (Hamabata et al., 2002). Moreover, patchouli oil could significantly decrease the expression of AQP3, AQP7, and AQP11 in the small intestine of rats via the vasoactive intestinal peptide (VIP)-cAMP-PKA signaling pathway, thereby restoring the intestinal water absorption for alleviating 5-fluorouracil-induced diarrhea (Gan et al., 2020). Notably, the antidiarrhoeal effect of Rhubarb tannins extract might be associated with its reduction in the expression of AQP2 and AQP3 in apical and lateral mucosal epithelial cells in the colon of diarrhea mice as well as in HT-29 cells induced by MgSO4 via downregulating PKA/CREB signal pathway (Liu et al., 2014). Another study also found that β-patchoulene ameliorated water transport and alleviated diarrhea by inhibiting AQP3 function via the cAMP/PKA/CREB signaling pathway in intestinal mucositis-induced rats (Wu et al., 2021). These results indicated that AQP3 may act as a new therapeutic target (Figure 1, created with BioRender.com) in diarrhea (Wu et al., 2021), and the activation of cAMP/PKA/p-CREB pathway was involved in regulating AQP3 expression in colonic epithelial cells.
Constipation is characterized as a functional bowel disorder with a difficult, irregular, or deficient defecation (Longstreth et al., 2006). Normally, the colon would absorb approximately 1.5–2.0 L of water daily via both transcellular and paracellular routes (Laforenza, 2012). Increasing data has indicated that AQP3 in the colon was closely related to fecal characteristics and was involved in the onset of constipation probably by promoting water absorption from the luminal side to the vascular side (Table 2). For example, AQP3 mRNA expression in the colon was upregulated with increasing serotonin (namely, 5-hydroxytrypamine, 5-HT) secretion in constipation-rats induced by morphine (Kon et al., 2015). Indeed, both AQP3 and AQP8 were upregulated in the colonic biopsies of patients with functional constipation (Lin et al., 2023). Moreover, the combination of HgCl2 and morphine could improve the morphine-induced constipation in mice (Kon et al., 2015). In a mouse model of constipation established by diphenoxylate, the expression of AQP3 in colonic mucosa was also found to be significantly upregulated (Sun et al., 2018). Similar pattern of increased AQP3 expression was found while using diphenoxylate hydrochloride to induce constipation of rats (Cong et al., 2019). Thus, most of these findings supported the notion that increased AQP3 might be an important characteristic of most functional constipations (Yuan et al., 2008). Notably, the use of diphenoxylate at much higher dosage for a long time would result in an opposite result, with colonic AQP3 expression downregulated in these constipation models (Zhan et al., 2020; Tan et al., 2021). Thus, these studies suggest that AQP3 functions as a new promising target for the development of laxatives (Ikarashi et al., 2012a), and may act as a new therapeutic target in alleviating the constipation (Ikarashi et al., 2016) (as summarized in Table 3).
Magnesium sulfate (MgSO4) represents as an important osmotic laxative, could increase the protein expression of AQP3 in the colon of rats (Ikarashi et al., 2011a; Zhu et al., 2019). Whereas the concomitant use of MgSO4 and bisacodyl induced a lower content of fecal water content than in the MgSO4 group, but increased the expression of AQP3 in the colon without enhancing the laxative effect (Ikarashi et al., 2012b).
Lactulose is another commonly used osmotic laxative in treating constipation. Previous study has shown that lactulose treatment at 3 g/kg for 4 weeks effectively alleviated the constipation damage in the colon of mice by upregulating the AQP3 expression through regulating the cAMP-PKA pathway (Tan et al., 2021). Similar results of AQP3 changes have been found when administrating lactulose (500 mg/kg) daily for 14 days in loperamide-induced functional constipation mice (Wang et al., 2023).
Fructose has also been shown to resolve symptoms in patients with chronic functional constipation (Mehta and Beg, 2018). This might be because the increased ingestion of fructose would increase fructose malabsorption with symptoms of insufficient absorption and subsequent bacterial fermentation of fructose, which resulted in a state of fructose intolerance to increase the intestinal motility for inducing looser stools (Mehta and Beg, 2018). Indeed, another study confirmed that the fructose intolerance and malabsorption was quite common in functional gastrointestinal disorders (Wilder-Smith et al., 2013). However, fructose treatment did not significantly affect the AQP3 protein expression in the colon of rats compared to those in the constipation model group (Cong et al., 2019).
AQPs represent important targets for traditional Chinese medicine in the treatment of digestive disorder including constipation (Huang et al., 2022). A recent study showed that Xuanhuang Runtong tablets could reverse the disorder of AQP3 expression and thus relieve slow transit constipation in mice (Liang et al., 2023). Moreover, the laxative effect of Daiokanzoto has been confirmed when used as a long-term treatment for chronic constipation probably by maintaining a persistent decrease of AQP3 expression and gut microbiota homeostasis (Kon et al., 2018b). Similar findings were reported in another constipated-rat model that administrations with a natural medicine Rhubarb extract or its core functional component sennoside A would decrease the AQP3 expression in the colon (Kon et al., 2014). The laxative effect of Rhubarb extract might be associated with the major metabolite of sennoside A rheinanthrone, which could activate the macrophages to secret prostaglandin E2 as a paracine factor for decreasing AQP3 expression in colon mucosa epithelial cells (Kon et al., 2014). Moreover, sennoside A (2.6 mg/kg for 7 days) exerts strong laxative effect by regulating gut microbiota and water channels of AQP1 and AQP3 in the colon of mice (Zhang et al., 2023). These results indicated that most stimulant laxatives could decrease AQP3 expression in the colon for alleviating constipation. Nevertheless, another study indicated that high doses of sennosides displayed prominent laxative actions by downregulating most AQPs (AQP4, 5, 6, 7, and 8) expression in the colon but upregulating AQP3 expression in diarrhea-rats (Cao et al., 2018). As sennoside A are the main components of sennosides, and could be metabolized into rhein, emodin, and aloe-emodin (Sakulpanich and Gritsanapan, 2009). A previous study also showed that the laxative effect of emodin was associated with the increased expression of AQP3 in mice colon and HT-29 cells by regulating PKA/p-CREB signal pathway (Zheng et al., 2014). Based on the aforementioned studies, the variations of different results may be related to the doses of treatments, delivery method, administration period, experiment design, and managements.
Notably, increasing data has indicated important relationship between AQP3 and prostaglandin E2 during the treatment of constipation. For example, the laxative effect of bisacodyl was attributable to decreased AQP3 expression in the colon induced by increased prostaglandin E2 secretion from macrophages (Ikarashi et al., 2011b). Similarly, orally administering a purifed resin glycoside fraction from pharbitis semen to rats, could mediate its laxative effect by downregulating AQP3 expression through the cyclooxygenase-2-mediated secretion of prostaglandin E2 (Zhu et al., 2019). These data indicated that the decreased expression of AQP3 by prostaglandin E2 stimulation would inhibit water transfer from the luminal to the vascular side, thereby leading to laxative effects of the above mentioned stimulant laxatives (Ikarashi et al., 2011b; Kon et al., 2014; Zhu et al., 2019).
The broadly distributed 5-HT4 receptors in the gastrointestinal tract have been well recognized as an important target for treating constipation and intestinal inflammation (Mawe et al., 2022). Serotonergic agents such as fluoxetine are usually displaying the selective laxative action on 5-HT4 receptors. Recent study has suggested that the enhancement of intestinal motility could be augmented by treatment with tryptophan-synthesizing bacteria through increased 5-HT signaling pathway with involvement of 5-HT4 receptor (Legan et al., 2023). Thus, the 5-HT4 receptor agonists used to treat functional constipation and colitis may involve the increased colonic motility by stimulation of serotonin release from enterochromaffin cells (Mawe et al., 2022) as well as the regulation of synthetic enzyme tryptophan hydroxylase in the intestine (Mawe and Hoffman, 2013). Previous study has shown that pretreatment with fluoxetine (inhibitor of 5-HT reuptake transporter) inhibited the upregulated AQP3 mRNA expression in the colon of rats with constipation (Kon et al., 2015).
Increasing evidence has suggested that probiotics and prebiotics display positive effects on colonic transit and defaecation frequency during functional constipation by targeting the VIP-cAMP-PKA-AQP3 signaling pathway (Cong et al., 2019; Tan et al., 2021). Specially, a mixture of probiotic containing Bifidobacterium breve DM8310, Lactobacillus acidophilus DM8302 and Lactobacillus casei DM8121 at a ratio of 1:1:1 could increase the fecal water content and ameliorate loperamide-induced constipation by regulating AQP3 expression in rat colon (Deng et al., 2018). Interestingly, postbiotic of hawthorn-porbiotic could improve intestinal movement and elevated AQP3, epithelial sodium channel gamma and mucin-2 expression in the colon for regulating intestinal water and sodium metabolism in constipated mice (Wei et al., 2023). Besides, rats receiving a diet containing 5% partially hydrolyzed guar gum (a watersoluble dietary fiber) had lower constipation symptoms probably by suppressing water transport from the luminal side to the vascular side through decreased colonic AQP3 expression (Kon et al., 2022). Furthermore, Cong et al., 2019 found that high specific volume polysaccharide, as a new type of dietary fiber, could regulate intestinal water metabolism in constipated rats by lowering the colonic AQP3 expression via cAMP-PKA pathway, and highlighted important role of the brain-gut-microbiome axis in the pathogenesis of constipation. Thus, these results indicate the potential effectiveness of probiotics and prebiotics on alleviation of constipation at least by partially targeting the colonic AQP3 expression. Notably, investigators have shown that dietary Lactobacillus rhamnosus GG significantly relieved gastrointestinal motility disorders by regulating 5-HT4R expression in a constipation mouse model (Gu et al., 2022). In line with this, a similar study has confirmed the involvement of 5-HT pathway and alteration of the gut microbiota in alleviating constipation by Ligilactobacillus salivarius Li01 (Qiu et al., 2022). Further evidence supporting this finding comes from studies in constipated-rats demonstrating the laxative effects of herbal extracts, including hot water extract of M. oleifera leaves (Li et al., 2023) and Chinese medicine Houpo Paiqi mixture (Wang J. et al., 2023), were related to gastrointestinal motility and intestinal fluid secretion via the modulation of 5-HT pathway. Another study also indicated that the yellow tea extract consumption protected against operamide-induced constipation in mice partially through the upregulation of 5-HT3 and 5-HT4 expression and downregulation of AQP3 and AQP4 expression in the colon (Cao et al., 2021). However, due to the varieties of probiotic strains, prebiotic, and complex ingredients of herbal extracts, their regulation on AQP3 expression may differ as well. Thus, further studies are required to reveal the potential mechanism of specific probiotic, prebiotic and herbal extract treatments on 5-HT4R expression through regulation of gut microbiota and and clarify their interactions with AQP3 regulation for constipation.
Lubricating agents (such as mineral oil, liquid paraffin, glycerol, and glycerol enema, etc.) have been commonly used to alleviate the constipation without changing the intestinal fluid homeostasis. For example, olive oil ointment can be used as a safe, well-tolerated, and effective herbal preparation in children with functional constipation (Arman-Asl et al., 2021). Moreover, the daily use of both mineral oil and plant oils (olive oil or flaxseed oil) was effective in the treatment of constipation in patients undergoing hemodialysis (Ramos et al., 2015). Currently, there have still been relatively few studies investigating the lubricating agents for constipation on the expression of AQP3. However, in one such study concerning a mouse model of constipation established by diphenoxylate, the authors found that treatment with rhein has been shown to effectively relieve the symptoms of constipation by reducing the expression of AQP3 in the colonic mucosa (Sun et al., 2018).
Previous study has indicated that the total diterpenoids extracted from the roots of Euphorbia pekinensis could increase water content in both fecal and colonic samples followed by severe diarrhea in mice, which might be associated with the upregulation of AQP3 and AQP4 in the colon (Wang et al., 2017). Notably, Pekinenin C, as a casbane diterpenoid in E. pekinensis, has also been shown to induce inflammation and increase both AQP3 mRNA and protein expression in HT-29 cells alone (Wang et al., 2017) or co-culturing with RAW 264.7 cells (Yu et al., 2018). It should be noted that the laxative effects of Maren pills (Zhan et al., 2020) or Pekinenin C (Yu et al., 2018) in alleviating constipation may be associated with the increased expression of AQP3 via nuclear factor κB (NF-κB) signal pathway. Moreover, a recent study reported that the citric acid-enriched extract of ripe Prunus mume (Siebold) effectively improved the symptoms of constipation without causing diarrhea and tolerance by regulating the expression of AQP3 and prostaglandin E2 in the rat colons (Na et al., 2022).
Interestingly, the seasonal changes could affect the colonic expression levels of AQP3, AQP4, and AQP8 through cAMP/PKA pathway to affect the intestinal water metabolism, which resulted in seasonal differences in intestinal flora (Li et al., 2023). However, mulberry (Morus atropurpurea) fruit had a protective effect against diphenoxylate-induced constipation through modulation of gut microbiota and decreased expression of AQP3, AQP4, AQP8, and AQP9 in the colon of mice (Hu et al., 2019). Besides, naringenin, a natural flavonoid widely found in citrus fruits and tomatoes, has great application potential in the treatment of constipation. Yin et al. (2018) also found that naringenin treatment at 300 mg/kg significantly increased the AQP3 mRNA and protein expression levels in the colon of mice, mainly in the apical and lateral mucosal epithelial cells, which were positively associated with the increase in fecal water content and the relief of constipation. Whereas a naturally occurring flavone luteolin effectively reversed loperamide-induced upregulation of AQP3, AQP4, and AQP8 in the colon and greatly alleviated the functional constipation in mice (Wang et al., 2023). In addition to plant extracts, another study indicated that oral administration of snail-derived mucin extract ameliorated constipation symptoms induced by loperamide in rats by partially downregulating the expression of AQP3 and AQP8 (Kim et al., 2023). Notably, due to the variations of the constipation model, treatment doses, sampling and analysis strategies, the regulation of other agents for constipation on AQP3 expression may involve different mechanisms. While the exact mechanisms for these agents for constipation on the expression of AQP3 are still unclear, which requires more investigations in future studies.
Increasing evidence has shown that decreased AQP3 expression is associated with gastrointestinal infections and plays an important role in the inflammatory process of several human diseases (Meli et al., 2018; Sisto et al., 2019). For example, inflammatory bowel diseases often involve with dysfunctions in electrolyte and water transport in the gut. Previous study has showed that the AQP3 expression at both mRNA and protein levels was significantly reduced in ileum, proximal colon and distal colon of 2,4,6-trinitrobenzene sulphonic acid-induced rat colitis, indicating its potential roles in the pathogenesis of inflammatory bowel disease (Zhao et al., 2014). Another study also found that the AQP3 mRNA expression was reduced in the colon of rats with irritable bowel syndrome via NF-κB pathway, which might be associated with liquid water metabolic abnormalities and intestinal permeability alterations (Chao and Zhang, 2017). Furthermore, the expression of AQP3 was significantly reduced in the ileal mucosa of patients with Crohn’s disease at early stage (Ricanek al., 2015). Previous study has also confirmed that AQP3, AQP7, AQP10, and AQP11 mRNAs were significantly decreased in duodenal biopsies of untreated celiac disease patients (Laforenza et al., 2010). It was also found that the transcription and protein expression of AQP3 in HT-29 cells were decreased by lipopolysaccharide challenge in a dose- and time-dependent manner via p38/c-Jun N-terminal kinase signaling pathway (Li et al., 2015). Previous study in young pigs also showed that the AQP3 mRNA and protein expression in the jejunum was significantly decreased after lipopolysaccharide challenge, while dietary α-ketoglutarate supplementation did not significantly attenuate the jejunal expression of AQP3 in lipopolysaccharide-challenged piglets (He et al., 2017). Collectively, most of these aforementioned studies indicated that AQP3 might be involved in the pathogenesis of inflammatory bowel diseases, and its expression may decrease in response to intestinal inflammation.
Inflammatory cytokines are closely involved in intestinal inflammation with disordered ion and water transport. Tumor necrosis factor alpha (TNF-α) downregulated the constitutive transcriptional activity of the AQP3 promoter (containing the TATA-box and SP1 site), probably via the regulation of methyl ethyl ketone (MEK)/extracellular regulated protein kinases (ERK) and NF-κB signaling pathways by suppression of the specificity protein transcription factor 3 (Sp3) in HT-29 cells (Peplowski et al., 2017). Moreover, interferon gamma (IFN-γ) has been demonstrated to suppress the expression of AQP3 in the enteroids derived from human colonic biopsies as well as in HT-29 cells through increased acetylation or decreased deacetylation in AQP3 promoter (Peplowski et al., 2018). By using luciferase reporter system and chromatin immunoprecipitation analysis, they also showed that the constitutive expression of AQP3 by IFN-γ treatment in HT-29 cells was dependently regulated by Sp3, since Sp3 could bind to the AQP3 promoter (Peplowski et al., 2018). Moreover, the expression of AQP3 transcript could be inhibited by a PKA inhibitor of cAMP pathway and was upregulated by VIP in HT-29 cells (Itoh et al., 2003), which confirmed previous reports on regulating AQP3 expression through cAMP pathways as mentioned above. Interestingly, another study also indicated that AQP3 in macrophage was involved in NOD-like receptor thermal protein domain associated protein 3 (NLRP3)-inflammasome activation, and AQP3 inhibition or silencing could block the setting of inflammatory responses and decreased the production of interleukin-6 (IL-6), proIL-1β, and TNF-α (da Silva et al., 2021). These results suggested that the inflammatory mediators in the gut lumen may regulate the expression of AQP3 by interacting the AQP3 promoter regions with transcription factors such as SP3, with its complex regulatory mechanisms currently not fully understood.
Apart from the changes of AQP3 expression, previous study showed that AQP3 has one N-glycosylation site, and the misrouting of AQP3 structure may result in fluid imbalance, thus impacting Crohn’s disease and ulcerative colitis (Cohly et al., 2008). This was confirmed in another study since glycosylation was essential for cell surface expression of AQPs (Camilleri et al., 2019).
Increasing evidence has suggested that AQP3 plays a critical role maintaining the normal intestinal integrity and the homeostasis of intestinal epithelial barrier function. Specially, knockdown of AQP3 in the Caco-2 cells resulted in an increase in bacterial translocation and epithelial paracellular permeability accompanied with a reduction of transendothelial electrical resistance and decreased expression of tight junction proteins (e.g., claudin-1 and occludin) (Zhang et al., 2011). Another study also indicated that the expression levels of AQP3 and occludin were significantly reduced in intestinal mucosa of septic mice accompanied by an increase of plasma diamine oxidase concentration, indicating AQP3 might alleviate intestinal damage caused by sepsis (Zhu et al., 2019). Recent study has also shown that dietary berberine supplementation significantly upregulated the mRNA expression of zona occludens 1 (ZO-1) and occludin in ETEC K88-challenged piglets (Zhu et al., 2023). Moreover, daidzein could reversed lipopolysaccharide-induced downregulation of ZO-1, occludin, claudin-1, and AQP3 expression, maintained tight junction and integrity of Caco-2 cells (Zhang et al., 2022). Interestingly, microRNA-874 could promote intestinal barrier dysfunction with increased paracellular permeability and altered expression of tight junction proteins (e.g., occludin and claudin-1) by suppressing the expression of AQP3 after binding to the 3′ untranslated region (UTR) of AQP3 mRNA as shown in Caco-2 cells (Zhi et al., 2014). Additional study from the same research group showed that human long noncoding RNA H19 may function as a competing endogenous RNA to suppress the AQP3 expression by sponging microRNA-874 in maintaining the intestinal barrier function, indicating an interaction of H19 with microRNA-874 to post-transcriptionally regulate the AQP3 protein (Su et al., 2016). In addition, the tight junction protein ZO-1 expression was decreased in AQP3 knockdown IPEC-J2 cells in response to PEDV infection (Wang et al., 2022). These updated studies indicated that AQP3 may function as a new target for improving the intestinal barrier function.
Interestingly, the AQP3-deficient mice displayed a reduced cell proliferation of enterocytes and developed severe colitis (Thiagarajah et al., 2007), probably due to an impaired transport of glycerol for maintaining normal production of ATP energy (Hara-Chikuma and Verkman, 2008). Similarly, AQP3 deficiency leads to glycerol uptake reduction and decreased energy and lipid production, thereby impairing the proliferation in gastric cancer cells (Li et al., 2016). This was true when oral glycerol administration could effectively improve survival and reduce the severity of colitis in AQP3-null mice (Thiagarajah et al., 2007). Moreover, AQP3 facilitates cell migration, proliferation and epithelialization during wound healing in the skin of pigs (Hamed et al., 2017). Interestingly, resveratrol effectively inhibited the proliferation of normal human epidermal keratinocytes by downregulating the expression of AQP3 via the silent mating type information regulation 2 homolog 1 (SIRT1)/aryl hydrocarbon receptor nuclear translocator (ARNT)/ERK dependent pathway (Wu et al., 2014). Similarly, AQP3 could also promote the migration and invasion of human extravillous trophblast cells (Nong et al., 2021).
On the other hand, AQP3 overexpression induced by human epidermal growth factor could facilitate colorectal carcinoma cell migration, while phosphatidylinositol 3-kinase (PI3K) and protein kinase B (Akt) inhibitor would inhibit the upregulated expression of AQP3 in human colorectal carcinoma cells, indicating the important role of AQP3 in cell growth and migration by regulation of PI3K/Akt signaling pathway (Li et al., 2013). In addition, AQP3 overexpression modified the cell cycle pattern, increased the cell proliferation, and reduced cell apoptosis by regulating cell volume and complexity as well as H2O2 permeability in mammalian cells (Galan-Cobo et al., 2015). Importantly, another study also indicated that the changes in AQP3 protein expression could cause alterations in cell membrane fluidity of Caco-2 cells and thus affect drug absorption rates (Ikarashi et al., 2019b). Cell growth and migration induced by epidermal growth factor stimulation were attenuated in AQP3 knockdown cancer cells A431 and H1666 (Hara-Chikuma et al., 2016). Both in vitro and in vivo studies showed that the expression level of AQP3 in breast cancer cells was related to their migration ability (Satooka and Hara-Chikuma et al., 2016). Previous results also suggested that overexpression of AQP3 reduced cell apoptosis via the ERK1/2 pathway in nucleus pulposus cells (Zhang et al., 2022).
Notably, microRNA-874 inhibited AQP3 expression by binding to the UTR of AQP3 mRNA in gastric cancer cells, and suppressed proliferation, migration and invasion for tumorigenesis (Jiang et al., 2014). Similarly, miR-877 has been found to be negatively correlated with AQP3 mRNA expression in gastric cancer tissues, and targeting AQP3 through microRNA-877 would suppress gastric cancer development and progression (Zhu et al., 2020). However, whether AQP3 could mediate the proliferation and migration of porcine intestinal epithelial cells and how the microRNA modulates its expression remains unknown.
It is suggested that all cells could produce and consume H2O2 (Forman et al., 2016). On the one hand, H2O2 occurs in normal metabolism in mammalian cells (Sies and Chance, 1970), and functions as a central redox signaling molecule in physiological oxidative stress (Sies, 2017). The production of H2O2 can be generated from the NADPH oxidase (Nox) enzyme in the plasma membrane, the oxidative phosphorylation in mitochondrial respiratory chain, and diverse oxidases, as well as the oxidative protein folding in the endoplasmic reticulum and the peroxisomes (Kakihana et al., 2012; Sies, 2017). On the other hand, the concentration of H2O2 within cells could be more than 100-fold lower than extracellular concentrations due to the presences of catalases, peroxidases, and peroxiredoxins for the elimination of H2O2 (Sies, 2017). Usually, the intracellular physiological range of H2O2 likely spans between 1 and 10 nM for maintaining the normal proliferation, migration and angiogenenesis, while adaptive stress and oxidative stress occur at higher concentrations (10–100 nM) (Sies, 2017). However, the supraphysiological concentrations of intracellular H2O2 (>100 nM) would cause inflammation, growth arrest and cell death (Sies, 2017). Interestingly, the plasma concentration of H2O2 is about 1–5 μM range as summarized in a systemic literature review (Forman et al., 2016). Thus, the dose-dependent effects of H2O2 concentration in maintaining cellular redox signaling and oxidative stress are important to the physiology and pathophysiology of human and animal cells.
It seems that the evidence for H2O2 transport through biological membranes is controversial based on the available literature. Simple diffusion (free diffusion) was thought to be the primary approach of H2O2 transmembrane transport (Crompton, 1999). However, current evidence has indicated that H2O2 is a molecule with similar chemical properties to water (e.g., chemical composition elements, hydrogen bond stability, and polarity) and easily soluble in water, and thus could traverses cellular membranes through specific AQPs channels called peroxiporins (Lismont et al., 2019). The earlier findings should be dated to 2000 when Henzler and Steudleused the isolated internodal cells of Chara corallina to prove the permeation of H2O2 across a cell membrane probably mediated by water channels, since the permeability of both water and H2O2 were substantially reduced by HgCl2 known as the inhibitor of water channels (Henzler and Steudle, 2000). Later, Bienert et al. for the first time identified specific AQPs to facilitate the diffusion of H2O2 across membranes (Bienert et al., 2006; 2007). To date, several peroxiporins have been identified including AQP3, AQP5, AQP6, AQP7, AQP8, AQP9, and AQP11 with permeability to H2O2 (Rodrigues et al., 2019; Bestetti et al., 2020; Wragg et al., 2020; Krüger et al., 2021; Pellavio et al., 2022; Ma et al., 2023), which may play important roles in oxidative stress regulation. Notably, the driving force of H2O2 permeability across membrane mediated by AQPs may be related to the gradients between extracellular and intracellular H2O2 concentrations as indicated above (Sies, 2017).
Importantly, human AQP3 facilitated H2O2 transport through its NPA motif was confirmed using a combination of metadynamics and transition path sampling methods (Wragg et al., 2020). Considering this role of NPA motifs, it could be explained why AQP3 mediated route of H2O2 transport could be more efficient than simple diffusion. Until now, numerous studies have proved the important function of AQP3 as one of the important peroxiporins to mediate the transport of H2O2 in various cells. For example, previous study has demonstrated that AQP3-mediated cellular H2O2 uptake was essential for TNF-α-induced NF-kB activation, while AQP3 deficiency would suppresses NF-kB activation and H2O2 induction in epidermal keratinocytes (Hara-Chikuma et al., 2015). Moreover, T cell migration toward chemokines was highly dependent on AQP3-mediated H2O2 uptake, and might play an important role in the immune responses (Hara-Chikuma et al., 2012). Notably, AQP3-mediated H2O2 transport into fibroblasts required activation of TGF-β1, while silencing AQP3 would decrease H2O2 levels and TGF-β1 expression, and alleviated fibrosis in mice (Luo et al., 2016). Another study also revealed the oxygen-dependent regulation of AQP3 expression could be significantly enhanced by hypoxia in L929 fibrosarcoma cells (Hoogewijs et al., 2016). Furthermore, the transport of H2O2 into the endosomal lumen mediated by AQP3 can regulate the endosomal lipid peroxidation closely related to oxidative stress (Nalle et al., 2020).
Increasing data has suggested that AQP3 may mediate the transport of H2O2 produced by cell-surface Nox enzyme. For example, Miller et al. (2010) used the genetically encoded fluorescent sensor to detect H2O2, and confirmed that AQP3 could mediate Nox-derived H2O2 uptake upon growth factor stimulation to regulate downstream intracellular signaling. Notably, another study also indicated that AQP3 could associate epidermal growth factor receptor and Nox2 to increase cellular H2O2 uptake (Hara-Chikuma et al., 2016). Consistently, the extracellular H2O2 produced by Nox2 activation could be transported into breast cancer cells via AQP3 (Satooka and Hara-Chikuma et al., 2016). Another study also reported an upregulation of Nox1/2 and downregulation of AQP3 under oxidative stress induced by H2O2 (Dong et al., 2020). These results indicated that Nox1/2 is an important source of H2O2, and plays important roles in AQP3-mediated H2O2 permeability.
Intestinal oxidative stress often occurs with inflammation and disturbs intestinal homeostasis. In diabetic mice, the increased oxidative stress was found to be accompanied with decreased AQP3 expression (Ikarashi et al., 2019a). Previous study has demonstrated that oxidative stress insult modulated the expression of peroxiporins which were associated with oxidative stress resistance in colon cancer cell lines (Cipak Gasparovic et al., 2021). These authors found that under oxidative stress exposure, the AQP3 expression in HCT 116 cells was significantly increased after treatment with 100 μM H2O2 compared to other colon cancer cell lines (Caco-2, HT-29, and SW620) (Cipak Gasparovic et al., 2021). This might be due to the fact that AQP3 could function as peroxiporin to mediate the entry of extracellular H2O2 into colonic epithelial cells, since defective repair after wounding and impaired H2O2 responses to pathogen infection were observed in both AQP3-deleted Caco-2 cells and AQP3 knockout mice (Thiagarajah et al., 2017). Indeed, the H2O2 entry through AQP3 would regulate extracellular Ca2+ influx during the honey-mediated wound healing (Martinotti et al., 2019). This was in accordance with another study that oxidative damage could significantly decrease the AQP3 expression and increase apoptosis of nucleus pulposus cells, while AQP3 over-expression could partially attenuate the cellular apoptosis through p38 mitogen-activated protein kinase (MAPK) pathway under oxidative damage (Xu et al., 2018). Collectively, these data indicated that AQP3 could function as an important regulator of redox status in intestinal epithelial cells, and may represent an promising targeting for the development of potential managements for alleviating the intestinal oxidative-associated disorders or diseases in human and animals. It should be noted that diverse cellular stresses reversibly inhibited the AQP8-dependent transport of H2O2 and water to impact cell growth and survival (Medraño-Fernandez et al., 2016). However, due to the concern of H2O2 as a highly reactive molecule, more functional researches are needed to clarify whether H2O2 could transports via AQP3 without oxidizing lipids and proteins and the water channel itself, as well as the regulation mechanism of H2O2 production and elimination in intestinal epithelial cells.
Previous study has shown that the protective effect of AQP3 in alleviating the oxidative damage might involve the elimination of intracellular reactive oxygen species (ROS) (Laforenza et al., 2016). Many studies on plant extracts have confirmed the involvement of AQP3 in regulating the oxidative stress. For example, pterostilbene, as a dimethylated analog of resveratrol, could significantly reduce particulate matter-induced intracellular ROS production and attenuate oxidative stress, and increase AQP3 expression in keratinocytes (Teng et al., 2021). Interestingly, monomethylfumarate treatment attenuated the generation of ROS following H2O2 treatment, and stimulated the increased AQP3 mRNA and protein expression in keratinocytes (Helwa et al., 2017). Similarly, Pellavio et al. (2017) has reported several natural antioxidant compounds reduced the H2O2 content of heat-stressed cells or prevent H2O2 cellular entry by regulation the functional properties of AQPs (1, 3, 8, and 11). The evidence of AQP3 in regulation of intestinal oxidative has also been recognized. By using an in vitro CELLigence system and in vivo evaluation in a colitis model of mice, Dong et al. (2020) found that compared to other plant bioactive components, quercetin most effectively alleviated the decreased cell index, reversed cell damage, and decreased reactive oxygen species and apoptosis ratio caused by H2O2. They concluded that the protective effects of quercetin in intestinal oxidative damage might be related to modulation of glutathione and H2O2 production and transport via Nox1/2 and AQP3, respectively (Dong et al., 2020). Importantly, meyloperoxidase (MPO) enzyme could use H2O2 to oxidize chloride for catalyzing the production of a strong oxidant hypochlorous acid (Dhiman et al., 2009). A recent study has indicated that quercetin effectively attenuated human vascular endothelial dysfunction by inhibiting MPO/H2O2-mediated hypochlorous acid production as well as Nox function (Li et al., 2023). Thus, the application of natural compounds may be helpful for reducing brain ischemia injury by targeting MPO-mediated oxidative stress and inflammation (Chen et al., 2020).
However, there were few evidence reporting the potential involvement of other antioxidant enzymes in regulating intestinal oxidative stress by targeting AQP3. Nonetheless, it should be noted that glutathione was supposed as a key determinant in the elimination of peroxides by the intestine (Aw, 2005). A previous study has demonstrated that Centella asiatica extracts were able to increase the content of glutathione and superoxide dismutase while reducing intracellular ROS generation, accompanied with downregulation of inflammatory factors and upregulation of AQP3 expression (Lin et al., 2023). Importantly, ulcerative colitis is often associated with oxidative stress and inflammation. For the therapies of ulcerative colitis, synbiotics could inhibit the oxidative stress’ effects and alleviate the colonic inflammation by lowering the ROS and improving the level of antioxidant enzymes such as catalase, glutathione peroxidase, and superoxide dismutase (Ashique et al., 2023). Moreover, a previous study found that the strong antioxidant properties of cell-free culture supernatant from the Bifidobacterium infantis strain on the skin, might be associated with reduced intracellular ROS and malondialdehyde contents and improved activities of catalase, glutathione peroxidase, and superoxide dismutase as well as upregulation of AQP3 in H2O2 -treated HaCaT cells after treatment (Ma et al., 2022).
Autophagy is closely associated with the development of intestinal oxidative stress, and may involve with the change of AQP3 expression. Since AQP3 may also mediate arsenic uptake, and upregulation of AQP3 expression would contribute to arsenic-induced autophagy in keratinocytes (Yu et al., 2021). The previous in vitro study has also found that AQP3 overexpression promoted the conversion of autophagy-related protein LC3-I to LC3-II and upregulated the ATG5 and Beclin-1 expression in gastric cancer cell line AGS, whereas AQP3 knockdown inhibited the transformation of LC3-I to LC3-II in MGC803 and SGC7901 cells, indicating that AQP3 expression regulated autophagy differently in different gastric cancer cells (Dong et al., 2016). Interestingly, AQP3 silencing inhibited cell viability and autophagy in gastric cancer cells while AQP3 overexpression activated autophagy via Krüpper like factor 5 as confirmed by chromatin immunoprecipitation and dual luciferase reporter assays (Dai et al., 2023). Moreover, increasing data has indicated that ETEC infection caused significant autophagy in porcine intestinal epithelial cells (Tang et al., 2014; Xia et al., 2019; Luo et al., 2022). In addition to ETEC, a recent study showed that PEDV induced autophagy in Vero CCL81 cells (Ren et al., 2022). As mentioned above, whether ETEC or PEDV-induced autophagy is associated with regulation of AQP3 in the gut of piglets remained unclear and needs further investigations.
Previous study has shown that the diffusion and uptake of exogenous H2O2 mediated by AQP3 significantly increased the intracellular reactive oxygen species levels and inhibited rapamycin-induced autophagy via activating the Akt/mammalian target of rapamycin (mTOR) pathway in lung adenocarcinoma cells (Wang et al., 2021). Indeed, another study has also confirmed that mTOR signaling pathway could regulate AQP3 expression, and overexpression of AQP3 could induce autophagy and promote proliferation and migration of gastric cancer cells (Yu et al., 2022). Moreover, latent membrane protein 2A (LMP2A) induced the phosphorylation of ERK, which thereby activated DNMT3a transcription and induced loss of AQP3 expression through CpG island methylation of AQP3 promoter in Epstein-Barr virus-associated gastric carcinoma (Wang et al., 2019). This was true since LMP2A could downregulate AQP3 by inhibiting mTOR signaling pathway to promote autophagy of gastric cancer cells (Yu et al., 2022). These studies suggested that LMP2A may regulate the AQP3 expression and activate autophagy by targeting mTOR pathways. However, it remained unclear how mTOR pathway might regulate autophagy of intestinal epithelial cells in human and animals by targeting AQP3, and therefore needs further investigations.
Current progress has indicated that AQP3 may act an important target for the disorders that involve disruption of intestinal fluid homeostasis (as summarized in Figure 2, created with BioRender.com), such as diarrhea, constipation, inflammatory bowel disease, and irritable bowel syndrome. It has been recognized that AQP3 is essential for maintaining intestinal proliferation and migration as well as intestinal barrier function, and mediates the transport of H2O2 via Nox, which thereby plays important roles in intestinal oxidative stress and autophagy. Great progress has been made through dietary nutrients to regulate intestinal health by targeting AQP3. However, significant work should be done concerning the specific roles of AQP3 mediating the potential protective intervention strategies for regulating gut health in human and animals under physiological and pathological conditions. Importantly, histone methylations and acetylations or modifications of DNA methylation status may affect gene expression of AQP3. Thus, given the aformentioned findings and important implications, more investigations are necessary to elucidate the potential mechanism of AQP3 in gut health at both post-transcription and post-translation levels in future studies.
CZ: Funding acquisition, Writing–original draft, Writing–review and editing. XN: Writing–review and editing. QL: Writing–review and editing. YB: Writing–review and editing. ZJ: Supervision, Writing–review and editing.
The author(s) declare financial support was received for the research, authorship, and/or publication of this article. This research was funded by the grants from the Guangdong Basic and Applied Basic Research Foundation, China (Grant Number 2022A1515011185).
The authors declare that the research was conducted in the absence of any commercial or financial relationships that could be construed as a potential conflict of interest.
All claims expressed in this article are solely those of the authors and do not necessarily represent those of their affiliated organizations, or those of the publisher, the editors and the reviewers. Any product that may be evaluated in this article, or claim that may be made by its manufacturer, is not guaranteed or endorsed by the publisher.
Alhotan R. A., Al-Sagan A. A., Al-Abdullatif A. A., Hussein E. O. S., Saadeldin I. M., Azzam M. M., et al. (2021). Interactive effects of dietary amino acid density and environmental temperature on growth performance and expression of selected amino acid transporters, water channels, and stress-related transcripts. Poult. Sci. 100, 101333. doi:10.1016/j.psj.2021.101333
Argenzio R. A., Whipp S. C. (1983). Comparison of the effects of disodium ethylenediaminetetraacetate, theophylline, and deoxycholic acid on colonic ion transport and permeability. Am. J. Vet. Res. 44, 1480–1487.
Arman-Asl H., Mohammadpour A. H., Hamedi A., Emami S. A., Abbaspour M., Sahebkar A., et al. (2021). Evaluation of the anti-constipation effects of abdominal application of olive oil ointment in children 1-4 years old: a pilot placebo-controlled, double-blind, randomized clinical trial. Adv. Exp. Med. Biol. 1328, 411–419. doi:10.1007/978-3-030-73234-9_27
Arslan G. G., Eşer I. (2011). An examination of the effect of castor oil packs on constipation in the elderly. Complement. Ther. Clin. Pract. 17, 58–62. doi:10.1016/j.ctcp.2010.04.004
Ashique S., Mishra N., Garg A., Sibuh B. Z., Taneja P., Rai G., et al. (2023). Recent updates on correlation between reactive oxygen species and synbiotics for effective management of ulcerative colitis. Front. Nutr. 10, 1126579. doi:10.3389/fnut.2023.1126579
Aw T. Y. (2005). Intestinal glutathione: determinant of mucosal peroxide transport, metabolism, and oxidative susceptibility. Toxicol. Appl. Pharmacol. 204, 320–328. doi:10.1016/j.taap.2004.11.016
Benga G. (2009). Water channel proteins (later called aquaporins) and relatives: past, present, and future. IUBMB Life 61, 112–133. doi:10.1002/iub.156
Bestetti S., Galli M., Sorrentino I., Pinton P., Rimessi A., Sitia R., et al. (2020). Human aquaporin-11 guarantees efficient transport of H2O2 across the endoplasmic reticulum membrane. Redox Biol. 28, 101326. doi:10.1016/j.redox.2019.101326
Bienert G. P., Moller A. L., Kristiansen K. A., Schulz A., Moller I. M., Schjoerring J. K., et al. (2007). Specific aquaporins facilitate the diffusion of hydrogen peroxide across membranes. J. Biol. Chem. 282, 1183–1192. doi:10.1074/jbc.M603761200
Bienert G. P., Schjoerring J. K., Jahn T. P. (2006). Membrane transport of hydrogen peroxide. Biochim. Biophys. Acta. 1758, 994–1003. doi:10.1016/j.bbamem.2006.02.015
Bottino C., Vazquez M., Devesa V., Laforenza U. (2016). Impaired aquaporins expression in the gastrointestinal tract of rat after mercury exposure. J. Appl. Toxicol. 36, 113–120. doi:10.1002/jat.3151
Camilleri M. (2015). Intestinal secretory mechanisms in irritable bowel syndrome-diarrhea. Clin. Gastroenterol. Hepatol. 13, 1051–1057. doi:10.1016/j.cgh.2014.07.020
Camilleri M., Carlson P., Chedid V., Vijayvargiya P., Burton D., Busciglio I. (2019). Aquaporin expression in colonic mucosal biopsies from irritable bowel syndrome with diarrhea. Clin. Transl. Gastroenterol. 10, e00019. doi:10.14309/ctg.0000000000000019
Cao M., Yang M., Ou Z., Li D., Geng L., Chen P., et al. (2014). Involvement of aquaporins in a mouse model of rotavirus diarrhea. Virol. Sin. 29, 211–217. doi:10.1007/s12250-014-3469-z
Cao P. Q., Li X. P., Ou-Yang J., Jiang R. G., Huang F. F., Wen B. B., et al. (2021). The protective effects of yellow tea extract against loperamide-induced constipation in mice. Food Funct. 12, 5621–5636. doi:10.1039/d0fo02969f
Cao Y., He Y., Wei C., Li J., Qu L., Zhang H., et al. (2018). Aquaporins alteration profiles revealed different actions of senna, sennosides, and sennoside A in diarrhea-rats. Int. J. Mol. Sci. 19, 3210. doi:10.3390/ijms19103210
Chao G., Wang Z., Yang Y., Zhang S. (2020). LncRNA H19 as a competing endogenous RNA to regulate AQP expression in the intestinal barrier of IBS-D patients. Front. Physiol. 11, 602076. doi:10.3389/fphys.2020.602076
Chao G., Zhang S. (2017). Aquaporins 1, 3 and 8 expression in irritable bowel syndrome rats' colon via NF-κB pathway. Oncotarget 8, 47175–47183. doi:10.18632/oncotarget.17565
Chen S., Chen H., Du Q., Shen J. (2020a). Targeting myeloperoxidase (MPO) mediated oxidative stress and inflammation for reducing brain ischemia injury: potential application of natural compounds. Front. Physiol. 11, 433. doi:10.3389/fphys.2020.00433
Chen W., Peng X., Yu J., Chen X., Yuan M., Xiang R., et al. (2020b). FengLiao affects gut microbiota and the expression levels of Na+/H+ exchangers, aquaporins and acute phase proteins in mice with castor oil-induced diarrhea. PLoS One 15, e0236511. doi:10.1371/journal.pone.0236511
Cipak Gasparovic A., Milkovic L., Rodrigues C., Mlinaric M., Soveral G. (2021). Peroxiporins are induced upon oxidative stress insult and are associated with oxidative stress resistance in colon cancer cell lines. Antioxidants (Basel) 10, 1856. doi:10.3390/antiox10111856
Cohly H. H., Isokpehi R., Rajnarayanan R. V. (2008). Compartmentalization of aquaporins in the human intestine. Int. J. Environ. Res. Public Health. 5, 115–119. doi:10.3390/ijerph5020115
Cong L., Duan L. W., Su W. P., Hao S., Li D. F. (2019). Efficacy of high specific volume polysaccharide - a new type of dietary fiber - on molecular mechanism of intestinal water metabolism in rats with constipation. Med. Sci. Monit. 25, 5028–5035. doi:10.12659/MSM.916526
Crompton M. (1999). The mitochondrial permeability transition pore and its role in cell death. Biochem. J. 341, 233–249. doi:10.1042/bj3410233
Dai X., Chen Y., Chen N., Dou J., Zhuang H., Wang J., et al. (2023). KLF5-mediated aquaporin 3 activated autophagy to facilitate cisplatin resistance of gastric cancer. Immunopharmacol. Immunotoxicol. 45, 140–152. doi:10.1080/08923973.2022.2122498
da Silva I. V., Cardoso C., Martínez-Banaclocha H., Casini A., Pelegrín P., Soveral G. (2021). Aquaporin-3 is involved in NLRP3-inflammasome activation contributing to the setting of inflammatory response. Cell Mol. Life Sci. 78, 3073–3085. doi:10.1007/s00018-020-03708-3
Deng Y., Li M., Mei L., Cong L. M., Liu Y., Zhang B. B., et al. (2018). Manipulation of intestinal dysbiosis by a bacterial mixture ameliorates loperamide-induced constipation in rats. Benef. Microbes 9, 453–464. doi:10.3920/BM2017.0062
Dhiman M., Estrada-Franco J. G., Pando J. M., Ramirez-Aguilar F. J., Spratt H., Vazquez-Corzo S., et al. (2009). Increased myeloperoxidase activity and protein nitration are indicators of inflammation in patients with Chagas' disease. Clin. Vaccine Immunol. 16, 660–666. doi:10.1128/CVI.00019-09
Doar N. W., Samuthiram S. D. (2023). Qualitative analysis of the efficacy of probiotic strains in the prevention of antibiotic-associated diarrhea. Cureus 15, e40261. doi:10.7759/cureus.40261
Dong X., Wang Y., Zhou Y., Wen J., Wang S., Shen L. (2016). Aquaporin 3 facilitates chemoresistance in gastric cancer cells to cisplatin via autophagy. Cell Death Discov. 2, 16087. doi:10.1038/cddiscovery.2016.87
Dong Y., Hou Q., Lei J., Wolf P. G., Ayansola H., Zhang B. (2020). Quercetin alleviates intestinal oxidative damage induced by H2O2 via modulation of GSH: in vitro screening and in vivo evaluation in a colitis model of mice. ACS Omega 5, 8334–8346. doi:10.1021/acsomega.0c00804
Duan T., Cil O., Tse C. M., Sarker R., Lin R., Donowitz M., et al. (2019). Inhibition of CFTR-mediated intestinal chloride secretion as potential therapy for bile acid diarrhea. FASEB J. 33, 10924–10934. doi:10.1096/fj.201901166R
Fan Y., Zhao Q., Wei Y., Wang H., Ga Y., Zhang Y., et al. (2023). Pingwei San ameliorates spleen deficiency-induced diarrhea through intestinal barrier protection and gut microbiota modulation. Antioxidants (Basel). 12, 1122. doi:10.3390/antiox12051122
Forman H. J., Bernardo A., Davies K. J. (2016). What is the concentration of hydrogen peroxide in blood and plasma? Arch. Biochem. Biophys. 603, 48–53. doi:10.1016/j.abb.2016.05.005
Galan-Cobo A., Ramirez-Lorca R., Serna A., Echevarria M. (2015). Overexpression of AQP3 modifies the cell cycle and the proliferation rate of mammalian cells in culture. PLoS One 10, e0137692. doi:10.1371/journal.pone.0137692
Gan Y., Ai G., Wu J., Luo H., Chen L., Huang Q., et al. (2020). Patchouli oil ameliorates 5-fluorouracil-induced intestinal mucositis in rats via protecting intestinal barrier and regulating water transport. J. Ethnopharmacol. 250, 112519. doi:10.1016/j.jep.2019.112519
Gu Y., Qin X., Zhou G., Wang C., Mu C., Liu X., et al. (2022). Lactobacillus rhamnosus GG supernatant promotes intestinal mucin production through regulating 5-HT4R and gut microbiota. Food Funct. 13, 12144–12155. doi:10.1039/d2fo01900k
Guttman J. A., Samji F. N., Li Y., Deng W., Lin A., Finlay B. B., et al. (2007). Aquaporins contribute to diarrhoea caused by attaching and effacing bacterial pathogens. Cell Microbiol. 9, 131–141. doi:10.1111/j.1462-5822.2006.00773.x
Hamabata T., Liu C., Takeda Y. (2002). Positive and negative regulation of water channel aquaporins in human small intestine by cholera toxin. Microb. Pathog. 32, 273–277. doi:10.1006/mpat.2002.0502
Hamed S., Ullmann Y., Egozi D., Keren A., Daod E., Anis O., et al. (2017). Topical erythropoietin treatment accelerates the healing of cutaneous burn wounds in diabetic pigs through an aquaporin-3-dependent mechanism. Diabetes 66, 2254–2265. doi:10.2337/db16-1205
Hara-Chikuma M., Chikuma S., Sugiyama Y., Kabashima K., Verkman A. S., Inoue S., et al. (2012). Chemokine-dependent T cell migration requires aquaporin-3-mediated hydrogen peroxide uptake. J. Exp. Med. 209, 1743–1752. doi:10.1084/jem.20112398
Hara-Chikuma M., Satooka H., Watanabe S., Honda T., Miyachi Y., Watanabe T., et al. (2015). Aquaporin-3-mediated hydrogen peroxide transport is required for NF-κB signalling in keratinocytes and development of psoriasis. Nat. Commun. 6, 7454. doi:10.1038/ncomms8454
Hara-Chikuma M., Verkman A. S. (2008). Prevention of skin tumorigenesis and impairment of epidermal cell proliferation by targeted aquaporin-3 gene disruption. Mol. Cell. Biol. 28, 326–332. doi:10.1128/MCB.01482-07
Hara-Chikuma M., Watanabe S., Satooka H. (2016). Involvement of aquaporin-3 in epidermal growth factor receptor signaling via hydrogen peroxide transport in cancer cells. Biochem. Biophys. Res. Commun. 471, 603–609. doi:10.1016/j.bbrc.2016.02.010
He L., Huang N., Li H., Tian J., Zhou X., Li T., et al. (2017). AMPK/α-Ketoglutarate Axis regulates intestinal water and ion homeostasis in young pigs. J. Agric. Food Chem. 65, 2287–2298. doi:10.1021/acs.jafc.7b00324
Helwa I., Choudhary V., Chen X., Kaddour-Djebbar I., Bollag W. B. (2017). Anti-psoriatic drug monomethylfumarate increases nuclear factor erythroid 2-related factor 2 levels and induces aquaporin-3 mRNA and protein expression. J. Pharmacol. Exp. Ther. 362, 243–253. doi:10.1124/jpet.116.239715
Henzler T., Steudle E. (2000). Transport and metabolic degradation of hydrogen peroxide in Chara corallina: model calculations and measurements with the pressure probe suggest transport of H2O2 across water channels. J. Exp. Bot. 51, 2053–2066. doi:10.1093/jexbot/51.353.2053
Hong Y., Chen Z., Li N., Zhang M. (2020). Prognostic value of serum aquaporin-1, aquaporin-3 and galectin-3 for young patients with colon cancer. Ann. Clin. Biochem. 57, 404–411. doi:10.1177/0004563220961752
Hoogewijs D., Vogler M., Zwenger E., Krull S., Zieseniss A. (2016). Oxygen-dependent regulation of aquaporin-3 expression. Hypoxia (Auckl). 4, 91–97. doi:10.2147/HP.S97681
Hu T. G., Wen P., Fu H. Z., Lin G. Y., Liao S. T., Zou Y. X. (2019). Protective effect of mulberry (Morus atropurpurea) fruit against diphenoxylate-induced constipation in mice through the modulation of gut microbiota. Food Funct. 10, 1513–1528. doi:10.1039/c9fo00132h
Huang Y. C., Yan S. D., Su Z. X., Xia L., Xie J. L., Zhang F., et al. (2022). Aquaporins: a new target for traditional Chinese medicine in the treatment of digestive system diseases. Front. Pharmacol. 13, 1069310. doi:10.3389/fphar.2022.1069310
Ikarashi N., Baba K., Ushiki T., Kon R., Mimura A., Toda T., et al. (2011b). The laxative effect of bisacodyl is attributable to decreased aquaporin-3 expression in the colon induced by increased PGE2 secretion from macrophages. Am. J. Physiol. Gastrointest. Liver Physiol. 301, G887–G895. doi:10.1152/ajpgi.00286.2011
Ikarashi N., Kon R., Iizasa T., Suzuki N., Hiruma R., Suenaga K., et al. (2012a). Inhibition of aquaporin-3 water channel in the colon induces diarrhea. Biol. Pharm. Bull. 35, 957–962. doi:10.1248/bpb.35.957
Ikarashi N., Kon R., Sugiyama K. (2016). Aquaporins in the colon as a new therapeutic target in diarrhea and constipation. Int. J. Mol. Sci. 17, 1172. doi:10.3390/ijms17071172
Ikarashi N., Mimura A., Kon R., Iizasa T., Omodaka M., Nagoya C., et al. (2012b). The concomitant use of an osmotic laxative, magnesium sulphate, and a stimulant laxative, bisacodyl, does not enhance the laxative effect. Eur. J. Pharm. Sci. 45, 73–78. doi:10.1016/j.ejps.2011.10.024
Ikarashi N., Mizukami N., Kon R., Kaneko M., Uchino R., Fujisawa I., et al. (2019a). Study of the mechanism underlying the onset of diabetic xeroderma focusing on an aquaporin-3 in a streptozotocin-induced diabetic mouse model. Int. J. Mol. Sci. 20, 3782. doi:10.3390/ijms20153782
Ikarashi N., Nagoya C., Kon R., Kitaoka S., Kajiwara S., Saito M., et al. (2019b). Changes in the expression of aquaporin-3 in the gastrointestinal tract affect drug absorption. Int. J. Mol.Sci. 20, 1559. doi:10.3390/ijms20071559
Ikarashi N., Ushiki T., Mochizuki T., Toda T., Kudo T., Baba K., et al. (2011a). Effects of magnesium sulphate administration on aquaporin 3 in rat gastrointestinal tract. Biol. Pharm. Bull. 34, 238–242. doi:10.1248/bpb.34.238
Itoh A., Tsujikawa T., Fujiyama Y., Bamba T. (2003). Enhancement of aquaporin-3 by vasoactive intestinal polypeptide in a human colonic epithelial cell line. J. Gastroenterol. Hepatol. 18, 203–210. doi:10.1046/j.1440-1746.2003.02949.x
Jiang B., Li Z., Zhang W., Wang H., Zhi X., Feng J., et al. (2014). miR-874 inhibits cell proliferation, migration and invasion through targeting aquaporin-3 in gastric cancer. J. Gastroenterol. 49, 1011–1025. doi:10.1007/s00535-013-0851-9
Kakihana T., Nagata K., Sitia R. (2012). Peroxides and peroxidases in the endoplasmic reticulum: integrating redox homeostasis and oxidative folding. Antioxid. Redox Signal 16, 763–771. doi:10.1089/ars.2011.4238
Kaya G., Usta D., Sag E., Aydin Z. G., Buruk C. K., Ozkaya E., et al. (2023). Incidence and risk factors for antibiotic-associated diarrhea among hospitalized children. Pediatr. Infect. Dis. J. 42, 745–749. doi:10.1097/INF.0000000000003994
Kim H., Jeong E. J., Park C., Lee J. S., Kim W. J., Yu K. W., et al. (2023). Modulation of gut microbiota ecosystem by a glucan-rich snail mucin heteropolysaccharide attenuates loperamide-induced constipation. Int. J. Biol. Macromol. 126560, 126560. doi:10.1016/j.ijbiomac.2023.126560
Kim K., Song M., Liu Y., Ji P. (2022). Enterotoxigenic Escherichia coli infection of weaned pigs: intestinal challenges and nutritional intervention to enhance disease resistance. Front. Immunol. 13, 885253. doi:10.3389/fimmu.2022.885253
Kon R., Ikarashi N., Hayakawa A., Haga Y., Fueki A., Kusunoki Y., et al. (2015). Morphine-induced constipation develops with increased aquaporin-3 expression in the colon via increased serotonin secretion. Toxicol. Sci. 145, 337–347. doi:10.1093/toxsci/kfv055
Kon R., Ikarashi N., Nagoya C., Takayama T., Kusunoki Y., Ishii M., et al. (2014). Rheinanthrone, a metabolite of sennoside A, triggers macrophage activation to decrease aquaporin-3 expression in the colon, causing the laxative effect of rhubarb extract. J. Ethnopharmacol. 152, 190–200. doi:10.1016/j.jep.2013.12.055
Kon R., Ikarashi N., Onuma K., Yasukawa Z., Ozeki M., Sakai H., et al. (2022). Effect of partially hydrolyzed guar gum on the expression of aquaporin-3 in the colon. Food Sci. Nutr. 11, 1127–1133. doi:10.1002/fsn3.3150
Kon R., Tsubota Y., Minami M., Kato S., Matsunaga Y., Kimura H., et al. (2018a). CPT-11-induced delayed diarrhea develops via reduced aquaporin-3 expression in the colon. Int. J. Mol. Sci. 19, 170. doi:10.3390/ijms19010170
Kon R., Yamamura M., Matsunaga Y., Kimura H., Minami M., Kato S., et al. (2018b). Laxative effect of repeated Daiokanzoto is attributable to decrease in aquaporin-3 expression in the colon. J. Nat. Med. 72, 493–502. doi:10.1007/s11418-018-1174-1
Koyama Y., Yamamoto T., Tani T., Nihei K., Kondo D., Funaki H., et al. (1999). Expression and localization of aquaporins in rat gastrointestinal tract. Am. J. Physiol. 276, C621–C627. doi:10.1152/ajpcell.1999.276.3.C621
Krone K. J. E., Agyekum A. K., Ter Borgh M., Hamonic K., Penner G. B., Columbus D. A. (2019). Characterization of urea transport mechanisms in the intestinal tract of growing pigs. Am. J. Physiol. Gastrointest. Liver Physiol. 317, G839-G844–G844. doi:10.1152/ajpgi.00220.2019
Krüger C., Waldeck-Weiermair M., Kaynert J., Pokrant T., Komaragiri Y., Otto O., et al. (2021). AQP8 is a crucial H2O2 transporter in insulin-producing RINm5F cells. Redox Biol. 43, 101962. doi:10.1016/j.redox.2021.101962
Laforenza U. (2012). Water channel proteins in the gastrointestinal tract. Mol. Asp. Med. 33, 642–650. doi:10.1016/j.mam.2012.03.001
Laforenza U., Cova E., Gastaldi G., Tritto S., Grazioli M., LaRusso N. F., et al. (2005). Aquaporin-8 is involved in water transport in isolated superficial colonocytes from rat proximal colon. J. Nutr. 135, 2329–2336. doi:10.1093/jn/135.10.2329
Laforenza U., Miceli E., Gastaldi G., Scaffino M. F., Ventura U., Fontana J. M., et al. (2010). Solute transporters and aquaporins are impaired in celiac disease. Biol. Cell 102, 457–467. doi:10.1042/BC20100023
Laforenza U., Pellavio G., Marchetti A. L., Omes C., Todaro F., Gastaldi G. (2016). Aquaporin-mediated water and hydrogen peroxide transport is involved in normal human spermatozoa functioning. Int. J. Mol. Sci. 18, 66. doi:10.3390/ijms18010066
Legan T. B., Lavoie B., Norberg E., Ley I. C., Tack S., Tompkins T. A., et al. (2023). Tryptophan-synthesizing bacteria enhance colonic motility. Neurogastroenterol. Motil. 35, e14629. doi:10.1111/nmo.14629
Li A., Lu D., Zhang Y., Li J., Fang Y., Li F., et al. (2013). Critical role of aquaporin-3 in epidermal growth factor-induced migration of colorectal carcinoma cells and its clinical significance. Oncol. Rep. 29, 535–540. doi:10.3892/or.2012.2144
Li F. X., Huang L. Z., Dong C., Wang J. P., Wu H. J., Shuang S. M. (2015). Down-regulation of aquaporin3 expression by lipopolysaccharide via p38/c-Jun N-terminal kinase signalling pathway in HT-29 human colon epithelial cells. World J. Gastroenterol. 21, 4547–4554. doi:10.3748/wjg.v21.i15.4547
Li J., Sun Y., Wang R., Ma S., Shi L., Wang K., et al. (2023a). Seasonal differences in intestinal flora are related to rats' intestinal water metabolism. Front. Microbiol. 14, 1109696. doi:10.3389/fmicb.2023.1109696
Li J. X., Tian R., Lu N. (2023b). Quercetin attenuates vascular endothelial dysfunction in atherosclerotic mice by inhibiting myeloperoxidase and NADPH oxidase function. Chem. Res. Toxicol. 36, 260–269. doi:10.1021/acs.chemrestox.2c00334
Li W., Zhang S., Wang Y., Bian H., Yu S., Huang L., et al. (2023c). Complex probiotics alleviate ampicillin-induced antibiotic-associated diarrhea in mice. Front. Microbiol. 14, 1156058. doi:10.3389/fmicb.2023.1156058
Li Z., Li B., Zhang L., Chen L., Sun G., Zhang Q., et al. (2016). The proliferation impairment induced by AQP3 deficiency is the result of glycerol uptake and metabolism inhibition in gastric cancer cells. Tumour Biol. 37, 9169–9179. doi:10.1007/s13277-015-4753-8
Li Z., Li X., Tang S., Gao Q., Li C., Chen P., et al. (2023d). Moringa oleifera Lam. Leaf improves constipation of rats induced by low-fiber-diet: a proteomics study. J. Ethnopharmacol. 318, 116936. doi:10.1016/j.jep.2023.116936
Liang X., Wan D., Cai Y., Yue W., Wang X., Zhou H., et al. (2023). Xuanhuang Runtong tablets relieve slow transit constipation in mice by regulating TLR5/IL-17A signaling mediated by gut microbes. Evid. Based Complement. Altern. Med. 2023, 6506244. doi:10.1155/2023/6506244
Lin C., He H., Kim J. J., Zheng X., Huang Z., Dai N. (2023a). Osmotic pressure induces translocation of aquaporin-8 by P38 and JNK MAPK signaling pathways in patients with functional constipation. Dig. Liver Dis. S1590-8658, 1049–1059. doi:10.1016/j.dld.2023.01.162
Lin P., Shi H. Y., Lu Y. Y., Lin J. (2023b). Centella asiatica alleviates psoriasis through JAK/STAT3-mediated inflammation: an in vitro and in vivo study. J. Ethnopharmacol. 317, 116746. doi:10.1016/j.jep.2023.116746
Lin X., Fang Y., Cheng Y., Wang Q. (2023c). Chinese herbal medicine for irinotecan-induced diarrhea: a systematic review and meta-analysis. Explore (NY) S1550-8307 (23), 00174–X. doi:10.1016/j.explore.2023.08.003
Lismont C., Koster J., Provost S., Baes M., Van Veldhoven P. P., Waterham H. R., et al. (2019). Deciphering the potential involvement of PXMP2 and PEX11B in hydrogen peroxide permeation across the peroxisomal membrane reveals a role for PEX11B in protein sorting. Biochim. Biophys. Acta Biomembr. 1861, 182991. doi:10.1016/j.bbamem.2019.05.013
Liu C., Song C., Wang Y., Xiao Y., Zhou Z., Cao G., et al. (2023). Deep-fried Atractylodes lancea rhizome alleviates spleen deficiency diarrhea-induced short-chain fatty acid metabolic disorder in mice by remodeling the intestinal flora. J. Ethnopharmacol. 303, 115967. doi:10.1016/j.jep.2022.115967
Liu C., Zheng Y., Xu W., Wang H., Lin N. (2014). Rhubarb tannins extract inhibits the expression of aquaporins 2 and 3 in magnesium sulphate-induced diarrhoea model. Res. Int. 2014, 619465. doi:10.1155/2014/619465
Longstreth G. F., Thompson W. G., Chey W. D., Houghton L. A., Mearin F., Spiller R. C. (2006). Functional bowel disorders. Gastroenterology 130, 1480–1491. doi:10.1053/j.gastro.2005.11.061
Luo J., Liu X., Liu J., Jiang M., Luo M., Zhao J. (2016). Activation of TGF-β1 by AQP3-mediated H2O2 transport into fibroblasts of a bleomycin-induced mouse model of scleroderma. J. Invest. Dermatol. 136, 2372–2379. doi:10.1016/j.jid.2016.07.014
Luo X., Wu S., Jia H., Si X., Song Z., Zhai Z., et al. (2022). Resveratrol alleviates enterotoxigenic Escherichia coli K88-induced damage by regulating SIRT-1 signaling in intestinal porcine epithelial cells. Food Funct. 13, 7346–7360. doi:10.1039/d1fo03854k
Lv H., Li Y., Xue C., Dong N., Bi C., Shan A. (2022). Aquaporin: targets for dietary nutrients to regulate intestinal health. J. Anim. Physiol. Anim. Nutr. Berl. 106, 167–180. doi:10.1111/jpn.13539
Lv Y., Li X., Zhang L., Shi Y., Du L., Ding B., et al. (2018). Injury and mechanism of recombinant E. coli expressing STa on piglets colon. J. Vet. Med. Sci. 80, 205–212. doi:10.1292/jvms.17-0528
Ma T., Verkman A. S. (1999). Aquaporin water channels in gastrointestinal physiology. J. Physiol. 517, 317–326. doi:10.1111/j.1469-7793.1999.0317t.x
Ma X., Pan Y., Zhao W., Sun P., Zhao J., Yan S., et al. (2022). Bifidobacterium infantis strain YLGB-1496 possesses excellent antioxidant and skin barrier-enhancing efficacy in vitro. Exp. Dermatol 31, 1089–1094. doi:10.1111/exd.14583
Ma Y., Zhang J., Li Y., Hu H., Ye Q., Yang C., et al. (2023). Aquaporin-7 facilitates proliferation and adipogenic differentiation of mouse bone marrow mesenchymal stem cells by regulating hydrogen peroxide transport. Stem Cell Rev. Rep. 19, 2378–2390. doi:10.1007/s12015-023-10588-0
Martinotti S., Laforenza U., Patrone M., Moccia F., Ranzato E. (2019). Honey-mediated wound healing: H2O2 entry through AQP3 determines extracellular Ca2+ influx. Int. J. Mol. Sci. 20, 764. doi:10.3390/ijms20030764
Masyuk A. I., Marinelli R. A., LaRusso N. F. (2002). Water transport by epithelia of the digestive tract. Gastroenterology 122, 545–562. doi:10.1053/gast.2002.31035
Mawe G. M., Hoffman J. M. (2013). Serotonin signalling in the gut--functions, dysfunctions and therapeutic targets. Nat. Rev. Gastroenterol. Hepatol. 10, 473–486. doi:10.1038/nrgastro.2013.105
Mawe G. M., Hurd M., Hennig G. W., Lavoie B. (2022). Epithelial 5-HT4 receptors as a target for treating constipation and intestinal inflammation. Adv. Exp. Med. Biol. 1383, 329–334. doi:10.1007/978-3-031-05843-1_30
Mazhar N., Mohtashem U. l. H. M., Baig S. G., Ahmed S., Jaffery R., Ikram R. (2022). Diuretic and anti-diarrheal potential of four fruit extracts of Capsicum annum L. Pak J. Pharm. Sci. 35, 1357–1362.
Medraño-Fernandez I., Bestetti S., Bertolotti M., Bienert G. P., Bottino C., Laforenza U., et al. (2016). Stress regulates aquaporin-8 permeability to impact cell growth and survival. Antioxid. Redox Signal 24, 1031–1044. doi:10.1089/ars.2016.6636
Mego M., Danis R., Chovanec J., Jurisova S., Bystricky B., Porsok S., et al. (2023). Randomized double-blind, placebo-controlled multicenter phase III study of prevention of irinotecan-induced diarrhea by a probiotic mixture containing Bifidobacterium BB-12® Lactobacillus rhamnosus LGG® in colorectal cancer patients. Front. Oncol. 13, 1168654. doi:10.3389/fonc.2023.1168654
Mehta M., Beg M. (2018). Fructose intolerance: cause or cure of chronic functional constipation. Pediatr. Health. 5, 2333794X18761460. doi:10.1177/2333794X18761460
Meli R., Pirozzi C., Pelagalli A. (2018). New perspectives on the potential role of aquaporins (AQPs) in the physiology of inflammation. Front. Physiol. 9, 101. doi:10.3389/fphys.2018.00101
Michael M., Brittain M., Nagai J., Feld R., Hedley D., Oza A., et al. (2004). Phase II study of activated charcoal to prevent irinotecan-induced diarrhea. J. Clin. Oncol. 22, 4410–4417. doi:10.1200/JCO.2004.11.125
Miller E. W., Dickinson B. C., Chang C. J. (2010). Aquaporin-3 mediates hydrogen peroxide uptake to regulate downstream intracellular signaling. Proc. Natl. Acad. Sci.USA. 107, 15681–15686. doi:10.1073/pnas.1005776107
Mobasheri A., Wray S., Marples D. (2005). Distribution of AQP2 and AQP3 water channels in human tissue microarrays. J. Mol. Histol. 36, 1–14. doi:10.1007/s10735-004-2633-4
Mollajew R., Zocher F., Horner A., Wiesner B., Klussmann E., Pohl P. (2010). Routes of epithelial water flow: aquaporins versus cotransporters. Biophys. J. 99, 3647–3656. doi:10.1016/j.bpj.2010.10.021
Na J. R., Kim E., Na C. S., Kim S. (2022). Citric acid-enriched extract of ripe Prunus mume (siebold) siebold and zucc. Induces laxative effects by regulating the expression of aquaporin 3 and prostaglandin E2 in rats with loperamide-induced constipation. J. Med. Food. 25, 12–23. doi:10.1089/jmf.2021.K.0138
Nalle S. C., Barreira Da Silva R., Zhang H., Decker M., Chalouni C., Xu M., et al. (2020). Aquaporin-3 regulates endosome-to-cytosol transfer via lipid peroxidation for cross presentation. PLoS One 15, e0238484. doi:10.1371/journal.pone.0238484
Nong Y. Q., Li S. F., Liu W. J., Zhang X. Q., Fan L., Chen Y., et al. (2021). Aquaporin 3 promotes human extravillous trophoblast migration and invasion. Reprod. Biol. Endocrinol. 19, 49. doi:10.1186/s12958-021-00726-z
Pan X., Xu K., Xiong R., Chen Z. M., Hu C. J., Feng J., et al. (2019). Therapeutic effect of Ershen pill (二神丸) extract on Pi (spleen)-Shen (kidney) Yang deficiency-induced diarrhea in rat model. Chin. J. Integr. Med. 25, 536–542. doi:10.1007/s11655-017-2766-4
Pellavio G., Martinotti S., Patrone M., Ranzato E., Laforenza U. (2022). Aquaporin-6 may increase the resistance to oxidative stress of malignant pleural mesothelioma cells. Cells 11, 1892. doi:10.3390/cells11121892
Pellavio G., Rui M., Caliogna L., Martino E., Gastaldi G., Collina S., et al. (2017). Regulation of aquaporin functional properties mediated by the antioxidant effects of natural compounds. Int. J. Mol. Sci. 18, 2665. doi:10.3390/ijms18122665
Peng P., Deng D., Chen S., Li C., Luo J., Romeo A., et al. (2020). The effects of dietary porous zinc oxide supplementation on growth performance, inflammatory cytokines and tight junction's gene expression in early-weaned piglets. J. Nutr. Sci. Vitaminol. (Tokyo). 66, 311–318. doi:10.3177/jnsv.66.311
Peplowski M. A., Dicay M., Baggio C. H., Wysokinski F., Renaux B., Hollenberg M. D., et al. (2018). Interferon gamma decreases intestinal epithelial aquaporin 3 expression through downregulation of constitutive transcription. J. Mol. Med. Berl. 96, 1081–1093. doi:10.1007/s00109-018-1681-2
Peplowski M. A., Vegso A. J., Iablokov V., Dicay M., Zaheer R. S., Renaux B., et al. (2017). Tumor necrosis factor α decreases aquaporin 3 expression in intestinal epithelial cells through inhibition of constitutive transcription. Physiol. Rep. 5, e13451. doi:10.14814/phy2.13451
Qiu B., Zhu L., Zhang S., Han S., Fei Y., Ba F., et al. (2022). Prevention of loperamide-induced constipation in mice and alteration of 5-hydroxytryotamine signaling by Ligilactobacillus salivarius Li01. Nutrients 14, 4083. doi:10.3390/nu14194083
Radziszewska M., Smarkusz-Zarzecka J., Ostrowska L. (2023). Nutrition, physical activity and supplementation in irritable bowel syndrome. Nutrients 15, 3662. doi:10.3390/nu15163662
Ramírez-Lorca R., Vizuete M. L., Venero J. L., Revuelta M., Cano J., Ilundáin A. A., et al. (1999). Localization of aquaporin-3 mRNA and protein along the gastrointestinal tract of Wistar rats. Pflugers Arch. 438, 94–100. doi:10.1007/s004240050884
Ramos C. I., Andrade de Lima A. F., Grilli D. G., Cuppari L. (2015). The short-term effects of olive oil and flaxseed oil for the treatment of constipation in hemodialysis patients. J. Ren. Nutr. 25, 50–56. doi:10.1053/j.jrn.2014.07.009
Ren J., Zeng W., Jiang C., Li C., Zhang C., Cao H., et al. (2022). Inhibition of porcine epidemic diarrhea virus by cinchonine via inducing cellular autophagy. Front. Cell Infect. Microbiol. 12, 856711. doi:10.3389/fcimb.2022.856711
Ricanek P., Lunde L. K., Frye S. A., Stoen M., Nygard S., Morth J. P., et al. (2015). Reduced expression of aquaporins in human intestinal mucosa in early stage inflammatory bowel disease. Clin. Exp. Gastroenterol. 8, 49–67. doi:10.2147/CEG.S70119
Rodrigues C., Pimpão C., Mósca A. F., Coxixo A. S., Lopes D., da Silva I. V., et al. (2019). Human aquaporin-5 facilitates hydrogen peroxide permeation affecting adaption to oxidative stress and cancer cell migration. Cancers (Basel). 11, 932. doi:10.3390/cancers11070932
Saha L. (2014). Irritable bowel syndrome: pathogenesis, diagnosis, treatment, and evidence-based medicine. World J. Gastroenterol. 20, 6759–6773. doi:10.3748/wjg.v20.i22.6759
Sakulpanich A., Gritsanapan W. (2009). Laxative anthraquinone contents in fresh and cooked Senna siamea leaves. Southeast Asian J. Trop. Med. Public Health. 40, 835–839.
Satooka H., Hara-Chikuma M. (2016). Aquaporin-3 controls breast cancer cell migration by regulating hydrogen peroxide transport and its downstream cell signaling. Mol. Cell Biol. 36, 1206–1218. doi:10.1128/MCB.00971-15
Shi K., Qu L. H., Lin X., Xie Y., Tu J. Y., Liu X. Q., et al. (2019). Deep-fried Atractylodis Rhizoma protects against spleen deficiency-induced diarrhea through regulating intestinal inflammatory response and gut microbiota. Int. J. Mol. Sci. 21, 124. doi:10.3390/ijms21010124
Sies H. (2017). Hydrogen peroxide as a central redox signaling molecule in physiological oxidative stress: oxidative eustress. Redox Biol. 11, 613–619. doi:10.1016/j.redox.2016.12.035
Sies H., Chance B. (1970). The steady state level of catalase compound I in isolated hemoglobin-free perfused rat liver. FEBS Lett. 11, 172–176. doi:10.1016/0014-5793(70)80521-x
Silberstein C., Kierbel A., Amodeo G., Zotta E., Bigi F., Berkowski D., et al. (1999). Functional characterization and localization of AQP3 in the human colon. Braz. J. Med. Biol. Res. 32, 1303–1313. doi:10.1590/s0100-879x1999001000018
Sisto M., Ribatti D., Lisi S. (2019). Aquaporin water channels: new perspectives on the potential role in inflammation. Adv. Protein Chem. Struct. Biol. 116, 311–345. doi:10.1016/bs.apcsb.2018.11.010
Su Z. R., Zhi X. F., Zhang Q., Yang L., Xu H., Xu Z. K. (2016). LncRNA H19 functions as a competing endogenous RNA to regulate AQP3 expression by sponging miR-874 in the intestinal barrier. FEBS Lett. 590, 1354–1364. doi:10.1002/1873-3468.12171
Sun L. L., Jiang H. B., Liu B. Y., Li W. D., Du A. L., Luo X. Q., et al. (2018). Effects of rhein on intestinal transmission, colonic electromyography and expression of aquaporin-3 by colonic epithelium cells in constipated mice. Int. J. Clin. Exp. Pathol. 11, 614–623.
Tan Q., Hu J., Zhou Y. J., Wan Y. X., Zhang C. L., Liu X., et al. (2021). Inhibitory Effect of Lactococcus lactis subsp. lactis HFY14 on diphenoxylate-induced constipation in mice by regulating the VIP-cAMP-PKA-AQP3 signaling pathway. Drug Des. devel. Ther. 15, 1971–1980. doi:10.2147/DDDT.S309675
Tang Y. L., Li F., Tan B., Liu G., Kong X. F., Hardwidge P. R., et al. (2014). Enterotoxigenic Escherichia coli infection induces intestinal epithelial cell autophagy. Vet. Microbiol. 171, 160–164. doi:10.1016/j.vetmic.2014.03.025
Teng W. L., Huang P. H., Wang H. C., Tseng C. H., Yen F. L. (2021). Pterostilbene attenuates particulate matter-induced oxidative stress, inflammation and aging in keratinocytes. Antioxidants (Basel). 10, 1552. doi:10.3390/antiox10101552
Thiagarajah J. R., Chang J., Goettel J. A., Verkman A. S., Lencer W. I. (2017). Aquaporin-3 mediates hydrogen peroxide-dependent responses to environmental stress in colonic epithelia. Proc. Natl. Acad. Sci. USA. 114, 568–573. doi:10.1073/pnas.1612921114
Thiagarajah J. R., Zhao D., Verkman A. S. (2007). Impaired enterocyte proliferation in aquaporin-3 deficiency in mouse models of colitis. Gut 56, 1529–1535. doi:10.1136/gut.2006.104620
Tsujikawa T., Itoh A., Fukunaga T., Satoh J., Yasuoka T., Fujiyama Y. (2003). Alteration of aquaporin mRNA expression after small bowel resection in the rat residual ileum and colon. J. Gastroenterol. Hepatol. 18, 803–808. doi:10.1046/j.1440-1746.2003.03033.x
Turvill J. L., Farthing M. J. (1999). Water and electrolyte absorption and secretion in the small intestine. Curr. Opin. Gastroenterol. 15, 108–112. doi:10.1097/00001574-199903000-00004
Wang H. F., Bi Z. B., Dai K. Y., Li P. H., Huang R. H., Wu S. L., et al. (2022). A functional variant in the aquaporin-3 promoter modulates its expression and correlates with resistance to porcine epidemic virus infection in porcine intestinal epithelial cells. Front. Microbiol. 13, 877644. doi:10.3389/fmicb.2022.877644
Wang J. Y., Liu W., Zhang X. Y., Zhang Y., Xiao H., Luo B. (2019). LMP2A induces DNA methylation and expression repression of AQP3 in EBV-associated gastric carcinoma. Virology 534, 87–95. doi:10.1016/j.virol.2019.06.006
Wang K. L., Liu L., Huang J. Y., Yu H. L., Wu H., Duan Y., et al. (2017). Laxative effects of total diterpenoids extracted from the roots of Euphorbia pekinensis are attributable to alterations of aquaporins in the colon. Molecules 22, 465. doi:10.3390/molecules22030465
Wang R., Lu X., Zhao L., Zhang W., Zhang S. (2023a). Houpo paiqi mixture promotes intestinal motility in constipated rats by modulating gut microbiota and activating 5-HT-cAMP-PKA signal pathway. J. Appl. Microbiol. 134, lxad153. doi:10.1093/jambio/lxad153
Wang Y. H., Liu T. T., Kung W. M., Chen C. C., Wen Y. T., Lin I. C., et al. (2015). Expression of aquaporins in intestine after heat stroke. Int. J. Clin. Exp. Pathol. 8, 8742–8753.
Wang Y. J., Jiang H., Wang L. J., Gan H. P., Xiao X. C., Huang L. W., et al. (2023b). Luteolin ameliorates loperamide-induced functional constipation in mice. Braz. J. Med. Biol. Res. 56, e12466. doi:10.1590/1414-431X2023e12466
Wang Y. W., Chen D., Liu Y., Zhang Y., Duan C., Otkur W. X., et al. (2021). AQP3-mediated H2O2 uptake inhibits LUAD autophagy by inactivating PTEN. Cancer Sci. 112, 3278–3292. doi:10.1111/cas.15008
Wei Y., Huang N., Ye X. Y., Liu M., Wei M. L., Huang Y. L. (2023). The postbiotic of hawthorn-probiotic ameliorating constipation caused by loperamide in elderly mice by regulating intestinal microecology. Front. Nutr. 10, 1103463. doi:10.3389/fnut.2023.1103463
Wilder-Smith C. H., Materna A., Wermelinger C., Schuler J. (2013). Fructose and lactose intolerance and malabsorption testing: the relationship with symptoms in functional gastrointestinal disorders. Aliment. Pharmacol. Ther. 37, 1074–1083. doi:10.1111/apt.12306
Wragg D., Leoni S., Casini A. (2020). Aquaporin-driven hydrogen peroxide transport: a case of molecular mimicry? RSC Chem. Biol. 1, 390–394. doi:10.1039/d0cb00160k
Wright E. M., Loo D. D. (2000). Coupling between Na+, sugar, and water transport across the intestine. Ann. N. Y. Acad. Sci. 915, 54–66. doi:10.1111/j.1749-6632.2000.tb05223.x
Wu J. Y., Wang F., Wu Z. C., Wu S. L., Bao W. B. (2020). Regulatory effect of methylation of the porcine AQP3 gene promoter region on its expression level and porcine epidemic diarrhea virus resistance. Genes (Basel). 11, 1167. doi:10.3390/genes11101167
Wu J. Z., Gan Y. X., Luo H. J., Xu N., Chen L. P., Li M. Y., et al. (2021). β-patchoulene ameliorates water transport and the mucus barrier in 5-fluorouracil-induced intestinal mucositis rats via the cAMP/PKA/CREB signaling pathway. Front. Pharmacol. 12, 689491. doi:10.3389/fphar.2021.689491
Wu X., Su D. (2018). Enterotoxigenic Escherichia coli infection induces tight junction proteins expression in mice. J. Vet. Res. 19, 35–40.
Wu Z. W., Uchi H., Morino-Koga S., Shi W. M., Furue M. (2014). Resveratrol inhibition of human keratinocyte proliferation via SIRT1/ARNT/ERK dependent downregulation of aquaporin 3. J. Dermatol. Sci. 75, 16–23. doi:10.1016/j.jdermsci.2014.03.004
Xia Y. Y., Chen S. Y., Zhao Y. Y., Chen S., Huang R. L., Zhu G. Q., et al. (2019). GABA attenuates ETEC-induced intestinal epithelial cell apoptosis involving GABAAR signaling and the AMPK-autophagy pathway. Food Funct. 10, 7509–7522. doi:10.1039/c9fo01863h
Xie Y., Zhan X., Tu J. Y., Xu K., Sun X. J., Liu C. L., et al. (2021). Atractylodes oil alleviates diarrhea-predominant irritable bowel syndrome by regulating intestinal inflammation and intestinal barrier via SCF/c-kit and MLCK/MLC2 pathways. J. Ethnopharmacol. 272, 113925. doi:10.1016/j.jep.2021.113925
Xu Y. C., Yao H., Wang Q. Y., Xu W. B., Liu K. H., Zhang J. B., et al. (2018). Aquaporin-3 attenuates oxidative stress-induced nucleus pulposus cell apoptosis through regulating the p38 MAPK pathway. Cell Physiol. biochem. 50, 1687–1697. doi:10.1159/000494788
Yang Q., Hu Z., Lei Y., Li X., Xu C., Zhang J., et al. (2023). Overview of systematic reviews of probiotics in the prevention and treatment of antibiotic-associated diarrhea in children. Front. Pharmacol. 14, 1153070. doi:10.3389/fphar.2023.1153070
Yde J., Keely S., Wu Q., Borg J. F., Lajczak N., O'dwyer A., et al. (2016). Characterization of AQPs in mouse, rat, and human colon and their selective regulation by bile acids. Front. Nutr. 3, 46. doi:10.3389/fnut.2016.00046
Ye Y., Ran J., Yang B., Mei Z. (2023). Aquaporins in digestive system. Adv. Exp. Med. Biol. 1398, 145–154. doi:10.1007/978-981-19-7415-1_10
Yi D., Li B. C., Hou Y. Q., Wang L., Zhao D., Chen H. B., et al. (2018). Dietary supplementation with an amino acid blend enhances intestinal function in piglets. Amino Acids 50, 1089–1100. doi:10.1007/s00726-018-2586-7
Yi R. K., Tian Y., Tan F., Li W. F., Mu J. F., Long X. Y., et al. (2020). Intervention effect of Malus pumila leaf flavonoids on senna-induced acute diarrhea in BALB/c mice. Food. Sci. Nutr. 8, 2535–2542. doi:10.1002/fsn3.1549
Yin J. Q., Liang Y. C., Wang D., Yan Z. P., Yin H. H., Wu D., et al. (2018). Naringenin induces laxative effects by upregulating the expression levels of c-Kit and SCF, as well as those of aquaporin 3 in mice with loperamide-induced constipation. Int. J. Mol. Med. 41, 649–658. doi:10.3892/ijmm.2017.3301
Yu C. X., Zhang X., Wang J. Y., Song H., Liu W., Luo B. (2022). Molecular mechanism of aquaporin 3 (AQP3) regulating by LMP2A and its crosstalk with 4E-BP1 via ERK signaling pathway in EBV-associated gastric cancer. Virus Res. 322, 198947. doi:10.1016/j.virusres.2022.198947
Yu H. L., Liu L., Wang K. L., Wu H., Wang W., Zhang X. D., et al. (2018). Upregulation of aquaporin 3 expression by diterpenoids in Euphorbia pekinensis is associated with activation of the NF-κB signaling pathway in the co-culture system of HT-29 and RAW 264.7 cells. Cells. Biochim. 144, 153–159. doi:10.1016/j.biochi.2017.11.006
Yu S., Li L. H., Lee C. H., Jeyakannu P., Wang J. J., Hong C. H. (2021). Arsenic leads to autophagy of keratinocytes by increasing aquaporin 3 expression. Sci. Rep. 11, 17523. doi:10.1038/s41598-021-96822-6
Yuan W. T., Yang H. F., Zhang Z. Y., Liu J. B. (2008). Expression and significance of aquaporin 3 and aquaporin 9 in colonic mucosa of patients with functional constipation. Chin. J. Gastrointest. Surg. 11, 57–60. doi:10.3760/cma.j.issn.1671-0274.2008.01.016
Yue B., Gao R., Lv C., Yu Z., Wang H., Geng X., et al. (2021). Berberine improves irinotecan-induced intestinal mucositis without impairing the anti-colorectal cancer efficacy of irinotecan by inhibiting bacterial β-glucuronidase. Front. Pharmacol. 12, 774560. doi:10.3389/fphar.2021.774560
Yue Y., He Z. J., Zhou Y. H., Ross R. P., Stanton C., Zhao J. X., et al. (2020). Lactobacillus plantarum relieves diarrhea caused by enterotoxin-producing Escherichia coli through inflammation modulation and gut microbiota regulation. Food Funct. 11, 10362–10374. doi:10.1039/d0fo02670k
Zeuthen T. (2010). Water-transporting proteins. J. Membr. Biol. 234, 57–73. doi:10.1007/s00232-009-9216-y
Zhan Y., Tang X. G., Xu H., Tang S. Y. (2020). Maren pills improve constipation via regulating AQP3 and NF-κB signaling pathway in slow transit constipation in vitro and in vivo. Evid. Based Complement. Altern. Med. 2020, 9837384. doi:10.1155/2020/9837384
Zhang B. P., Wei X. H., Ding M. Z., Luo Z. Y., Tan X. M., Zheng Z. Z. (2022a). Daidzein protects Caco-2 cells against lipopolysaccharide-induced intestinal epithelial barrier injury by suppressing PI3K/AKT and p38 pathways. Molecules 27, 8928. doi:10.3390/molecules27248928
Zhang D., Zhang K. Q., Su W. H., Zhao Y., Ma X., Qian G., et al. (2017). Aquaporin-3 is down-regulated in jejunum villi epithelial cells during enterotoxigenic Escherichia coli-induced diarrhea in mice. Microb. Pathog. 107, 430–435. doi:10.1016/j.micpath.2017.04.031
Zhang J. P., Li S. W., Deng F., Baikeli B., Yu W. J., Liu G. Q. (2019). Distribution of aquaporins and sodium transporters in the gastrointestinal tract of a desert hare, Lepus yarkandensis. Sci. Rep. 9, 16639. doi:10.1038/s41598-019-53291-2
Zhang M. M., Gong Z. C., Zhao Q., Xu D. Q., Fu R. J., Tang Y. P., et al. (2023). Time-dependent laxative effect of sennoside A, the core functional component of rhubarb, is attributed to gut microbiota and aquaporins. J. Ethnopharmacol. 311, 116431. doi:10.1016/j.jep.2023.116431
Zhang W., Zhu B., Xu J., Liu Y., Qiu E., Li Z., et al. (2018). Bacteroides fragilis protects against antibiotic-associated diarrhea in rats by modulating intestinal defenses. Front. Immunol. 9, 1040. doi:10.3389/fimmu.2018.01040
Zhang W. J., Xu Y., Chen Z., Xu Z. K., Xu H. (2011). Knockdown of aquaporin 3 is involved in intestinal barrier integrity impairment. FEBS Lett. 585, 3113–3119. doi:10.1016/j.febslet.2011.08.045
Zhang Y., Tan P., Zhao Y., Ma X. (2022b). Enterotoxigenic Escherichia coli: intestinal pathogenesis mechanisms and colonization resistance by gut microbiota. Gut Microbes 214, 2055943. doi:10.1080/19490976.2022.2055943
Zhang Z. T., Zhao C., Zhang R. J., Wang Y. Y., Hu Y. Z., Zhou Q., et al. (2022c). Overexpression of Aquaporin-3 alleviates hyperosmolarity-induced nucleus pulposus cell apoptosis via regulating the ERK1/2 pathway. Pain. Res. Manag. 2022, 1639560. doi:10.1155/2022/1639560
Zhao G. X., Dong P. P., Peng R., Li J., Zhang D. Y., Wang J. Y., et al. (2016). Expression, localization and possible functions of aquaporins 3 and 8 in rat digestive system. Biotech. Histochem. 91, 269–276. doi:10.3109/10520295.2016.1144079
Zhao G. X., Li J., Wang J. Y., Shen X. Z., Sun J. Y. (2014). Aquaporin 3 and 8 are down-regulated in TNBS-induced rat colitis. Biochem. Biophys. Res. Commun. 443, 161–166. doi:10.1016/j.bbrc.2013.11.067
Zhao L., Zhang Y., Wang Y., Qiao H., Wang Y., Ren J., et al. (2023). Gut microbiota diversity of hospitalized older adult patients with and without antibiotic-associated diarrhea. Aging Clin. Exp. Res. 35, 1541–1555. doi:10.1007/s40520-023-02436-5
Zheng Y. F., Liu C. F., Lai W. F., Xiang Q., Li Z. F., Wang H., et al. (2014). The laxative effect of emodin is attributable to increased aquaporin 3 expression in the colon of mice and HT-29 cells. Fitoterapia 96, 25–32. doi:10.1016/j.fitote.2014.04.002
Zhi X. F., Tao J. Q., Li Z. L., Jiang B. F., Feng J., Yang L., et al. (2014). MiR-874 promotes intestinal barrier dysfunction through targeting AQP3 following intestinal ischemic injury. FEBS Lett. 588, 757–763. doi:10.1016/j.febslet.2014.01.022
Zhong L., Cao X., Li L., He Y., Liu Y., Chen W., et al. (2023). Renzhu ointment regulates L-type voltage-dependent calcium channel in mice Model of senna-induced diarrhea by transdermal administration. Drug Des. Devel Ther. 17, 2355–2368. doi:10.2147/DDDT.S419626
Zhu C., Chen Z., Jiang Z. Y. (2016). Expression, distribution and role of aquaporin water channels in human and animal stomach and intestines. Int. J. Mol. Sci. 17, 1399. doi:10.3390/ijms17091399
Zhu C., Le M. F., He Z. T., Bai Y. S., Yang J., Ye J. L., et al. (2023). Dietary berberine supplementation improves growth performance and alleviates gut injury in weaned piglets by modulating ileal microbiota and metabolites. Food Funct. 14, 4143–4162. doi:10.1039/d3fo01044a
Zhu C., Ye J. L., Yang J., Yang K. M., Chen Z., Liang R., et al. (2017a). Differential expression of intestinal ion transporters and water channel aquaporins in young piglets challenged with enterotoxigenic Escherichia coli K88. J. Anim. Sci. 95, 5240–5252. doi:10.2527/jas2017.1806
Zhu D. R., Chen C., Bai L. J., Kong L. Y., Luo J. G. (2019a). Downregulation of aquaporin 3 mediated the laxative effect in the rat colon by a purified resin glycoside fraction from pharbitis semen. Evid. Based Complement. Altern. Med. 2019, 9406342. doi:10.1155/2019/9406342
Zhu H. Y., Wu Y. L., Kang M. X., Zhang B. (2020). MiR-877 suppresses gastric cancer progression by downregulating AQP3. J. Int. Med. Res. 48, 300060520903661. doi:10.1177/0300060520903661
Zhu J., Yu L., Fan Y., Zhang H., Li F., Li X., et al. (2022). Camelina sativa oil treatment alleviates castor oil-induced diarrhea in ICR mice by regulating intestinal flora composition. Evid. Based Complement. Altern. Med. 2022, 5394514. doi:10.1155/2022/5394514
Zhu S., Ran J. H., Yang B. X., Mei Z. C. (2017b). Aquaporins in digestive system. Adv. Exp. Med. Biol. 969, 123–130. doi:10.1007/978-94-024-1057-0_8
Keywords: Aquaporin 3, gut health, barrier function, inflammation, oxidative stress, autophagy
Citation: Zhu C, Nie X, Lu Q, Bai Y and Jiang Z (2023) Roles and regulation of Aquaporin-3 in maintaining the gut health: an updated review. Front. Physiol. 14:1264570. doi: 10.3389/fphys.2023.1264570
Received: 21 July 2023; Accepted: 15 November 2023;
Published: 28 November 2023.
Edited by:
Thomas S. Weiss, University Children’s Hospital Regensburg, GermanyReviewed by:
Andreia Matos, Universidade do Porto, PortugalCopyright © 2023 Zhu, Nie, Lu, Bai and Jiang. This is an open-access article distributed under the terms of the Creative Commons Attribution License (CC BY). The use, distribution or reproduction in other forums is permitted, provided the original author(s) and the copyright owner(s) are credited and that the original publication in this journal is cited, in accordance with accepted academic practice. No use, distribution or reproduction is permitted which does not comply with these terms.
*Correspondence: Zongyong Jiang, amlhbmd6eUBnZGFhcy5jbg==
Disclaimer: All claims expressed in this article are solely those of the authors and do not necessarily represent those of their affiliated organizations, or those of the publisher, the editors and the reviewers. Any product that may be evaluated in this article or claim that may be made by its manufacturer is not guaranteed or endorsed by the publisher.
Research integrity at Frontiers
Learn more about the work of our research integrity team to safeguard the quality of each article we publish.