- Agricultural Genomics Institute at Shenzhen, Chinese Academy of Agricultural Sciences, Shenzhen, China
Introduction: The development of insecticide resistance in Spodoptera frugiperda populations is a serious threat to the crop industry. Given the spread of invasive resistant populations, prospective monitoring should be accelerated, and the development of diagnostic tools for rapid and accurate assessments of insecticide resistance is essential.
Methods: First, the discriminating dose and diagnostic time of the kit were determined by the glass vial method based on a susceptible strain. Then, pests that were collected from field populations were used to determine their susceptibility to seven insecticides by using the diagnostic kit. Finally, the accuracy of the kit was verified based on correlation analyses and the likelihood of insecticide control failure was assessed.
Results: Here, we describe a diagnostic kit that enables the rapid detection of resistance to chlorpyrifos, bifenthrin, deltamethrin, lambda-cyhalothrin, phoxim, chlorantraniliprole and chlorfenapyr within 1-2 h in S. frugiperda at diagnostic doses of 0.98, 0.84, 0.38, 1.64, 0.0082, 1.75 and 0.65 μg/cm2, respectively. The linear equation between mortalities under diagnostic doses and actual resistance ratios measured by the diet-overlay bioassay was determined. The high correlation indicates that the insecticide resistance levels diagnosed by the kit were consistent with the results of the diet-overlay bioassay. Moreover, we found a significant negative correlation between diagnostic mortality and the likelihood of control failure for bifenthrin (r = −0.899, p = 0.001), deltamethrin (r = −0.737, p = 0.024) and lambda-cyhalothrin (r = −0.871, p = 0.002).
Discussion: The insecticide resistance diagnostic kit for S. frugiperda is a user-friendly tool (portable, short detection time). Its excellent performance qualifies the kit as a reliable screening tool for identifying effective insecticides in sustainable resistance management.
1 Introduction
The fall army worm (FAW), Spodoptera frugiperda (Lepidoptera: Noctuidae), is a major transboundary migratory pest that is native to tropical and subtropical regions of the Americas (Xiao, 2021). Currently, its presence has rapidly expanded to more than 100 countries worldwide (Blanco et al., 2016; Burtet et al., 2017; Ganiger et al., 2018; Guo et al., 2022). It invaded China through the Yunnan Province on 11 December 2018 and quickly spread across the country via its long-distance migration abilities (Sun et al., 2021). S. frugiperda is a highly polyphagous pest that attacks more than 353 host plants, causing major damage to maize, sugarcane, rice, wheat, sorghum, other vegetable crops and cotton. This represents a great threat to food security and results in economic loss (Baudron et al., 2019; Xiao, 2021).
Although maize hybrids that express Bacillus thuringiensis insecticidal proteins as well as biological control agents have been developed and applied against S. frugiperda (Jin et al., 2021a), chemical control remains the most common practice for the management of S. frugiperda worldwide (Omoto et al., 2016; Muraro et al., 2021). However, as a consequence of the pest’s genetic plasticity, high fecundity and particularly strong selection pressure, S. frugiperda has developed resistance against 45 active pesticide ingredients, and its reported cases of resistance amount to 200 (APRD, 2023). In particular, considering that the invaded S. frugiperda populations in China have a genetic background of multi-insecticide resistance due to its origin in the tropical and subtropical regions of Americas (Li et al., 2020a; Zhao et al., 2020), subsequent use of higher concentrations of these insecticides to maintain control will necessarily result in an increased frequency of resistant individuals and eventually a control failure (Zhang et al., 2016; Li et al., 2020b). The primary strategy for mitigating the detrimental effects of insecticide resistance is the development of an insecticide resistance management plan, in which the concept of resistance monitoring was developed as a means to minimize overuse and prevent or at least delay the development of resistance (Ruscoe, 1987; Mao et al., 2019a; Liao et al., 2020). In S. frugiperda, current resistance monitoring methods are mainly based on the diet-overlay bioassay or leaf disk method (Lira et al., 2020; Chen and Palli, 2022). Although these methods can be used effectively to monitor resistance to insecticides, one of their limitations is their complexity, which requires a range of operational procedures and specialist technicians, and that it takes at least 48 h to obtain results. This is contrary to the interests of growers, who need reliable data in a short amount of time, especially during periods of high pest infestation. Therefore, a reliable and rapid method of resistance detection needs to be developed and correlated with the field efficacy of the corresponding insecticide, thus scientifically avoiding the use of ineffective insecticides and contributing to reversing existing levels of resistance.
The glass vial bioassay, as a user-friendly method (short detection time and easy to perform), has been successfully used to detect insecticide resistance levels in a wide range of insects, such as Drosophila suzukii, Tetranychus urticae, Phlebotomus papatasi and Lutzomyia longipalpis (Kwon et al., 2010; Denlinger et al., 2016; Van Timmeren et al., 2019). Previous research has developed a diagnostic kit for the rapid detection of resistance to imidacloprid, nitenpyram, clothianidin, dinotefuran, thiamethoxam, isoprocarb and chlorpyrifos in Sogatella furcifera based on the glass vial bioassay (Mao et al., 2021). Huang et al. (2022) developed an insecticide phenotypic resistance diagnostic kit for Aphis gossypii, where the results can be obtained within 3 h. These successful applications show that an efficient and rapid method of detecting the resistance levels of S. frugiperda to insecticides will be possible.
Therefore, the objective of this study was to develop a diagnostic kit that provides the estimation of resistance to chlorpyrifos, bifenthrin, deltamethrin, lambda-cyhalothrin, phoxim, chlorantraniliprole and chlorfenapyr in 1–2 h. Multiple field populations of S. frugiperda will be used to verify the accuracy and efficiency of this diagnostic kit. The application of this kit can help practitioners or farmers quickly screen available agents and filter out ineffective insecticides, providing an important technical tool for pest resistance management.
2 Materials and methods
2.1 Insect collection
The field populations of S. frugiperda were collected from field crops (primarily maize) that were grown in Ruili of Yunnan (RL), Jiangcheng of Yunnan (JC), Sanya of Hainan (SY), Jingzhou of Hubei (JZ), Hanzhong of Shanxi (HZ), Nanchang of Jiangxi (NC), Dongyang of Zhejiang (DY), Huiyang of Guangdong (HY) and Nanning of Guangxi (NN) during 2021–2022 (Figure 1 and Supplementary Table S1). A susceptible strain (SS) of S. frugiperda originated from a population in Yunnan maize fields in 2019 and was reared on an artificial diet without any insecticide exposure in the laboratory. Third-instar larvae of the parental (F0) or first generation (F1) were used for the susceptibility bioassay. S. frugiperda populations were then reared under chamber conditions [25°C ± 1°C, 70%–80% relative humidity (RH), 14-h/10-h light/dark photoperiod] for several discrete generations until they were used in bioassays.
2.2 Reagents
The chlorpyrifos (97.5%), bifenthrin (97%), deltamethrin (98%), lambda-cyhalothrin (97%), phoxim (96%), chlorantraniliprole (95%) and chlorfenapyr (96%) used in this study were technical grade and were provided by Prof. Chaobin Xue (Shandong Agricultural University, Tai’an, China). Triton X-100 was purchased from Sigma‒Aldrich (St. Louis, MO, United States) and acetone (reagent grade) was purchased from Sinopharm Chemical Reagent Co., Ltd.
2.3 Diet-overlay bioassay
The ingestion bioassay employed a diet overlay method as described previously (Bolzan et al., 2019). The insecticides were dissolved in acetone as stock solutions and then diluted to a series of concentration gradients (concentration selection based on pre-experiments with different populations) with distilled water containing 0.1% Triton X-100. An aliquot of 40 μL insecticide solution was applied to each well, and after drying, the larvae were placed on contaminated feed (one 3rd instar larva per well), and a solution containing only distilled water and surfactant was used as the control treatment. For complete bioassays, five concentrations, plus a control (without insecticide), were used for each population and a total of 48 larvae were treated for each concentration. Each concentration was replicated three times with sixteen larvae per replicate. All treatments were maintained at 25°C ± 1°C under 70%–80% relative humidity and a 14-h/10-h light/dark photoperiod. Mortality was assessed after 72 h, and larvae showing no coordinated movement were recorded as dead when touched with a brush.
2.4 Glass vial bioassay
The concentration-mortality bioassay was administered by a glass vial method as described previously (Mao et al., 2021). The internal surface area of each glass vial (Guangzhou Li-ge Technology Co., LTD.) was 35.2 cm2 (diameter was 2.3 cm, and height was 4.3 cm). Concentrations of the insecticide were diluted in acetone, and five-seven concentrations were used to obtain concentration-mortality curves for the SS strain. Briefly, a glass vial containing 350 μL of insecticide solution and a roller mixing device was used to ensure that its interior was evenly coated with insecticide, and a total of thirty 3rd instar larvae were treated for each concentration. There were three replicates for each concentration and 6–9 doses for each insecticide. Mortality was recorded after 60 min of exposure to chlorpyrifos, bifenthrin, deltamethrin, lambda-cyhalothrin and phoxim and after 120 min of exposure to chlorantraniliprole and chlorfenapyr. Glass vials were placed in a chamber at 25°C ± 1°C and 70%–80% RH. For mortality detection in nine field-collected populations, the experimental design was completely randomized with eight replicates per population (10 larval replicates−1).
2.5 Development and accuracy verification of diagnostic kit
The diagnostic kit was designed to include seven insecticides test vials (chlorpyrifos, bifenthrin, deltamethrin, lambda-cyhalothrin, phoxim, chlorantraniliprole, and chlorfenapyr), and the LD90 for each insecticide was used as the discriminating dose based on the susceptible strain of S. frugiperda prepared by the glass vial bioassay.
A total of nine field populations were used to test and validate the accuracy of the kit. Mortalities of S. frugiperda field populations (RL, JC, SY, JZ, HZ, and NC) were exposed to LD90 of seven insecticides determined with the glass vial method and resistance determined with the diet-overlay bioassay. Seven mortality-resistance ratio linear correlation equations were conducted. By detecting the mortalities under the discriminating dose, the theoretical resistance ratio was calculated according to the mortality-resistance ratio correlation equation. Significant difference and correlation analyses between theoretical and actual resistance ratios were performed for seven insecticides evaluated in the DY, HY, and NN field populations.
2.6 Assessment of the control failure likelihood
The minimum recommended label rates of registered insecticides are shown in Supplementary Table S2 (http://www.chinapesticide.org.cn/). Based on the toxicity regression equation, the minimum recommended concentration of insecticide is converted to obtain the achieved mortality, and the achieved mortality is calculated with the expected mortality (80%) according to the following formula to evaluate the control failure likelihood. The control failure likelihood (CFL) = [1- achieved mortality (%)/expected mortality (e.g., 80%)] × 100%, where the value of the control failure likelihood >0% indicates a risk of control failure (Guedes, 2017).
2.7 Statistical analysis
The mortality data were corrected using the control mortality with Abbott’s formula (Abbott, 1925). Probit analysis was performed to evaluate the LC50 (the insecticide concentration that is required to kill 50% of the tested larvae) value, LC90 (the insecticide concentration that is required to kill 90% of the tested larvae) value, confidence limit (95% CL) and slopes, χ2 and degrees of freedom (df) (Finney, 1964). Mortality of S. frugiperda to insecticides in different field populations was tested by one-way ANOVA and Tukey’s multiple comparison test, and differences with different small letters were statistically significant (p < 0.05). The correlation between mortality and the resistance ratio was calculated using Pearson’s method, as well as significant differences between theoretical and actual resistance ratios using t-tests via IBM SPSS Statistics 20.0, and p values that were less than 0.05 were considered statistically significant.
3 Results
3.1 Development of a diagnostic kit to detect insecticide resistance
To rapidly detect S. frugiperda’s resistance levels to insecticides, a diagnostic time of 1–2 h was determined by pretesting based on a susceptible strain. The LD90 (0.98, 0.84, 0.38, 1.64, 0.0082, 1.75 and 0.65 μg/cm2, respectively) of chlorpyrifos, bifenthrin, deltamethrin, lambda-cyhalothrin, phoxim, chlorantraniliprole and chlorfenapyr was identified as the discriminating dose (Table 1). Under this dose, the mortality of the tested insecticide against the SS strain ranged from 88.8% to 95% (Table 2), which was in line with the expected mortality of 90%.
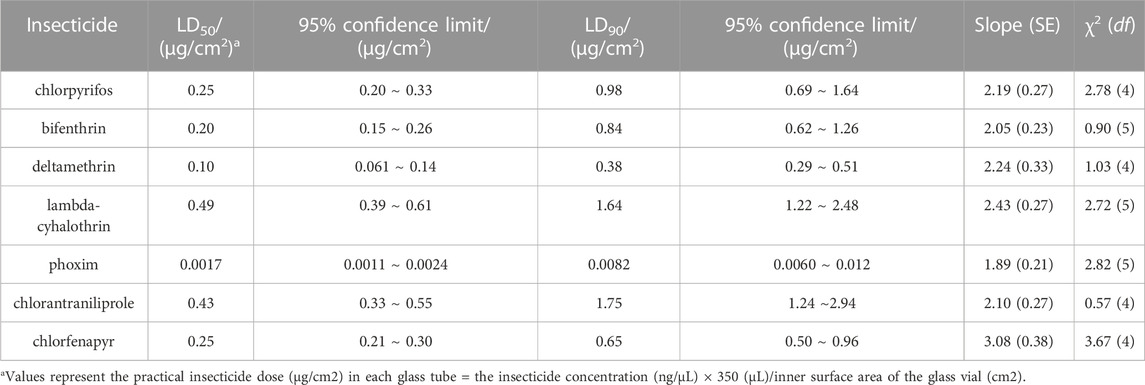
TABLE 1. The toxicity of seven insecticides to the susceptible strain (SS) of S. frugiperda determined with the glass vial method.
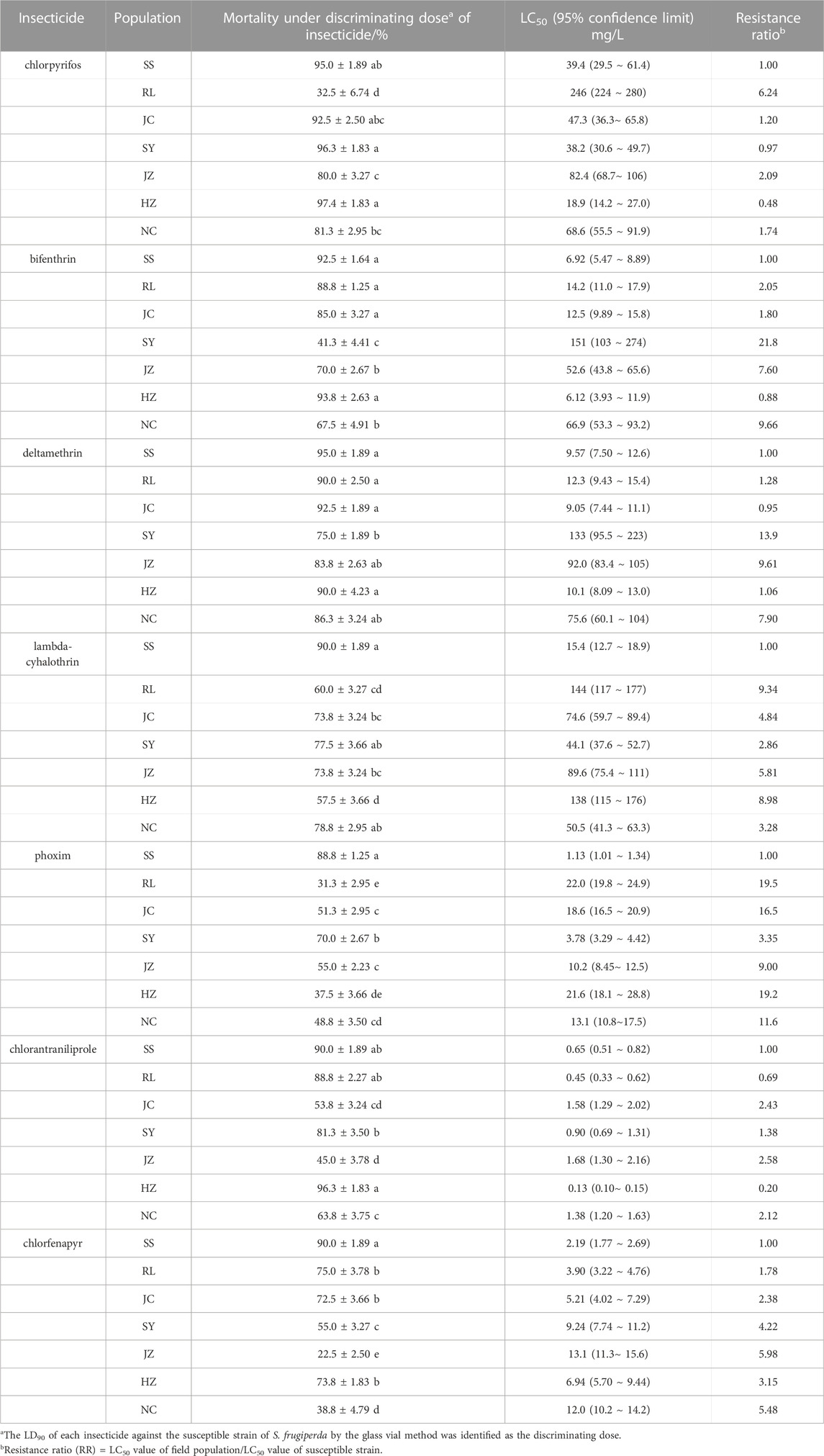
TABLE 2. Mortalities of S. frugiperda field populations exposed to LD90 of seven insecticides determined with the glass vial method and resistance determined with the diet-overlay bioassay.
3.2 Detection by the kit in field populations of S. frugiperda
Pests that were collected from six field populations (RL, JC, SY, JZ, HZ and NC) were used to rapidly determine their susceptibility to seven insecticides by using the diagnostic kit. As shown in Table 2, all six S. frugiperda strains exhibited resistance to lambda-cyhalothrin, phoxim and chlorfenapyr relative to the SS strain, with diagnostic mortality ranging from 55.7% to 78.8%, 31.3%–70.0% and 22.5%–75.0%, respectively. Only one field population remained susceptible to bifenthrin and chlorantraniliprole (mortality ≥90%), while the other five populations showed resistance to bifenthrin (41.3%–88.8%) and chlorantraniliprole (45.0%–88.8%). In addition, half of the field populations remained susceptible to chlorpyrifos at the diagnostic dose. The detection results showed that the kit could quickly distinguish between resistant and sensitive populations and assessed their susceptibility levels to the seven insecticides within 1–2 h.
3.3 Verification of kit accuracy
The LC50 values that were obtained from the diet-overlay bioassay were 18.9–246 mg/L (chlorpyrifos), 6.12–151 mg/L (bifenthrin), 9.05–133 mg/L (deltamethrin), 44.1–144 mg/L (lambda-cyhalothrin), 3.78–22.0 mg/L (phoxim), 0.13–1.68 mg/L (chlorantraniliprole) and 3.90–13.1 mg/L (chlorfenapyr) for the six field populations (Table 2). By combining the mortalities at diagnostic doses with the insecticide resistance ratios as measured by the diet-overlay bioassay, seven mortality-resistance ratio linear correlation equations were obtained (Figure 2). Furthermore, a pairwise correlation analysis showed that the diagnostic mortality of the field population was significantly correlated with the resistance ratio for chlorpyrifos (r = −0.996, p = 0.000), bifenthrin (r = −0.988, p = 0.000), deltamethrin (r = −0.947, p = 0.001), lambda-cyhalothrin (r = −0.976, p = 0.000), phoxim (r = −0.944, p = 0.001), chlorantraniliprole (r = −0.972, p = 0.000) and chlorfenapyr (r = −0.969, p = 0.000) (Table 3).
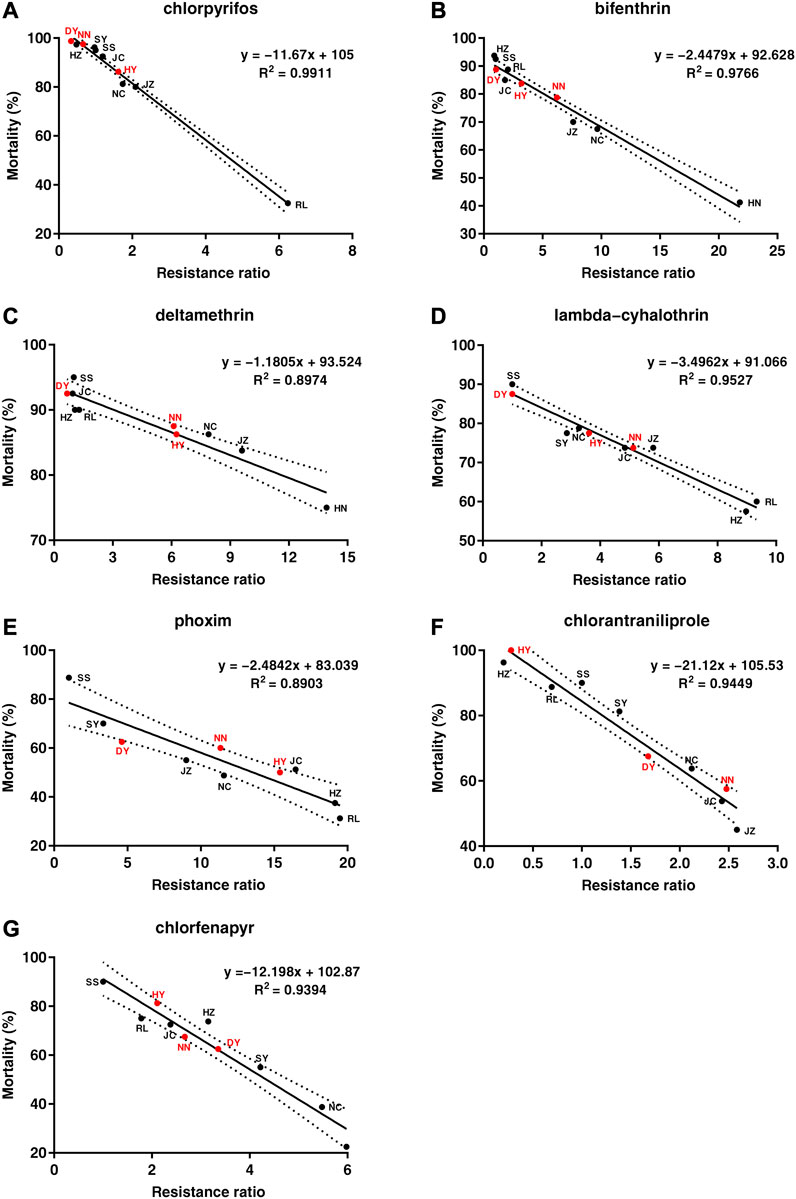
FIGURE 2. The correlation analysis between the mortality of Spodoptera frugiperda field populations under the discriminating dose of seven insecticides and the resistance ratio. (A) chlorpyrifos, (B) bifenthrin, (C) deltamethrin, (D) lambda-cyhalothrin, (E) phoxim, (F) chlorantraniliprole, (G) chlorfenapyr. The black spot represents the mortality-resistance ratio for the SS, RL, JC, SY, JZ, HZ and NC field populations. The red spot represents the mortality-resistance ratio for the three field populations (DY, HY and NN). The dashed line represents the 95% confidence interval for the mortality-resistance ratio linear equation.

TABLE 3. Pairwise correlation coefficient comparison between the mortalities of S. frugiperda field populations under the discriminating doses of seven insecticides and the resistance ratio.
Additionally, three populations (DY, HY and NN) of S. frugiperda that were collected in the field in 2022 were used to verify the accuracy of the kit. Exposure to the diagnostic doses of the seven insecticides that exhibited mortality corresponded to field populations of DY (98.8%, 95.0%, 93.8%, 91.3%, 62.5%, 67.5% and 62.5%), HY (86.3%, 83.8%, 86.3%, 77.5%, 50.0%, 100% and 81.3%) and NN (97.5%, 78.8%, 87.5%, 73.8%, 60.0%, 57.5% and 67.5%) (Table 4). The theoretical resistance ratios that were calculated from the mortality-resistance ratio correlation equation ranged from 0.54 to 8.27, 0.26–13.3 and 0.64–9.27 for DY, HY and NN, respectively, all of which were within the 95% confidence interval of the linear equation, while the resistance ratios that were determined by the diet-overlay bioassay were 0.33–4.62, 0.028–15.4 and 0.66–11.3 (Table 4), with significant correlations between the two data sets (r = 0.960, p = 0.000), and no significant differences (p > 0.05) were found between the theoretical and actual resistance ratios in the three field populations (Table 4).
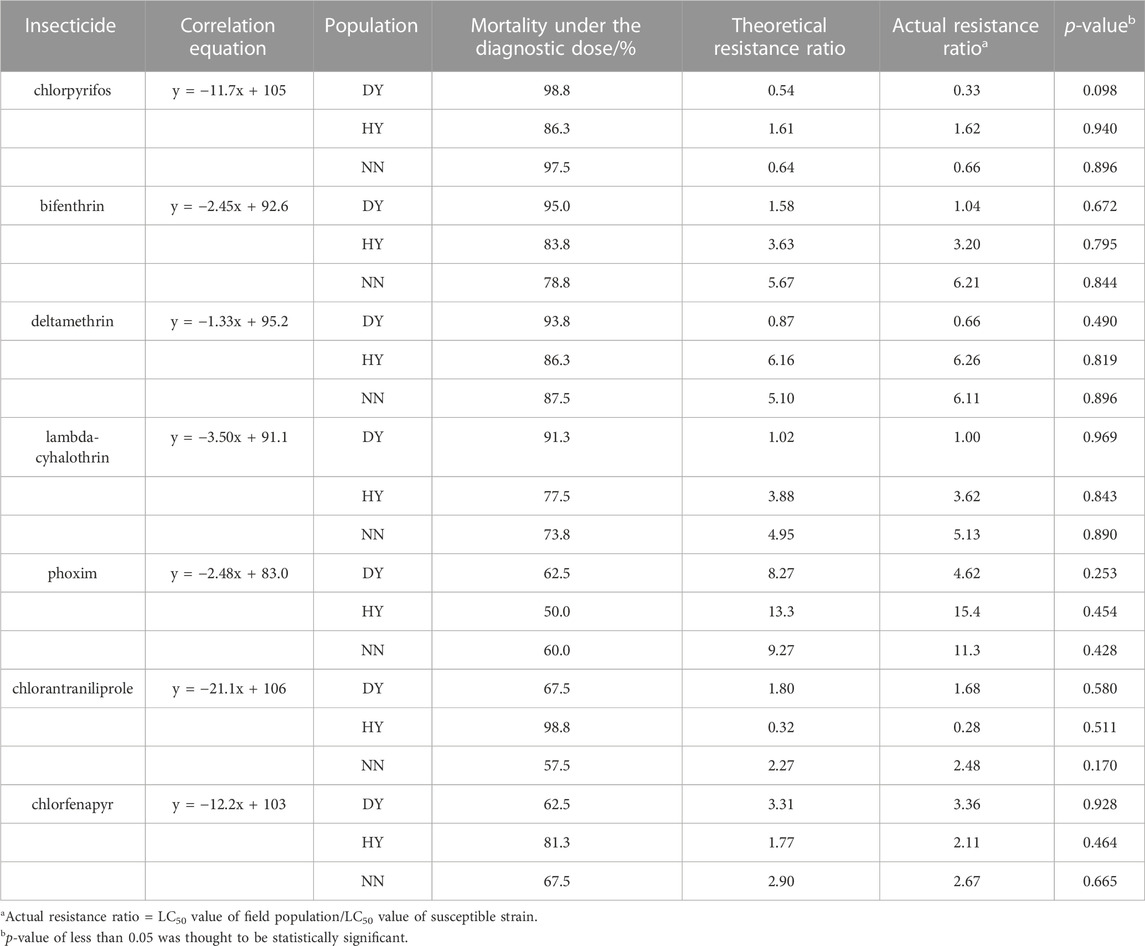
TABLE 4. Verification of the accuracy of the tested resistance levels to seven insecticides in the field populations of S. frugiperda under the discriminating dose.
3.4 Assessment of the control failure likelihood by the diagnostic kit
We conducted studies that estimated the likelihood of chemical control failure in nine field populations of S. frugiperda. The likelihood of control failure for deltamethrin was reported in all populations, and lambda-cyhalothrin and bifenthrin were at risk of control failure in one and five out of nine populations, respectively. Potential control failure populations were not identified for chlorpyrifos, phoxim, chlorantraniliprole and chlorfenapyr (Figure 3). Furthermore, a significant negative correlation was found between diagnostic mortality and the likelihood of control failure for bifenthrin (r = −0.899, p = 0.001), deltamethrin (r = −0.737, p = 0.024) and lambda-cyhalothrin (r = −0.871, p = 0.002) (Supplementary Table S3). Finally, three linear models were constructed for bifenthrin (y = −1.99x + 175), deltamethrin (y = −4.25x + 443) and lambda-cyhalothrin (y = −2.98x + 271), and based on this, it was hypothesized that the diagnostic mortality rate could only be used to control brown flies after exceeding 88.4%, 100% and 91.2%, respectively.
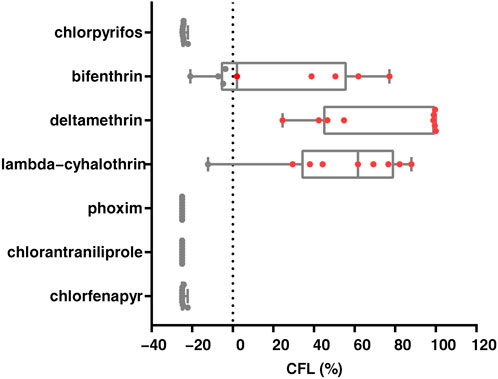
FIGURE 3. Likelihood of control failure of seven insecticides for Spodoptera frugiperda in nine field populations. Each dot represents a population, while the red dots (CFL> 0%) represent populations that control failure likelihood.
4 Discussion
Resistance monitoring and recording are critical to understanding and addressing both existing and developing pesticide resistance issues, and the development of reliable bioassays facilitates the reporting, sharing and straightforward comparison of resistance data (Chanda et al., 2016; Sparks et al., 2021). Here, we present a diagnostic kit that enables practitioners and farmers to quickly detect the resistance levels of S. frugiperda to seven insecticides and thereby reduce the impact of S. frugiperda on crop yields worldwide.
The kit was developed based on a glass vial assay, which is suitable for diagnosing susceptibility to a wide range of insecticide classes, thus meeting the criteria for easier use (Snodgrass, 1996). The bioassays in this study (30 min vial drying time and health assessments at 1 or 2 h) were designed to maximize efficiency, which is much easier than the diet-overlay bioassay or leaf disc method (Lira et al., 2020; Chen and Palli, 2022). In addition, the low cost of monitoring ensures the sustainability of enacted plans (Sikaala et al., 2014). In previous studies, we developed rapid diagnostic kits for resistance to seven insecticides based on the approach in S. furcifera, and the simple glass vials greatly reduced monitoring costs (Mao et al., 2021). Glass vials that contain a given insecticide can be prepared in advance and can be made in large quantities with simple roller equipment. Having an easy method with inexpensive equipment increases its likelihood to be adopted by crop scouts, extension agents, and growers to conduct resistance testing. Given the advantages of the kit’s low cost and simplicity of operation, it is possible to increase the number of sentinel sites for S. frugiperda on a national scale, such as community-based monitoring (CDCP, 2010; Sikaala et al., 2014), which allows the continuous detection of resistance changes during the annual in and out migration periods (Ge et al., 2022).
This diagnostic kit has greater efficiency (1 or 2 h diagnosis time) and is easier to implement than the traditional bioassay method. As a comparison, all kit-based assays can be performed immediately after field collection of S. frugiperda, whereas other bioassays usually complete testing at F1 or F2, which results in a minimum of 1 month to obtain resistance data (Jin et al., 2021b; Li et al., 2022). Because the S. frugiperda has overlapping generations in the field, it is guaranteed that insects of the appropriate life stage required for the test will be present. This not only minimizes the risk of not detecting resistant populations due to loss of selection pressure when rearing multiple generations in the laboratory (Tabashnik et al., 1994; Zhang et al., 2021), but also allows for a “race” with the pest, as the feeding of S. frugiperda is phenomenal. Once this kit is promoted at the agroecosystem level, farmers are expected to screen available insecticides in a short time, thus avoiding the use of ineffective crop protection compounds and the loss of valuable products from the overuse of single insecticides.
Using a diagnostic dose method can provide rapid and reliable monitoring to support the widespread implementation of resistance monitoring. The WHO has established criteria that indicate resistance in mosquitoes if they have a mortality that is less than 90% to insecticides (WHO, 2013). In the present study, the LD90 values of the insecticides that were identified for susceptible S. frugiperda were used as the diagnostic doses, and they were able to effectively separate susceptible and resistant populations of S. frugiperda from nine geographical populations. Furthermore, linear equations for the mortality-resistance ratio were obtained for seven insecticides that were based on six field populations and susceptible strains. Subsequently, the validity of this equation was verified in the other three field populations. The high correlation indicated that the actual resistance ratios that were estimated by the linear equation were consistent with those obtained with the diet-overlay bioassay. In particular, we found a high risk of control failure for the control of S. frugiperda with bifenthrin, deltamethrin and lambda-cyhalothrin (Figure 3), and these results are consistent with those of previous studies also showing that invasive S. frugiperda populations have developed resistance to pyrethroids (Li et al., 2022), which points to the relevance of assessing the likelihood of control failure based on the concentration of the pesticide in commercial formulations. Notably, we found a significant negative correlation between diagnostic mortality and the likelihood of control failure (bifenthrin, deltamethrin and lambda-cyhalothrin), and based on the model set, the detection criteria that would need to be met for the insecticide to be reintroduced. In addition, we found that chlorpyrifos, phoxim, chlorantraniliprole and chlorfenapyr had good control effects on S. frugiperda (CFL < −22%), which indicates that the use of these insecticides can continue to be recommended, but the risk of cross-resistance needs to be added to resistance management (Zhao et al., 2020). The results provide important guidance on the value of re-excavating insecticides.
The development of timely and accurate resistance monitoring methods in resistance monitoring and management must be integrated into S. frugiperda control programs so that available insecticides can be used judiciously and the efficacy of chemical-based control can be sustained for the long term (Mao et al., 2019b; Tay et al., 2023). The standardization of testing techniques and the promotion of products can facilitate extensive cooperation between agriculture technical extension centers or local plant protection stations, family farms and individual households so that the effectiveness of resistance management can be maximized (Chanda et al., 2016). The successful development of this kit demonstrates that this methodology can be applied to other lepidopteran pests, as well as to different insecticides.
Data availability statement
The original contributions presented in the study are included in the article/Supplementary Material, further inquiries can be directed to the corresponding author.
Author contributions
K-KM: Conceptualization, Data curation, Formal Analysis, Funding acquisition, Project administration, Resources, Writing–original draft. H-RL: Investigation, Writing–review and editing. J-YZ: Investigation, Writing–review and editing. M-HJ: Project administration, Supervision, Writing–review and editing. PW: Formal Analysis, Investigation, Writing–review and editing. YP: Project administration, Supervision, Writing–review and editing. Y-TX: Conceptualization, Data curation, Formal Analysis, Funding acquisition, Investigation, Methodology, Project administration, Software, Supervision, Validation, Writing–original draft, Writing–review and editing.
Funding
The author(s) declare financial support was received for the research, authorship, and/or publication of this article. This research was supported by grants from the Shenzhen Science and Technology Program (JCYJ20200109150629266), the National Natural Science Foundation of China (32202352), the China Postdoctoral Science Foundation (2022M713428) and the Agricultural Science and Technology Innovation Program.
Conflict of interest
The authors declare that the research was conducted in the absence of any commercial or financial relationships that could be construed as a potential conflict of interest.
Publisher’s note
All claims expressed in this article are solely those of the authors and do not necessarily represent those of their affiliated organizations, or those of the publisher, the editors and the reviewers. Any product that may be evaluated in this article, or claim that may be made by its manufacturer, is not guaranteed or endorsed by the publisher.
Supplementary material
The Supplementary Material for this article can be found online at: https://www.frontiersin.org/articles/10.3389/fphys.2023.1254765/full#supplementary-material
References
Abbott, W. A. (1925). A method of computing the effectiveness of an insecticide. J. Econ. Entomol. 18, 265–267. doi:10.1093/jee/18.2.265a
Arthropod Pesticide Resistance Database (2023). Arthropod pesticide resistance database. Available at: https://www.pesticideresistance.org/(Accessed July 7, 2023).
Baudron, F., Zaman-Allah, M. A., Chaipa, I., Chari, N., and Chinwada, P. (2019). Understanding the factors influencing fall armyworm (Spodoptera frugiperda J.E. Smith) damage in African smallholder maize fields and quantifying its impact on yield. A case study in eastern Zimbabwe. Crop Prot. 120, 141–150. doi:10.1016/j.cropro.2019.01.028
Blanco, C. A., Chiaravalle, W., Dalla-Rizza, M., Farias, J. R., García-Degano, M. F., Gastaminza, G., et al. (2016). Current situation of pests targeted by Bt crops in Latin America. Curr. Opin. Insect Sci. 15, 131–138. doi:10.1016/j.cois.2016.04.012
Bolzan, A., Padovez, F. E., Nascimento, A. R., Kaiser, I. S., Lira, E. C., Amaral, F. S., et al. (2019). Selection and characterization of the inheritance of resistance of Spodoptera frugiperda (Lepidoptera: noctuidae) to chlorantraniliprole and cross-resistance to other diamide insecticides. Pest Manag. Sci. 75, 2682–2689. doi:10.1002/ps.5376
Burtet, L. M., Bernardi, O., Melo, A. A., Pes, M. P., Strahl, T. T., and Guedes, J. V. C. (2017). Managing fall armyworm, Spodoptera frugiperda (Lepidoptera: noctuidae), with Bt maize and insecticides in southern Brazil. Pest Manag. Sci. 73, 2569–2577. doi:10.1002/ps.4660
Centers for Disease Control and Prevention (2010). Guideline for evaluating insecticide resistance in vectors using the CDC bottle bioassay. Atlanta: The Centers.
Chanda, E., Thomsen, E. K., Musapa, M., Kamuliwo, M., Brogdon, W. G., Norris, D. E., et al. (2016). An operational framework for insecticide resistance management planning. Emerg. Infect. Dis. 22, 773–779. doi:10.3201/eid2205.150984
Chen, X., and Palli, S. R. (2022). Transgenic overexpression of P450 genes confers deltamethrin resistance in the fall armyworm, Spodoptera frugiperda. J. Pest Sci. 95, 1197–1205. doi:10.1007/s10340-021-01452-6
Denlinger, D. S., Creswell, J. A., Anderson, J. L., Reese, C. K., and Bernhardt, S. A. (2016). Diagnostic doses and times for Phlebotomus papatasi and Lutzomyia longipalpis sand flies (Diptera: psychodidae: phlebotominae) using the CDC bottle bioassay to assess insecticide resistance. Parasit. Vectors 9, 212. doi:10.1186/s13071-016-1496-3
Finney, D. J. (1964). Probit analysis: A statistical treatment of the sigmoid response curve. London: Cambridge University Press.
Ganiger, P. C., Yeshwanth, H. M., Muralimohan, K., Vinay, N., Kumar, A. R. V., and Chandrashekara, K. (2018). Occurrence of the new invasive pest, fall armyworm, Spodoptera frugiperda (J.E. Smith) (Lepidoptera: noctuidae), in the maize fields of Karnataka, India. Curr. Sci. 115, 621–623. doi:10.18520/cs/v115/i4/621-623
Ge, S. S., Zhang, H. W., Liu, D. Z., Lv, C. Y., Cang, X. Z., Sun, X. X., et al. (2022). Seasonal migratory activity of SPODOPTERA FRUGIPERDA (J.E. Smith) (Lepidoptera: noctuidae) across China and Myanmar. Pest Manag. Sci. 78, 4975–4982. doi:10.1002/ps.7120
Guedes, R. N. C. (2017). Insecticide resistance, control failure likelihood and the First Law of Geography. Pest Manag. Sci. 73, 479–484. doi:10.1002/ps.4452
Guo, Z. M., Jin, R. H., Guo, Z. P., Cai, T. W., Zhang, Y. H., Gao, J. Y., et al. (2022). Insecticide susceptibility and mechanism of Spodoptera frugiperda on different host plants. J. Agric. Food Chem. 70, 11367–11376. doi:10.1021/acs.jafc.2c04189
Huang, W., Yang, Q., Ji, X., Wang, L., Wang, Q., Rui, C., et al. (2022). An insecticide phenotypic resistance diagnostic kit for cotton aphid Aphis gossypii Glover (Hemiptera Aphididae). Pest Manag. Sci. 78, 5463–5470. doi:10.1002/ps.7169
Jin, M. H., Tao, J. H., Li, Q., Cheng, Y., Sun, X. X., Wu, K. M., et al. (2021b). Genome editing of the SfABCC2 gene confers resistance to Cry1F toxin from Bacillus thuringiensis in Spodoptera frugiperda. J. Integr. Agric. 20, 815–820. doi:10.1016/s2095-3119(19)62772-3
Jin, M. H., Yang, Y. C., Shan, Y. X., Chakrabarty, S., Cheng, Y., Soberón, M., et al. (2021a). Two ABC transporters are differentially involved in the toxicity of two Bacillus thuringiensis Cry1 toxins to the invasive crop-pest Spodoptera frugiperda (J. E. Smith). Pest Manag. Sci. 77, 1492–1501. doi:10.1002/ps.6170
Kwon, D. H., Song, D. Y., Kang, S., Ahn, J. J., Lee, J. H., Choi, B. R., et al. (2010). Residual contact vial bioassay for the on-site detection of acaricide resistance in the two-spotted spider mite. J. Asia Pac. Entomol. 13, 333–337. doi:10.1016/j.aspen.2010.05.005
Li, Q., Jin, M. H., Yu, S., Cheng, Y., Shan, Y. X., Wang, P., et al. (2022). Knockout of the ABCB1 gene increases susceptibility to emamectin benzoate, beta-cypermethrin and chlorantraniliprole in Spodoptera frugiperda. Insects 13, 137. doi:10.3390/insects13020137
Li, W. H., Mao, K. K., Liu, C. Y., Gong, P. P., Xu, P. F., Wu, G., et al. (2020a). Resistance monitoring and assessment of the control failure likelihood of insecticides in field populations of the whitebacked planthopper Sogatella furcifera (Horvath). Crop Prot. 127, 104973. doi:10.1016/j.dib.2019.104973
Li, X. J., Wu, M. F., Ma, J., Gao, B. Y., Wu, Q. L., Chen, A. D., et al. (2020b). Prediction of migratory routes of the invasive fall armyworm in eastern China using a trajectory analytical approach. Pest Manag. Sci. 76, 454–463. doi:10.1002/ps.5530
Liao, X., Xu, P. F., Gong, P. P., Wan, H., and Li, J. H. (2020). Current susceptibilities of brown planthopper Nilaparvata lugens to triflumezopyrim and other frequently used insecticides in China. Insect Sci. 28, 115–126. doi:10.1111/1744-7917.12764
Lira, E. C., Bolzan, A., Nascimento, A. R., Amaral, F. S., Kanno, R. H., Kaiser, I. S., et al. (2020). Resistance of Spodoptera frugiperda (Lepidoptera: noctuidae) to spinetoram: inheritance and cross-resistance to spinosad. Pest Manag. Sci. 76, 2674–2680. doi:10.1002/ps.5812
Mao, K. K., Li, W. H., Liao, X., Liu, C. Y., Qin, Y., Ren, Z. J., et al. (2019b). Dynamics of insecticide resistance in different geographical populations of Chilo suppressalis (Lepidoptera: crambidae) in China 2016-2018. J. Econ. Entomol. 112, 1866–1874. doi:10.1093/jee/toz109
Mao, K. K., Ren, Z. J., Li, W. H., Liu, C. Y., Xu, P. F., He, S., et al. (2021). An insecticide resistance diagnostic kit for whitebacked planthopper Sogatella furcifera (Horvath). J. Pest Sci. 94, 531–540. doi:10.1007/s10340-020-01277-9
Mao, K. K., Zhang, X. L., Ali, E., Liao, X., Jin, R. H., Ren, Z. J., et al. (2019a). Characterization of nitenpyram resistance in Nilaparvata lugens (Stål). Pestic. Biochem. Physiol. 157, 26–32. doi:10.1016/j.pestbp.2019.03.001
Muraro, D. S., Abbade, Neto, D. de O., Kanno, R. H., Kaiser, I. S., Bernardi, O., and Omoto, C. (2021). Inheritance patterns, cross-resistance and synergism in Spodoptera frugiperda (Lepidoptera: noctuidae) resistant to emamectin benzoate. Pest Manag. Sci. 77, 5049–5057. doi:10.1002/ps.6545
Omoto, C., Bernardi, O., Salmeron, E., Sorgatto, R. J., Dourado, P. M., Crivellari, A., et al. (2016). Field-evolved resistance to Cry1Ab maize by Spodoptera frugiperda in Brazil. Pest Manag. Sci. 72, 1727–1736. doi:10.1002/ps.4201
Ruscoe, C. N. E. (1987). The attitude and role of the agrochemical industry towards pesticide resistance,” in Combating resistance to xenobiotics: biological and chemical approaches. Editors M. G. Ford, and D. W. Holloman (Chichester: Ellis Horwood, Ltd), 26–36.
Sikaala, C. H., Chinula, D., Chanda, J., Hamainza, B., Mwenda, M., Mukali, I., et al. (2014). A cost-effective, community-based, mosquito-trapping scheme that captures spatial and temporal heterogeneities of malaria transmission in rural Zambia. Malar. J. 13, 225. doi:10.1186/1475-2875-13-225
Snodgrass, G. L. (1996). Glass-vial bioassay to estimate insecticide resistance in adult tarnished plant bugs (heteroptera: miridae). J. Econ. Entomol. 89, 1053–1059. doi:10.1093/jee/89.5.1053
Sparks, T. C., Storer, N., Porter, A., Slater, R., and Nauen, R. (2021). Insecticide resistance management and industry: the origins and evolution of the insecticide resistance action committee (IRAC) and the mode of action classification scheme. Pest Manag. Sci. 77, 2609–2619. doi:10.1002/ps.6254
Sun, X. X., Hu, C. X., Jia, H. R., Wu, Q. L., Shen, X. J., Zhao, S. Y., et al. (2021). Case study on the first immigration of fall armyworm, Spodoptera frugiperda invading into China. J. Integr. Agric. 20, 664–672. doi:10.1016/s2095-3119(19)62839-x
Tabashnik, B. E., Finson, N., Groeters, F. R., Moar, W. J., Johnson, M. W., Luo, K., et al. (1994). Reversal of resistance to Bacillus thuringiensis in Plutella xylostella. Proc. Natl. Acad. Sci. 91, 4120–4124. doi:10.1073/pnas.91.10.4120
Tay, W. T., Meagher, R. L., Czepak, C., and Groot, A. T. (2023). Spodoptera frugiperda: ecology, evolution, and management options of an invasive species. Annu. Rev. Entomol. 68, 299–317. doi:10.1146/annurev-ento-120220-102548
Van Timmeren, S., Sial, A. A., Lanka, S. K., Spaulding, N. R., and Isaacs, R. (2019). Development of a rapid assessment method for detecting insecticide resistance in spotted wing Drosophila (Drosophila suzukii Matsumura). Pest Manag. Sci. 75, 1782–1793. doi:10.1002/ps.5341
World Health Organization (2013). Test procedures for insecticide resistance monitoring in malaria vector mosquitoes. Geneva: WHO.
Xiao, Y. T. (2021). Research on the invasive pest of fall armyworm (Spodoptera frugiperda) in China. J. Integr. Agric. 20, 633–636. doi:10.1016/s2095-3119(21)63623-7
Zhang, X. L., Liao, X., Mao, K. K., Zhang, K., Wan, H., and Li, J. H. (2016). Insecticide resistance monitoring and correlation analysis of insecticides in field populations of the brown planthopper Nilaparvata lugens (stål) in China 2012-2014. Pestic. Biochem. Physiol. 132, 13–20. doi:10.1016/j.pestbp.2015.10.003
Zhang, X. L., Mao, K. K., Liao, X., Jiang, T. Y., and Li, J. H. (2021). Inheritance mode and realized heritability of resistance to nitenpyram in the brown planthoper, Nilaparvata lugens stål (Hemiptera: delphacidae). Crop Prot. 146, 105660. doi:10.1016/j.cropro.2021.105660
Zhao, Y. X., Huang, J. M., Ni, H., Guo, D., Yang, F. X., Wang, X., et al. (2020). Susceptibility of fall armyworm, Spodoptera frugiperda (J.E.Smmith), to eight insecticides in China, with special reference to lambda-cyhalothrin. Pestic. Biochem. Physiol. 168, 104623. doi:10.1016/j.pestbp.2020.104623
Keywords: Spodoptera frugiperda, insecticide, resistance monitoring, diagnostic kit, accuracy verification
Citation: Mao K-K, Li H-R, Zhu J-Y, Jin M-H, Wang P, Peng Y and Xiao Y-T (2023) Rapid test to detect insecticide resistance in field populations of Spodoptera frugiperda (Lepidoptera: Noctuidae). Front. Physiol. 14:1254765. doi: 10.3389/fphys.2023.1254765
Received: 07 July 2023; Accepted: 14 August 2023;
Published: 23 August 2023.
Edited by:
Hongbo Jiang, Southwest University, ChinaReviewed by:
Jianhong Li, Huazhong Agricultural University, ChinaSarita Kumar, University of Delhi, India
Copyright © 2023 Mao, Li, Zhu, Jin, Wang, Peng and Xiao. This is an open-access article distributed under the terms of the Creative Commons Attribution License (CC BY). The use, distribution or reproduction in other forums is permitted, provided the original author(s) and the copyright owner(s) are credited and that the original publication in this journal is cited, in accordance with accepted academic practice. No use, distribution or reproduction is permitted which does not comply with these terms.
*Correspondence: Yu-Tao Xiao, eGlhb3l1dGFvQGNhYXMuY24=