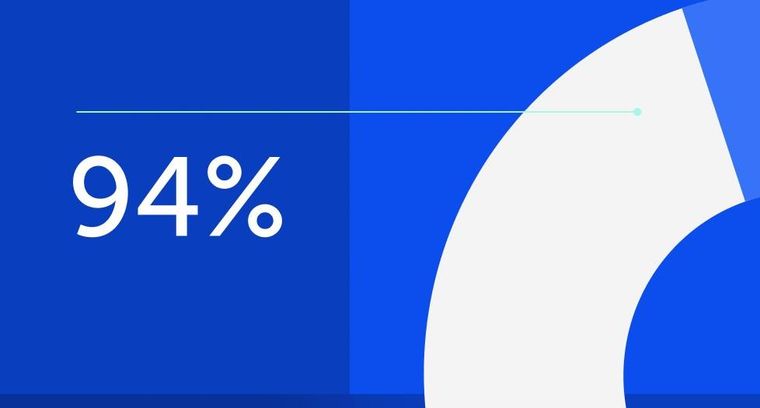
94% of researchers rate our articles as excellent or good
Learn more about the work of our research integrity team to safeguard the quality of each article we publish.
Find out more
MINI REVIEW article
Front. Physiol., 01 September 2023
Sec. Skin Physiology
Volume 14 - 2023 | https://doi.org/10.3389/fphys.2023.1252972
Insulin affects metabolic processes in different organs, including the skin. The sebaceous gland (SG) is an important appendage in the skin, which responds to insulin-mediated signals, either directly or through the insulin growth factor 1 (IGF-1) axis. Insulin cues are differently translated into the activation of metabolic processes depending on several factors, including glucose levels, receptor sensitivity, and sebocyte differentiation. The effects of diet on both the physiological function and pathological conditions of the SG have been linked to pathways activated by insulin and IGF-1. Experimental evidence and theoretical speculations support the association of insulin resistance with acne vulgaris, which is a major disorder of the SG. In this review, we examined the effects of insulin on the SG function and their implications in the pathogenesis of acne.
The cross-talk among different skin cell types is crucial in maintaining cutaneous homeostasis. These functional interactions are mediated by several, locally secreted factors including growth factors, small molecules, lipid mediators, and neuroendocrine agents (Picardo et al., 2015). In this context, the sebaceous gland (SG) activity has long been underestimated and SG has been considered “a living fossil of the skin” (Ligman, 1963). However, in recent times, the regulation of SG function has become one of the foci of scientific discussion in the field of dermatology for its relevant role in skin health and integrity (Melnik, 2015; Zouboulis et al., 2016; Zouboulis et al., 2020). The activities of the SG are controlled by various local and systemic stimuli mediated through a constellation of receptors expressed on the sebocytes (Krause et al., 2007; Pelle et al., 2008; Zouboulis, 2009). These factors not only affect the sebocyte function but also the pathomechanisms of various disorders arising from the gland. Acne vulgaris, a common disease affecting the pilo-sebaceous unit, is initiated and sustained by some of these stimuli (Dreno, 2017). Insulin, alongside the sibling hormone insulin-like growth factor-1 (IGF-1), is among the hormonal factors that affect the function of the SG (Deplewski and Rosenfield, 1999; Mirdamadi et al., 2015). They influence the metabolism of nutrients by the SG and are important players in the mechanisms of some of the diseases arising from the gland. In this review, we will touch upon the anatomy and physiology of the SG, the modes of action of insulin, and its effect on the SG function. Finally, the implication of insulin-mediated responses of the SG will be considered in the context of investigations and therapeutics of acne.
The SG is a multi-acinar, multi-lobular holocrine gland bound to a hair shaft in the dermal layer of the skin except for the palms, soles, and dorsum of the feet (Zouboulis and Tsatsou, 2014). Each acinus empties into a duct, which converges with others to a common duct that tethers the hair follicle to form the pilo-sebaceous unit, which includes the hair, the hair follicle, and the erector pili muscle (Schneider et al., 2009; Zouboulis et al., 2016). The surrounding connective tissue sheath contains fibroblasts, small nerve fibers, and blood vessels, as well as components of the extracellular matrix (Clayton et al., 2019). There are also several examples of SGs “not associated with hair follicles” like those found at mucosal sites, including oral epithelium, perianal region, and eyelids (meibomian glands) (Ahmed et al., 2022). The SG comprises sebocytes around the lumen in a centripetally layered structure corresponding to various stages of differentiation of the sebocyte units (Figure 1). Undifferentiated and proliferative sebocytes populate the outermost part of the SG, called the peripheral zone. Differentiating sebocytes, which accumulate lipid droplets and dramatically increase cell size, reside in the maturation zone, adjacent to the peripheral one. Finally, in the necrotic zone, fully differentiated sebocytes disintegrate resulting in the holocrine secretion of sebum through the ducts. The sebocyte differentiation process takes around 7–14 days (Clayton et al., 2019; Clayton et al., 2020). Sebum is a complex mixture of different types of lipids, namely, triglycerides, fatty acids, wax esters, squalene, cholesterol esters, and cholesterol. Triglycerides and free fatty acids, taken together, account for the predominant proportion (40%–60%), followed by wax esters (20%–30%), squalene (10%–20%), and cholesterol and its esters (2%–10%). Squalene and wax esters are the most characteristic products of sebaceous secretion. Sebum is enriched in squalene, sebum-specific fatty acids, i.e., terminally branched-ones and the monounsaturated sapienic acid, and wax esters. Their exceptional amounts are unique to sebum and not found anywhere else in the body nor among the epidermal surface lipids (Pappas et al., 2009; Picardo et al., 2009; Camera et al., 2016). Sebum is a skin surface protectant serving several key functions in the skin, including lubrication, moisturization, and thermoregulation. By providing an antimicrobial and antioxidant barrier, sebum contributes to shielding environmental and pro-aging insults (Zouboulis et al., 2016; Clayton et al., 2020). Sebum synthesis is regulated by various mediators, which target the numerous regulatory receptors expressed by sebocytes and are consequently involved in the control of sebum secretion. The major receptors include the corticotrophin–releasing hormone (CRH) receptor, melanocortin receptors (MC-R1-5), opiate-R, androgen receptor (AR), growth hormone receptor (GHR), IGF-1 receptor (IGF-1R), insulin receptor (IR), retinoid X receptor (RXR), retinoic acid receptor (RAR), peroxisome proliferator-activated receptors (PPAR), neuropeptide Y receptor (NYR), etc., (Zouboulis et al., 2016). The multiplicity of interactions established between endogenous factors and the sebocytes ensures the continuous and the inherent activity of the SG under physiological conditions. Beyond the production of sebum, the SG presents intrinsic metabolic, neuroendocrine, and immunological functions, which places the SG among the key players in the multicellular network underlying skin homeostasis (Picardo et al., 2015; Zouboulis et al., 2016; Szollosi et al., 2018; Clayton et al., 2020).
FIGURE 1. Schematic representation of the overall SG structure and partition into zones corresponding to various stages of sebocyte differentiation. Parts of Figure 1 were drawn using images from Servier Medical Art, provided by Servier, licensed under a Creative Commons Attribution 3.0 unported license.
Insulin synthesis and secretion is exclusive to the islet cells of the pancreas. The primary trigger of insulin secretion from the pancreas is glucose. Other factors like ketones, fatty acids, incretins like glucagon-like peptide (peptides released from the gut in response to food) can stimulate insulin release. After meals, insulin secretion increases once the concentration of glucose in the blood rises beyond 3.9 mmol/L. The insulin facilitates the upload of glucose from the blood into the tissue for storage in various forms like glycogen in the liver and muscles and lipids in the adipose tissue. Analogously to liver and adipose tissue, the SG accumulates glycogen. The glycogen-derived glycerophosphate has been addressed as a key player in the synthesis of sebum triacylglycerides by the SG from acetate (Downie and Kealey, 1998). Insulin ensures glucose entries cells and, at the same time, blood sugar remains within normal limit for maintaining body homeostasis (Raptis et al., 1975; Baumgard et al., 2016). Insulin virtually affects all the organs of the body by binding to the IR expressed at the cell surface. Insulin also binds IGF-1R but with less affinity (Giustina et al., 2015). The IR and IGF-1R are similar in their structure (Ullrich et al., 1986). In addition, there are insulin/IGF-1 hybrid receptors in most organs (Soos et al., 1990; Seely et al., 1995; Bailyes et al., 1997). These hybrid receptors have the capacity to dimerize and bind either insulin or IGF-1. The binding of insulin/IGF-1 to the receptors triggers the phosphatidylinositide-3-kinase (PI3K) second messenger pathway (Clemmons, 2012). This generates multiple effects in different tissues and organs. The overall effects are promoted cell growth, reduced blood glucose levels, increased glucose storage, protein synthesis and lipogenesis (Powers, 2012). In addition, insulin increases the serum levels of free IGF-1 by stimulating hepatic IGF-1 synthesis and suppressing the hepatic synthesis of IGFBP-1 (Clemmons, 2012).
Insulin affects the function of the SG by inducing the increase in the size and number of sebocytes, as well as lipogenesis (Ding et al., 2015), through the binding to IR and IGF-1R and the consequent activation of the PI3K/Akt pathway (Mirdamadi et al., 2015; Briganti et al., 2020). The stimulation of IGF-1R is also enhanced by the incretion of free IGF-1 that occurs when insulin secretion increases. A critical downstream element in the Akt pathway is the mammalian target of rapamycin (mTOR) (Melnik and Schmitz, 2009; Huang and Fingar, 2014; Monfrecola et al., 2016), a nutrient-sensitive regulatory factor involved, through the mTOR complex 1 (mTORC1) (Wang and Proud, 2009), in the upregulation of the sterol response element binding protein-1 (SREBP-1) (Porstmann et al., 2009; Quinn and Baum, 2012) and the gamma isoform of PPAR (PPARγ) (Porstmann et al., 2009; Laplante and Sabatini, 2013), which are both involved in the lipid synthesis occurring in sebocytes (Ricoult and Manning, 2013). The activation of the PI3K/Akt pathway produced the concomitant reduction of the nuclear level of forkhead box class-O1 (FoxO1) transcription factor (Van Der Heide et al., 2004; Huang and Fingar, 2014), thus removing the suppression of SREBP1 (Smith et al., 2006; Smith et al., 2008b), PPARg (Trivedi et al., 2006; Dozsa et al., 2014), androgen receptors (AR) (Li and Al-Azzawi, 2009; Lai et al., 2012), liver X receptor (LXR) (Russell et al., 2007; Hong et al., 2008), leading to the further promotion of sebocyte lipogenesis, cell growth, and proliferation (Armoni et al., 2006; Fan et al., 2007; Fan et al., 2009). Furthermore, the PI3K/Akt signalling is able to activate hypoxia-inducible factor 1a (HIF-1a), a transcription factor involved also in lipid accumulation in sebocytes and the upregulation of the inflammatory mediators (Semenza, 2013; Choi et al., 2021) (Figure 2).
FIGURE 2. Insulin effects on the sebaceous gland. Insulin binding to IR/IGF-1R triggers the PI3K/Akt pathway and the critical downstream element mTORC1, which in turn activates the key lipogenic transcription factor SREBP1 and induces hyperproliferation. Moreover, insulin signaling enhances androgen synthesis as well as inflammatory response. A high glycemic diet and a sebocyte low differentiation grade exacerbate insulin stimulus. TG, triglycerides; FFA, free fatty acids; WE, wax esters; CE, cholesterol esters; CH, cholesterol. Parts of Figure 2 were drawn using images from Servier Medical Art, provided by Servier, licensed under a Creative Commons Attribution 3.0 unported license.
The effect of insulin on sebocytes is affected by the level of sebocyte differentiation. Low differentiated SZ95 sebocytes tend to have a higher basal expression of IGF-1R and IR at the protein level, associated with an upregulation of phospho-Akt (pAkT) and phospho-S6 (pS6) and a down-modulation of phosphatase and tensin homolog (PTEN) expression, compared to differentiated ones (Ottaviani et al., 2020). This leads to increased susceptibility to insulin stimulus of poorly differentiated SZ95 sebocytes.
Diseases of the SG like acne vulgaris are related to abnormal hormonal stimulation of the gland. The reports of insulin resistance in patients with severe acne (Del Prete et al., 2012; Emiroglu et al., 2015; Nagpal et al., 2016) and the association of acne with diets delivering high glycaemic loads (Adebamowo et al., 2008; Smith et al., 2008a; Melnik, 2015) create a strong basis to examine the role of insulin in SG function. No acne has been found in non-Westernized populations still living under Paleolithic dietary conditions constraining carbohydrates, milk, and dairy products (Cordain et al., 2002; Melnik, 2018). On the other hand, consumption of dairy milk products has been associated with a higher frequency and severity of acne, as its components enhance the effects of insulin and IGF-1 on the production of androgen hormones and sebum and stimulate the formation of comedones (Bhate and Williams, 2014; Bronsnick et al., 2014; Albalwa et al., 2023). Acne manifests mostly at puberty, coincidentally with peaking androgens levels and insulin/IGF-1 signalling. Insulin reinforces the entire androgen axis: The pituitary gland, where it acts as a gonadotrophin amplifier; the gonads where it stimulates androgen synthesis; the adrenal glands, where it stimulates the production of androgenic precursors; the liver, where it inhibits the production of sex hormone binding globulin (SHBG), an important carrier for regulating androgen activity, and the skin, where SG is the major site of androgen biosynthesis (Chen et al., 2011; Nikolakis et al., 2016; Ju et al., 2017; Zhao et al., 2023). Insulin/IGF-1 and androgens trigger the mTOR pathway, which represents a central node between puberty, diet, and acne onset. In post-adolescence acne patients, increased glycaemia levels have been observed (Imperato-McGinley et al., 1993; Dozsa et al., 2014; Balta et al., 2015). The plasma glucose levels, which are directly associated with glucose intake, provide a carbon source for fatty acid synthesis (Strable and Ntambi, 2010; Lodhi et al., 2011) and stimulate the release of insulin and its signalling (Ameer et al., 2014), affecting de novo lipogenesis (DNL). DNL is a complex and highly regulated pathway that synthetizes fatty acids from exceeding carbohydrates (Ameer et al., 2014) and local DNL within SG is primarily responsible for human sebum production (Esler et al., 2019). Deregulated DNL is commonly associated with insulin resistance (Jensen-Urstad and Semenkovich, 2012). A low-glycaemic diet results in an improvement of insulin sensitivity and acne severity (Raptis et al., 1975; Imperato-McGinley et al., 1993; Ding et al., 2015) associated also with an improvement in the fatty acid composition of sebum (Smith et al., 2008b). The insulin/IGF1-dependent hyperstimulation of DNL promotes an increased release of fatty acids, leading to lipotoxicity, inflammation, and further promotion of the IR downstream signalling (Jensen-Urstad and Semenkovich, 2012; Ameer et al., 2014; Kim et al., 2017; Esler et al., 2019). Moreover, inflammatory cues contribute to creating a microenvironment facilitating the initiation and the aggravation of acne manifestations. The interplay of inflammatory cytokines and oxidated lipids, which are part of compositional modifications of acne sebum, concur to the acne lesions onset and progression (Capitanio et al., 2009; Capitanio et al., 2010; Ottaviani et al., 2010). This process also involves keratinocytes of the pilosebaceous duct, contributing to comedone formation due to increased proliferation of the infundibular epithelium (Zouboulis et al., 2014; Mastrofrancesco et al., 2017; Manfredini et al., 2019; Briganti et al., 2020). Acne may also be a common component of many systemic diseases or syndromes, which are also linked to insulin resistance (Chen et al., 2011). Patients with the polycystic ovarian syndrome (PCOS), hyperandrogenemia, insulin resistance, and acanthosis nigricans (HAIR-AN) syndrome, where hyperinsulinemia is present, also develop hyperseborrhea and acne (Elmer and George, 2001; Lowenstein, 2006; Kelekci et al., 2010). Acne may serve as a cutaneous marker for these syndromes. In metabolic diseases like type 2 diabetes mellitus and metabolic syndrome, characterized by insulin resistance (hyperinsulinemia) and increased mTORC1 signaling, acne may be a cutaneous feature (Nagpal et al., 2016; Melnik, 2018). There is a need for an improved dietary management of acne like in the other metabolic disorders, like type 2 diabetes mellitus, where balanced diets are essential. More studies are required to invigorate the connection between acne and these metabolic diseases. It is possible that in the future, acne will be viewed as a component of metabolic syndrome in adults.
Due to the implication of a high glycemic diet and insulin/IGF-1 in the pathogenesis of acne, mechanisms of abatement of insulin level or action are useful targets in the treatment of this disorder. Sensitizers of the IR like the biguanides, have been evaluated as adjunct therapy in moderate to severe acne vulgaris. Metformin improves insulin sensitivity resulting in the increased uptake of glucose in peripheral tissues and inhibits the mTORC1 complex action (Fabbrocini et al., 2016; Lee and Smith, 2017; Albalat et al., 2022). Acetyl-Coenzyme A Carboxylase (ACC), which catalyzes the conversion of acetyl-CoA to malonyl-CoA, is the rate-limiting enzyme in DNL, a process highly responsive to insulin. The pharmacological inhibition of ACC, leading to the suppression of sebum production, may also be exploited as a potential therapeutic option for the treatment of acne (Esler et al., 2019). Another possible therapeutic strategy may target sebocytes with a low grade of differentiation, which present upregulated expression of IR and enhanced mTOR signalling, resulting in a higher susceptibility to insulin stimulus. Scientific evidence suggests a role of altered sebocyte differentiation in the development of acne (Ottaviani et al., 2020), in agreement with genetic data on the involvement of the differentiation process of the pilosebaceous unit in the pathogenic mechanisms (Barrault et al., 2015; Clayton et al., 2019; Common et al., 2019). Induction of differentiation in sebocytes by means of a PPARγ modulator has proven effective in abating the effects of the insulin challenge in sebocyte inflammation and sebogenesis. Topical application of a PPARγ modulator in acne patients has resulted in amelioration of clinical signs and improvement of the lipid balance in sebum (Mastrofrancesco et al., 2017; Ottaviani et al., 2020).
SG is an active partaker in the cell-cell cross talk in the skin. The SG activity is relevant to both physiological homeostasis and pathological processes. A plethora of autocrine and paracrine factors regulates the SG functions. Affecting the metabolic processes in the SG, insulin is a major hormonal trigger in acne development. Insulin induces an increase in the size and number of sebocytes, as well as lipogenesis and inflammatory response, contributing to the initiation and aggravation of acne manifestations. These effects, predominantly in low differentiated sebocytes, are essentially mediated through the Insulin/IGF-1 receptor and the PI3K/Akt signalling pathway. By increasing glucose loads and stimulating insulin secretion, high glycaemic diets worsen the effects of insulin/IGF-1 on the SG. In addition, acne is a common cutaneous feature of diseases and syndromes linked to insulin resistance and related hyperinsulinemia, such as PCOS. Acne could be viewed as a metabolic disease, or a component of the metabolic syndrome where dietary management is very important. A better understanding of the mechanisms of insulin action on the SG may stimulate the development of novel therapeutic strategies targeting specific molecular pathways in the management of acne.
OO and EC conceived the manuscript idea; OO, EC, EF, and MO contributed to writing and revising of the manuscript. All authors contributed to the article and approved the submitted version.
This work was supported by public funds from the Italian Ministry of Health.
We are grateful to Dr. Sarah Mosca for her support in creating the Figure 1. Figure 1 and Figure 2 were created using images from Servier Medical Art Commons Attribution 3.0 Unported License (http://smart.servier.com).
The authors declare that the research was conducted in the absence of any commercial or financial relationships that could be construed as a potential conflict of interest.
All claims expressed in this article are solely those of the authors and do not necessarily represent those of their affiliated organizations, or those of the publisher, the editors and the reviewers. Any product that may be evaluated in this article, or claim that may be made by its manufacturer, is not guaranteed or endorsed by the publisher.
Adebamowo, C. A., Spiegelman, D., Berkey, C. S., Danby, F. W., Rockett, H. H., Colditz, G. A., et al. (2008). Milk consumption and acne in teenaged boys. J. Am. Acad. Dermatol. 58 (5), 787–793. doi:10.1016/j.jaad.2007.08.049
Ahmed, N. S., Foote, J. B., and Singh, K. K. (2022). Impaired mitochondria promote aging-associated sebaceous gland dysfunction and pathology. Am. J. Pathology 192 (11), 1546–1558. doi:10.1016/j.ajpath.2022.07.006
Albalat, W., Darwish, H., Abd-Elaal, W. H., AbouHadeed, M. H., and Essam, R. (2022). The potential role of insulin-like growth factor 1 in acne vulgaris and its correlation with the clinical response before and after treatment with metformin. J. Cosmet. Dermatology 21 (11), 6209–6214. doi:10.1111/jocd.15210
Albalwa, K., Kenawy, M., El-Fallah, A. A., and Salem, R. M. (2023). Serum and salivary adipsin levels and its association with insulin resistance in acne vulgaris patients. J. Cosmet. Dermatology 22 (4), 1354–1360. doi:10.1111/jocd.15545
Ameer, F., Scandiuzzi, L., Hasnain, S., Kalbacher, H., and Zaidi, N. (2014). De novo lipogenesis in health and disease. Metabolism Clin. Exp. 63 (7), 895–902. doi:10.1016/j.metabol.2014.04.003
Armoni, M., Harel, C., Karni, S., Chen, H., Bar-Yoseph, F., Ver, M. R., et al. (2006). FOXO1 represses peroxisome proliferator-activated receptor-gamma1 and -gamma2 gene promoters in primary adipocytes. A novel paradigm to increase insulin sensitivity. J. Biol. Chem. 281 (29), 19881–19891. doi:10.1074/jbc.M600320200
Bailyes, E. M., Nave, B. T., Soos, M. A., Orr, S. R., Hayward, A. C., and Siddle, K. (1997). Insulin receptor/IGF-I receptor hybrids are widely distributed in mammalian tissues: quantification of individual receptor species by selective immunoprecipitation and immunoblotting. Biochem. J. 327, 209–215. doi:10.1042/bj3270209
Balta, I., Ekiz, O., Ozuguz, P., Ustun, I., Karaca, S., Kacar, S. D., et al. (2015). Insulin resistance in patients with post-adolescent acne. Int. J. Dermatol. 54 (6), 662–666. doi:10.1111/ijd.12426
Barrault, C., Garnier, J., Pedretti, N., Cordier-Dirikoc, S., Ratineau, E., Deguercy, A., et al. (2015). Androgens induce sebaceous differentiation in sebocyte cells expressing a stable functional androgen receptor. J. Steroid Biochem. Mol. Biol. 152, 15234–15244. doi:10.1016/j.jsbmb.2015.04.005
Baumgard, L. H., Hausman, G. J., and Fernandez, M. V. S. (2016). Insulin: pancreatic secretion and adipocyte regulation. Domest. Anim. Endocrinol., 5476–5484. doi:10.1016/j.domaniend.2015.07.001
Bhate, K., and Williams, H. C. (2014). What's new in acne? An analysis of systematic reviews published in 2011-2012. Clin. Exp. Dermatol. 39 (3), 273–277. doi:10.1111/ced.12270
Briganti, S., Flori, E., Mastrofrancesco, A., and Ottaviani, M. (2020). Acne as an altered dermato-endocrine response problem. Exp. Dermatol. 29 (9), 833–839. doi:10.1111/exd.14168
Bronsnick, T., Murzaku, E. C., and Rao, B. K. (2014). Diet in dermatology: part I. Atopic dermatitis, acne, and nonmelanoma skin cancer. J. Am. Acad. Dermatol. 71 (6), 1039.e1–1039. doi:10.1016/j.jaad.2014.06.015
Camera, E., Ludovici, M., Tortorella, S., Sinagra, J., Capitanio, B., Goracci, L., et al. (2016). Use of lipidomics to investigate sebum dysfunction in juvenile acne. J. Lipid Res. 57 (6), 1051–1058. doi:10.1194/jlr.M067942
Capitanio, B., Sinagra, J. L., Bordignon, V., Fei, P. C., Picardo, M., and Zouboulis, C. C. (2010). Underestimated clinical features of postadolescent acne. J. Am. Acad. Dermatol. 63 (5), 782–788. doi:10.1016/j.jaad.2009.11.021
Capitanio, B., Sinagra, J. L., Ottaviani, M., Bordignon, V., Amantea, A., and Picardo, M. (2009). Acne and smoking. Dermato-Endocrinology 1 (3), 129–135. doi:10.4161/derm.1.3.9638
Chen, W., Obermayer-Pietsch, B., Hong, J., Melnik, B. C., Yamasaki, O., Dessinioti, C., et al. (2011). Acne-associated syndromes: models for better understanding of acne pathogenesis. J. Eur. Acad. Dermatology Venereol. JEADV 25 (6), 637–646. doi:10.1111/j.1468-3083.2010.03937.x
Choi, K., Jin, M., Zouboulis, C. C., and Lee, Y. (2021). Increased lipid accumulation under hypoxia in SZ95 human sebocytes. Dermatology 237 (1), 131–141. doi:10.1159/000505537
Clayton, R. W., Gobel, K., Niessen, C. M., Paus, R., Steensel, M. A. M. v., and Lim, X. (2019). Homeostasis of the sebaceous gland and mechanisms of acne pathogenesis. Br. J. Dermatol. 181 (4), 677–690. doi:10.1111/bjd.17981
Clayton, R. W., Langan, E. A., Ansell, D. M., Vos, I. J. H. M. d., Gobel, K., Schneider, M. R., et al. (2020). Neuroendocrinology and neurobiology of sebaceous glands. Biol. Rev. Camb. Philos. Soc. 95 (3), 592–624. doi:10.1111/brv.12579
Clemmons, D. R. (2012). Metabolic actions of insulin-like growth factor-I in normal physiology and diabetes. Endocrinol. Metab. Clin. North Am. 41 (2), 425–443. doi:10.1016/j.ecl.2012.04.017
Common, J. E. A., Barker, J. N., and Steensel, M. A. M. v. (2019). What does acne genetics teach us about disease pathogenesis? Br. J. Dermatol. 181 (4), 665–676. doi:10.1111/bjd.17721
Cordain, L., Lindeberg, S., Hurtado, M., Hill, K., Eaton, S. B., and Brand-Miller, J. (2002). Acne vulgaris: A disease of western civilization. Arch. Dermatol. 138 (12), 1584–1590. doi:10.1001/archderm.138.12.1584
Del Prete, M., Mauriello, M. C., Faggiano, A., Di Somma, C., Monfrecola, G., Fabbrocini, G., et al. (2012). Insulin resistance and acne: A new risk factor for men? Endocrine 42 (3), 555–560. doi:10.1007/s12020-012-9647-6
Deplewski, D., and Rosenfield, R. L. (1999). Growth hormone and insulin-like growth factors have different effects on sebaceous cell growth and differentiation. Endocrinology 140 (9), 4089–4094. doi:10.1210/endo.140.9.6957
Ding, J., Liu, Y., and Sullivan, D. A. (2015). Effects of insulin and high glucose on human meibomian gland epithelial cells. Invest. Ophthalmol. Vis. Sci. 56 (13), 7814–7820. doi:10.1167/iovs.15-18049
Downie, M. M., and Kealey, T. (1998). Lipogenesis in the human sebaceous gland: glycogen and glycerophosphate are substrates for the synthesis of sebum lipids. J. Invest. Dermatol. 111 (2), 199–205. doi:10.1046/j.1523-1747.1998.00264.x
Dozsa, A., Dezso, B., Toth, B. I., Bacsi, A., Poliska, S., Camera, E., et al. (2014). PPARγ-mediated and arachidonic acid-dependent signaling is involved in differentiation and lipid production of human sebocytes. J. Invest. Dermatol. 134 (4), 910–920. doi:10.1038/jid.2013.413
Dreno, B. (2017). What is new in the pathophysiology of acne, an overview. J. Eur. Acad. Dermatology Venereol. JEADV 31, 58–12. doi:10.1111/jdv.14374
Elmer, K. B., and George, R. M. (2001). HAIR-AN syndrome: A multisystem challenge. Am. Fam. Physician 63 (12), 2385–2390.
Emiroglu, N., Cengiz, F. P., and Kemeriz, F. (2015). Insulin resistance in severe acne vulgaris. Postepy Dermatol. i Alergol. 32 (4), 281–285. doi:10.5114/pdia.2015.53047
Esler, W. P., Tesz, G. J., Hellerstein, M. K., Beysen, C., Sivamani, R., Turner, S. M., et al. (2019). Human sebum requires de novo lipogenesis, which is increased in acne vulgaris and suppressed by acetyl-CoA carboxylase inhibition. Sci. Transl. Med. 11 (492), eaau8465. doi:10.1126/scitranslmed.aau8465
Fabbrocini, G., Izzo, R., Faggiano, A., Prete, M. D., Donnarumma, M., Marasca, C., et al. (2016). Low glycaemic diet and metformin therapy: A new approach in male subjects with acne resistant to common treatments. Clin. Exp. Dermatol. 41 (1), 38–42. doi:10.1111/ced.12673
Fan, W., Imamura, T., Sonoda, N., Sears, D. D., Patsouris, D., Kim, J. J., et al. (2009). FOXO1 transrepresses peroxisome proliferator-activated receptor gamma transactivation, coordinating an insulin-induced feed-forward response in adipocytes. J. Biol. Chem. 284 (18), 12188–12197. doi:10.1074/jbc.M808915200
Fan, W., Yanase, T., Morinaga, H., Okabe, T., Nomura, M., Daitoku, H., et al. (2007). Insulin-like growth factor 1/insulin signaling activates androgen signaling through direct interactions of Foxo1 with androgen receptor. J. Biol. Chem. 282 (10), 7329–7338. doi:10.1074/jbc.M610447200
Giustina, A., Berardelli, R., Gazzaruso, C., and Mazziotti, G. (2015). Insulin and GH-IGF-I axis: endocrine pacer or endocrine disruptor? Acta Diabetol. 52 (3), 433–443. doi:10.1007/s00592-014-0635-6
Hong, I., Lee, M., Na, T., Zouboulis, C. C., and Lee, M. (2008). LXRalpha enhances lipid synthesis in SZ95 sebocytes. J. Invest. Dermatol. 128 (5), 1266–1272. doi:10.1038/sj.jid.5701134
Huang, K., and Fingar, D. C. (2014). Growing knowledge of the mTOR signaling network. Semin. Cell Dev. Biol. 3679, 79–90. doi:10.1016/j.semcdb.2014.09.011
Imperato-McGinley, J., Gautier, T., Cai, L. Q., Yee, B., Epstein, J., and Pochi, P. (1993). The androgen control of sebum production. Studies of subjects with dihydrotestosterone deficiency and complete androgen insensitivity. J. Clin. Endocrinol. Metab. 76 (2), 524–528. doi:10.1210/jcem.76.2.8381804
Jensen-Urstad, A. P. L., and Semenkovich, C. F. (2012). Fatty acid synthase and liver triglyceride metabolism: housekeeper or messenger? Biochim. Biophys. Acta 1821 (5), 747–753. doi:10.1016/j.bbalip.2011.09.017
Ju, Q., Tao, T., Hu, T., Karadag, A. S., Al-Khuzaei, S., and Chen, W. (2017). Sex hormones and acne. Clin. Dermatol. 35 (2), 130–137. doi:10.1016/j.clindermatol.2016.10.004
Kelekci, K. H., Kelekci, S., Incki, K., Ozdemir, O., and Yilmaz, B. (2010). Ovarian morphology and prevalence of polycystic ovary syndrome in reproductive aged women with or without mild acne. Int. J. Dermatol. 49 (7), 775–779. doi:10.1111/j.1365-4632.2009.04389.x
Kim, H., Moon, S. Y., Sohn, M. Y., and Lee, W. J. (2017). Insulin-like growth factor-1 increases the expression of inflammatory biomarkers and sebum production in cultured sebocytes. Ann. Dermatology 29 (1), 20–25. doi:10.5021/ad.2017.29.1.20
Krause, K., Schnitger, A., Fimmel, S., Glass, E., and Zouboulis, C. C. (2007). Corticotropin-releasing hormone skin signaling is receptor-mediated and is predominant in the sebaceous glands. Hormone Metabolic Res. 39 (2), 166–170. doi:10.1055/s-2007-961811
Lai, J., Chang, P., Lai, K., Chen, L., and Chang, C. (2012). The role of androgen and androgen receptor in skin-related disorders. Arch. Dermatol. Res. 304 (7), 499–510. doi:10.1007/s00403-012-1265-x
Laplante, M., and Sabatini, D. M. (2013). Regulation of mTORC1 and its impact on gene expression at a glance. J. Cell. Sci. 126 (Pt 8), 1713–1719. doi:10.1242/jcs.125773
Lee, J. K., and Smith, A. D. (2017). Metformin as an adjunct therapy for the treatment of moderate to severe acne vulgaris. Dermatology Online J. 23 (11), 13030–qt53m2q13s. doi:10.5070/d32311037242
Li, J., and Al-Azzawi, F. (2009). Mechanism of androgen receptor action. Maturitas 63 (2), 142–148. doi:10.1016/j.maturitas.2009.03.008
Ligman, M. (1963). in Advances in biology of the skin. Editors W. Montagna, R. A. Ellis, and A. F. Silver (New York: Macmillan), 110.
Lodhi, I. J., Wei, X., and Semenkovich, C. F. (2011). Lipoexpediency: de novo lipogenesis as a metabolic signal transmitter. Trends Endocrinol. Metabolism TEM 22 (1), 1–8. doi:10.1016/j.tem.2010.09.002
Lowenstein, E. J. (2006). Diagnosis and management of the dermatologic manifestations of the polycystic ovary syndrome. Dermatol. Ther. 19 (4), 210–223. doi:10.1111/j.1529-8019.2006.00077.x
Manfredini, M., Bettoli, V., Sacripanti, G., Farnetani, F., Bigi, L., Puviani, M., et al. (2019). The evolution of healthy skin to acne lesions: A longitudinal, in vivo evaluation with reflectance confocal microscopy and optical coherence tomography. J. Eur. Acad. Dermatology Venereol. JEADV 33 (9), 1768–1774. doi:10.1111/jdv.15641
Mastrofrancesco, A., Ottaviani, M., Cardinali, G., Flori, E., Briganti, S., Ludovici, M., et al. (2017). Pharmacological PPARγ modulation regulates sebogenesis and inflammation in SZ95 human sebocytes. Biochem. Pharmacol. 138, 13896–14106. doi:10.1016/j.bcp.2017.04.030
Melnik, B. C. (2018). Acne vulgaris: the metabolic syndrome of the pilosebaceous follicle. Clin. Dermatol. 36 (1), 29–40. doi:10.1016/j.clindermatol.2017.09.006
Melnik, B. C. (2015). Linking diet to acne metabolomics, inflammation, and comedogenesis: an update. Clin. Cosmet. Investigational Dermatology 8, 8371–8388. doi:10.2147/CCID.S69135
Melnik, B. C., and Schmitz, G. (2009). Role of insulin, insulin-like growth factor-1, hyperglycaemic food and milk consumption in the pathogenesis of acne vulgaris. Exp. Dermatol. 18 (10), 833–841. doi:10.1111/j.1600-0625.2009.00924.x
Mirdamadi, Y., Thielitz, A., Wiede, A., Goihl, A., Papakonstantinou, E., Hartig, R., et al. (2015). Insulin and insulin-like growth factor-1 can modulate the phosphoinositide-3-kinase/Akt/FoxO1 pathway in SZ95 sebocytes in vitro. Mol. Cell. Endocrinol. 415, 41532–41544. doi:10.1016/j.mce.2015.08.001
Monfrecola, G., Lembo, S., Caiazzo, G., Vita, V. D., Caprio, R. D., Balato, A., et al. (2016). Mechanistic target of rapamycin (mTOR) expression is increased in acne patients' skin. Exp. Dermatol. 25 (2), 153–155. doi:10.1111/exd.12885
Nagpal, M., De, D., Handa, S., Pal, A., and Sachdeva, N. (2016). Insulin resistance and metabolic syndrome in young men with acne. JAMA Dermatol. 152 (4), 399–404. doi:10.1001/jamadermatol.2015.4499
Nikolakis, G., Stratakis, C. A., Kanaki, T., Slominski, A., and Zouboulis, C. C. (2016). Skin steroidogenesis in health and disease. Rev. Endocr. Metabolic Disord. 17 (3), 247–258. doi:10.1007/s11154-016-9390-z
Ottaviani, M., Camera, E., and Picardo, M. (2010). Lipid mediators in acne. Mediators Inflamm. 201010.1155/2010/858176. Epub 2010 Aug 25. doi:10.1155/2010/858176
Ottaviani, M., Flori, E., Mastrofrancesco, A., Briganti, S., Lora, V., Capitanio, B., et al. (2020). Sebocyte differentiation as a new target for acne therapy: an in vivo experience. J. Eur. Acad. Dermatology Venereol. JEADV 34 (8), 1803–1814. doi:10.1111/jdv.16252
Pappas, A., Johnsen, S., Liu, J., and Eisinger, M. (2009). Sebum analysis of individuals with and without acne. Dermato-Endocrinology 1 (3), 157–161. doi:10.4161/derm.1.3.8473
Pelle, E., McCarthy, J., Seltmann, H., Huang, X., Mammone, T., Zouboulis, C. C., et al. (2008). Identification of histamine receptors and reduction of squalene levels by an antihistamine in sebocytes. J. Invest. Dermatol. 128 (5), 1280–1285. doi:10.1038/sj.jid.5701160
Picardo, M., Mastrofrancesco, A., and Biro, T. (2015). Sebaceous gland-a major player in skin homoeostasis. Exp. Dermatol. 24 (7), 485–486. doi:10.1111/exd.12720
Picardo, M., Ottaviani, M., Camera, E., and Mastrofrancesco, A. (2009). Sebaceous gland lipids. Dermato-Endocrinology 1 (2), 68–71. doi:10.4161/derm.1.2.8472
Porstmann, T., Santos, C. R., Lewis, C., Griffiths, B., and Schulze, A. (2009). A new player in the orchestra of cell growth: SREBP activity is regulated by mTORC1 and contributes to the regulation of cell and organ size. Biochem. Soc. Trans. 37 (Pt 1), 278–283. doi:10.1042/BST0370278
Powers, A. C. (2012). Diabetes mellitus. USA: McGraw-Hill. Longo DL, Fauci AS, Kasper DL, Hauser SL, Jameson JL and Loscalzo J.
Quinn, W. J.3., and Baum, M. J. (2012). Distinct mTORC1 pathways for transcription and cleavage of SREBP-1c. Proc. Natl. Acad. Sci. U. S. A. 109 (40), 15974–15975. doi:10.1073/pnas.1214113109
Raptis, S., Dollinger, H. C., Berger, L. v., Kissing, J., Schroder, K. E., Klor, U., et al. (1975). Effect of lipids on insulin, growth hormone and exocrine pancreatic secretion in man. Eur. J. Clin. Invest. 5 (6), 521–526. doi:10.1111/j.1365-2362.1975.tb00485.x
Ricoult, S. J. H., and Manning, B. D. (2013). The multifaceted role of mTORC1 in the control of lipid metabolism. EMBO Rep. 14 (3), 242–251. doi:10.1038/embor.2013.5
Russell, L. E., Harrison, W. J., Bahta, A. W., Zouboulis, C. C., Burrin, J. M., and Philpott, M. P. (2007). Characterization of liver X receptor expression and function in human skin and the pilosebaceous unit. Exp. Dermatol. 16 (10), 844–852. doi:10.1111/j.1600-0625.2007.00612.x
Schneider, M. R., Schmidt-Ullrich, R., and Paus, R. (2009). The hair follicle as a dynamic miniorgan. Curr. Biol. CB 19 (3), 132–R142. doi:10.1016/j.cub.2008.12.005
Seely, B. L., Reichart, D. R., Takata, Y., Yip, C., and Olefsky, J. M. (1995). A functional assessment of insulin/insulin-like growth factor-I hybrid receptors. Endocrinology 136 (4), 1635–1641. doi:10.1210/endo.136.4.7895674
Semenza, G. L. (2013). HIF-1 mediates metabolic responses to intratumoral hypoxia and oncogenic mutations. J. Clin. Invest. 123 (9), 3664–3671. doi:10.1172/JCI67230
Smith, R., Mann, N., Makelainen, H., Roper, J., Braue, A., and Varigos, G. (2008b). A pilot study to determine the short-term effects of a low glycemic load diet on hormonal markers of acne: A nonrandomized, parallel, controlled feeding trial. Mol. Nutr. Food Res. 52 (6), 718–726. doi:10.1002/mnfr.200700307
Smith, R. N., Braue, A., Varigos, G. A., and Mann, N. J. (2008a). The effect of a low glycemic load diet on acne vulgaris and the fatty acid composition of skin surface triglycerides. J. Dermatol. Sci. 50 (1), 41–52. doi:10.1016/j.jdermsci.2007.11.005
Smith, T. M., Cong, Z., Gilliland, K. L., Clawson, G. A., and Thiboutot, D. M. (2006). Insulin-like growth factor-1 induces lipid production in human SEB-1 sebocytes via sterol response element-binding protein-1. J. Invest. Dermatol. 126 (6), 1226–1232. doi:10.1038/sj.jid.5700278
Soos, M. A., Whittaker, J., Lammers, R., Ullrich, A., and Siddle, K. (1990). Receptors for insulin and insulin-like growth factor-I can form hybrid dimers. Characterisation of hybrid receptors in transfected cells. Biochem. J. 270 (2), 383–390. doi:10.1042/bj2700383
Strable, M. S., and Ntambi, J. M. (2010). Genetic control of de novo lipogenesis: role in diet-induced obesity. Crit. Rev. Biochem. Mol. Biol. 45 (3), 199–214. doi:10.3109/10409231003667500
Szollosi, A. G., Olah, A., Biro, T., and Toth, B. I. (2018). Recent advances in the endocrinology of the sebaceous gland. Dermato-Endocrinology 9 (1), e1361576. doi:10.1080/19381980.2017.1361576
Trivedi, N. R., Cong, Z., Nelson, A. M., Albert, A. J., Rosamilia, L. L., Sivarajah, S., et al. (2006). Peroxisome proliferator-activated receptors increase human sebum production. J. Invest. Dermatol. 126 (9), 2002–2009. doi:10.1038/sj.jid.5700336
Ullrich, A., Gray, A., Tam, A. W., Yang-Feng, T., Tsubokawa, M., Collins, C., et al. (1986). Insulin-like growth factor I receptor primary structure: comparison with insulin receptor suggests structural determinants that define functional specificity. Embo J. 5 (10), 2503–2512. doi:10.1002/j.1460-2075.1986.tb04528.x
Van Der Heide, , Lars, P., Hoekman, M. F. M., and Smidt, M. P. (2004). The ins and outs of FoxO shuttling: mechanisms of FoxO translocation and transcriptional regulation. Biochem. J. 380 (Pt 2), 297–309. doi:10.1042/BJ20040167
Wang, X., and Proud, C. G. (2009). Nutrient control of TORC1, a cell-cycle regulator. Trends Cell Biol. 19 (6), 260–267. doi:10.1016/j.tcb.2009.03.005
Zhao, H., Wang, D., Xing, C., Lv, B., Wang, X., and He, B. (2023). Pioglitazone can improve liver sex hormone-binding globulin levels and lipid metabolism in polycystic ovary syndrome by regulating hepatocyte nuclear factor-4α. J. Steroid Biochem. Mol. Biol. 229106265, 106265. doi:10.1016/j.jsbmb.2023.106265
Zouboulis, C. C., Jourdan, E., and Picardo, M. (2014). Acne is an inflammatory disease and alterations of sebum composition initiate acne lesions. J. Eur. Acad. Dermatology Venereol. JEADV 28 (5), 527–532. doi:10.1111/jdv.12298
Zouboulis, C. C., Picardo, M., Ju, Q., Kurokawa, I., Torocsik, D., Biro, T., et al. (2016). Beyond acne: current aspects of sebaceous gland biology and function. Rev. Endocr. Metabolic Disord. 17 (3), 319–334. doi:10.1007/s11154-016-9389-5
Zouboulis, C. C. (2009). Sebaceous gland receptors. Dermato-Endocrinology 1 (2), 77–80. doi:10.4161/derm.1.2.7804
Zouboulis, C. C., and Tsatsou, F. (2014). “Anatomy of the sabaceous gland,” in Pathogenesis and Treatment of Acne and rosacea. Editors C. C. Zouboulis, A. D. Katsambas, and A. M. Kligman, 27–31.
Keywords: insulin, sebaceous gland, sebocytes, MTOR signaling, acne
Citation: Okoro OE, Camera E, Flori E and Ottaviani M (2023) Insulin and the sebaceous gland function. Front. Physiol. 14:1252972. doi: 10.3389/fphys.2023.1252972
Received: 04 July 2023; Accepted: 17 August 2023;
Published: 01 September 2023.
Edited by:
Sandrine Dubrac, Innsbruck Medical University, AustriaReviewed by:
Vivek Krishnan, Charles River Laboratories, United StatesCopyright © 2023 Okoro, Camera, Flori and Ottaviani. This is an open-access article distributed under the terms of the Creative Commons Attribution License (CC BY). The use, distribution or reproduction in other forums is permitted, provided the original author(s) and the copyright owner(s) are credited and that the original publication in this journal is cited, in accordance with accepted academic practice. No use, distribution or reproduction is permitted which does not comply with these terms.
*Correspondence: Enrica Flori, ZW5yaWNhLmZsb3JpQGlmby5pdA==
Disclaimer: All claims expressed in this article are solely those of the authors and do not necessarily represent those of their affiliated organizations, or those of the publisher, the editors and the reviewers. Any product that may be evaluated in this article or claim that may be made by its manufacturer is not guaranteed or endorsed by the publisher.
Research integrity at Frontiers
Learn more about the work of our research integrity team to safeguard the quality of each article we publish.