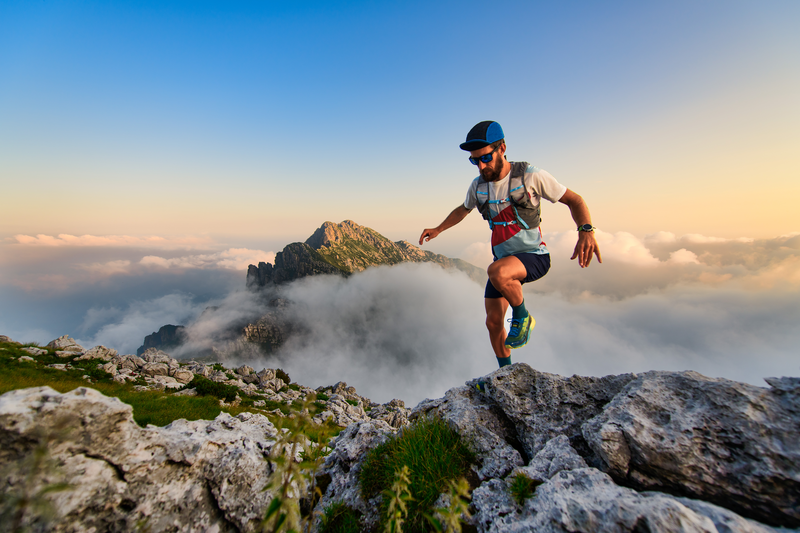
95% of researchers rate our articles as excellent or good
Learn more about the work of our research integrity team to safeguard the quality of each article we publish.
Find out more
EDITORIAL article
Front. Physiol. , 05 July 2023
Sec. Invertebrate Physiology
Volume 14 - 2023 | https://doi.org/10.3389/fphys.2023.1248654
This article is part of the Research Topic Symbiotic Organs in Insects: Development, Metabolism, and Physiological Regulation View all 9 articles
Editorial on the Research Topic
Symbiotic organs in insects: development, metabolism, and physiological regulation
Associations with microbial symbionts enable insects to thrive on nutritionally unbalanced diets or in adverse environmental conditions (Baumann, 2005; Douglas, 2015). These beneficial symbionts can live intracellularly, inside specialized host cells, or extracellularly, in expansions or modifications of the host organs. These structures are biological innovations, pivotal for maintaining symbionts through host generations. Studies are needed to elucidate the mechanisms underlying their development and morphogenesis, their anatomical diversity, and their integration in host physiology. This research topic was designed to cover those aspects. It contains seven original articles and one review, expected to inform, and inspire researchers in the field.
Four papers focus on bacteriocytes/bacteriomes. These cells/organs are specialized in harboring microbial symbionts (Buchner, 1965), and have emerged independently in several insect orders. Their localization differs depending on insect physiology and the evolutionary history of the association. In the weevil Sitophilus oryzae (Coleoptera: Curculionidea), with the recently acquired endosymbiont Sodalis pierantonius, somatic bacteriomes are eliminated in adults by apoptosis and autophagy (Vigneron et al., 2014), once the symbiont has provided tyrosine for cuticle formation. Conversely, ovarian bacteriomes are present in all stages, as they are involved in maternal symbiont transmission. The work of Ferrarini et al. shows how endosymbionts colonize the germarium in one-week-old Sitophilus females. Transcriptomic analysis of weevil ovarian bacteriomes shows that immune effectors are downregulated at the onset of sexual maturation, suggesting that relaxation of endosymbiont control by antimicrobial peptides allows bacterial migration and oocyte infection. Aphids (Hemiptera: Aphididae) have evolved a long-lasting association with the obligatory, metabolically highly integrated gammaproteobacterium Buchnera aphidicola. Symbionts are present throughout the aphid life cycle, and bacteriocyte and endosymbiont dynamics are highly coordinated and regulated by processes involving novel cell death mechanisms (Simonet et al., 2018). Ribeiro Lopes et al. show that aphid bacteriocyte numbers and sizes, and cell death processes they undergo, are remarkably plastic and vary in an environmental stress-specific manner (amino acid stress, starvation). This suggests that bacteriocytes are more than a privileged environment to house symbionts, and that studies are needed to understand the crosstalk between these cells and the rest of the body. Michalik et al. explored the diversity of heritable symbionts in planthoppers (Hemiptera: Fulgoromorpha). This hemipteran group presents very complex symbiotic systems. It is associated with ancestral symbionts Sulcia and Vidania (Michalik et al., 2021) which, in many lineages, are accompanied or have been replaced by other heritable bacteria (e.g., Sodalis, Arsenophonus, Purcelliella) or yeast-like fungi. The authors compared symbiont tissue distributions and bacteriome organization in 15 planthopper families. They found at least seven different types of bacteriocytes, with symbionts also colonizing the cytoplasm or the nuclei of the fat body, the rectal organ, or the hemolymph and the gut lumen. This correlates with the acquisition, replacement patterns, and functions of symbionts. The review by Alarcón et al. recalls the history of bacteriocyte discovery across insect orders, summarizes the profound molecular changes potentially brought about by acquisitions of symbionts and the diversity of these novel cells, and discusses their evolutionary developmental origin and ontogeny.
More recently emerged symbionts tend to be less dependent on host tissues and can be housed extracellularly. In phytophagous stinkbugs (Hemiptera: Pentatomomorpha), the symbionts are housed in sac-like structures in the posterior midgut. Stinkbugs can bear vertically transmitted Gammaproteobacteria (Fukatsu and Hosokawa, 2002) or Betaproteobacteria horizontally acquired at each new generation (Kikuchi et al., 2011). The study of Moriyama and Fukatsu focuses on the plataspid stinkbug Megacopta punctatissima, and its obligatory Gammaproteobacteria “Candidatus Ishikawella capsulata”. Ishikawella has lost some metabolic pathways redundant with the host and retained pathways involved in the production of essential amino acids, suggesting a potential role as a nutritional symbiont. The authors characterized the effect of symbiont removal on essential amino acid content and measured amino acid production in symbiotic organs cultured in vitro, then demonstrating, for the first time, that an extracellular symbiont functions similarly to nutritional symbionts residing in bacteriocytes. They establish a framework to study the mechanistic basis of these associations. Jang et al. focus on the association between the bean bug Riptortus terrestris and the betaproteobacteria Caballeronia. These authors demonstrate that symbiont colonization activates stem cell proliferation in the symbiont-bearing crypts and prolongs cellular life span of their epithelial cells by suppressing apoptosis, thereby establishing the drastic morphological changes that result in a stable symbiotic association. This opens the way for studies aimed at identifying the molecular mechanisms and signals regulating crypt morphogenesis in stinkbug symbioses.
Janke et al. focus on the association between Lagria darkling beetles (Coleoptera: Tenebrionoidea) and the community of symbionts (dominated by Burkholderia) colonizing the female accessory glands (Floréz and Kaltenpoth, 2017). The symbionts produce antibiotics that protect eggs and larvae against fungal infection. The authors describe how symbiont transmission occurs externally, via the host surface, from female pupae to adults. They also show that symbiont loss in males begins at the pupal stage, and is accompanied by morphological changes of the symbiotic organ during metamorphosis. The association with symbiotic bacteria capable of protecting offspring from fungal infection is not unique to beetles. This also constitutes the basis of the association between solitary digger wasps of the tribe Philanthini, (Hymenoptera: Crabronidae) and Streptomyces bacteria. Here the symbionts are housed in antennal glands (Kaltenpoth et al., 2014). Goettler et al. investigated the morphology of antennal glands of 14 Philanthus species from the Palearctic, Paleotropic, and Nearctic. They combined 3D-models of the glands with phylogenetic analyses and found that, despite their overall similarity, the morphology of the glands differs gradually across species, largely related to geographic origin and phylogenetic position (African and European species showing more complex structures compared to North-American species). These results corroborate the hypothesis that new-world and old-world species represent distinct subgroups within this genus.
We believe that the availability of high-quality insect genome sequences and of new imaging technologies constitutes a unique opportunity for in-depth and comparative studies into i) the evolutionary developmental origin of symbiotic organs across species, ii) the ways in which symbionts subvert cellular mechanisms to invade and colonize host tissues, and iii) how symbiotic organs are integrated in and contribute to host physiology and metabolism.
FC led the drafting of this editorial. PC, YM, and AM reviewed and edited the draft. All authors contributed to the article and approved the submitted version.
We would like to thank all authors who contributed to this Research Topic. We extend our gratitude to the reviewers and invited editors for generously dedicating their time and helped improving manuscripts with their comments and precious suggestions, as well as the Frontiers in Physiology editorial team for their support on the Research Topic management.
The authors declare that the research was conducted in the absence of any commercial or financial relationships that could be construed as a potential conflict of interest.
All claims expressed in this article are solely those of the authors and do not necessarily represent those of their affiliated organizations, or those of the publisher, the editors and the reviewers. Any product that may be evaluated in this article, or claim that may be made by its manufacturer, is not guaranteed or endorsed by the publisher.
Baumann, P. (2005). Biology bacteriocyte-associated endosymbionts of plant sap-sucking insects. Annu. Rev. Microbiol. 59, 155–189. doi:10.1146/annurev.micro.59.030804.121041
Buchner, P. (1965). Endosymbiosis of animals with plant microorganisms. New York: Interscience Publishers.
Douglas, A. E. (2015). Multiorganismal insects: Diversity and function of resident microorganisms. Annu. Rev. Entomol. 60, 17–34. doi:10.1146/annurev-ento-010814-020822
Flórez, L. V., and Kaltenpoth, M. (2017). Symbiont dynamics and strain diversity in the defensive mutualism between Lagria beetles and Burkholderia. Environ. Microbiol. 19, 3674–3688. doi:10.1111/1462-2920.13868
Fukatsu, T., and Hosokawa, T. (2002). Capsule-transmitted gut symbiotic bacterium of the Japanese common plataspid stinkbug, Megacopta punctatissima. Appl. Environ. Microbiol. 68 (1), 389–396. doi:10.1128/aem.68.1.389-396.2002
Kaltenpoth, M., Roeser-Mueller, K., Koehler, S., Peterson, A., Nechitaylo, T. Y., Stubblefield, J. W., et al. (2014). Partner choice and fidelity stabilize coevolution in a cretaceous-age defensive symbiosis. Proc. Natl. Acad. Sci. U. S. A. 111, 6359–6364. doi:10.1073/pnas.1400457111
Kikuchi, Y., Hosokawa, T., and Fukatsu, T. (2011). An ancient but promiscuous host–symbiont association between Burkholderia gut symbionts and their heteropteran hosts. ISME J. 5 (3), 446–460. doi:10.1038/ismej.2010.150
Michalik, A., Castillo Franco, D., Kobiałka, M., Szklarzewicz, T., Stroiński, A., and Łukasik, P. (2021). Alternative transmission patterns in independently acquired nutritional co-symbionts of Dictyopharidae planthoppers. mBio 12 (4), e0122821. doi:10.1128/mBio.01228-21
Simonet, P., Gaget, K., Balmand, S., Ribeiro Lopes, M., Parisot, N., Buhler, K., et al. (2018). Bacteriocyte cell death in the pea aphid/Buchnera symbiotic system. Proc. Natl. Acad. Sci. U. S. A. 1, E1819–E1828. doi:10.1073/pnas.1720237115
Keywords: insects, bacterial symbionts, bacteriocytes, gut symbiosis, insect glands, development, anatomy, symbiont transmission
Citation: Calevro F, Callaerts P, Matsuura Y and Michalik A (2023) Editorial: Symbiotic organs in insects: development, metabolism, and physiological regulation. Front. Physiol. 14:1248654. doi: 10.3389/fphys.2023.1248654
Received: 27 June 2023; Accepted: 29 June 2023;
Published: 05 July 2023.
Edited and reviewed by:
Sylvia Anton, Institut National de recherche pour l’agriculture, l’alimentation et l’environnement (INRAE), FranceCopyright © 2023 Calevro, Callaerts, Matsuura and Michalik. This is an open-access article distributed under the terms of the Creative Commons Attribution License (CC BY). The use, distribution or reproduction in other forums is permitted, provided the original author(s) and the copyright owner(s) are credited and that the original publication in this journal is cited, in accordance with accepted academic practice. No use, distribution or reproduction is permitted which does not comply with these terms.
*Correspondence: Federica Calevro, ZmVkZXJpY2EuY2FsZXZyb0BpbnNhLWx5b24uZnI=; Patrick Callaerts, cGF0cmljay5jYWxsYWVydHNAa3VsZXV2ZW4uYmU=; Yu Matsuura, eXVtYXRzdUBjb21iLnUtcnl1a3l1LmFjLmpw; Anna Michalik, YS5taWNoYWxpa0B1ai5lZHUucGw=
Disclaimer: All claims expressed in this article are solely those of the authors and do not necessarily represent those of their affiliated organizations, or those of the publisher, the editors and the reviewers. Any product that may be evaluated in this article or claim that may be made by its manufacturer is not guaranteed or endorsed by the publisher.
Research integrity at Frontiers
Learn more about the work of our research integrity team to safeguard the quality of each article we publish.