- 1Department of Dermatology, Shenzhen People’s Hospital (The Second Clinical Medical College, Jinan University, The First Affiliated Hospital, Southern University of Science and Technology), Shenzhen, Guangdong, China
- 2Department of Dermatology, Nanfang Hospital, Southern Medical University, Guangzhou, China
- 3Department of Dermatology, Dermatology Hospital, Southern Medical University, Guangzhou, China
- 4Department of Dermatology and Venereology, Nanfang Hospital, Southern Medical University, Guangzhou, China
- 5Department of Dermatology, Xiangya Hospital, Central South University, Changsha, Hunan, China
Exosomes, ranging from 40 to 160 nm in diameter, are extracellular lipid bilayer microvesicles that regulate the body’s physiological and pathological processes and are secreted by cells that contain proteins, nucleic acids, amino acids and other metabolites. Previous studies suggested that mesenchymal stem cell (MSC)-derived exosomes could either suppress or support keloid and hypertrophic scar progression. Although previous research has identified the potential value of MSC-exosomes in keloid and hypertrophic scar, a comprehensive analysis of different sources of MSC-exosome in keloid and hypertrophic scar is still lacking. This review mainly discusses different insights regarding the roles of MSC-exosomes in keloid and hypertrophic scar treatment and summarizes possible underlying mechanisms.
1 Introduction
1.1 Mesenchymal stem cells (MSCs) and conditioned medium
1.1.1 Mesenchymal stem cells (MSCs)
MSCs are the most widely studied stem cells, featuring self-renewal and multilineage differentiation capacity. MSCs are generally classified according to their source: bone marrow, trabecular bone, adipose tissue, synovium, skeletal muscle, lung, deciduous teeth, and human umbilical cord (Baksh et al., 2004). Different sources of MSCs possess similar morphological and biological features. MSCs have displayed promising potential in immune modulation, higher proliferation, tissue regeneration and angiogenesis (Hoang et al., 2022). It was suggested that human umbilical cord mesenchymal stem cells (HUCMSCs) exhibit stronger attachment than bone marrow-derived stem cells (BMSCs) and adipose-derived stem cells (ADSCs) (Wang and Yan, 2013). ADSCs display higher adipogenic ability, while BMSCs exhibit stronger chondrogenic and osteogenic capacity (Mohamed-Ahmed et al., 2018). Notably, HUCMSCs can differentiate into osteocytes, chondrocytes or adipocytes, displaying stronger chondrogenic and osteogenic properties than BMSCs(Han et al., 2017). Several studies have indicated the potential efficacy of BMSCs in skin burns (Rasulov et al., 2005; Xu et al., 2012), whereas ADSCs might have advantages due to their biological features in enhancing keratinocyte growth and secreting factors that facilitate wound healing (Li et al., 2019; Zhou et al., 2021). However, the limited sources and low survival rate of MSCs in vivo, as well as the host immune response towards MSCs, have severely restricted the use of stem cell-based therapy (Nakamura et al., 2007).
1.1.2 Conditioned medium
Emerging evidence suggests that MSCs exert effects by generating a wide range of bioactive factors. The factors are referred to as conditioned medium, consisting of growth factors, hormones, cytokines, chemokines, cell adhesion molecules, lipid mediators, ectosomes and exosomes (Li et al., 2019; Praveen Kumar et al., 2019). MSC-conditioned medium can perform a major role in immune regulation, tissue repair and regeneration and angiogenesis (Tokhanbigli et al., 2019; Lin et al., 2021). Compared with direct MSC transplantation, MSC-conditioned medium is more convenient and safer to use, displaying greater potential in clinical application (Lin et al., 2021).
1.2 Biological characteristics of exosomes
1.2.1 Biogenesis
Generally, extracellular vesicles (EVs) are classified into ectosomes and exosomes. Ectosomes (50 nm–1 μm in diameter) are vesicles derived from outwards budding of the plasma membrane and consist of microvesicles, microparticles, and large vesicles. Exosomes (40–160 nm in diameter) are endosomal vesicles produced by double invagination of the plasma membrane (Colombo et al., 2014). The invagination of the plasma membrane generates early-sorting endosomes (ESEs), which then evolve into late-sorting endosomes (LSEs) and eventually form multivesicular bodies (MVBs) with intraluminal vesicles (ILVs). MVBs can be broken down by lysosomes or autophagosomes, or release ILVs as exosomes by fusing with the plasma membrane (He et al., 2018).
1.2.2 Isolation
Currently, various technologies are employed for EV isolation, including 1) differential ultracentrifugation, which is most common and simple but time-consuming (Tauro et al., 2012; Greening et al., 2015); 2) density gradient ultracentrifugation, which is complicated and time-consuming but can isolate exosomes with high purity (Tauro et al., 2012; Greening et al., 2015); 3) size exclusion chromatography, economical and can keep EVs intact but with no specificity for nonexosomal substances and lower yield (Böing et al., 2014); 4) tangential flow filtration, simple and efficient but isolates exosomes with reduced purity (Heinemann et al., 2014; McNamara et al., 2018); 5) affinity capture, highly specific and simple but less efficient and with low yield (Tauro et al., 2012); 6) polyethylene glycol (PEG) precipitation, simple and cost-effective but isolating exosomes with rather low purity (Rider et al., 2016); and 7) reagent kits such as the exoEasy Maxi kit (QIAGEN), simple but expensive (Zhang et al., 2020). Despite the development of numerous methods for the extraction of exosomes, no standard method for exosome isolation has been established. Therefore, to facilitate the yield and purity, combining multiple extraction methods might be more efficient.
1.2.3 Characterization
Generally, the characterization of isolated exosomes consists of three aspects: 1) detection of the morphological structure of exosomes by scanning electron microscopy (SEM) or transmission electron microscopy (TEM) (Pisitkun et al., 2004); 2) identification of the size and concentration of exosomes by nanoparticle tracking analysis technology (NTA) (Maas et al., 2015) or only the size of exosomes by dynamic light scattering technology (Gercel-Taylor et al., 2012); and 3) detection of negative markers, such as calnexin, and positive markers, including integral exosomal membrane proteins (e.g., CD63, CD9, and CD81) and inner peripheral membrane proteins (e.g., TSG101, ALIX), by Western blotting, enzyme-linked immunosorbent analysis or flow cytometry (Pospichalova et al., 2015; Shao et al., 2018; Théry et al., 2018). The International Society for Extracellular Vesicles (ISEV) suggested that at least one negative and three positive EV protein markers should be detected (Théry et al., 2018).
1.2.4 Function
Exosomes derived from mammals or plants are similar in morphology and immunophenotype and share common biological functions, such as proliferation, migration, adhesion, and apoptosis; however, they present heterogeneous components and characteristics (Wang et al., 2020; Dad et al., 2021). Unlike mammals, plants are free of zoonotic or human pathogens. Therefore, plants derived exosomes exhibit non-immunogenic and innocuous property over mammals derived exosomes, which is also attributed to their efficient uptake by recipient cells and delivery of therapeutic agents, and cost-efficient production (Dad et al., 2021). Up to date, research suggested exosomes regulate processes such as development, immune responses, cardiovascular and metabolic disease, neurodegeneration and cancer (Kalluri and LeBleu, 2020). Exosomes derived from tissue-specific MSC exhibit heterogeneous characteristics and application (Table 1). Compared with MSC, MSC-exosomes display unique advantages, such as easier access and storage, few ethical issues, superior bio-compatibility and intrinsic homing effect, possessing promising therapeutic potential (Zhou et al., 2022).
2 Effect of exosomes and conditioned medium on keloids and hypertrophic scars
2.1 Therapeutic role of exosomes in keloids and hypertrophic scars
ADSC exosomes are the most widely studied and used intervention in keloid and hypertrophic scar treatment so far. Researchers have found that ADSC exosomes inhibit proliferation and extracellular matrix (ECM) production keloid fibroblast (Li et al., 2022) and hypertrophic scar fibroblast (Yuan et al., 2021). In addition, human amniotic epithelial cell exosomes suppress hypertrophic scar formation (Zhao et al., 2017). Meanwhile, inhibition of lncRNA-ASLNCS5088 and LINC01605 M2 macrophage-derived exosomes impairs fibroblast proliferation, migration and invasion (Chen et al., 2019b; Zhu et al., 2021) (Table 2).
2.2 Therapeutic role of conditioned medium in keloids and hypertrophic scars
Exosomes are essential and crucial components of the conditioned medium, rich in signaling molecules such as protein, mRNA, and miRNA, but the isolation methods are complicated, time-consuming, and with low yield and purity. Compared with exosomes, conditioned medium is rich in growth factors, cytokines, chemokines, but the preparation process includes trypsin digestion and in vitro culture, adding the risk of biological contamination (Cai et al., 2020). Studies have shown the therapeutic effect of conditioned medium on keloids and hypertrophic scars. It was revealed that ADSC- and Amnion-MSC-conditioned medium attenuated keloid fibroblast activation (Liu et al., 2018; Sato et al., 2018). Human fetal dermal mesenchymal stem cell- and human Wharton’s jelly stem cell-conditioned medium exerted similar effects on keloid fibroblasts (Fong et al., 2014; Jiao et al., 2017). Chyle fat-derived stem cell-conditioned medium prevents hypertrophic scar fibroblast activation (Chen et al., 2019a). Hu et al. (2019) observed that hypertrophic scar formation was inhibited by bone marrow concentrate-induced MSC-conditioned medium(Table 3).
More importantly, combination therapy assisted conditioned medium in reducing hypertrophic scarring. Botulinum toxin type A combined with mesenchymal stem cell-conditioned medium could effectively treat hypertrophic scars (Hu et al., 2020). In addition, hypertrophic scars could be reduced by combining fractional laser and human umbilical cord mesenchymal stem cell HUCMSC-conditioned medium (Zhang et al., 2022). In addition, hydrogels combined with lyophilized ADSC-conditioned medium reduce scar formation (Zhang et al., 2021). Consistent with previous research, the combination of β-glycerophosphate hydrogel and HUCMSC-conditioned medium prevented the formation of hypertrophic scar tissue (Zhou. et al., 2019) (Table 4).
2.3 Pathogenic advances of exosomes in keloids and hypertrophic scars
Exosomes derived from keloids and hypertrophic scars might contribute to the occurrence and development of keloids and hypertrophic scars (Table 5). Keloid fibroblast exosomes release miR-21 and increase cell proliferation and collagen production (Li et al., 2021). Consistently, hypertrophic scar fibroblast-released exosomes promote normal fibroblast proliferation and migration (Cui et al., 2022). Moreover, exosomes derived from keloid patient plasma could enhance normal fibroblast proliferation and fibrogenesis (Hu et al., 2022). Intracellular communication via exosomes between melanocytes and fibroblasts plays a key role in forming scars and keloids. Melanocyte-derived exosome miR-7704 facilitates keloid formation by activating the TGF-β/Smad pathway (SHEN et al., 2022).
3 Possible mechanisms of exosomes in keloids and hypertrophic scars
Hypertrophic scars and keloids are benign fibroproliferative disorders that may arise after skin injury. Keloids are dermal tumors characterized by abnormal fibroblast proliferation and excessive deposition of extracellular matrix. Clinically, keloids usually manifest as a hard raised scar that extends beyond the boundary of the injury. Hypertrophic scars resemble keloids but exhibit differences in clinical manifestation, histology, and epidemiology. Hypertrophic scars are generally soft, with normal skin color, do not grow beyond the original site of the wound, have low recurrence rates, and histologically exhibit well-organized type III collagen bundles. Keloids exhibit disorganized, large thick, type I and III collagen bundles with no myofibroblast nodules (Gauglitz et al., 2011). Hypertrophic scars and keloids possess common pathological processes to varying degrees, involving proliferation, apoptosis inhibition, fibrosis, angiogenesis, inflammatory response and epithelial mesenchymal transition (EMT) (Limandjaja et al., 2020; Wang et al., 2022), which might indicate the possible therapeutic mechanism of exosomes in keloids and hypertrophic scars (Figure 1).
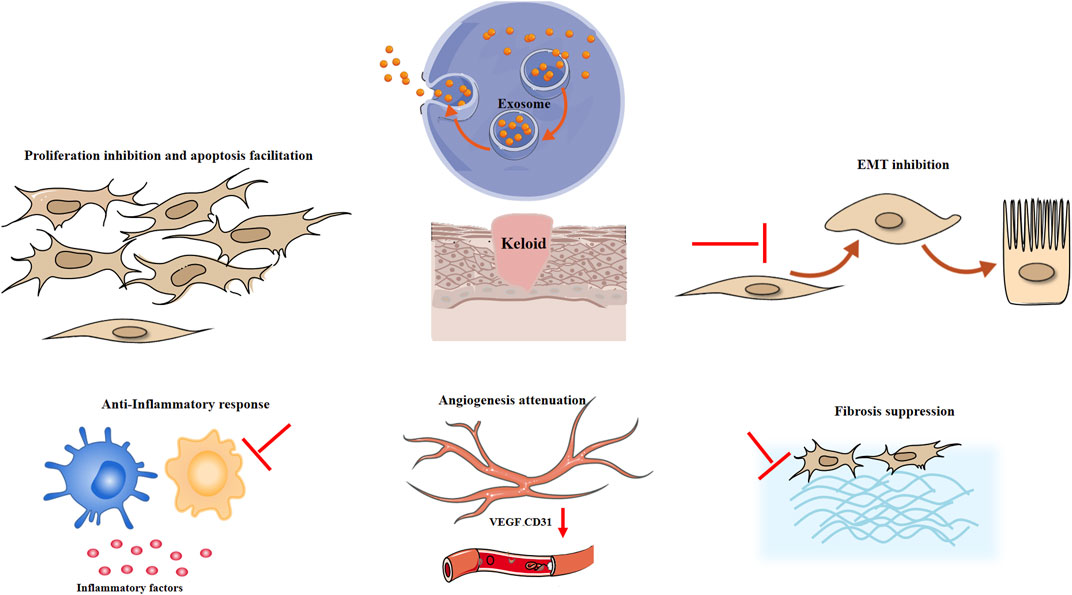
FIGURE 1. Possible mechanism of exosomes in keloids and hypertrophic scars. EMT epithelial mesenchymal transition, VEGF vascular endothelial growth factor.
3.1 Proliferation inhibition and apoptosis promotion
ADSC exosomes may attenuate the proliferation and migration and promote the apoptosis of keloid fibroblasts by inhibiting the TGF-β1/Smad pathway (Wu et al., 2021). ADSC-derived exosomes also ameliorated the proliferation and migration of hypertrophic scar fibroblasts (Li et al., 2021). It was shown that MSC-exosomes might facilitate tissue regeneration. However, it was reported that ADSC exosomes facilitate cell growth at 5 and 10 μg/mL (Ren et al., 2022) but suppress cell proliferation at 100 μg/mL (Li et al., 2022). It is hypothesized that the microenvironment and heterogeneity of fibroblasts might be responsible for the dual role of exosomes in tissue synthesis. The wound healing process can be divided into inflammatory phase, proliferative phase and remodelling phase (Monaco and Lawrence, 2003). In the proliferative phases, myofibroblasts are activated, producing ECM components and contracting wound. In the remodelling phase, myofibroblasts secreted matrix metalloproteinase and synthesizing collagen type I. Research suggests that fibroblast in different phase of wound healing display different function and response to growth factors and other molecules (Talbott et al., 2022). Therefore, exosomes might display different role towards fibroblasts, promoting tissue regeneration in the early phase (Hu et al., 2016) and inhibiting excessive ECM synthesis to prevent scar formation in the latter remodelling phase (Wang et al., 2017). However, due to complicated process of wound healing, further research is required to elucidate the role of exosomes in different phase of wounding healing and various subtypes of fibroblasts, such as reticular fibroblasts, papillary fibroblasts and myofibroblasts. In addition, ADSC-conditioned medium suppresses keloid fibroblast growth and facilitates apoptosis through the arachidonic acid-derived cyclooxygenase-2/prostaglandin E2 cascade (Yang et al., 2021). Consistently, human fetal dermal mesenchymal stem cells suppressed the growth of keloid fibroblasts and induced apoptosis by regulating BCL2/BAX protein expression (Jiao et al., 2017). However, BMSC-conditioned medium inhibited hypertrophic scar fibroblast and keloid fibroblast proliferation and migration but did not induce apoptosis (Fang et al., 2016). Similarly, Wharton’s jelly mesenchymal stem cell conditioned medium significantly prevented the growth of keloid fibroblasts, with no significant effect on the apoptosis rate (Arno et al., 2014). Except for exosomes, the conditioned medium includes soluble factors, which might exhibit anti-apoptotic effects.
3.2 Fibrosis
Keloids and hypertrophic scars are fibroproliferative diseases characterized by the pathological accumulation of ECM (Andrews et al., 2016; Lian and Li, 2016). A large and growing body of literature has demonstrated that MSC-exosomes display antifibrotic effects on hypertrophic scars and keloid fibroblasts. To determine the antifibrotic effect of ADSC-exosomes, Wang et al. detected the mRNA expression of ECM-related genes in keloid fibroblasts. The results showed that the mRNA levels of PAI-1, TIMP-1, and collagen 1 were significantly inhibited by ADSC-conditioned medium (Wang et al., 2018). Meanwhile, ADSC-derived exosomes may inhibit the proliferation, migration, and collagen synthesis of keloid fibroblasts by inhibiting the TGF-β1/Smad pathway, thus reducing scar formation (Wu et al., 2021). In addition, Yuan et al. (2021) found that miR-29a-modified ADSC-exosome therapy can downregulate the TGF-β2/Smad3 signaling pathway to attenuate collagen deposition and ECM synthesis in hypertrophic scar fibroblasts. It was reported that miR-192-5p prevents hypertrophic scar fibrosis by targeting IL17RA (Li et al., 2021). miR-let-7d mimics effectively ameliorated hypertrophic scar fibrosis (Zhao et al., 2023). Collectively, these studies outlined a crucial antifibrotic role of exosomes in hypertrophic scars and keloid fibroblasts.
3.3 Angiogenesis
During the wound healing process, excessive angiogenesis significantly facilitates keloid formation by continuously supplying nutrients, which is similar to tumours (Viallard and Larrivée, 2017; Korntner et al., 2019). Thus, we mainly discussed the effect of exosomes on angiogenesis in tumors. Wang et al. (2018) first demonstrated that ADSC-exosomes disrupted the microvessel structure in keloid tissue explants, with reduced CD31+ and CD34+ vessels. Similarly, it was reported that BMSC exosome-derived miR-16 could attenuate angiogenesis and tumor progression by directly targeting vascular endothelial growth factor (VEGF) in breast cancer (Lee et al., 2013). In addition, BMSC exosome-derived miR-100 inhibited VEGF expression via the mTOR/HIF-1α pathway, thereby suppressing the angiogenesis of breast cancer (Pakravan et al., 2017). However, it was revealed that MSC-exosomes activated the extracellular signal-regulated kinase 1/2 (ERK1/2) pathway, thereby elevating VEGF expression and eventually contributing to tumor angiogenesis (Zhu et al., 2012). Overall, these studies suggested a critical role of MSC-exosomes in angiogenesis.
3.4 Inflammatory response
Excessive inflammation in the wound healing phase causes abnormal scarring that contributes to a range of abnormal phenotypes, such as hypertrophic and keloid scars (Ogawa, 2017). Studies have revealed that macrophages, mast cells, dendritic cells (DCs) and regulatory T cells are involved in the occurrence of keloids (Zhang et al., 2006; Onodera et al., 2007; Murao et al., 2014; Direder et al., 2022). MSC-exosomes exert immunomodulatory effects and alleviate the inflammatory response by suppressing immune cell function and the synthesis of inflammatory cytokines (Harrell et al., 2019). M2 macrophage polarization and regulatory T-cell expansion were induced by MSC-exosomes (Cho et al., 2018; Sun et al., 2022). MSC-exosomes could decrease the number of mast cells in skin lesions and the maturation of bone marrow DCs, alleviating DC-induced immune responses (Shahir et al., 2020). BMSC exosomes prevented the growth and induced the apoptosis of CD4+ T cells (Del Fattore et al., 2015). The proliferation and differentiation of B lymphocytes was inhibited by BMSC exosomes (Conforti et al., 2014). In microglial cells treated with MSC-exosomes, the production of inflammatory cytokines (TNFα and IL-1β) was inhibited, while the generation of anti-inflammatory cytokines (IL-10 and TGF-β) was enhanced (Harrell et al., 2019). These findings suggested that MSC-exosomes exhibit anti-inflammatory effects by transforming proinflammatory immune cells (M1 macrophages, DCs, CD4+ T cells) into anti-inflammatory M2 macrophages, tolerogenic DCs and regulatory T cells.
3.5 EMT
EMT is a cellular process in which epithelial cells acquire a mesenchymal phenotype, elevating invasiveness. Epithelial-mesenchymal transition plays a role in the development of hypertrophic scars and keloids (Dongre and Weinberg, 2019; Xia et al., 2022). Numerous studies have revealed that MSC-derived EVs ameliorate EMT. It was found that MSC-exosomes alleviated the EMT of radiation-induced alveolar epithelial cells (Li et al., 2022). HUCMSC-derived exosomes suppressed EMT in cholangiocarcinoma (Li and Wang, 2022) and significantly downregulated colorectal cancer cell EMT via the miR-100/mTOR/miR-143 pathway (Jahangiri et al., 2022). In addition, the EMT of breast cancer cells was restrained by BMSC-derived exosomes (Zhang et al., 2022). In contrast, Shi et al. (2016) found that BMSC-derived exosomes enhanced the EMT of nasopharyngeal carcinoma cells. Similarly, Zhou et al. (2019) observed that HUCMSC-EVs induced EMT by activating the ERK pathway, contributing to breast cancer development and metastasis. These findings collectively suggested that exosomes might exhibit a dual role in EMT, and their specific role in keloids and hypertrophic scars needs to be explored in the future.
4 Conclusion
In summary, MSC-exosomes exhibit multiple effects on keloid and hypertrophic scar formation and progression and function as a promising clinical cell-free therapy. Differences in exosome dose and source might explain its dual role in keloids and hypertrophic scars. To date, researchers have only explored the role of MSC-exosomes in keloids and hypertrophic scar fibroblasts. In contrast, the impact of MSC-exosomes on keloid and hypertrophic scar keratinocytes and immune cells remains unknown. In addition, the clinical application and combination therapy of MSC-exosomes in keloid and hypertrophic scar treatment is still at the initial stage. Therefore, further research is required to elucidate their molecular mechanism and facilitate clinical application.
Author contributions
YiZ, YoZ, AY, and ZZ conceived and wrote the manuscript. KX, ZD, QW, and JZ reviewed and revised the manuscript. All authors contributed to the article and approved the submitted version.
Funding
This work was supported by the National Natural Science Foundation of China (82073019, 82073018, and 82202851), Shenzhen Science and Technology Innovation Commission, China (Natural Science Foundation of Shenzhen), (JCYJ20210324113001005, JCYJ20210324114212035, and JCYJ20220530151817038). This work was supported by Grant No. from the National Natural Science Foundation; and Grant No. from Shenzhen Science and Technology Planning Project.
Conflict of interest
The authors declare that the research was conducted in the absence of any commercial or financial relationships that could be construed as a potential conflict of interest.
Publisher’s note
All claims expressed in this article are solely those of the authors and do not necessarily represent those of their affiliated organizations, or those of the publisher, the editors and the reviewers. Any product that may be evaluated in this article, or claim that may be made by its manufacturer, is not guaranteed or endorsed by the publisher.
References
Andrews J. P., Marttala J., Macarak E., Rosenbloom J., Uitto J. (2016). Keloids: the paradigm of skin fibrosis – pathomechanisms and treatment. Matrix Biol. 51, 37–46. doi:10.1016/j.matbio.2016.01.013
Arno A. I., Amini-Nik S., Blit P. H., Al-Shehab M., Belo C., Herer E., et al. (2014). Effect of human Wharton’s jelly mesenchymal stem cell paracrine signaling on keloid fibroblasts. Stem Cells Transl. Med. 3, 299–307. doi:10.5966/sctm.2013-0120
Baksh D., Song L., Tuan R. S. (2004). Adult mesenchymal stem cells: characterization, differentiation, and application in cell and gene therapy. J. Cell Mol. Med. 8, 301–316. doi:10.1111/j.1582-4934.2004.tb00320.x
Böing A. N., van der Pol E., Grootemaat A. E., Coumans F. A. W., Sturk A., Nieuwland R. (2014). Single-step isolation of extracellular vesicles by size-exclusion chromatography. J. Extracell. Vesicles 3, 23430. doi:10.3402/jev.v3.23430
Cai Y., Li J., Jia C., He Y., Deng C. (2020). Therapeutic applications of adipose cell-free derivatives: A review. Stem Cell Res. Ther. 11, 312. doi:10.1186/s13287-020-01831-3
Chen J., Li Z., Huang Z., Liang L., Chen M. (2019a). Chyle fat-derived stem cells conditioned medium inhibits hypertrophic scar fibroblast activity. Ann. Plast. Surg. 83, 271–277. doi:10.1097/SAP.0000000000001932
Chen J., Zhou R., Liang Y., Fu X., Wang D., Wang C. (2019b). Blockade of lncRNA-ASLNCS5088-enriched exosome generation in M2 macrophages by GW4869 dampens the effect of M2 macrophages on orchestrating fibroblast activation. FASEB J. 33, 12200–12212. doi:10.1096/fj.201901610
Cho B. S., Kim J. O., Ha D. H., Yi Y. W. (2018). Exosomes derived from human adipose tissue-derived mesenchymal stem cells alleviate atopic dermatitis. Stem Cell Res. Ther. 9, 187. doi:10.1186/s13287-018-0939-5
Colombo M., Raposo G., Théry C. (2014). Biogenesis, secretion, and intercellular interactions of exosomes and other extracellular vesicles. Annu. Rev. Cell Dev. Biol. 30, 255–289. doi:10.1146/annurev-cellbio-101512-122326
Conforti A., Scarsella M., Starc N., Giorda E., Biagini S., Proia A., et al. (2014). Microvescicles derived from mesenchymal stromal cells are not as effective as their cellular counterpart in the ability to modulate immune responses in vitro. Stem Cells Dev. 23, 2591–2599. doi:10.1089/scd.2014.0091
Cui H. S., Kim D. H., Joo S. Y., Cho Y. S., Kim J.-B., Seo C. H. (2022). Exosomes derived from human hypertrophic scar fibroblasts induces smad and TAK1 signaling in normal dermal fibroblasts. Arch. Biochem. Biophys. 722, 109215. doi:10.1016/j.abb.2022.109215
Dad H. A., Gu T.-W., Zhu A.-Q., Huang L.-Q., Peng L.-H. (2021). Plant exosome-like nanovesicles: emerging therapeutics and drug delivery nanoplatforms. Mol. Ther. 29, 13–31. doi:10.1016/j.ymthe.2020.11.030
Del Fattore A., Luciano R., Pascucci L., Goffredo B. M., Giorda E., Scapaticci M., et al. (2015). Immunoregulatory effects of mesenchymal stem cell-derived extracellular vesicles on T lymphocytes. Cell Transpl. 24, 2615–2627. doi:10.3727/096368915X687543
Direder M., Weiss T., Copic D., Vorstandlechner V., Laggner M., Pfisterer K., et al. (2022). Schwann cells contribute to keloid formation. Matrix Biol. 108, 55–76. doi:10.1016/j.matbio.2022.03.001
Dongre A., Weinberg R. A. (2019). New insights into the mechanisms of epithelial-mesenchymal transition and implications for cancer. Nat. Rev. Mol. Cell Biol. 20, 69–84. doi:10.1038/s41580-018-0080-4
Fan L., Liu C., Chen X., Zheng L., Zou Y., Wen H., et al. (2022). Exosomes-loaded electroconductive hydrogel synergistically promotes tissue repair after spinal cord injury via immunoregulation and enhancement of myelinated axon growth. Adv. Sci. (Weinh) 9, e2105586. doi:10.1002/advs.202105586
Fang F., Huang R.-L., Zheng Y., Liu M., Huo R. (2016). Bone marrow derived mesenchymal stem cells inhibit the proliferative and profibrotic phenotype of hypertrophic scar fibroblasts and keloid fibroblasts through paracrine signaling. J. Dermatol Sci. 83, 95–105. doi:10.1016/j.jdermsci.2016.03.003
Fong C.-Y., Biswas A., Subramanian A., Srinivasan A., Choolani M., Bongso A. (2014). Human keloid cell characterization and inhibition of growth with human Wharton’s jelly stem cell extracts. J. Cell Biochem. 115, 826–838. doi:10.1002/jcb.24724
Gauglitz G. G., Korting H. C., Pavicic T., Ruzicka T., Jeschke M. G. (2011). Hypertrophic scarring and keloids: pathomechanisms and current and emerging treatment strategies. Mol. Med. 17, 113–125. doi:10.2119/molmed.2009.00153
Gercel-Taylor C., Atay S., Tullis R. H., Kesimer M., Taylor D. D. (2012). Nanoparticle analysis of circulating cell-derived vesicles in ovarian cancer patients. Anal. Biochem. 428, 44–53. doi:10.1016/j.ab.2012.06.004
Greening D. W., Xu R., Ji H., Tauro B. J., Simpson R. J. (2015). A protocol for exosome isolation and characterization: evaluation of ultracentrifugation, density-gradient separation, and immunoaffinity capture methods. Methods Mol. Biol. 1295, 179–209. doi:10.1007/978-1-4939-2550-6_15
Han I., Kwon B.-S., Park H.-K., Kim K. S. (2017). Differentiation potential of mesenchymal stem cells is related to their intrinsic mechanical properties. Int. Neurourol. J. 21, S24–S31. doi:10.5213/inj.1734856.428
Harrell C. R., Jovicic N., Djonov V., Arsenijevic N., Volarevic V. (2019). Mesenchymal stem cell-derived exosomes and other extracellular vesicles as new remedies in the therapy of inflammatory diseases. Cells 8, 1605. doi:10.3390/cells8121605
He C., Zheng S., Luo Y., Wang B. (2018). Exosome theranostics: biology and translational medicine. Theranostics 8, 237–255. doi:10.7150/thno.21945
He L., He T., Xing J., Zhou Q., Fan L., Liu C., et al. (2020). Bone marrow mesenchymal stem cell-derived exosomes protect cartilage damage and relieve knee osteoarthritis pain in a rat model of osteoarthritis. Stem Cell Res. Ther. 11, 276. doi:10.1186/s13287-020-01781-w
Heinemann M. L., Ilmer M., Silva L. P., Hawke D. H., Recio A., Vorontsova M. A., et al. (2014). Benchtop isolation and characterization of functional exosomes by sequential filtration. J. Chromatogr. A 1371, 125–135. doi:10.1016/j.chroma.2014.10.026
Hoang D. M., Pham P. T., Bach T. Q., Ngo A. T. L., Nguyen Q. T., Phan T. T. K., et al. (2022). Stem cell-based therapy for human diseases. Signal Transduct. Target Ther. 7, 272. doi:10.1038/s41392-022-01134-4
Hu C.-H., Tseng Y.-W., Chiou C.-Y., Lan K.-C., Chou C.-H., Tai C.-S., et al. (2019). Bone marrow concentrate-induced mesenchymal stem cell conditioned medium facilitates wound healing and prevents hypertrophic scar formation in a rabbit ear model. Stem Cell Res. Ther. 10, 275. doi:10.1186/s13287-019-1383-x
Hu C.-H., Tseng Y.-W., Lee C.-W., Chiou C.-Y., Chuang S.-S., Yang J.-Y., et al. (2020). Combination of mesenchymal stem cell-conditioned medium and botulinum toxin type A for treating human hypertrophic scars. J. Plast. Reconstr. Aesthet. Surg. 73, 516–527. doi:10.1016/j.bjps.2019.07.010
Hu H., Mao G., Zheng J., Guo F. (2022). Keloid patient plasma-derived exosomal hsa_circ_0020792 promotes normal skin fibroblasts proliferation, migration, and fibrogenesis via modulating miR-193a-5p and activating TGF-β1/smad2/3 signaling. Drug Des. Devel Ther. 16, 4223–4234. doi:10.2147/DDDT.S386786
Hu L., Wang J., Zhou X., Xiong Z., Zhao J., Yu R., et al. (2016). Exosomes derived from human adipose mensenchymal stem cells accelerates cutaneous wound healing via optimizing the characteristics of fibroblasts. Sci. Rep. 6, 32993. doi:10.1038/srep32993
Jahangiri B., Khalaj-Kondori M., Asadollahi E., Purrafee Dizaj L., Sadeghizadeh M. (2022). MSC-Derived exosomes suppress colorectal cancer cell proliferation and metastasis via miR-100/mTOR/miR-143 pathway. Int. J. Pharm. 627, 122214. doi:10.1016/j.ijpharm.2022.122214
Jiao Y., Wang X., Zhang J., Qi Y., Gong H., Jiang D. (2017). Inhibiting function of human fetal dermal mesenchymal stem cells on bioactivities of keloid fibroblasts. Stem Cell Res. Ther. 8, 170. doi:10.1186/s13287-017-0624-0
Kalluri R., LeBleu V. S. (2020). The biology, function, and biomedical applications of exosomes. Science 367, eaau6977. doi:10.1126/science.aau6977
Korntner S., Lehner C., Gehwolf R., Wagner A., Grütz M., Kunkel N., et al. (2019). Limiting angiogenesis to modulate scar formation. Adv. Drug Deliv. Rev. 146, 170–189. doi:10.1016/j.addr.2018.02.010
Lee J.-K., Park S.-R., Jung B.-K., Jeon Y.-K., Lee Y.-S., Kim M.-K., et al. (2013). Exosomes derived from mesenchymal stem cells suppress angiogenesis by down-regulating VEGF expression in breast cancer cells. PLoS One 8, e84256. doi:10.1371/journal.pone.0084256
Li J., Li Z., Wang S., Bi J., Huo R. (2022a). Exosomes from human adipose-derived mesenchymal stem cells inhibit production of extracellular matrix in keloid fibroblasts via downregulating transforming growth factor-β2 and Notch-1 expression. Bioengineered 13, 8515–8525. doi:10.1080/21655979.2022.2051838
Li L., Ngo H. T. T., Hwang E., Wei X., Liu Y., Liu J., et al. (2019). Conditioned medium from human adipose-derived mesenchymal stem cell culture prevents UVB-induced skin aging in human keratinocytes and dermal fibroblasts. Int. J. Mol. Sci. 21, 49. doi:10.3390/ijms21010049
Li N., Wang B. (2022). Suppressive effects of umbilical cord mesenchymal stem cell-derived exosomal miR-15a-5p on the progression of cholangiocarcinoma by inhibiting CHEK1 expression. Cell Death Discov. 8, 205. doi:10.1038/s41420-022-00932-7
Li Q., Fang L., Chen J., Zhou S., Zhou K., Cheng F., et al. (2021a). Exosomal MicroRNA-21 promotes keloid fibroblast proliferation and collagen production by inhibiting Smad7. J. Burn Care Res. 42, 1266–1274. doi:10.1093/jbcr/irab116
Li Y., Shen Z., Jiang X., Wang Y., Yang Z., Mao Y., et al. (2022b). Mouse mesenchymal stem cell-derived exosomal miR-466f-3p reverses EMT process through inhibiting AKT/GSK3β pathway via c-MET in radiation-induced lung injury. J. Exp. Clin. Cancer Res. CR 41, 128. doi:10.1186/s13046-022-02351-z
Li Y., Zhang J., Shi J., Liu K., Wang X., Jia Y., et al. (2021b). Exosomes derived from human adipose mesenchymal stem cells attenuate hypertrophic scar fibrosis by miR-192-5p/IL-17RA/Smad axis. Stem Cell Res. Ther. 12, 221. doi:10.1186/s13287-021-02290-0
Lian N., Li T. (2016). Growth factor pathways in hypertrophic scars: molecular pathogenesis and therapeutic implications. Biomed. Pharmacother. 84, 42–50. doi:10.1016/j.biopha.2016.09.010
Limandjaja G. C., Niessen F. B., Scheper R. J., Gibbs S. (2020). The keloid disorder: heterogeneity, histopathology, mechanisms and models. Front. Cell Dev. Biol. 8, 360. doi:10.3389/fcell.2020.00360
Lin H., Chen H., Zhao X., Chen Z., Zhang P., Tian Y., et al. (2021). Advances in mesenchymal stem cell conditioned medium-mediated periodontal tissue regeneration. J. Transl. Med. 19, 456. doi:10.1186/s12967-021-03125-5
Liu J., Ren J., Su L., Cheng S., Zhou J., Ye X., et al. (2018). Human adipose tissue-derived stem cells inhibit the activity of keloid fibroblasts and fibrosis in a keloid model by paracrine signaling. Burns 44, 370–385. doi:10.1016/j.burns.2017.08.017
Maas S. L. N., de Vrij J., van der Vlist E. J., Geragousian B., van Bloois L., Mastrobattista E., et al. (2015). Possibilities and limitations of current technologies for quantification of biological extracellular vesicles and synthetic mimics. J. Control Release 200, 87–96. doi:10.1016/j.jconrel.2014.12.041
McNamara R. P., Caro-Vegas C. P., Costantini L. M., Landis J. T., Griffith J. D., Damania B. A., et al. (2018). Large-scale, cross-flow based isolation of highly pure and endocytosis-competent extracellular vesicles. J. Extracell. Vesicles 7, 1541396. doi:10.1080/20013078.2018.1541396
Mohamed-Ahmed S., Fristad I., Lie S. A., Suliman S., Mustafa K., Vindenes H., et al. (2018). Adipose-derived and bone marrow mesenchymal stem cells: A donor-matched comparison. Stem Cell Res. Ther. 9, 168. doi:10.1186/s13287-018-0914-1
Murao N., Seino K.-I., Hayashi T., Ikeda M., Funayama E., Furukawa H., et al. (2014). Treg-enriched CD4+ T cells attenuate collagen synthesis in keloid fibroblasts. Exp. Dermatol 23, 266–271. doi:10.1111/exd.12368
Monaco J. L., Lawrence W. T. (2003). Acute wound healing an overview. Clin. Plast. Surg. 30 (1), 1–12. doi:10.1016/s0094-1298(02)00070-6
Nakamura Y., Wang X., Xu C., Asakura A., Yoshiyama M., From A. H. L., et al. (2007). Xenotransplantation of long-term-cultured swine bone marrow-derived mesenchymal stem cells. Stem Cells 25, 612–620. doi:10.1634/stemcells.2006-0168
Ogawa R. (2017). Keloid and hypertrophic scars are the result of chronic inflammation in the reticular dermis. Int. J. Mol. Sci. 18, 606. doi:10.3390/ijms18030606
Onodera M., Ueno M., Ito O., Suzuki S., Igawa H. H., Sakamoto H. (2007). Factor XIIIa-positive dermal dendritic cells in keloids and hypertrophic and mature scars. Pathol. Int. 57, 337–342. doi:10.1111/j.1440-1827.2007.02105.x
Pakravan K., Babashah S., Sadeghizadeh M., Mowla S. J., Mossahebi-Mohammadi M., Ataei F., et al. (2017). MicroRNA-100 shuttled by mesenchymal stem cell-derived exosomes suppresses in vitro angiogenesis through modulating the mTOR/HIF-1α/VEGF signaling axis in breast cancer cells. Cell Oncol. (Dordr) 40, 457–470. doi:10.1007/s13402-017-0335-7
Pisitkun T., Shen R.-F., Knepper M. A. (2004). Identification and proteomic profiling of exosomes in human urine. Proc. Natl. Acad. Sci. U. S. A. 101, 13368–13373. doi:10.1073/pnas.0403453101
Pospichalova V., Svoboda J., Dave Z., Kotrbova A., Kaiser K., Klemova D., et al. (2015). Simplified protocol for flow cytometry analysis of fluorescently labeled exosomes and microvesicles using dedicated flow cytometer. J. Extracell. Vesicles 4, 25530. doi:10.3402/jev.v4.25530
Praveen Kumar L., Kandoi S., Misra R., Vijayalakshmi S., Rajagopal K., Verma R. S. (2019). The mesenchymal stem cell secretome: A new paradigm towards cell-free therapeutic mode in regenerative medicine. Cytokine Growth Factor Rev. 46, 1–9. doi:10.1016/j.cytogfr.2019.04.002
Rasulov M. F., Vasilchenkov A. V., Onishchenko N. A., Krasheninnikov M. E., Kravchenko V. I., Gorshenin T. L., et al. (2005). First experience of the use bone marrow mesenchymal stem cells for the treatment of a patient with deep skin burns. Bull. Exp. Biol. Med. 139, 141–144. doi:10.1007/s10517-005-0232-3
Ren S., Chen J., Guo J., Liu Y., Xiong H., Jing B., et al. (2022). Exosomes from adipose stem cells promote diabetic wound healing through the eHSP90/LRP1/AKT Axis. Cells 11, 3229. doi:10.3390/cells11203229
Rider M. A., Hurwitz S. N., Meckes D. G. (2016). ExtraPEG: A polyethylene glycol-based method for enrichment of extracellular vesicles. Sci. Rep. 6, 23978. doi:10.1038/srep23978
Sato C., Yamamoto Y., Funayama E., Furukawa H., Oyama A., Murao N., et al. (2018). Conditioned medium obtained from amnion-derived mesenchymal stem cell culture prevents activation of keloid fibroblasts. Plast. Reconstr. Surg. 141, 390–398. doi:10.1097/PRS.0000000000004068
Shahir M., Mahmoud Hashemi S., Asadirad A., Varahram M., Kazempour-Dizaji M., Folkerts G., et al. (2020). Effect of mesenchymal stem cell-derived exosomes on the induction of mouse tolerogenic dendritic cells. J. Cell Physiol. 235, 7043–7055. doi:10.1002/jcp.29601
Shao H., Im H., Castro C. M., Breakefield X., Weissleder R., Lee H. (2018). New technologies for analysis of extracellular vesicles. Chem. Rev. 118, 1917–1950. doi:10.1021/acs.chemrev.7b00534
Shen Z., Shao J., Sun J., Xu J. (2022). Exosomes released by melanocytes modulate fibroblasts to promote keloid formation: A pilot study. J. Zhejiang Univ. Sci. B 23, 699–704. doi:10.1631/jzus.B2200036
Shi S., Zhang Q., Xia Y., You B., Shan Y., Bao L., et al. (2016). Mesenchymal stem cell-derived exosomes facilitate nasopharyngeal carcinoma progression. Am. J. Cancer Res. 6, 459–472.
Sun W., Yan S., Yang C., Yang J., Wang H., Li C., et al. (2022). Mesenchymal stem cells-derived exosomes ameliorate lupus by inducing M2 macrophage polarization and regulatory T cell expansion in MRL/lpr mice. Immunol. Invest. 51, 1785–1803. doi:10.1080/08820139.2022.2055478
Talbott H. E., Mascharak S., Griffin M., Wan D. C., Longaker M. T. (2022). Wound healing, fibroblast heterogeneity, and fibrosis. Cell Stem Cell 29, 1161–1180. doi:10.1016/j.stem.2022.07.006
Tauro B. J., Greening D. W., Mathias R. A., Ji H., Mathivanan S., Scott A. M., et al. (2012). Comparison of ultracentrifugation, density gradient separation, and immunoaffinity capture methods for isolating human colon cancer cell line LIM1863-derived exosomes. Methods 56, 293–304. doi:10.1016/j.ymeth.2012.01.002
Théry C., Witwer K. W., Aikawa E., Alcaraz M. J., Anderson J. D., Andriantsitohaina R., et al. (2018). Minimal information for studies of extracellular vesicles 2018 (MISEV2018): A position statement of the international society for extracellular vesicles and update of the MISEV2014 guidelines. J. Extracell. Vesicles 7, 1535750. doi:10.1080/20013078.2018.1535750
Tokhanbigli S., Baghaei K., Asadirad A., Hashemi S. M., Asadzadeh-Aghdaei H., Zali M. R. (2019). Immunoregulatory impact of human mesenchymal-conditioned media and mesenchymal derived exosomes on monocytes. Mol. Biol. Res. Commun. 8, 79–89. doi:10.22099/mbrc.2019.33346.1397
Viallard C., Larrivée B. (2017). Tumor angiogenesis and vascular normalization: alternative therapeutic targets. Angiogenesis 20, 409–426. doi:10.1007/s10456-017-9562-9
Wang Q., Zhong Y., Li Z., Zhu D., Lu H., Chen P., et al. (2022). Multitranscriptome analyses of keloid fibroblasts reveal the role of the HIF-1α/HOXC6/ERK axis in keloid development. Burns Trauma 10, tkac013. doi:10.1093/burnst/tkac013
Wang X., Ma Y., Gao Z., Yang J. (2018). Human adipose-derived stem cells inhibit bioactivity of keloid fibroblasts. Stem Cell Res. Ther. 9, 40. doi:10.1186/s13287-018-0786-4
Wang Z., He Z., Liang S., Yang Q., Cheng P., Chen A. (2020). Comprehensive proteomic analysis of exosomes derived from human bone marrow, adipose tissue, and umbilical cord mesenchymal stem cells. Stem Cell Res. Ther. 11, 511. doi:10.1186/s13287-020-02032-8
Wang Z., Yan X. (2013). CD146, a multi-functional molecule beyond adhesion. Cancer Lett. 330, 150–162. doi:10.1016/j.canlet.2012.11.049
Wang L., Hu L., Zhou X., Xiong Z., Zhang C., Shehada H., et al. (2017). Exosomes secreted by human adipose mesenchymal stem cells promote scarless cutaneous repair by regulating extracellular matrix remodelling. Sci. Rep. 7, 13321. doi:10.1038/s41598-017-12919-x
Wu Z.-Y., Zhang H.-J., Zhou Z.-H., Li Z.-P., Liao S.-M., Wu Z.-Y., et al. (2021). The effect of inhibiting exosomes derived from adipose-derived stem cells via the TGF-β1/Smad pathway on the fibrosis of keloid fibroblasts. Gland. Surg. 10, 1046–1056. doi:10.21037/gs-21-4
Xia Y., Wang Y., Shan M., Hao Y., Liu H., Chen Q., et al. (2022). Advances in the pathogenesis and clinical application prospects of tumor biomolecules in keloid. Burns Trauma 10, tkac025. doi:10.1093/burnst/tkac025
Xu Y., Huang S., Fu X. (2012). Autologous transplantation of bone marrow-derived mesenchymal stem cells: A promising therapeutic strategy for prevention of skin-graft contraction. Clin. Exp. Dermatol 37, 497–500. doi:10.1111/j.1365-2230.2011.04260.x
Xue J., Sun N., Liu Y. (2022). Self-assembled nano-peptide hydrogels with human umbilical cord mesenchymal stem cell spheroids accelerate diabetic skin wound healing by inhibiting inflammation and promoting angiogenesis. Int. J. Nanomedicine 17, 2459–2474. doi:10.2147/IJN.S363777
Yang J., Li S., He L., Chen M. (2021). Adipose-derived stem cells inhibit dermal fibroblast growth and induce apoptosis in keloids through the arachidonic acid-derived cyclooxygenase-2/prostaglandin E2 cascade by paracrine. Burns Trauma 9, tkab020. doi:10.1093/burnst/tkab020
Yuan R., Dai X., Li Y., Li C., Liu L. (2021). Exosomes from miR-29a-modified adipose-derived mesenchymal stem cells reduce excessive scar formation by inhibiting TGF-β2/Smad3 signaling. Mol. Med. Rep. 24, 758. doi:10.3892/mmr.2021.12398
Zhang C., Wang T., Zhang L., Chen P., Tang S., Chen A., et al. (2021). Combination of lyophilized adipose-derived stem cell concentrated conditioned medium and polysaccharide hydrogel in the inhibition of hypertrophic scarring. Stem Cell Res. Ther. 12, 23. doi:10.1186/s13287-020-02061-3
Zhang H., Wang H., Jiang C., Wang H., Xia Y., Shen D., et al. (2022a). Fractional laser-assisted administration of human umbilical cord mesenchymal stem cells to reduce hypertrophic scars in rabbit ears. Lasers Surg. Med. 54, 554–564. doi:10.1002/lsm.23508
Zhang Q., Kelly A. P., Wang L., French S. W., Tang X., Duong H. S., et al. (2006). Green tea extract and (-)-epigallocatechin-3-gallate inhibit mast cell-stimulated type I collagen expression in keloid fibroblasts via blocking PI-3K/AkT signaling pathways. J. Invest. Dermatol 126, 2607–2613. doi:10.1038/sj.jid.5700472
Zhang W., Bai X., Zhao B., Li Y., Zhang Y., Li Z., et al. (2018). Cell-free therapy based on adipose tissue stem cell-derived exosomes promotes wound healing via the PI3K/Akt signaling pathway. Exp. Cell Res. 370, 333–342. doi:10.1016/j.yexcr.2018.06.035
Zhang Y., Bi J., Huang J., Tang Y., Du S., Li P. (2020). Exosome: A review of its classification, isolation techniques, storage, diagnostic and targeted therapy applications. Int. J. Nanomedicine 15, 6917–6934. doi:10.2147/IJN.S264498
Zhang Y., Lai X., Yue Q., Cao F., Zhang Y., Sun Y., et al. (2022b). Bone marrow mesenchymal stem cells-derived exosomal microRNA-16-5p restrains epithelial-mesenchymal transition in breast cancer cells via EPHA1/NF-κB signaling axis. Genomics 114, 110341. doi:10.1016/j.ygeno.2022.110341
Zhao B., Shi X., Feng D., Han J., Hu D. (2023). MicroRNA let-7d attenuates hypertrophic scar fibrosis through modulation of iron metabolism by reducing DMT1 expression. J. Mol. Histol. 54, 77–87. doi:10.1007/s10735-023-10113-0
Zhao B., Zhang Y., Han S., Zhang W., Zhou Q., Guan H., et al. (2017). Exosomes derived from human amniotic epithelial cells accelerate wound healing and inhibit scar formation. J. Mol. Histol. 48, 121–132. doi:10.1007/s10735-017-9711-x
Zhou P., Li X., Zhang B., Shi Q., Li D., Ju X. (2019a). A human umbilical cord mesenchymal stem cell-conditioned medium/chitosan/collagen/β-glycerophosphate thermosensitive hydrogel promotes burn injury healing in mice. Biomed. Res. Int. 2019, 5768285. doi:10.1155/2019/5768285
Zhou X., Cao H., Guo J., Yuan Y., Ni G. (2022). Effects of BMSC-derived EVs on bone metabolism. Pharmaceutics 14, 1012. doi:10.3390/pharmaceutics14051012
Zhou X., Li T., Chen Y., Zhang N., Wang P., Liang Y., et al. (2019b). Mesenchymal stem cell-derived extracellular vesicles promote the in vitro proliferation and migration of breast cancer cells through the activation of the ERK pathway. Int. J. Oncol. 54, 1843–1852. doi:10.3892/ijo.2019.4747
Zhou Y., Zhao B., Zhang X.-L., Lu Y., Lu S.-T., Cheng J., et al. (2021). Combined topical and systemic administration with human adipose-derived mesenchymal stem cells (hADSC) and hADSC-derived exosomes markedly promoted cutaneous wound healing and regeneration. Stem Cell Res. Ther. 12, 257. doi:10.1186/s13287-021-02287-9
Zhu W., Huang L., Li Y., Zhang X., Gu J., Yan Y., et al. (2012). Exosomes derived from human bone marrow mesenchymal stem cells promote tumor growth in vivo. Cancer Lett. 315, 28–37. doi:10.1016/j.canlet.2011.10.002
Zhu Z., Chen B., Peng L., Gao S., Guo J., Zhu X. (2021). Blockade of LINC01605-enriched exosome generation in M2 macrophages impairs M2 macrophage-induced proliferation, migration, and invasion of human dermal fibroblasts. Int. J. Immunopathol. Pharmacol. 35, 20587384211016724. doi:10.1177/20587384211016724
Keywords: exosomes, conditioned medium, mesenchymal stem cell, keloid, hypertrophic scar
Citation: Zhong Y, Zhang Y, Yu A, Zhang Z, Deng Z, Xiong K, Wang Q and Zhang J (2023) Therapeutic role of exosomes and conditioned medium in keloid and hypertrophic scar and possible mechanisms. Front. Physiol. 14:1247734. doi: 10.3389/fphys.2023.1247734
Received: 26 June 2023; Accepted: 29 August 2023;
Published: 12 September 2023.
Edited by:
John Connelly, Queen Mary University of London, United KingdomReviewed by:
Georgios Theocharidis, Beth Israel Deaconess Medical Center and Harvard Medical School, United StatesJong Hee Lee, Sungkyunkwan University, Republic of Korea
Copyright © 2023 Zhong, Zhang, Yu, Zhang, Deng, Xiong, Wang and Zhang. This is an open-access article distributed under the terms of the Creative Commons Attribution License (CC BY). The use, distribution or reproduction in other forums is permitted, provided the original author(s) and the copyright owner(s) are credited and that the original publication in this journal is cited, in accordance with accepted academic practice. No use, distribution or reproduction is permitted which does not comply with these terms.
*Correspondence: Yixiu Zhong, 846783501@qq.com; Qi Wang, w8614@hotmail.com; Jianglin Zhang, zhang.jianglin@szhospital.com