- 1College of Physical Education and Health Sciences, Zhejiang Normal University, Jinhua, China
- 2College of Education, Anyang Normal University, Anyang, China
- 3Exercise and Metabolism Research Center, College of Physical Education and Health Sciences, Zhejiang Normal University, Jinhua, China
Purpose: In this systematic review and meta-analysis, blood flow restriction (BFR) with low-load resistance training (BFR-RT) was compared with high-load resistance training (HL-RT) on muscle strength in healthy adults. The characteristics of cuff pressure suitable for muscle strength gain were also investigated by analyzing the effects of applying different occlusion pressure prescriptions and cuff inflation patterns on muscle strength gain.
Methods: Literature search was conducted using PubMed, Ovid Medline, ProQuest, Cochrane Library, Embase, and Scopus databases to identify literature published until May 2023. Studies reporting the effects of BFR-RT interventions on muscle strength gain were compared with those of HL-RT. The risk of bias in the included trials was assessed using the Cochrane tool, followed by a meta-analysis to calculate the combined effect. Subgroup analysis was performed to explore the beneficial variables.
Results: Nineteen articles (42 outcomes), with a total of 458 healthy adults, were included in the meta-analysis. The combined effect showed higher muscle strength gain with HL-RT than with BFR-RT (p = 0.03, SMD = −0.16, 95% CI: −0.30 to −0.01). The results of the subgroup analysis showed that the BFR-RT applied with incremental and individualized pressure achieved muscle strength gain similar to the HL-RT (p = 0.8, SMD = −0.05, 95% CI: −0.44 to 0.34; p = 0.68, SMD = −0.04, 95% CI: −0.23 to 0.15), but muscle strength gain obtained via BFR-RT applied with absolute pressure was lower than that of HL-RT (p < 0.05, SMD = −0.45, 95% CI: −0.71 to −0.19). Furthermore, muscle strength gain obtained by BFR-RT applied with intermittent pressure was similar to that obtained by HL-RT (p = 0.88, SMD = −0.02, 95% CI: −0.27 to 0.23), but muscle strength gain for BFR-RT applied with continuous pressure showed a less prominent increase than that for HL-RT (p < 0.05, SMD = −0.3, 95% CI: −0.48 to −0.11).
Conclusion: In general, HL-RT produces superior muscle strength gains than BFR-RT. However, the application of individualized, incremental, and intermittent pressure exercise protocols in BFR-RT elicits comparable muscle strength gains to HL-RT. Our findings indicate that cuff pressure characteristics play a significant role in establishing a BFR-RT intervention program for enhancing muscle strength in healthy adults.
Clinical Trial Registration: https://www.crd.york.ac.uk/PROSPERO/#recordDetails; Identifier: PROSPERO (CRD42022364934).
1 Introduction
Muscle strength is important for normal survival and daily living. It is also considered an important predictor of cardiometabolic risk and is associated with morbidity in adults and older adults (Silventoinen et al., 2009). Studies have identified low grip strength as a potential risk factor for skeletal sarcopenia, functional limitation, and disability (Rantanen et al., 1999; Manini and Clark, 2012); it is also considered a valuable indicator of frailty in older adults (Takahashi et al., 2017). In addition, a meta-analysis study of data from approximately 20,000 men and women associated high levels of upper and lower limb muscle strength with a reduced risk of mortality in adults (García-Hermoso et al., 2018). Another follow-up study over a period of 33 years showed a negative association between muscle strength and all-cause mortality (Stenholm et al., 2014). This correlation was also observed in people with specific diseases, including cardiovascular disease, peripheral arterial disease, cancer, renal failure, chronic occlusive lung disease, and rheumatoid arthritis, and among critically ill patients (Jochem et al., 2019). Therefore, muscle strength can be regarded as a potential predictor of morbidity and mortality risk in the general population (Leon et al., 2005; Peterson and Krishnan, 2015), and it plays a key role in protecting human health.
The American College of Sports Medicine previously suggested that the use of high-load resistance training [(HL-RT, ≥70% 1RM)] is an effective means of promoting muscle strength gain (Garber et al., 2011). However, this training method has drawbacks, with HL-RT exercise increasing the likelihood of sports-related injuries. Furthermore, for patients undergoing rehabilitation, HL-RT exercise is not recommended in the early stages of recovery, as it may increase the risk of reinjury (Hughes et al., 2019). In elderly individuals, the presence of comorbidities, such as coronary heart disease, diabetes, or musculoskeletal injuries, may further complicate the use of HL-RT (Papa et al., 2017). However, low-load exhaustive exercise, while also effective in improving muscle strength, may cause subjective discomfort in older adults and rehabilitating patients. Moreover, prolonged recovery time from fatigue may lead to red blood cell abnormalities, increasing the likelihood of tissue damage or the exacerbation of existing injuries (Brun et al., 1994). Emerging blood flow restriction (BFR) training with low-load resistance training (BFR-RT) techniques can circumvent these problems.
BFR training, also known as KAATSU training or vascular occlusion training, refers to the external compression of the limb during exercise by using a special compression device that causes venous occlusion and partial arterial occlusion to improve the training effect (Sato, 2005; Loenneke et al., 2014). BFR-RT produces a local metabolic crisis via physical compression, which induces the brain to secrete growth hormones to promote anabolism, improve muscle strength, and accelerate tissue repair (Fry et al., 2010). Common practice dictates that more muscle strength gain can be obtained with BFR-RT than with low-load resistance training. However, controversy arises when BFR-RT is compared with HL-RT, which is known as the gold standard for increasing muscle strength. For example, some studies have shown that BFR-RT training can produce muscle strength gain similar to HL-RT (Libardi et al., 2015; Centner et al., 2022; Chang et al., 2022), whereas other studies have shown that BFR-RT training leads to less muscle strength gain than HL-RT (Lixandrao et al., 2018; Sieljacks et al., 2019).
It is necessary to discuss these controversies based on cuff pressure characteristics. A meta-analysis explored the effect of using different occlusion pressure prescriptions (individualized and non-individualized pressures) on muscle strength gain during BFR-RT exercise, results indicated that the BFR-RT group demonstrated lower muscle strength gain than the HL-RT group, even after controlling for the abovementioned factors (Lixandrao et al., 2018). Interestingly, however, Individualized pressure appears to be widely accepted owing to its logical basis, but it does not justify the choice of individualized pressure when setting up a BFR-RT exercise program, and more theoretical support needs to be found for the use of individualized pressure for BFR-RT (Clarkson et al., 2020). Another related meta-analysis (Sinclair et al., 2022) described that the cuff inflation pattern (continuous and intermittent pressure) was not a variable affecting muscle strength gain, but the results of the analysis were limited to two articles, entailed limited guidance value in terms of enhancing muscle strength with BFR-RT interventions that use different cuff inflation patterns. In addition. To our knowledge, no meta-analysis has yet considered the effects of BFR-RT occlusion pressure prescription, cuff inflation mode, and participant characteristics on muscle strength adaptation simultaneously.
Therefore, the objectives of our study were threefold. Firstly, we aimed to examine the adaptation of muscle strength in individuals undergoing BFR-RT compared to those undergoing HL-RT. Secondly, we aimed to analyze the impact of occlusion pressure prescription and cuff inflation pattern on the adaptation of muscle strength. Lastly, we sought to investigate the cuff pressure characteristics that are suitable for promoting muscle strength adaptation in diverse participants.
2 Methods
The systematic review was performed according to the latest guidelines of Preferred Reporting Items for Systematic Reviews and Meta-Analyses (PRISMA) (Page et al., 2021). This study was also registered with PROSPERO (CRD42022364934).
2.1 Search strategy
A systematic literature search was conducted by two researchers (HC and GL) to identify relevant studies. The following electronic databases were searched from database creation to 31 December 2022: PubMed, Ovid Medline, ProQuest, Cochrane Library, Embase, and Scopus. The following specific keywords were used to search the articles: “blood flow restriction training” OR “Kaatsu training” OR “occlusion training” OR “vascular occlusion” OR “KAATSU” OR “blood flow occlusion” AND “muscle strength” OR “muscle force” OR “dynamic” OR “isokinetic” OR “muscle power” AND “randomized controlled trial” OR “RCT.” We conducted an additional search on 20 May 2023, to identify potential studies published since the last search. The detailed search strategy implemented in the PubMed database is provided in Supplementary Table S1. In addition, we manually searched the lists of references to obtain other suitable articles.
2.2 Inclusion and exclusion criteria
The selection criteria were established based on Participants, Interventions, Control, Outcome, and Study design (PICOS).
Before inclusion, the titles and abstracts of the retrieved articles were screened for relevance. Subsequently, the full texts of the articles were obtained and reviewed based on the inclusion criteria. To determine the articles to be included in this study, we adopted the following inclusion criteria: 1) participants: healthy adults (age ≥18 years); 2) control group [resistance training without vascular occlusion (HL-RT) at ≥70% 1RM] and intervention group [resistance training with vascular occlusion (BFR-RT) at <50% 1RM)]; 3) outcome: before the start of the experiment and at the end of the experiment to assess muscle strength (i.e., dynamic, isometric, or isokinetic test); and 4) study design: the studies should be RCTs with parallel-groups. We excluded studies based on the following criteria: 1) studies conducted with animals as subjects; 2) trials involving drug supplements affecting muscle strength; 3) the experimental group and the control group were the left and right sides of the experimental subject; 4) non-original research articles (experimental protocols, meta-analyses, and systematic reviews); and 5) articles not published in English. Relevant articles were reviewed and assessed independently by two authors (HC and GL) according to the inclusion/exclusion criteria. First, the titles and abstracts of the identified articles were screened for relevance. Second, the full text of specific articles was obtained, and the full text of each article was fully assessed against the inclusion criteria. Any discrepancies were resolved by discussion with another author (JZ) to reach a final consensus. The detailed study selection process according to the PRISMA guidelines is shown in Figure 1.
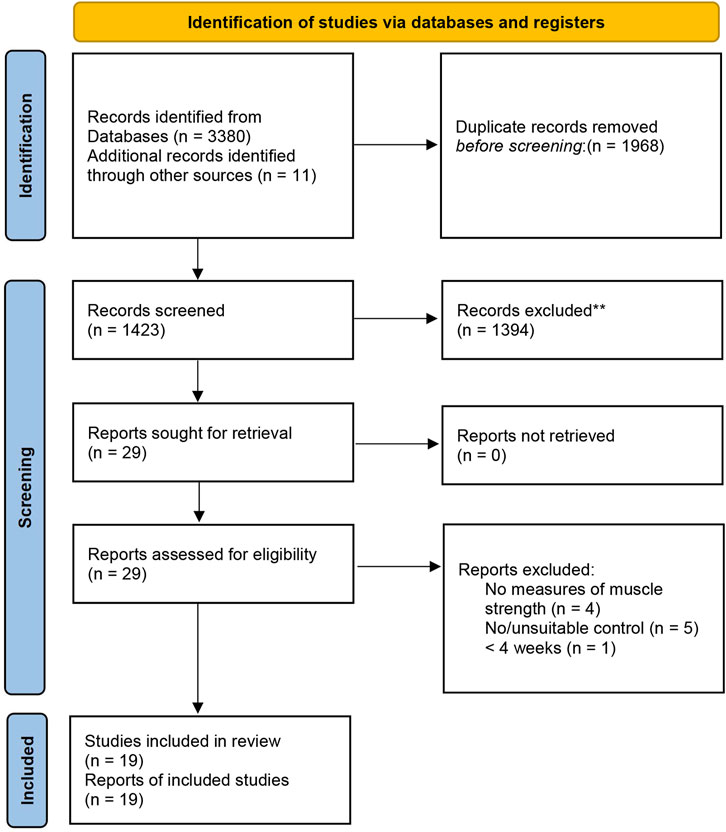
FIGURE 1. Flowchart of the study selection according to the latest Preferred Reporting Items for Systematic Review and Meta-Analysis (PRISMA).
2.3 Outcome measures and data extraction
The study characteristics were extracted independently by two authors (CH and JY) by using an Excel spreadsheet. The following characteristics were extracted from each article: 1) surname of the first author; 2) characteristics of participants (number and gender); 3) exercise/intervention characteristics (exercise load, frequency, and duration); 4) muscle strength tests (i.e., dynamic, isometric, and isokinetic); and 5) proportion of muscle strength gain and comparison between experimental and control groups. When the article provided data in graph format, the data were extracted via the Web Plot Digitizer. The percentage change in muscle strength [((Meanpost − Meanpre)/Meanpre) × 100] was calculated for each study. In the case of multiple assessment methods, the minimum and maximum mean values for each method were reported (Table 1). Notably, in the assessment of post-intervention muscle strength across multiple periods, the analyses were based on the last available time point. In case of any disagreement between the two authors regarding the included features, a third reviewer (JZ) was consulted. Finally, the extracted features were cross-checked and analyzed in depth by the other authors. To obtain missing or additional data, the corresponding author was contacted.
2.4 Quality assessment for the included trials
The Cochrane risk of bias tool (Higgins et al., 2011) was used in this study. The tool includes the following items: random sequence generation/assignment concealment (selection bias), subject/person blinding (performance bias), outcome assessment blinding (detection bias), incomplete outcome data (attrition bias), selective reporting (reporting bias), and other biases. The quality of each domain was rated as “low risk,” “high risk,” or “unclear” and was indicated by green (+), red (−), and yellow (?) colors and symbols. The quality of the trial was assessed by two reviewers (HC and JY). In case of disagreement, consensus was reached through discussion. Persistent differences were resolved by discussion with a third reviewer (JZ) to reach a consensus.
2.5 Statistical analysis
Review Manager (RevMan 5.4., Copenhagen, Denmark) was used to statistically analyze the effect of BFR-RT intervention on muscle strength gain in healthy adults. Due to significant differences between the measurement tools and units of each outcome index, the standardized mean differences (SMDs) and 95% confidence intervals (95% CIs) were calculated using the random effect model to summarize the outcome indicators. The I2 statistic was used to determine the heterogeneity. For each comparison, the pooled effect size (ES) calculated, and the alpha level was set at p < 0.05. Data are reported as mean ± standard deviation. The I2 was labeled as having low heterogeneity when the value was less than 25%, moderate heterogeneity when the value was between 25% and 75%, and high heterogeneity when the value was greater than 75%.
This research first compared the effects of the BFR-RT and HL-RT groups in terms of muscle strength gain. A subgroup analysis of cuff pressure characteristics (occlusion pressure prescription and cuff inflatable pattern) was also conducted to examine the effect of different occlusion pressure prescriptions (individualized, incremental, and absolute pressures) and cuff inflatable patterns (continuous and intermittent pressures) on muscle strength gain. The inclusion criteria for subgroups are shown in Supplementary Table S2. On this basis, we further analyzed the effect of participants’ age and gender on subgroup outcomes. In addition, also used Origin (Origin 2021; Massachusetts, United States) to draw 3D images to investigate the combined effect on muscle strength gain.
The sensitivity analysis was performed via a meta-analysis after removing each study to determine if any of the studies were biased against the combined results. A study was considered to bias the pooled results when the estimate after the removal of a study exceeded the 95% CI for the joint effect. An Egger test was performed using Stata version 12 (StataCorp., College Station, TX, United States), and a funnel plot was constructed to check for potential bias in the included RCTs (Supplementary Figure S1).
3 Results
3.1 Search results and selection of studies
A total of 3391 articles were identified in the systematic search. Among them, 3380 articles were from electronic databases (PubMed, Ovid Medline, ProQuest, Cochrane Library, Embase, and Scopus databases), and 11 articles were from other sources (i.e., manual searches of the included reference lists of included studies and related reviews). After removing duplicates (1968 articles), 1423 articles were obtained for subsequent evaluation. A careful review of the titles and abstracts of the 1423 articles led to the exclusion of an additional 1394 articles. Finally, the full texts of the remaining articles were thoroughly checked. Nineteen articles met the requirements of the qualitative analysis. Figure 1 shows the process flowchart.
3.2 Summary of the included studies
3.2.1 Study characteristics and participants
19 articles were selected for analysis based on the inclusion and exclusion criteria, all of which were published between 2010 and 2023. Among them, 19 articles had subjects with no training experience or no recent (≥2 months) systematic resistance training. We included a total of 458 subjects, 257 in the HL-RT group and 201 in the BFR-RT group. Of these, 2 articles had female subjects, totaling 46 and they’re all elderly, with a mean age of 64.54 years; 12 articles had male subjects, totaling 310, ranging in age from 18 to 64 years, with a mean age of 27.82 years; and 3 articles that did not differentiate between the genders of the subjects included 45 males and 23 females, all of whom were young adults, with an average age of 23.02 years; 2 articles failed to provide information regarding the participants’ gender, encompassing a total of 34 individuals who were all elderly adults, with a mean age of 64.37 years.
3.2.2 Intervention characteristics
The exercise load in all articles was between 20% and 40% 1RM for the BFR-RT group and between 70% and 90% 1RM for the HL-RT group. 6 articles had a training frequency of 2 days/week, 11 articles had a training frequency of 3 days/week, 1 article had a training frequency of 4 days/week, and 1 article had a training frequency of 5 days/week. The overall training period was 4–16 weeks 12 articles showed that BFR-RT produced comparable muscle strength gain to HL-RT (Clark et al., 2011; Laurentino et al., 2012; Ozaki et al., 2013; Thiebaud et al., 2013; Libardi et al., 2015; Letieri et al., 2018; Centner et al., 2019; Mendonca et al., 2021; Bemben et al., 2022; Centner et al., 2022; Centner et al., 2023; Horiuchi et al., 2023); 6 articles showed that BFR-RT produced lower muscle strength gain than HL-RT (Karabulut et al., 2010; Yasuda et al., 2011; Martín-Hernández et al., 2013; Lixandrão et al., 2015; Vechin et al., 2015; Brandner et al., 2019), and 1 article showed that BFR-RT could lead to greater muscle strength gain than HL-RT (Laswati et al., 2018). Of all the articles, occlusion pressure prescription applied individualized pressure in 10 articles, incremental pressure in 5 articles, and absolute pressure in 5 articles; inflation mode applied intermittent pressure in 7 articles and continuous pressure in 13 articles. Table 1 and Supplementary Table S5 show the population characteristics and exercise characteristics.
3.3 Summary of risk of bias
The included articles were assessed using the Cochrane risk of bias tool (Figure 2; Figure 3). Regarding the selection bias, 12 articles indicated a low risk of random sequence generation, 4 articles did not report whether allocation concealment was performed, and 1 article is high risk. The blinding of subjects and researchers may not be feasible given that BFR-RT was the primary intervention in all trials; this approach resulted in a high risk of performance bias in articles. Despite reporting a high risk of performance bias, the test quality was unaffected, and 2 articles were considered to have measurement bias. Overall, no studies had reporting bias, and there were no other forms of bias in 15 articles.
3.4 Results of the meta-analysis
3.4.1 Muscle strength adaptation: BFR-RT vs HL-RT
We included 19 articles (including 42 outcomes) for meta-analysis to compare the differences in muscle strength gain between the BFR-RT and HL-RT groups. The combined SMD showed low heterogeneity (I2 = 9%, p = 0.31). Figure 4 shows the pooled results of the meta-analysis. Statistically significant muscle strength gain was obtained in the HL-RT group compared with the BFR-RT group (SMD = −0.16, 95% CI: −0.30 to −0.01, p < 0.05). The heterogeneity of the muscle strength gain suggests the effect of different BFR-RT exercise protocols on muscle strength gain in healthy adults.
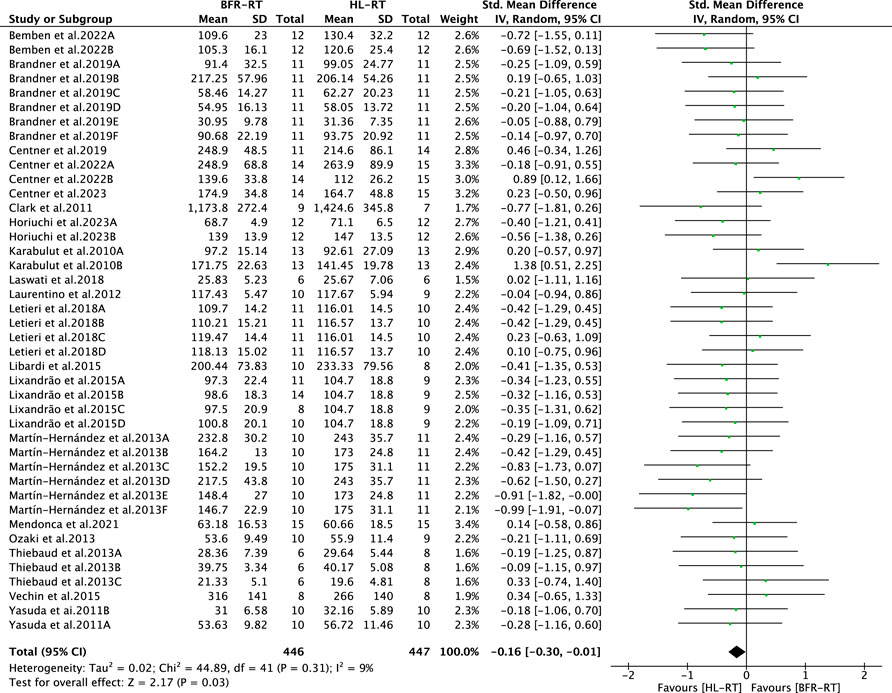
FIGURE 4. Summary results of the effect of the BFR-RT intervention on muscle strength. Different letters in the same study represent different methods of assessing muscle strength.
3.4.2 Subgroup analysis of occlusion pressure prescription
The results of the subgroup analysis for the BFR-RT occlusion pressure prescription (individualized, incremental, and absolute pressure) are shown in Figure 5. The muscle strength gain obtained in the HL-RT group was significantly higher than that in the BFR-RT group when absolute pressure was applied (SMD = −0.45, 95% CI = −0.71 to −0.19, p < 0.05, I2 = 0). When cuff pressure was applied in the form of incremental and individualized pressures, the muscle strength gain obtained in the HL-RT and BFR-RT groups did not show any statistically significant difference (SMD = −0.05, 95% CI = −0.44 to 0.34, p = 0.80, I2 = 46%; SMD = −0.04, 95% CI = −0.23 to 0.15, p = 0.68, I2 = 0). The subgroup tests also revealed differences between groups (p < 0.05), highlighting a correlation between the manner of applying cuff pressure and muscle strength gain (Figure 5).
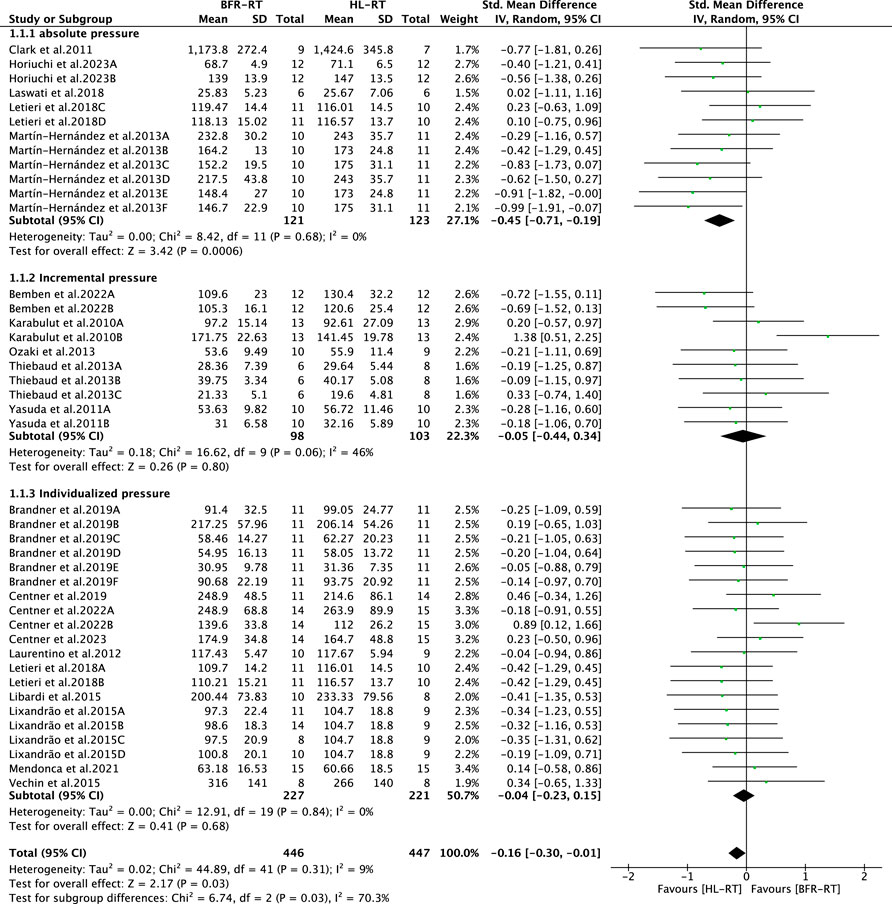
FIGURE 5. Subgroup analysis of the effect of the BFR-RT intervention on muscle strength. Different letters in the same study represent different methods of muscle strength assessment.
3.4.3 Subgroup analysis of cuff inflation patterns
The results of the subgroup analysis of the BFR-RT cuff pattern of inflation (intermittent and continuous pressure) are shown in Figure 6. The difference in muscle strength gain between the BFR-RT and HL-RT groups was not significant for intermittent pressure (SMD = −0.02, 95% CI = −0.27 to 0.23, p = 0.88, I2 = 41%). Regarding the application of continuous pressure, the degree of muscle strength gain of the BFR-RT group was lower than that of the HL-RT group (SMD = −0.30, 95% CI = −0.48 to −0.11, p < 0.05, I2 = 0). The subgroup tests revealed differences between the groups (p < 0.05), highlighting the correlation between the cuff pattern of inflation and muscle strength gain (Figure 6).
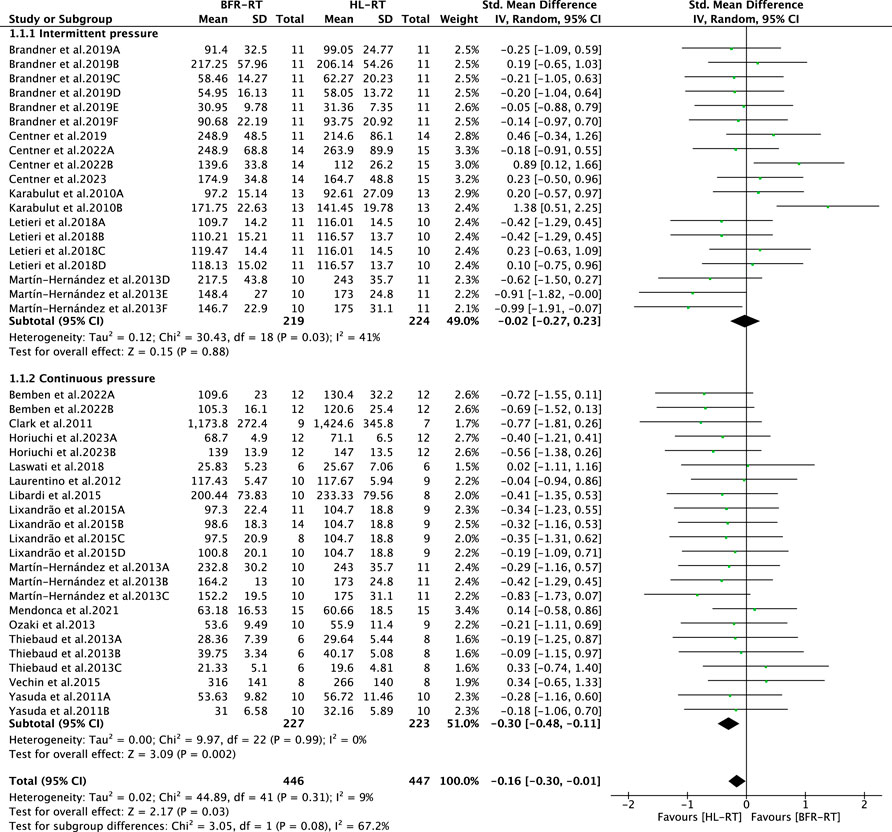
FIGURE 6. Subgroup analysis of the effect of the BFR-RT intervention on muscle strength. Different letters in the same study represent different methods of muscle strength assessment.
3.4.4 Subgroup analysis based on age and gender meta-regression
The findings from the meta-regression analysis revealed a significant correlation between age and changes in muscular strength adaptation (Coef. = 0.01, 95% CI = 0.001 to 0.02, p < 0.05), while no significant correlation was observed between gender and changes in muscular strength adaptation (Coef. = −0.17, 95% CI = −0.61 to 0.27, p = 0.438). Consequently, participants were classified into two groups, older and younger adults, to investigate their association with cuff pressure characteristics. Subgroup analyses demonstrated that older participants achieved comparable muscle strength adaptations to HL-RT when applied any of the exercise protocols involving cuff pressure characteristics in BFR-RT (Individualized pressures, SMD = −0.27, 95% CI = −0.72 to 0.19, p = 0.25, I2 = 0; Incremental pressures, SMD = 0.40, 95% CI = −0.02 to 0.82, p = 0.06, I2 = 47.9%; Absolute pressures, SMD = −0.17, 95% CI = −0.43 to 0.78, p = 0.575, I2 = 0; Continuous pressures, SMD = −0.02, 95% CI = −0.47 to 0.44, p = 0.944, I2 = 0; Intermittent pressures, SMD = 0.18, 95% CI = −0.16 to 0.53, p = 0.294, I2 = 57.9%); among younger participants, BFR-RT applied individualized and intermittent pressures yielded similar muscle strength adaptations as HL-RT (SMD = 0.00, 95% CI = −0.20 to 0.21, p = 0.963, I2 = 0; SMD = −0.09, 95% CI = −0.32 to 0.14, p = 0.462, I2 = 37.3%), while BFR-RT applied incremental, absolute, and continuous pressures did not produce comparable results (SMD = −0.45, 95% CI = −0.83 to −0.06, p < 0.05, I2 = 0; SMD = −0.61, 95% CI = −0.89 to −0.33, p < 0.05, I2 = 0; SMD = −0.37, 95% CI = −0.57 to −0.16, p < 0.05, I2 = 0). The findings of the meta-regression and subgroup analyses about age and gender can be observed in Supplementary Tables S3, S4.
3.4.5 Combination of occlusion pressure prescription and cuff inflation patterns
We used 3D maps as a visual aid to analyze the cuff pressure characteristics that favor muscle strength gain. The results showed that the application of BFR-RT exercise protocols with both “individualized pressure + intermittent pressure” and “incremental pressure + intermittent pressure” produced better adaptations for muscle strength gain (Figure 7).
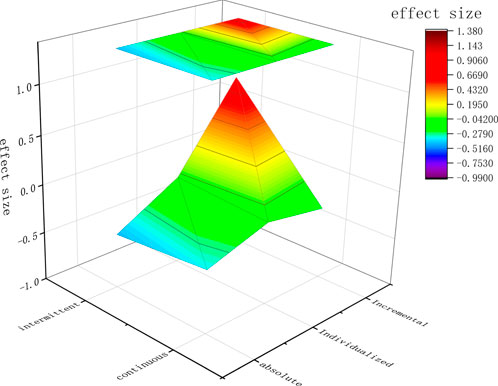
FIGURE 7. The pattern of inflation for the X-axis, occlusion pressure prescription for the Y-axis, and the effect size of the Z-axis.
3.4.6 Publication bias and sensitivity analysis
Finally, to assess potential publication bias in muscle strength gain, we conducted an Egger’s test and visual inspection of funnel plots, which showed that this study did not have publication bias (p = 0.184). In addition, sensitivity analyses were performed after individually excluding each of the 42 outcomes with respect to the effect of BFR-RT intervention on muscle strength gain. No significant changes in the combined effect values were found in the meta-analysis, indicating stable results.
4 Discussion
The main purpose of this meta-analysis was to compare the effects of BFR-RT on muscle strength in healthy adults. The included studies reported the effects of BFR-RT (20%–40% 1RM) on muscle strength in healthy adults and compared these with HL-RT (70%–90% 1RM) methods without BFR. The comprehensive results showed that BFR-RT produced less muscle strength gain than HL-RT, which is consistent with the results of previous studies (Lixandrao et al., 2018). Subgroup analysis of the occlusion pressure prescription showed that the BFR-RT group applying individualized pressure produced muscle strength gain comparable to the HL-RT group. Furthermore, the BFR-RT group applying incremental pressure produced muscle strength gain similar to HL-RT, whereas the BFR-RT group applying absolute pressure achieved less muscle strength gain than the HL-RT group. Subgroup analysis of the cuff pattern of inflation showed that the BFR-RT group with intermittent pressure achieved muscle strength gain comparable to that of the HL-RT group, whereas the BFR-RT group with continuous pressure achieved less muscle strength gain than the HL-RT group. Our findings also suggest that better muscle strength gain could be achieved by both “individualized pressure + intermittent pressure” and “incremental pressure + intermittent pressure” in the BFR-RT exercise protocol.
Muscle strength forms the basis for all activities in the human body. Some studies have highlighted the importance of muscle strength for specific motor skills and reduced injury rates (Suchomel et al., 2016). In the elderly population, higher muscle strength in the lower limbs is positively associated with cognitive function (Frith and Loprinzi, 2018), helps to prevent falls, and can lead to increased levels of physical activity (Trombetti et al., 2016). In the adult population, muscle strength and risk factors are negatively associated in terms of metabolic traits, higher muscle strength can reduce this relevance (Fraser et al., 2016). For special populations, muscle strength is a key factor in promoting mobility, cardiovascular capacity, and performance in daily activities (Obrusnikova et al., 2022). Researchers previously thought that the best approach for improving muscle strength is to perform HL-RT; however, as time progressed, increasing evidence showed that BFR-RT can also effectively improve muscle strength (Takarada et al., 2000; Brandner et al., 2019; Chang et al., 2022). Interestingly, many studies have focused their research objectives on comparing the differences in muscle strength gain via BFR-RT and the without vascular occlusion resistance exercise, but the effects of some characteristics of BFR-RT itself on muscle strength gain are generally ignored.
Cuff pressure characteristics are a key factor in establishing a BFR-RT exercise protocol. The choice of prescription regarding cuff occlusion pressure has also evolved, and the initial approach was to implement BFR-RT cuff pressures over arbitrary absolute pressure (Takarada et al., 2000). With the evolution of BFR-RT, the concept of initial absolute pressure became less popular. Some scholars eventually chose to gradually increase pressure during training (Karabulut et al., 2010; Bemben et al., 2022), whereas other researchers chose individualized cuff pressure based on arterial occlusion pressure (Laurentino et al., 2012; Centner et al., 2023). The rationale for the choice of incremental pressure is multifaceted, with some scholars proposing that incremental cuff pressure increases the level of perceived effort in participants (Yasuda et al., 2014b; Yasuda et al., 2015); meanwhile, others proposed that incremental pressure can help participants to better adapt to the occlusion stimulus during the initial phase of training (Junior et al., 2019a; Junior et al., 2019b). The rationale for selecting individualized pressure is that individualized pressure motion prescriptions are more accurate than broad occlusion pressure motion prescriptions when applying cuff pressure during BFR-RT exercise, with individualized prescriptions accounting for device variations (e.g., different cuff widths) when setting the same absolute pressure, thus addressing the limitations caused by this variability (Mouser et al., 2018). Overall, BFR-RT interventions using different cuff pressure characteristics affected perceived effort and adaptation to occlusive stimuli but failed to demonstrate a positive effect on muscle strength adaptation.
This meta-analysis analyzed the effect of cuff pressure characteristics on muscle strength gain. For the first time, cuff occlusion pressure prescriptions were divided into individualized, incremental, and absolute pressures for subgroup analysis. The application of individualized pressure in BFR-RT leads to muscle strength gain that is comparable to that in HL-RT. This result differs from the meta-analysis of Lixandrao et al., 2018; the heterogeneity observed in studies may be attributed to the number of included articles. In this meta-analysis, the individualized pressure in the included articles ranged between 40% and 80% limb occlusion pressure. Lixandrão et al., 2015 implemented personalized occlusion pressures of 40% and 80%, along with exercise loads of 20% and 40% of 1RM, the results of this study indicated that higher occlusion pressures yielded greater benefits for muscle strength when exercise loads were lower, whereas exercise load played a more significant role when higher exercise load were implemented. Letieri et al., 2018 implemented a personalized occlusion pressure of 80% and an exercise load ranging from 20% to 30% of 1RM over a span of 16 weeks. The participants completed a total of 48 training sessions during this period, notably, during the initial 2 weeks of the study, three sets of each exercise were executed (30, 15, 15), while in subsequent sessions, four sets were performed (15, 15, 15, 15), this discrepancy in the number of sets may lead to a more rigorous exercise stimulus at a lower pre-exercise load. In addition, in the included articles applying 50% arterial occlusion pressure, the BFR-RT intervention yielded similar muscle strength adaptations as HL-RT. Regarding incremental and absolute pressures, a recent review examined the effect of BFR-RT cuff pressure characteristics on cardiovascular metrics (Cerqueira et al., 2021). Although the significance of cardiovascular metrics in BFR-RT interventions is important, no conclusions were given regarding whether incremental or absolute pressure could be better applied in BFR-RT. In the current study, we approached this research gap from the perspective of muscle strength gain. Of the articles included in this review, five articles applied incremental pressures with pressure increases of 40, 60, and 80 mmHg, with cuff pressures ranging from 80 mm Hg to 240 mmHg, with a mean pressure of approximately 158 mmHg, and reported cuff widths ranging from 3 to 5 cm; and five articles applied absolute pressures with cuff pressures ranging from 50 mm Hg to 187.5 mmHg, with reported cuff widths ranging from 6 to 14 cm. The results of this meta-analysis showed that the BFR-RT group applied with incremental pressure showed comparable muscle strength gain to the HL-RT group. The finding differed for the BFR-RT group applied with absolute pressure.
Moreover, subgroup analysis of the cuff pattern of inflation showed that the muscle strength gain of the BFR-RT group applied with intermittent pressure was comparable to that of the HL-RT group. By contrast, the muscle strength gain of the BFR-RT group applied with continuous pressure was less apparent than that of the HL-RT group. We explained the reasons for the differences in outcomes between subgroups in the following three ways. Regarding the cause of fatigue, BFR-RT may produce higher levels of discomfort and a higher rate of perceived exertion than HL-RT without blood flow occlusion; the subjects may have manifested significant intolerance to the BFR-RT exercise regimen (Bell et al., 2018; Soligon et al., 2018; Dankel et al., 2019). The rate of perceived exertion after exercise was lower in the BFR-RT group applied with intermittent pressure; this phenomenon was not observed in the BFR-RT group applied with continuous pressure (Corvino et al., 2017). This finding may be explained by the BFR-RT applied with continuous pressure leading to an immediate onset of physiological and metabolic stress, causing increased fatigue and decreased exercise performance (Wernbom et al., 2009; Loenneke et al., 2012), suggesting greater discomfort (Neto et al., 2018; Wilk et al., 2018; Okita et al., 2019) compared with the BFR-RT group applied with intermittent pressure. From an energy supply perspective, the phosphocreatine concentration gradually decreases in BFR-RT applied with continuous pressure because of blood flow occlusion; by contrast, the release of vascular occlusion and subsequent restoration of phosphocreatine concentration through the phosphocreatine shuttle system occurs in BFR-RT applied with intermittent pressure (Suga et al., 2012; Guimarães-Ferreira, 2014; Okita et al., 2019), prompting replenishment of stored adenosine triphosphate and an increase in muscle tissue energy status (Guimarães-Ferreira, 2014). From a body metabolism perspective, BFR-RT applied with intermittent pressure provides better perfusion, venous return, and shorter occlusion times compared with BFR-RT applied with continuous pressure, with the former further preventing anaerobic respiratory byproducts from pooling and accumulating in the body, further resulting in lower levels of expected metabolic disturbances (Pearson and Hussain, 2015; Teixeira et al., 2018; Okita et al., 2019).
Furthermore, when age was considered, we found that in the elderly population, all BFR-RT exercise protocols applying different cuff pressure characteristics achieved similar muscle strength gains to HL-RT; whereas in the younger population, only two exercise protocols applying intermittent and individualized pressures achieved similar muscle strength gains to HL-RT. However, the number of included articles is small and conclusions should be drawn with caution. To provide clinical staff with more accurate BFR-RT exercise protocols, we used 3D models as an aid in investigating the combined effects of occlusion pressure prescription and cuff pattern of inflation on muscle strength gain. The results showed that the two BFR-RT exercise protocols “individualized pressure + intermittent pressure” and “incremental pressure + intermittent pressure” produced better adaptations for muscle strength gain. This finding illustrates the need to consider cuff pressure characteristics when setting up BFR-RT exercise prescriptions for healthy adults.
While focusing on the many benefits of BFR training, it is also important to consider the safety and adherence rates of the subjects.BFR training has some inherent safety concerns, as it essentially pressurizes the limb, resulting in decreased arterial blood inflow and venous blood pooling, This can result in the occurrence of ischemia and hypoxia within the body (Yasuda et al., 2010), while the inadequate elimination of lactic acid and other metabolites generated during this process is observed (Yasuda et al., 2014a; Teixeira et al., 2018). Furthermore, it has been shown that 23 min of BFR-RT training under sustained stress conditions, even with moderate exercise, can lead to muscle damage (Wernbom et al., 2020; Wernbom et al., 2021). However, Nakajima et al.'s questionnaire survey of 12,600 individuals who had experienced BFR training found that the most common side effects of BFR were subcutaneous hemorrhage and temporary numbness, but that this response diminished and disappeared as the cuff was released and training progressed (Nakajima et al., 2006). Therefore, from the point of view of safety and compliance, the BFR-RT with intermittent compression and the BFR with incremental pressure protocols may be a good choice for physical training. This is because with intermittent pressurization, subjects can release the cuff pressure restriction during rest breaks between sets, and electronic compression devices can be easily deflated and inflated during rest breaks without interfering with the training process; intermittent pressurization is also a viable option for people with low tolerance for ischemic pain and discomfort, and can improve adherence ineffective this population (Davids et al., 2021); and incremental pressurization can allow the subject to have a process of adapting to the pressure during training (i.e., cuff pressure can be slowly increased), which would be safer for participants and have a more positive attitude toward subsequent training. Coupled with the fact that our findings also suggest that intermittent pressurized BFR and incremental pressurized BFR exercise interventions have positive muscle strength growth adaptations, we recommend the use of intermittent pressurized incremental pressurized BFR-RT exercise regimens for long-term BFR-RT training, which appears to improve subject safety and compliance rates as well as better training outcomes.
5 Limitations
First, the vast majority of the population included in this study was inexperienced in training, which provides limited guidance for those undergoing resistance training. Second, there is a lack of research on young female experimenters, and future research could focus on this population. Thirdly, the duration of the training period spanned a considerable timeframe ranging from 4 to 16 weeks, potentially influencing the observed results. Finally, considering multiple outcomes from the same study in a meta-analysis may also partially affect the homogeneity of results.
6 Conclusion
Our findings indicate that, in general, HL-RT produces greater muscle strength gains than BF-RT. However, upon further analysis, we observed that BFR-RT can yield similar muscle strength gains to HL-RT when considering specific cuff pressure characteristics, such as individualized pressure, incremental pressure, and intermittent pressure BFR-RT exercise protocols. Additionally, our results indicate that the combination of “individualized pressure + intermittent pressure” and “incremental pressure + intermittent pressure” BFR-RT exercise protocols display a tendency towards enhanced muscle strength gains. Overall, cuff pressure characteristics should be given more attention when setting up BFR-RT exercise protocols. It is important to note that age affects subgroup outcomes, indicating that the age factor should also be considered when considering the cuff pressure characteristics of the BFR-RT.
Data availability statement
The original contributions presented in the study are included in the article/Supplementary Material, further inquiries can be directed to the corresponding author.
Author contributions
HC, JZ, and JY contributed to conception and design of the study. HC, GL, and JZ organized the database. HC and JY performed statistical analyses and drafted the manuscript. JZ, GL, and BC assisted in interpretation of data and provided additional suggestions. HC and JZ revised and finalized the manuscript. All authors contributed to the article and approved the submitted version.
Conflict of interest
The authors declare that the research was conducted in the absence of any commercial or financial relationships that could be construed as a potential conflict of interest.
Publisher’s note
All claims expressed in this article are solely those of the authors and do not necessarily represent those of their affiliated organizations, or those of the publisher, the editors and the reviewers. Any product that may be evaluated in this article, or claim that may be made by its manufacturer, is not guaranteed or endorsed by the publisher.
Supplementary material
The Supplementary Material for this article can be found online at: https://www.frontiersin.org/articles/10.3389/fphys.2023.1244292/full#supplementary-material
References
Bell, Z. W., Buckner, S. L., Jessee, M. B., Mouser, J. G., Mattocks, K. T., Dankel, S. J., et al. (2018). Moderately heavy exercise produces lower cardiovascular, RPE, and discomfort compared to lower load exercise with and without blood flow restriction. Eur. J. Appl. Physiol. 118 (7), 1473–1480. doi:10.1007/s00421-018-3877-0
Bemben, D. A., Sherk, V. D., Buchanan, S. R., Kim, S., Sherk, K., and Bemben, M. G. (2022). Acute and chronic bone marker and endocrine responses to resistance exercise with and without blood flow restriction in young men. Front. Physiol. 13, 837631. doi:10.3389/fphys.2022.837631
Brandner, C. R., Clarkson, M. J., Kidgell, D. J., and Warmington, S. A. (2019). Muscular adaptations to whole body blood flow restriction training and detraining. Front. Physiol. 10, 1099. doi:10.3389/fphys.2019.01099
Brun, J., Supparo, I., Fons, C., El Bouhmadi, A., and Orsetti, A. (1994). Low values of blood viscosity and erythrocyte aggregation are associated with lower increases in blood lactate during submaximal exercise. Clin. Hemorheol. Microcirc. 14 (1), 105–116. doi:10.3233/ch-1994-14114
Centner, C., Jerger, S., Lauber, B., Seynnes, O., Friedrich, T., Lolli, D., et al. (2022). Low-load blood flow restriction and high-load resistance training induce comparable changes in patellar tendon properties. Med. Sci. Sports Exerc 54 (4), 582–589. doi:10.1249/mss.0000000000002824
Centner, C., Jerger, S., Lauber, B., Seynnes, O., Friedrich, T., Lolli, D., et al. (2023). Similar patterns of tendon regional hypertrophy after low-load blood flow restriction and high-load resistance training. Scand. J. Med. Sci. Sports 33 (6), 848–856. doi:10.1111/sms.14321
Centner, C., Lauber, B., Seynnes, O. R., Jerger, S., Sohnius, T., Gollhofer, A., et al. (2019). Low-load blood flow restriction training induces similar morphological and mechanical Achilles tendon adaptations compared with high-load resistance training. J. Appl. Physiol. 127(6), 1660–1667. doi:10.1152/japplphysiol.00602.2019
Cerqueira, M. S., Costa, E. C., Santos Oliveira, R., Pereira, R., and Brito Vieira, W. H. (2021). Blood flow restriction training: to adjust or not adjust the cuff pressure over an intervention period? Front. Physiol. 12, 678407. doi:10.3389/fphys.2021.678407
Chang, H., Yao, M., Chen, B., Qi, Y., and Zhang, J. (2022). Effects of blood flow restriction combined with low-intensity resistance training on lower-limb muscle strength and mass in post-middle-aged adults: a systematic review and meta-analysis. Int. J. Environ. Res. Public Health 19 (23), 15691. doi:10.3390/ijerph192315691
Clark, B. C., Manini, T. M., Hoffman, R. L., Williams, P. S., Guiler, M. K., Knutson, M. J., et al. (2011). Relative safety of 4 weeks of blood flow-restricted resistance exercise in young, healthy adults. Scand. J. Med. Sci. Sports 21 (5), 653–662. doi:10.1111/j.1600-0838.2010.01100.x
Clarkson, M. J., May, A. K., and Warmington, S. A. (2020). Is there rationale for the cuff pressures prescribed for blood flow restriction exercise? A systematic review. Scand. J. Med. Sci. Sports 30 (8), 1318–1336. doi:10.1111/sms.13676
Corvino, R. B., Rossiter, H. B., Loch, T., Martins, J. C., and Caputo, F. (2017). Physiological responses to interval endurance exercise at different levels of blood flow restriction. Eur. J. Appl. Physiol. 117 (1), 39–52. doi:10.1007/s00421-016-3497-5
Dankel, S. J., Jessee, M. B., Mattocks, K. T., Buckner, S. L., Mouser, J. G., Bell, Z. W., et al. (2019). Perceptual and arterial occlusion responses to very low load blood flow restricted exercise performed to volitional failure. Clin. Physiol. Funct. Imaging 39 (1), 29–34. doi:10.1111/cpf.12535
Davids, C. J., Raastad, T., James, L. P., Gajanand, T., Smith, E., Connick, M., et al. (2021). Similar morphological and functional training adaptations occur between continuous and intermittent blood flow restriction. J. Strength Cond. Res. 35 (7), 1784–1793. doi:10.1519/jsc.0000000000004034
Fraser, B. J., Huynh, Q. L., Schmidt, M. D., Dwyer, T., Venn, A. J., and Magnussen, C. G. (2016). Childhood muscular fitness phenotypes and adult metabolic syndrome. Med. Sci. Sports Exerc 48 (9), 1715–1722. doi:10.1249/mss.0000000000000955
Frith, E., and Loprinzi, P. D. (2018). The association between lower extremity muscular strength and cognitive function in a national sample of older adults. J. Lifestyle Med. 8 (2), 99–104. doi:10.15280/jlm.2018.8.2.99
Fry, C. S., Glynn, E. L., Drummond, M. J., Timmerman, K. L., Fujita, S., Abe, T., et al. (2010). Blood flow restriction exercise stimulates mTORC1 signaling and muscle protein synthesis in older men. J. Appl. Physiol. 108(5), 1199–1209. doi:10.1152/japplphysiol.01266.2009
Garber, C. E., Blissmer, B., Deschenes, M. R., Franklin, B. A., Lamonte, M. J., Lee, I. M., et al. (2011). American College of sports medicine position stand. Quantity and quality of exercise for developing and maintaining cardiorespiratory, musculoskeletal, and neuromotor fitness in apparently healthy adults: guidance for prescribing exercise. Med. Sci. Sports Exerc 43 (7), 1334–1359. doi:10.1249/MSS.0b013e318213fefb
García-Hermoso, A., Cavero-Redondo, I., Ramírez-Vélez, R., Ruiz, J. R., Ortega, F. B., Lee, D. C., et al. (2018). Muscular strength as a predictor of all-cause mortality in an apparently healthy population: a systematic review and meta-analysis of data from approximately 2 million men and women. Arch. Phys. Med. Rehabil. 99 (10), 2100–2113. doi:10.1016/j.apmr.2018.01.008
Guimarães-Ferreira, L. (2014). Role of the phosphocreatine system on energetic homeostasis in skeletal and cardiac muscles. Einstein (Sao Paulo) 12 (1), 126–131. doi:10.1590/s1679-45082014rb2741
Higgins, J. P., Altman, D. G., Gøtzsche, P. C., Jüni, P., Moher, D., Oxman, A. D., et al. (2011). The Cochrane Collaboration’s tool for assessing risk of bias in randomised trials. Bmj 343, d5928. doi:10.1136/bmj.d5928
Horiuchi, M., Stoner, L., and Poles, J. (2023). The effect of four weeks blood flow restricted resistance training on macro- and micro-vascular function in healthy, young men. Eur. J. Appl. Physiol. 2023. doi:10.1007/s00421-023-05230-3
Hughes, L., Rosenblatt, B., Haddad, F., Gissane, C., McCarthy, D., Clarke, T., et al. (2019). Comparing the effectiveness of blood flow restriction and traditional heavy load resistance training in the post-surgery rehabilitation of anterior cruciate ligament reconstruction patients: a UK national health service randomised controlled trial. Sports Med. 49 (11), 1787–1805. doi:10.1007/s40279-019-01137-2
Jochem, C., Leitzmann, M., Volaklis, K., Aune, D., and Strasser, B. (2019). Association between muscular strength and mortality in clinical populations: a systematic review and meta-analysis. J. Am. Med. Dir. Assoc. 20 (10), 1213–1223. doi:10.1016/j.jamda.2019.05.015
Junior, A. F., Schamne, J. C., Altimari, L. R., Okano, A. H., and Okuno, N. M. (2019a). Effect of walk training combined with blood flow restriction on resting heart rate variability and resting blood pressure in middle-aged men. Mot. Rev. Educ. Física 25 (2). doi:10.1590/s1980-6574201900020005
Junior, A. F., Schamne, J. C., Perandini, L. A. B., Chimin, P., and Okuno, N. M. (2019b). Effects of walking training with restricted blood flow on HR and HRV kinetics and HRV recovery. Int. J. Sports Med. 40 (9), 585–591. doi:10.1055/a-0942-7479
Karabulut, M., Abe, T., Sato, Y., and Bemben, M. G. (2010). The effects of low-intensity resistance training with vascular restriction on leg muscle strength in older men. Eur. J. Appl. Physiol. 108 (1), 147–155. doi:10.1007/s00421-009-1204-5
Laswati, H., Sugiarto, D., Poerwandari, D., Pangkahila, J. A., and Kimura, H. (2018). Low-Intensity exercise with blood flow restriction increases muscle strength without altering hsCRP and fibrinogen levels in healthy subjects. Chin. J. Physiol. 61 (3), 188–195. doi:10.4077/cjp.2018.Bag567
Laurentino, G. C., Ugrinowitsch, C., Roschel, H., Aoki, M. S., Soares, A. G., Neves, M., et al. (2012). Strength training with blood flow restriction diminishes myostatin gene expression. Med. Sci. Sports Exerc 44 (3), 406–412. doi:10.1249/MSS.0b013e318233b4bc
Leon, A. S., Franklin, B. A., Costa, F., Balady, G. J., Berra, K. A., Stewart, K. J., et al. (2005). Cardiac rehabilitation and secondary prevention of coronary heart disease: an American heart association scientific statement from the council on clinical cardiology (subcommittee on exercise, cardiac rehabilitation, and prevention) and the council on nutrition, physical activity, and metabolism (subcommittee on physical activity), in collaboration with the American association of cardiovascular and pulmonary rehabilitation. Circulation 111 (3), 369–376. doi:10.1161/01.Cir.0000151788.08740.5c
Letieri, R. V., Teixeira, A. M., Furtado, G. E., Lamboglia, C. G., Rees, J. L., and Gomes, B. B. (2018). Effect of 16 weeks of resistance exercise and detraining comparing two methods of blood flow restriction in muscle strength of healthy older women: a randomized controlled trial. Exp. Gerontol. 114, 78–86. doi:10.1016/j.exger.2018.10.017
Libardi, C. A., Chacon-Mikahil, M. P., Cavaglieri, C. R., Tricoli, V., Roschel, H., Vechin, F. C., et al. (2015). Effect of concurrent training with blood flow restriction in the elderly. Int. J. Sports Med. 36 (5), 395–399. doi:10.1055/s-0034-1390496
Lixandrao, M. E., Ugrinowitsch, C., Berton, R., Vechin, F. C., Conceicao, M. S., Damas, F., et al. (2018). Magnitude of muscle strength and mass adaptations between high-load resistance training versus low-load resistance training associated with blood-flow restriction: a systematic review and meta-analysis. Sports Med. 48 (2), 361–378. doi:10.1007/s40279-017-0795-y
Lixandrão, M. E., Ugrinowitsch, C., Laurentino, G., Libardi, C. A., Aihara, A. Y., Cardoso, F. N., et al. (2015). Effects of exercise intensity and occlusion pressure after 12 weeks of resistance training with blood-flow restriction. Eur. J. Appl. Physiol. 115 (12), 2471–2480. doi:10.1007/s00421-015-3253-2
Loenneke, J., Balapur, A., Thrower, A., Barnes, J., and Pujol, T. (2012). Blood flow restriction reduces time to muscular failure. Eur. J. Sport Sci. 12 (3), 238–243. doi:10.1080/17461391.2010.551420
Loenneke, J. P., Thiebaud, R. S., Abe, T., and Bemben, M. G. (2014). Blood flow restriction pressure recommendations: the hormesis hypothesis. Med. Hypotheses 82 (5), 623–626. doi:10.1016/j.mehy.2014.02.023
Manini, T. M., and Clark, B. C. (2012). Dynapenia and aging: an update. J. Gerontol. A Biol. Sci. Med. Sci. 67 (1), 28–40. doi:10.1093/gerona/glr010
Martín-Hernández, J., Marín, P. J., Menéndez, H., Ferrero, C., Loenneke, J. P., and Herrero, A. J. (2013). Muscular adaptations after two different volumes of blood flow-restricted training. Scand. J. Med. Sci. Sports 23 (2), e114–e120. doi:10.1111/sms.12036
Mendonca, G. V., Vila-Chã, C., Teodósio, C., Goncalves, A. D., Freitas, S. R., Mil-Homens, P., et al. (2021). Contralateral training effects of low-intensity blood-flow restricted and high-intensity unilateral resistance training. Eur. J. Appl. Physiol. 121 (8), 2305–2321. doi:10.1007/s00421-021-04708-2
Mouser, J. G., Dankel, S. J., Mattocks, K. T., Jessee, M. B., Buckner, S. L., Abe, T., et al. (2018). Blood flow restriction and cuff width: effect on blood flow in the legs. Clin. Physiol. Funct. Imaging 38, 944–948. doi:10.1111/cpf.12504
Nakajima, T., Kurano, M., Iida, H., Takano, H., Oonuma, H., Morita, T., et al. (2006). Use and safety of KAATSU training:Results of a national survey. Int. J. KAATSU Train. Res. 2 (1), 5–13. doi:10.3806/ijktr.2.5
Neto, G. R., Novaes, J. S., Salerno, V. P., Gonçalves, M. M., Batista, G. R., and Cirilo-Sousa, M. S. (2018). Does a resistance exercise session with continuous or intermittent blood flow restriction promote muscle damage and increase oxidative stress? J. Sports Sci. 36 (1), 104–110. doi:10.1080/02640414.2017.1283430
Obrusnikova, I., Firkin, C. J., Cavalier, A. R., and Suminski, R. R. (2022). Effects of resistance training interventions on muscular strength in adults with intellectual disability: a systematic review and meta-analysis. Disabil. Rehabil. 44 (17), 4549–4562. doi:10.1080/09638288.2021.1910738
Okita, K., Takada, S., Morita, N., Takahashi, M., Hirabayashi, K., Yokota, T., et al. (2019). Resistance training with interval blood flow restriction effectively enhances intramuscular metabolic stress with less ischemic duration and discomfort. Appl. Physiol. Nutr. Metab. 44 (7), 759–764. doi:10.1139/apnm-2018-0321
Ozaki, H., Yasuda, T., Ogasawara, R., Sakamaki-Sunaga, M., Naito, H., and Abe, T. (2013). Effects of high-intensity and blood flow-restricted low-intensity resistance training on carotid arterial compliance: role of blood pressure during training sessions. Eur. J. Appl. Physiol. 113 (1), 167–174. doi:10.1007/s00421-012-2422-9
Page, M. J., McKenzie, J. E., Bossuyt, P. M., Boutron, I., Hoffmann, T. C., Mulrow, C. D., et al. (2021). The PRISMA 2020 statement: an updated guideline for reporting systematic reviews. Bmj 372, n71. doi:10.1136/bmj.n71
Papa, E. V., Dong, X., and Hassan, M. (2017). Skeletal muscle function deficits in the elderly: current perspectives on resistance training. J. Nat. Sci. 3 (1), e272.
Pearson, S. J., and Hussain, S. R. (2015). A review on the mechanisms of blood-flow restriction resistance training-induced muscle hypertrophy. Sports Med. 45 (2), 187–200. doi:10.1007/s40279-014-0264-9
Peterson, M. D., and Krishnan, C. (2015). Growth charts for muscular strength capacity with quantile regression. Am. J. Prev. Med. 49 (6), 935–938. doi:10.1016/j.amepre.2015.05.013
Rantanen, T., Guralnik, J. M., Foley, D., Masaki, K., Leveille, S., Curb, J. D., et al. (1999). Midlife hand grip strength as a predictor of old age disability. Jama 281 (6), 558–560. doi:10.1001/jama.281.6.558
Sato, Y. (2005). The history and future of KAATSU Training. Int. J. KAATSU Train. Res. 1 (1), 1–5. doi:10.3806/ijktr.1.1
Sieljacks, P., Wang, J., Groennebaek, T., Rindom, E., Jakobsgaard, J. E., Herskind, J., et al. (2019). Six weeks of low-load blood flow restricted and high-load resistance exercise training produce similar increases in cumulative myofibrillar protein synthesis and ribosomal biogenesis in healthy males. Front. Physiol. 10, 649. doi:10.3389/fphys.2019.00649
Silventoinen, K., Magnusson, P. K., Tynelius, P., Batty, G. D., and Rasmussen, F. (2009). Association of body size and muscle strength with incidence of coronary heart disease and cerebrovascular diseases: a population-based cohort study of one million Swedish men. Int. J. Epidemiol. 38 (1), 110–118. doi:10.1093/ije/dyn231
Sinclair, P., Kadhum, M., and Paton, B. (2022). Tolerance to intermittent vs. Continuous blood flow restriction training: a meta-analysis. Int. J. Sports Med. 43 (1), 3–10. doi:10.1055/a-1537-9886
Soligon, S. D., Lixandrão, M. E., Biazon, T., Angleri, V., Roschel, H., and Libardi, C. A. (2018). Lower occlusion pressure during resistance exercise with blood-flow restriction promotes lower pain and perception of exercise compared to higher occlusion pressure when the total training volume is equalized. Physiol. Int. 105 (3), 276–284. doi:10.1556/2060.105.2018.3.18
Stenholm, S., Mehta, N. K., Elo, I. T., Heliövaara, M., Koskinen, S., and Aromaa, A. (2014). Obesity and muscle strength as long-term determinants of all-cause mortality--a 33-year follow-up of the Mini-Finland Health Examination Survey. Int. J. Obes. (Lond) 38 (8), 1126–1132. doi:10.1038/ijo.2013.214
Suchomel, T. J., Nimphius, S., and Stone, M. H. (2016). The importance of muscular strength in athletic performance. Sports Med. 46 (10), 1419–1449. doi:10.1007/s40279-016-0486-0
Suga, T., Okita, K., Takada, S., Omokawa, M., Kadoguchi, T., Yokota, T., et al. (2012). Effect of multiple set on intramuscular metabolic stress during low-intensity resistance exercise with blood flow restriction. Eur. J. Appl. Physiol. 112 (11), 3915–3920. doi:10.1007/s00421-012-2377-x
Takahashi, T., Sugie, M., Nara, M., Koyama, T., Obuchi, S. P., Harada, K., et al. (2017). Femoral muscle mass relates to physical frailty components in community-dwelling older people. Geriatr. Gerontol. Int. 17 (10), 1636–1641. doi:10.1111/ggi.12945
Takarada, Y., Takazawa, H., Sato, Y., Takebayashi, S., Tanaka, Y., and Ishii, N. (2000). Effects of resistance exercise combined with moderate vascular occlusion on muscular function in humans. J. Appl. Physiol. 88(6), 2097–2106. doi:10.1152/jappl.2000.88.6.2097
Teixeira, E. L., Barroso, R., Silva-Batista, C., Laurentino, G. C., Loenneke, J. P., Roschel, H., et al. (2018). Blood flow restriction increases metabolic stress but decreases muscle activation during high-load resistance exercise. Muscle Nerve 57 (1), 107–111. doi:10.1002/mus.25616
Thiebaud, R. S., Loenneke, J. P., Fahs, C. A., Rossow, L. M., Kim, D., Abe, T., et al. (2013). The effects of elastic band resistance training combined with blood flow restriction on strength, total bone-free lean body mass and muscle thickness in postmenopausal women. Clin. Physiol. Funct. Imaging 33 (5), 344–352. doi:10.1111/cpf.12033
Trombetti, A., Reid, K. F., Hars, M., Herrmann, F. R., Pasha, E., Phillips, E. M., et al. (2016). Age-associated declines in muscle mass, strength, power, and physical performance: impact on fear of falling and quality of life. Osteoporos. Int. 27 (2), 463–471. doi:10.1007/s00198-015-3236-5
Vechin, F. C., Libardi, C. A., Conceição, M. S., Damas, F. R., Lixandrão, M. E., Berton, R. P., et al. (2015). Comparisons between low-intensity resistance training with blood flow restriction and high-intensity resistance training on quadriceps muscle mass and strength in elderly. J. Strength Cond. Res. 29 (4), 1071–1076. doi:10.1519/jsc.0000000000000703
Wernbom, M., Järrebring, R., Andreasson, M. A., and Augustsson, J. (2009). Acute effects of blood flow restriction on muscle activity and endurance during fatiguing dynamic knee extensions at low load. J. Strength Cond. Res. 23 (8), 2389–2395. doi:10.1519/JSC.0b013e3181bc1c2a
Wernbom, M., Paulsen, G., Bjørnsen, T., Cumming, K., and Raastad, T. (2021). Risk of muscle damage with blood flow-restricted exercise should not Be overlooked. Clin. J. Sport Med. 31 (3), 223–224. doi:10.1097/jsm.0000000000000755
Wernbom, M., Schoenfeld, B. J., Paulsen, G., Bjørnsen, T., Cumming, K. T., Aagaard, P., et al. (2020). Commentary: can blood flow restricted exercise cause muscle damage? Commentary on blood flow restriction exercise: considerations of methodology, application, and safety. Front. Physiol. 11, 243. doi:10.3389/fphys.2020.00243
Wilk, M., Krzysztofik, M., Gepfert, M., Poprzecki, S., Gołaś, A., and Maszczyk, A. (2018). Technical and training related aspects of resistance training using blood flow restriction in competitive sport - a review. J. Hum. Kinet. 65, 249–260. doi:10.2478/hukin-2018-0101
Yasuda, T., Abe, T., Brechue, W. F., Iida, H., Takano, H., Meguro, K., et al. (2010). Venous blood gas and metabolite response to low-intensity muscle contractions with external limb compression. Metabolism 59 (10), 1510–1519. doi:10.1016/j.metabol.2010.01.016
Yasuda, T., Fukumura, K., Fukuda, T., Iida, H., Imuta, H., Sato, Y., et al. (2014a). Effects of low-intensity, elastic band resistance exercise combined with blood flow restriction on muscle activation. Scand. J. Med. Sci. Sports 24 (1), 55–61. doi:10.1111/j.1600-0838.2012.01489.x
Yasuda, T., Fukumura, K., Fukuda, T., Uchida, Y., Iida, H., Meguro, M., et al. (2014b). Muscle size and arterial stiffness after blood flow-restricted low-intensity resistance training in older adults. Scand. J. Med. Sci. Sports 24 (5), 799–806. doi:10.1111/sms.12087
Yasuda, T., Fukumura, K., Uchida, Y., Koshi, H., Iida, H., Masamune, K., et al. (2015). Effects of low-load, elastic band resistance training combined with blood flow restriction on muscle size and arterial stiffness in older adults. J. Gerontol. A Biol. Sci. Med. Sci. 70 (8), 950–958. doi:10.1093/gerona/glu084
Keywords: blood flow restriction, muscle strength, occlusion pressure prescriptions, cuff inflation patterns, adult
Citation: Chang H, Yan J, Lu G, Chen B and Zhang J (2023) Muscle strength adaptation between high-load resistance training versus low-load blood flow restriction training with different cuff pressure characteristics: a systematic review and meta-analysis. Front. Physiol. 14:1244292. doi: 10.3389/fphys.2023.1244292
Received: 22 June 2023; Accepted: 14 August 2023;
Published: 25 August 2023.
Edited by:
José Antonio de Paz, University of León, SpainReviewed by:
Angel Gallego-Selles, University of Las Palmas de Gran Canaria, SpainMousa Khalafi, University of Kashan, Iran
Copyright © 2023 Chang, Yan, Lu, Chen and Zhang. This is an open-access article distributed under the terms of the Creative Commons Attribution License (CC BY). The use, distribution or reproduction in other forums is permitted, provided the original author(s) and the copyright owner(s) are credited and that the original publication in this journal is cited, in accordance with accepted academic practice. No use, distribution or reproduction is permitted which does not comply with these terms.
*Correspondence: Jianli Zhang, emhhbmdqbEB6am51LmVkdS5jbg==