- 1Department of Gastroenterology, The Second Xiangya Hospital, Central South University, Changsha, Hunan, China
- 2Research Center of Digestive Disease, Central South University, Changsha, Hunan, China
Sphingosine-1-phosphate (S1P), a type of bioactive sphingolipid, can regulate various cellular functions of distinct cell types in the human body. S1P is generated intracellularly by the catalysis of sphingosine kinase 1/2 (SphK1/2). S1P is transferred to the extracellular environment via the S1P transporter, binds to cellular S1P receptors (S1PRs) and subsequently activates S1P-S1PR downstream signaling. Dysbiosis of the intestinal microbiota, immune dysregulation and damage to epithelial barriers are associated with inflammatory bowel disease (IBD). Generally, S1P mainly exerts a proinflammatory effect by binding to S1PR1 on lymphocytes to facilitate lymphocyte migration to inflamed tissues, and increased S1P was found in the intestinal mucosa of IBD patients. Notably, there is an interaction between the distribution of gut bacteria and SphK-S1P signaling in the intestinal epithelium. S1P-S1PR signaling can also regulate the functions of intestinal epithelial cells (IECs) in mucosa, including cell proliferation and apoptosis. Additionally, increased S1P in immune cells of the lamina propria aggravates the inflammatory response by increasing the production of proinflammatory cytokines. Several novel drugs targeted at S1PRs have recently been used for IBD treatment. This review provides an overview of the S1P-S1PR signaling pathway and, in particular, summarizes the various roles of S1P in the gut mucosal microenvironment to deeply explore the function of S1P-S1PR signaling during intestinal inflammation and, more importantly, to identify potential therapeutic targets for IBD in the SphK-S1P-S1PR axis.
1 Introduction
Inflammatory bowel disease (IBD) is defined by gastrointestinal nonspecific chronic inflammation and includes Crohn’s disease (CD) and ulcerative colitis (UC). IBD occurs frequently in Western countries, with a rising incidence in newly industrialized countries in Africa, Asia and South America; furthermore, it has become a global health issue that places increasing financial and medical burdens on people (Ng et al., 2017). The pathogenesis of IBD is unclear, and it is associated with genetic susceptibility, environmental changes, intestinal microbiota imbalance and immune dysregulation (Xavier and Podolsky, 2007). Reducing mucosal inflammation and promoting intestinal mucosal wound healing are considered the main goals of IBD treatment. Conventional medicines such as 5-aminosalicylates, glucocorticoids and immunosuppressants do not have satisfactory anti-inflammatory effects and have some side effects. Currently, clinically applied biologic agents, including anti-TNF-α agents, anti-integrin agents and anti-interleukin 12/23 agents, have obtained tremendous advances, but there are still several deficiencies, such as individual differences in efficacy, failures in response and high recurrence rates (Al-Bawardy et al., 2021). Therefore, novel therapeutic agents targeting different pathways are urgently needed for IBD therapy. As representatives, S1P receptor modulators have received much attention.
Abnormal lipid metabolism is involved in the pathogenesis of IBD (Alhouayek et al., 2021). Metabolomic analysis showed that sphingomyelin (SM) levels in the ileums of TNF△ARE/WT mice that spontaneously experienced CD-like symptoms were significantly higher than those in WT control mice, indicating that SM may be associated with IBD pathogenesis (Baur et al., 2011). Sphingosine-1-phosphate (S1P) is a type of bioactive sphingolipid. The synthesis of S1P is catalyzed by sphingosine kinase 1/2 (SphK1/2) from sphingosine (Sph) intracellularly and is transferred to the extracellular environment via the S1P transporter (Spiegel et al., 2019). Secreted S1P can bind to five S1P receptors (S1PRs) on the cell membrane of various cell types and further activate downstream signaling, such as Rho/ROCK, PI3K/Akt and ERK, which regulate cell survival, proliferation, migration and apoptosis (Baeyens and Schwab, 2020; Gomez-Larrauri et al., 2020). Plasma S1P levels in UC patients are higher than those in healthy controls (Sun et al., 2019a). The SphK1 inhibitor PF-543 had an inhibitory effect in a DSS-induced colitis mouse model, indicating that the SphK-S1P axis is closely associated with the progression of IBD (Liu and Jiang, 2020).
The most studied mechanism of action of S1P is that it affects the inflammatory immune response by binding to S1PR1 on lymphocytes and regulating the migration of T and B cells out of the peripheral lymphoid tissue (Matloubian et al., 2004). S1PR modulators, which inhibit S1P-S1PR1 signaling, treat IBD by inhibiting the infiltration of lymphocytes into the inflamed intestinal lamina propria (Deguchi et al., 2006; Pérez-Jeldres et al., 2019). S1PR modulators have recently become a hotspot in IBD therapy, and they can induce and help maintain remission in moderate to severe UC and CD with marked improvement of clinical symptoms and few adverse events (Wang et al., 2022a). Beyond the role of S1P in lymphocyte migration, studies have also reported that S1P can be related to changes in the intestinal bacterial composition in IBD (Sun et al., 2019a), and it could affect mucosal inflammation by regulating the intestinal epithelial barrier (Dong et al., 2015) and the phenotypes of immunocytes such as macrophages (Wang et al., 2022b). Thus, in addition to regulating lymphocyte egress, the S1P-S1PR pathway is also related to the gut microbiota, intestinal epithelial cells (IECs) and immune cells in the lamina propria. It may be possible to develop additional drugs that target the S1P-S1PR pathway for intestinal disease. This review discusses the relationship between S1P and the gut microbiota, its various functions in different cell types in the intestinal mucosa, including epithelial cells and innate immune cells, and its roles in IBD pathogenesis. We also investigated the effects of the S1P-S1PR pathway in IBD therapeutics in hopes of developing novel drugs for IBD.
2 S1P: metabolism, receptors and functions
Sphingolipids are a class of bioactive lipids containing a backbone of sphingoid bases, consisting of SM, ceramide (Cer), Sph, S1P, etc. (Gomez-Larrauri et al., 2020). Sphingomyelinase catalyzes SM to generate Cer in the cell membrane or lysosome. The latter is then hydrolyzed by ceramidase to form Sph (Fyrst and Saba, 2010). S1P is generated intracellularly by phosphorylation of Sph by SphK. SphK has two isoenzymes called SphK1 and SphK2 (Pyne et al., 2009). Although SphK1/2 are highly homologous, they are different in their subcellular locations and functions. For example, SphK1 is mainly in the cytosol, while SphK2 exists in intracellular compartments and the cell nucleus (Maceyka et al., 2012). Overexpression of SphK1 promotes cell growth and reduces cell apoptosis, while SphK2 inversely promotes apoptosis (Spiegel and Milstien, 2003). Several studies have indicated that SphK1 levels are positively correlated with the production of secreted S1P (González-Fernández et al., 2017). SphK2 can inhibit SphK1 production, and SphK2 knockout significantly increases S1P expression in serum and colon tissue. S1P has three different modes of degradation that act either intracellularly or extracellularly. S1P phosphatase 1/2 (S1PP1/2) and S1P lyase (S1PL) catalyze S1P degradation intracellularly (Fyrst and Saba, 2010), and lipid phosphate phosphatase dephosphorylates S1P in the extracellular environment (Tang and Brindley, 2020). The activities of SphK1/2, S1PP and S1PL together determine the level of intracellular and secreted S1P, making these proteins potential therapeutic targets for S1P-associated diseases (Figure 1).
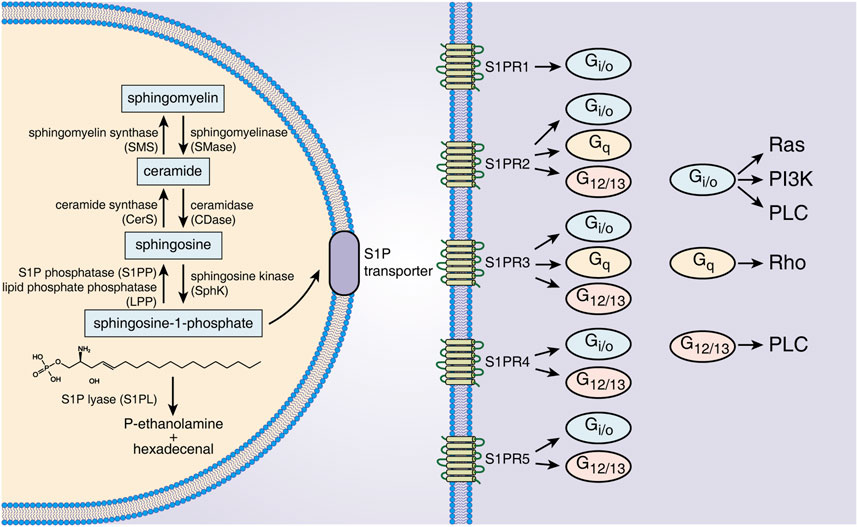
FIGURE 1. S1P metabolism, receptors and downstream signals. S1P is generated from Sph by intracellular SphK1/2 catalysis and is dephosphorylated to Sph by S1PP or irreversibly degraded by S1PL. S1P is transported out of cells through specific transporters on the cell membrane and binds to five S1PRs of the same or other cells. Different S1PRs couple to different G proteins and activate specific downstream signals. Abbreviations: S1P, sphingosine-1-phosphate; SMS, sphingomyelin synthase; SMase, sphingomyelinase; CerS, ceramide synthase; CDase, ceramidase; S1PP, sphingosine-1-phosphate phosphatase; LPP, lipid phosphate phosphatase; SphK, sphingosine kinase; S1PL, sphingosine-1-phosphate lyase; S1PR1∼5, sphingosine-1-phosphate receptor 1–5; Ras, rat sarcoma protein; PI3K, phosphatidylinositol 3-kinase; PLC, phospholipase C; Rho, ras homolog.
S1P regulates cell functions by binding with S1PRs and then activating downstream signals. Intracellularly generated S1P binds to S1PRs on the membrane of targeted cells in autocrine, paracrine and/or endocrine ways, which is called “inside-out signaling” of S1P (Deng et al., 2015). Because S1P has a polar head group, it cannot freely pass through the cell membrane and requires specific transporters on the cell membrane (Nagahashi et al., 2014). The following two types of S1P transporters have been identified: ATP-binding cassette transporters and spinster 2 (Spns2) transporters. Inhibition of these transporters can effectively decrease S1P secretion, and these transporters have been considered a potential target for several immune disease therapies, such as that for IBD, multiple sclerosis and rheumatoid arthritis (Donoviel et al., 2015). The five receptors of S1P (S1PR1-5) are all G protein-coupled receptors located on the cell membrane. S1PR1/2/3 are widely expressed, while S1PR4/5 are mainly expressed in lymph tissue, hemopoietic tissue and the central nervous system (Danese et al., 2018). Different S1PRs couple to different G proteins as follows: S1PR1 couples to Gi/o, S1PR2 and S1PR3 can both couple to G12/13, Gi/o and Gq, and S1PR4 and S1PR5 both couple to Gi/o and G12/13 (Spiegel and Milstien, 2003). By the interaction of S1PRs/G proteins, distinct downstream signals, including that of the ERK, Ras, Rho and PI3K pathways, are activated (Spiegel and Milstien, 2003). The S1P-S1PR1 pathway mainly regulates cell growth, proliferation, migration and differentiation, as well as affecting angiogenesis and lymphocyte egression, which is in opposition to the roles of the S1P-S1PR2 pathway (Wang et al., 2022a). S1P-S1PR3 signaling is closely related to immunocyte chemotaxis, recruitment and maturation (Li et al., 2021). S1PR4 could influence vasoconstriction and immunocyte differentiation, and S1PR5 affects natural killer cell migration (Nielsen et al., 2017). Thus, the distinct biological roles of S1PRs in tissues indicate the wide and complicated effects of S1P-S1PR signaling in different target cells.
3 S1P in IBD pathogenesis
The intestinal mucosal microenvironment mainly consists of the gut microbiota, the epithelial barrier, and innate and adaptive immune cells (Öhman et al., 2015). Bacteria-cell crosstalk and cell‒cell crosstalk in the epithelium are of great importance to intestinal homeostasis, and disordered crosstalk contributes to an abnormal microenvironment that is manifested by intestinal dysbacteriosis, increased intestinal permeability and immune dysfunction, all of which are acknowledged hallmarks of IBD pathogenesis (Zaidi et al., 2020). Due to the widespread expression of S1PRs in different cell types and the increased S1P expression in IBD (Karuppuchamy et al., 2017), in the gut mucosal microenvironment, S1P may have important regulatory roles in gut microbial and cellular function, thereby participating in the development and progression of IBD.
3.1 Expression of S1P in intestinal tissue
S1P expression is different between normal and inflammatory intestines. In normal intestinal tissue, the level of S1P is low due to lower expression of SphK1 and higher expression of S1PL (Suh and Saba, 2015; Karuppuchamy et al., 2017). Of note, SphK1 is overexpressed in the intestinal mucosa of UC and CD patients (Karuppuchamy et al., 2017). The levels of S1P either in the epithelium or lamina propria of the colon are increased in TNBS-induced mouse models (Maines et al., 2010). The expression of S1PRs is different in various cell types in the intestine (Table 1). Under homeostasis, all S1PRs except S1PR4 are present in IECs; among them, S1PR2 has the highest expression, followed by S1PR3 (Chen et al., 2017). Bone marrow-derived macrophages express mainly S1PR1 and S1PR2 with little expression of S1PR3/4 and no expression of S1PR5 (Michaud et al., 2010; Skoura et al., 2011). Immature dendritic cells (DCs) express all five S1PRs, and S1PR1/2/3 are comparatively highly expressed in immature DCs (Xiong et al., 2019). Natural killer cells (NK cells) express all S1PRs except S1PR3 (Drouillard et al., 2018), and neutrophils express all five S1PRs with relatively high expression of S1PR1/4 (Allende et al., 2011). Upon inflammation, epithelial cell damage as well as phenotypic changes in immune cells occur, such as neutrophil activation and transformation of macrophages and DCs into a proinflammatory phenotype, and the expression of S1PRs in various cell types is also changed during this period. A significant increase in S1PR2 expression was observed in macrophages in the intestinal mucosa of UC patients and mouse models (Wang et al., 2022b). LPS stimulates S1PR1/2/3 overexpression in DCs and neutrophils (Perez et al., 2019; Xiong et al., 2019).
The expression of S1PP and S1PL is also different in normal and inflammatory intestines. S1PL is highly expressed in intestinal tissues under homeostasis and is decreased in colitis model mice (Degagné et al., 2014; Karuppuchamy et al., 2017). Karuppuchamy et al. demonstrated that S1PP2 mRNA expression was downregulated in the intestinal tissues of UC patients and in a DSS-induced colitis mouse model. The expression of S1PP1 mRNA was also downregulated in a DSS-induced colitis mouse model (Karuppuchamy et al., 2017). In addition, the expression of the S1P transporter Spns2 is increased in the intestinal mucosa of UC and CD patients, which may contribute to the high S1P levels in the inflamed intestine (Karuppuchamy et al., 2017).
3.2 S1Ps interaction with gut microbiota
Microbiota dysbiosis is an important pathogenesis of IBD that can cause chronic intestinal inflammation. The therapeutic effects of antibiotics, probiotics and fecal microbiota transplantation on IBD reflect the value of therapeutic strategies targeting the gut microbiota (Sartor, 2004; Narula et al., 2017). Firmicutes and Bacteroidota dominate the intestinal flora of healthy adults (Lloyd-Price et al., 2019). The microbiota dysbiosis of IBD patients is characterized by decreased commensal bacterial diversity, increased abundance of Proteobacteria and decreased abundance of Firmicutes and Bacteroidota (Lloyd-Price et al., 2019). The abundances of Prevotellaceae and Ruminococcaceae were decreased in the gut of DSS-induced colitis model mice with an increased abundance of Desulfovibrio (Xie et al., 2019; Liu et al., 2020).
At present, there are limited studies on the relationship between the S1P-S1PR pathway and gut microbiota. Miao et al. reported that SphK2 (a major risk factor for IBD) knockout mice showed an increased abundance of Firmicutes and Verrucomicrobia through 16S rDNA amplicon sequencing of cecal contents (Miao et al., 2022). Uptake of bacteria-secreted particles (BSPs) secreted from enterotoxigenic Bacteroides fragilis by IECs increased SphK1/2 levels in IECs and elevated S1P levels in IEC-derived exosomes. Meanwhile, incubation of IECs with the supernatant of macrophages ingesting enteropathogenic BSPs also increased the expression of SphK1/2 in IECs (Deng et al., 2015). The effects of BSPs secreted from enterotoxigenic B. fragilis may be associated with the high content of sphingolipids in the cell membrane of B. fragilis (Sears, 2009; An et al., 2011). Moreover, the level of plasma S1P in UC patients was negatively correlated with the abundance of beneficial Roseburia in feces but was positively correlated with the abundance of pathogenic Klebsiella and Escherichia-Shigella (Sun et al., 2019a). In conclusion, upregulated SphK-S1P expression can aggravate gut inflammation by modulating the composition of the gut microbiota, resulting in the development of IBD, and intestinal bacteria can also affect the level of S1P in IECs through mutual interactions.
3.3 S1Ps interaction with the intestinal epithelial barrier
The intestinal epithelial barrier is the most important component of the gut mucosal barrier and is the key to defense against the invasion of various pathogens into the intestinal mucosa (Odenwald and Turner, 2017). Epithelial cells, which consist of absorptive cells, goblet cells, neuroendocrine cells, Paneth cells and intestinal stem cells (ISCs), are the main constituents of the intestinal epithelial barrier and play indispensable roles in the maintenance of intestinal homeostasis (Kurokawa et al., 2020). Damage to the intestinal epithelial barrier in IBD results in the translocation of bacteria into the lamina propria of the mucosa, thus initiating a mucosal immune response, causing aggravated intestinal inflammation, further promoting the death of IECs and barrier dysfunction (Wang et al., 2022a). Achieving mucosal healing is an important strategy for the treatment of UC (Kucharzik et al., 2020).
3.3.1 S1P-S1PR signaling helps maintain the intestinal epithelial barrier by strengthening intercellular junctions
Intercellular junctions are the keystone of the intestinal epithelial barrier. Tight junctions (including occludin, claudin protein family and ZO protein family) and adherens junctions (including cadherin and α/β/γ-catenin) are two important components of intercellular junctions. Intestinal epithelial barrier damage caused by intercellular junction dysfunction is an essential factor in IBD pathogenesis (Kaminsky et al., 2021). Upon homeostasis, S1P binding to S1PR2 on the surface of IECs can induce increased expression of intercellular junction-associated proteins such as E-cadherin and ZO-1 and promote E-cadherin localization to the edge of cells, thus strengthening the intestinal epithelial barrier and reducing the permeability of the intestinal epithelium (Greenspon et al., 2011; Chen et al., 2017). Chen et al. found that S1PR2 siRNA could damage intercellular tight junctions by electron microscopy and showed that S1PR2 siRNA significantly increased the fluorescein permeability of the Caco-2 cell layer. The protective effect of S1PR2 on the intestinal epithelium was correlated with activation of ERK1/2 and SphK2 and decreased expression of HDAC1/2 (Chen et al., 2022). Compared with DSS-treated WT mice, S1PR2 knockout mice presented with increased intestinal permeability and elevated levels of IFN-γ, IL-6 and TNF-α in colonic tissues (Chen et al., 2018). The S1PR1 agonist SEW2871 can restore the expression of occludin and ZO-1, which are destroyed by inflammation (Dong et al., 2015). In addition, S1PP2 knockout mice had increased resistance in a DSS-induced colitis mouse model. Compared with WT mice, S1PP2−/− mice had higher intestinal epithelial barrier integrity (manifested as increased expression of E-cadherin) and lower levels of TNF, IL-1β and IL-6 in intestinal tissue in the DSS-induced colitis mouse model (Huang et al., 2016). These results indicate that the S1P-S1PR pathway protects the epithelial barrier by strengthening the cell junctions between IECs. Strengthening of the epithelial barrier reduces the invasion of pathogens into the mucosa, thus inhibiting the activation of immune cells in the lamina propria and reducing mucosal inflammation in IBD.
3.3.2 S1P-S1PR signaling inhibits IEC apoptosis and promotes IEC proliferation
Physiological apoptosis of IECs usually occurs at the top of villi, while apoptosis at crypts is significantly elevated in IBD (Patankar and Becker, 2020). The S1P-S1PR pathway is characterized as antiapoptotic and prosurvival in epithelial cells. The number of TUNEL-positive IECs in the colonic mucosa of S1PP2−/− mice after DSS treatment was significantly reduced compared with that in WT mice, indicating that S1P can suppress IEC apoptosis during colitis (Huang et al., 2016). S1P-S1PR1 signaling can reduce caspase-3 activity by activating the Akt pathway and thus inhibiting IEC apoptosis induced by TNF-α/cycloheximide (Greenspon et al., 2009). It was also confirmed that an S1PR1 agonist can inhibit apoptosis of epithelial cells and improve epithelial barrier function to reduce colitis in an IL-10-knockout colitis mouse model (Dong et al., 2015). Thus, the S1P-S1PR pathway can protect the intestinal epithelial barrier by suppressing IEC apoptosis during gut inflammation.
IECs have a strong ability to regenerate after damage. Inflammatory factors secreted by inflammatory cells can bind to receptors on IECs and activate ISC-associated pathways such as the Hippo or Wnt/β-catenin pathway, which promotes ISC proliferation, migration and differentiation and further accelerates regeneration of the intestinal epithelium (Snippert et al., 2010; Giakountis et al., 2016; Deng et al., 2018). Previous studies have indicated that the S1P-S1PR pathway can promote the proliferation of multiple types of progenitor/stem cells, including endothelial progenitor cells and neural progenitor/stem cells (Ng et al., 2018). S1P can promote IEC proliferation and migration under gut homeostasis. Increased SphK1 expression promotes c-Myc translation by promoting checkpoint kinase 2 expression and HuR phosphorylation, ultimately leading to accelerated IEC proliferation (Jiang et al., 2013; Chen et al., 2017). S1P can also activate p38MAPK to promote the proliferation of Caco-2 cells (Thamilselvan et al., 2002). For epithelial injury, colonoscopic-guided pinch biopsies in the colons of mice caused remarkable increases in SphK1 and S1P in the mucosal wound bed (Montrose et al., 2018), indicating that S1P may participate in repair of the intestinal epithelium after injury. Further studies can deeply explore the mechanism underlying this interesting finding.
3.4 S1Ps interaction with the innate immune system of the gut
The content of S1P is high in blood and lymphatic fluid, while it is low in cell and interstitial fluid. This significant concentration gradient of S1P between efferent lymphatic vessels and lymph nodes drives lymphocytes to transfer into the blood from peripheral lymphoid organs through S1PR1 (Hla et al., 2008). S1PR modulators can block this concentration-dependent regulation of lymphocyte migration from peripheral lymphatic organs and exert a powerful immunosuppressive effect. The effect of S1PR modulators such as ozanimod and etrasimod on IBD has been reviewed in previous studies (Hirten and Sands, 2021; Verstockt et al., 2022). Here, we will review the role of the S1P-S1PR signaling pathway in regulating innate immune cells of the gut, including neutrophils, macrophages, DCs and NK cells, beyond its regulation in adaptive immunity.
3.4.1 S1P and neutrophils
Neutrophils are important components of innate immunity and are generally considered the first responders to inflammation, which is associated with the occurrence of IBD. The effects of S1P on neutrophils under IBD mainly focus on the regulation of cell migration from the bone marrow to peripheral blood and survival. Previous studies have shown that elevated SphK1-S1P in a DSS-induced colitis mouse model led to increased neutrophilia in circulation and increased neutrophil infiltration in the colon (Snider et al., 2009; Pulkoski-Gross et al., 2017). Zhao et al. showed that S1PR1/2/3 was detected in mouse bone marrow neutrophils and differentiated human neutrophil-like cells (dHL60), and S1P powerfully boosted the migration and cytoskeletal remodeling of bone marrow neutrophils through S1PR1 or S1PR2 in liver injury (Zhao et al., 2021), indicating that S1P-S1PR signaling mediated effects on neutrophil recruitment, thus triggering tissue inflammation.
The lifespan of mature neutrophils is short under physiological conditions, while that of activated neutrophils under inflammation is significantly extended, which contributes to the initiation of inflammation (Zhao et al., 2020). S1P can inhibit neutrophil apoptosis by activating Gi/o and downstream p38 MAPK signaling (Chihab et al., 2003; Lin et al., 2011). Activation of the S1P-S1PR2-Gi/o-p38 MAPK pathway can promote the formation of neutrophil extracellular traps (Zhao et al., 2020), which could aggravate and prolong intestinal inflammation and is closely related to the development of IBD (Drury et al., 2021). Moreover, S1PR1/2/3 inhibitors increase caspase-3-dependent neutrophil apoptosis (Perez et al., 2019). Therefore, S1P may promote the development of IBD by inducing neutrophil recruitment and inhibiting neutrophil apoptosis to enhance its proinflammatory activity.
3.4.2 S1P and macrophages
Macrophages are important constituents of the innate immune system that can polarize into the following two phenotypes after different stimuli in the microenvironment: M1 (classically activated macrophages) and M2 (alternatively activated macrophages) (Funes et al., 2018). M1 macrophages mainly produce proinflammatory cytokines (TNF-α, IL-6, IL-23, etc.), which are involved in inflammation aggravation and tissue injury (You et al., 2016). In contrast, M2 macrophages are considered to have anti-inflammatory effects by releasing several anti-inflammatory cytokines (You et al., 2016). Dysregulation of the M1/M2 macrophage balance might be a crucial pathogenesis of IBD. Administering M2 macrophages by intravenous injection significantly alleviates colitis and accelerates wound healing (Liang et al., 2022). The S1P-S1PR pathway is also involved in the regulation of intestinal macrophage polarization. Yang et al. reported that S1PR2/3 promoted the M1 polarization of bone marrow-derived macrophages by activating the G(α)i/o/PI3K/JNK pathway (Yang et al., 2018). Wang et al. demonstrated that S1P acting on S1PR2 induced M1 polarization of intestinal macrophages through the RhoA/ROCK1 pathway in a DSS-induced colitis mouse model (Wang et al., 2022b), revealing that S1P-S1PRs contribute to aggravated intestinal mucosal inflammation by promoting the M1 polarization of macrophages and the production of proinflammatory cytokines.
The infiltration of macrophages in the intestinal mucosa of IBD patients was significantly increased (Kühl et al., 2015; Wang et al., 2022b). Newly infiltrated macrophages have high NF-κB signal activity and mainly secrete proinflammatory cytokines, leading to increased intestinal inflammation (Kühl et al., 2015). Chemokines such as CCL2 and CCL7 are involved in the recruitment of macrophages in the intestinal mucosa in IBD (Kühl et al., 2015; He et al., 2019). S1P-S1PRs can promote the migration of macrophages to local inflamed sites. Studies in the liver (Liao et al., 2018) and lung (Nagahashi et al., 2018) have shown that the S1P-S1PR1 pathway can mediate chemotactic infiltration of macrophages to S1P-rich sites. S1PR2 knockout significantly decreased the number of macrophages in atherosclerotic plaques (Skoura et al., 2011). These studies suggest that S1P-S1PRs mediate macrophage chemotaxis and migration to resident inflamed sites. For the intestinal tract, triptolide downregulated SphK1 and S1PR1/2 expression and inhibited macrophage recruitment in the colons of DSS/AOM-induced colitis-associated cancer model mice in a dose-dependent manner (Li et al., 2020). FTY720, a functional inhibitor of S1PR1, can significantly decrease the number of macrophages in the colonic mucosa of mice infected with Citrobacter rodentium, suggesting that S1P-S1PR1 signaling is involved in the recruitment of macrophages to the injured gut (Murphy et al., 2012). However, it should be noted that S1P-S1PR signaling is also required for macrophage emigration from inflammatory sites during inflammation regression, and inhibition of the S1P-S1PR pathway may block the restoration of tissue homeostasis (Weichand et al., 2013). Therefore, S1P-S1PRs play important roles in macrophage recruitment and emigration at different stages of inflammation, and it is necessary to pay attention to the appropriate timing of administration of drugs targeting the S1P-S1PR pathway.
3.4.3 S1P and dendritic cells
DCs are specialized antigen-presenting cells that act as sentinels in the immune system. DCs mainly include conventional DCs and plasmacytoid DCs. In active IBD patients, chemokines induce the migration of plasmacytoid DCs in circulation to inflamed sites of the gut and promote the production of proinflammatory cytokines such as IL-6 and TNF-α, which play an important role in the initiation of acute inflammation in the intestine (Arimura et al., 2017; Liu et al., 2019). Many factors, such as pathogenic microbial composition, can induce the maturation and activation of DCs and stimulate the expression of costimulatory molecules such as CD86, CD80 and CD40 (Ueno et al., 2010; Mbongue et al., 2017). These costimulatory molecules bind to CD28 or CD40L on the Th0 cell membrane, which triggers DC secretion of IL-12, resulting in T-cell differentiation and activation (Mbongue et al., 2017; Bernardo et al., 2018). DC-LMP1/CD40 chimeric mice spontaneously experienced IBD-like symptoms, manifested with massive granulocyte and lymphocyte infiltration in the intestinal mucosa (Kusters et al., 2017), indicating the pathological role of DCs in IBD.
SphK1-S1P was found to be required for plasmacytoid DC activation and the production of type I IFN and proinflammatory cytokines stimulated by the Toll-like receptor 7/9 ligand (Seo et al., 2012; Mohammed et al., 2020). SphK1-S1P can stimulate the expression of MHC II and CD40/CD80/CD86 in DCs to subsequently induce T-cell activation (Jung et al., 2007; Xu et al., 2013). FTY720, a functional inhibitor of S1PR1, significantly downregulates the number of CD11chiMHC II+CD4+DCs in the bone marrow of CLP mice (a human multibacterial bacteremia model) (Smirnov et al., 2017). Moreover, knockout of S1PR2 or use of its inhibitor suppressed LPS-induced DC maturation, accompanied by decreased expression of MHC II and CD86, CD80, and CD40 in DCs (Park and Im, 2019). Additionally, S1P-S1PR4 signaling is required for plasmacytoid DC progenitor cells to differentiate into plasmacytoid DCs (Dillmann et al., 2015). S1P could promote the secretion of proinflammatory cytokines, including IL-6 and IL-8, in mature DCs in the presence of stimulus (Oz-Arslan et al., 2006). These results indicate that the S1P-S1PR pathway could regulate DC maturation and activation, which are important for triggering the inflammatory response in mucosal immunity.
3.5 Contradiction of the role of S1P in the epithelium and lamina propria and crosstalk
Based on the above studies, the regulatory effect of S1P-S1PR signaling on the intestinal mucosa is proinflammatory. This was further confirmed by performing animal experiments using PF-543 (a SphK1 inhibitor), which inhibits DSS-induced colitis (Liu and Jiang, 2020), and gut-specific knockout of S1PL, which leads to increased S1P levels in the colon and aggravates IBD symptoms (Suh and Saba, 2015). However, it should be noted that the use of 4-deoxypyridoxine hydrochloride and 2-acetyl-4 (tetrahydroxybutyl) imidazole, which markedly increase local intestinal S1P levels, attenuates CD-like chronic ileitis in TNF△ARE mice by suppressing thymocyte maturation (Karuppuchamy et al., 2020). These different roles of S1P reveal the complicated functions of S1P in distinct biological processes. In addition, it should not be ignored that the S1P-S1PR axis can also promote the proliferation and repair of epithelial cells and may protect the intestinal epithelial barrier (Figure 2). Huang et al. demonstrated that epithelial-specific knockout of S1PP2 in mice led to milder colitis, lower levels of TNF-α, IL-6 and IL-1β, and higher mucosal integrity than that in WT mice after DSS induction, suggesting the protective effect of epithelial S1P on the intestinal mucosal barrier under acute colitis (Huang et al., 2016). However, the effects of S1P on the intestinal epithelial barrier under chronic colitis are still poorly understood.
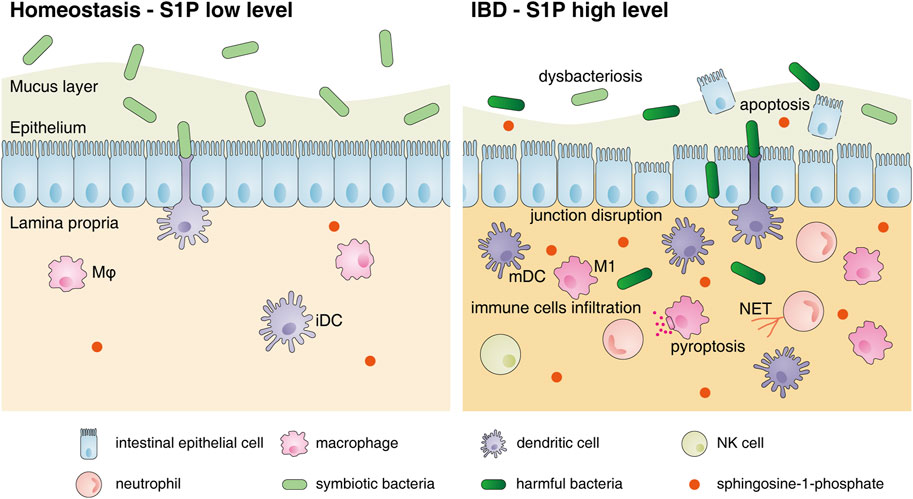
FIGURE 2. Changes in the intestinal mucosal microenvironment and S1P functions under IBD. The changes in the intestinal mucosal microenvironment under IBD conditions are as follows: decreased commensal bacterial diversity, increased IEC apoptosis, destroyed intercellular connections and increased infiltration of immune cells, including neutrophils, macrophages, DCs and NK cells, in the lamina propria. S1P can restore the connections between IECs and inhibit their apoptosis to protect the intestinal epithelial barrier. However, S1P also promotes the migration of various innate immune cells to inflamed sites, induces neutrophils to form NETs, stimulates M1 polarization and accelerates DC activation to aggravate intestinal inflammation. Abbreviations: S1P, sphingosine-1-phosphate; Mφ, macrophage; iDC, immature dendritic cell; mDC, mature dendritic cell; M1, classically activated macrophages; NET, neutrophil extracellular trap.
S1PR modulators, represented by fingolimod and etrasimod or ozanimod, which more selectivity targets S1PR1, cause the internalization, degradation and subsequent downregulation of this receptor (Jo et al., 2005). These drugs have a profound anti-inflammatory effect by preventing the migration of lymphocytes into peripheral tissues. Ozanimod and etrasimod have been tested for effectiveness and safety in IBD patients in several clinical trials (Wang et al., 2022a). However, less attention has been focused on the direct effects of S1PR modulators on the intestinal epithelium. SEW2871, an S1PR1 agonist, has been proven to be therapeutic in IL-10 gene-deficient mice by reducing IEC apoptosis and improving epithelial barrier function (Dong et al., 2015). Additionally, S1PR1 was proven to inhibit TNF-α/cycloheximide-induced IEC apoptosis in a cell experiment (Greenspon et al., 2009), indicating the protective role of S1PR1 on the intestinal epithelium under inflammation. Thus, it is valuable to explore the effect of S1P modulators on IECs when S1PR1 signaling in IECs is inhibited. In addition, S1PR2, another S1P receptor that is independent of the pharmacology of S1PR modulators, is involved in various cell functions in the intestine. Chen et al. revealed the general pathogenic role of S1PR2 in a DSS-induced colitis mouse model (Chen et al., 2022). S1PR2 upregulates the expression of serum TNF-α and IL-18, as well as IL-18 in colon tissue, which is consistent with its proinflammatory effects on innate immune cells discussed above. However, as the most highly expressed S1PR in IECs under homeostasis, S1PR2 promotes the proliferation of IECs and protects the epithelial barrier. These contradictory results suggest the complicated regulatory functions of S1P in the intestinal microenvironment and that cell-specific inhibition of S1P may need to be considered in the development of new drugs to avoid inhibiting intestinal epithelial repair during anti-inflammation periods.
The crosslink between IECs and immune cells in the lamina propria is an important field in IBD research. The cytokines and factors secreted from epithelial cells or immune cells in the lamina propria can mutually achieve message transfer and thus impact signal transduction and cellular function. Our previous studies also confirmed that miR-590-3p in M2 macrophage-derived exosomes could promote epithelial cell regeneration and alleviate DSS-induced mucosal damage by activating the LATS1/YAP/β-Catenin pathway in IECs (Deng et al., 2021). S1P, as a bioactive molecule acting on cell membranes of its own or adjacent cells by autocrine or paracrine mechanisms to activate downstream pathways, may act as a “signal transmitter” between IECs and immune cells. Studies have confirmed that the phagocytosis activity of alveolar macrophages was positively correlated with the expression level of Spns2 in bronchial epithelial cells, suggesting the regulatory role of epithelial-derived Spns2 on macrophages (Tran et al., 2017). Exosomes can be produced by various types of cells and construct signal interactions between different cells. Enteropathogenic BSPs can stimulate SphK1 expression in MC38 cells and then increase the expression of S1P in IEC-derived exosomes (Deng et al., 2015). Moreover, the biological effects of S1P contained in intestinal mucosa-derived exosome-like nanoparticles promoted colon cancer in mice (Deng et al., 2015), suggesting the important regulation of S1P in the epithelium and lamina propria during gut inflammation and cancer.
4 S1Ps involvement in IBD treatment
S1PR modulators, as a novel kind of small molecule drug, have been considered potential therapies for IBD (Verstockt et al., 2022). The side effects of fingolimod, including heart rate reduction and temporary delay in atrioventricular conduction, due to its nontherapeutic binding with S1PRs in the cardiovascular system, limit its clinical use in IBD (Camm et al., 2014). However, this shortcoming was well overcome with the emergence of selective S1PR modulators targeting S1PR1, such as ozanimod and etrasimod, which have been used for IBD treatment. Ozanimod was approved by the US FDA and the European Commission for the treatment of UC in 2021, while further clinical trials are ongoing to confirm its efficacy and safety in adult and pediatric UC and CD patients. Based on previous studies, UC patients who received either ozanimod or etrasimod showed a higher incidence of clinical remission and response than those who received placebo (Sandborn et al., 2021; Vermeire et al., 2021; Sandborn et al., 2023). Ozanimod is also considered “a promising new player” due to its ideal curative effects in the treatment of CD (Feagan et al., 2020; Sleutjes et al., 2020). Adverse reactions to ozanimod and etrasimod that need attention include worsening of UC/CD, infection, and anemia and elevated liver aminotransferase levels (Feagan et al., 2020; Sandborn et al., 2021; Vermeire et al., 2021; Sandborn et al., 2023). Cardiovascular side effects were not significant and included transient, asymptomatic and low-grade atrioventricular block in only a few patients who received etrasimod (Sandborn et al., 2020). Altogether, S1PR modulators are promising to open a new era of IBD treatment due to their unique therapeutic targeting.
In addition to targeting S1PR1, increasing studies have also suggested the therapeutic effects of other factors in the S1P-S1PR pathway on IBD, but they were still limited to preclinical studies. PF543, a SphK1-specific inhibitor, improved colitis symptoms in a DSS-induced colitis mouse model and downregulated IL-1β and IL-6 mRNA levels in the colon (Sun et al., 2019b). Another SphK1-specific inhibitor, LCL351, has similar therapeutic effects on DSS-induced mice (Pulkoski-Gross et al., 2017). Knockout of the S1P transporter Spns2 significantly improved DSS- or oxazolone-induced colitis in mice (Donoviel et al., 2015). The S1PL inhibitor 4-deoxypyridoxine can reduce CD4+CD8+ double-positive immature thymocytes in the thymus and lead to the accumulation and apoptosis of mature thymocytes in the thymus medulla, thereby exerting a central immunosuppressive effect and improving CD-like ileitis in TNFΔARE mice (Karuppuchamy et al., 2020). In addition to receptor inhibitors targeting S1PR1, the S1PR2 inhibitor JTE-013 significantly reduced the histological score of colon inflammation in a DSS-induced colitis mouse model (Chen et al., 2022). Therefore, all factors in the SphK-S1P-S1PR pathway might serve as therapeutic targets for IBD, while because of the wide distribution of S1PRs in the body and the distinct effects mediated by S1PRs in cells, higher receptor selectivity should be considered in the development of S1P-related drugs.
Many IBD treatments have been found to be associated with S1P-S1PRs. Mesalazine reduced colon inflammation by decreasing the serum level of S1P in DSS-treated mice (Sun et al., 2019b). Stem cell therapy is a new treatment method for IBD (Misselwitz et al., 2020). Adipose-derived mesenchymal stem cell transplantation significantly downregulates SphK1 mRNA levels in the colonic mucosa of TNBS-induced mice to resolve colitis, and SphK1 mRNA can be further decreased by combination therapy with mesenchymal stromal cells and sulfasalazine (Yousefi-Ahmadipour et al., 2019). Metformin can reduce oxazolidone-induced colitis by downregulating plasma SphK1-S1P activity in rats (El-Mahdy et al., 2021). In addition, some active ingredients in plants, such as hesperidin and baicalin, have also been confirmed to alleviate DSS-induced colitis by inhibiting the SphK1-S1P-S1PR signaling pathway (Shafik et al., 2019; Yao et al., 2020).
5 Conclusion
In conclusion, S1P, a bioactive molecule, is widely distributed in the intestinal mucosa and its expression is increased in IBD. It exerts different biological effects on various cell types in the intestinal mucosa. Increased S1P can disturb the structural balance of the gut microflora and promote the development of colitis. For intestinal epithelial cells, S1P can promote cell proliferation and migration, inhibit cell apoptosis and strengthen intercellular connections to protect the intestinal epithelial barrier. For innate immune cells, S1P is prone to exert a proinflammatory effect to trigger and aggravate inflammation. S1P can promote the migration of immune cells to inflamed sites, inhibit the apoptosis of neutrophils, induce M1 macrophage polarization, and promote the maturation and activation of DCs to enhance the production of proinflammatory cytokines. SphK-S1P-S1PR pathway-associated drugs were newly used in clinical and animal studies for IBD treatment. In summary, beyond the role of S1P in lymphocyte trafficking, S1P exerts important and complicated effects on the regulation of different cell functions in the mucosal microenvironment of colitis. Selective S1P receptor modulators are needed to avoid inhibition of epithelial repair when exerting their anti-inflammatory functions in immune cells. Further in-depth and larger studies could focus on this interesting regulation of S1P in the intestinal mucosa and its roles in gut inflammation and IBD.
Author contributions
FZ: study concept and manuscript draft; SW: illustration drawing and manuscript revision; MX and ZW: manuscript revision; FD: manuscript revision, study supervision and obtained funding. All authors contributed to the article and approved the submitted version.
Funding
This work was supported by the National Natural Science Foundation of China (grant number 81900478).
Conflict of interest
The authors declare that the research was conducted in the absence of any commercial or financial relationships that could be construed as a potential conflict of interest.
Publisher’s note
All claims expressed in this article are solely those of the authors and do not necessarily represent those of their affiliated organizations, or those of the publisher, the editors and the reviewers. Any product that may be evaluated in this article, or claim that may be made by its manufacturer, is not guaranteed or endorsed by the publisher.
Abbreviations
BSP, bacteria-secreted particle; CD, Crohn’s disease; Cer, ceramide; DC, dendritic cell; IBD, inflammatory bowel disease; IEC, intestinal epithelial cell; ISC, intestinal stem cell; M1, classically activated macrophage; M2, alternative activated macrophage; S1P, sphingosine-1-phosphate; S1PR, sphingosine-1-phosphate receptor; SM, sphingomyelin; Spns2, spinster 2; Sph, sphingosine; SphK1/2, sphingosine kinase 1/2; S1PL, sphingosine-1-phosphate lyase; S1PP1/2, sphingosine-1-phosphate phosphatase 1/2; UC, ulcerative colitis.
References
Al-Bawardy, B., Shivashankar, R., and Proctor, D. D. (2021). Novel and emerging therapies for inflammatory bowel disease. Front. Pharmacol. 12, 651415. doi:10.3389/fphar.2021.651415
Alhouayek, M., Ameraoui, H., and Muccioli, G. G. (2021). Bioactive lipids in inflammatory bowel diseases - from pathophysiological alterations to therapeutic opportunities. Biochimica Biophysica Acta. Mol. Cell Biol. Lipids 1866 (2), 158854. doi:10.1016/j.bbalip.2020.158854
Allende, M. L., Bektas, M., Lee, B. G., Bonifacino, E., Kang, J., Tuymetova, G., et al. (2011). Sphingosine-1-phosphate lyase deficiency produces a pro-inflammatory response while impairing neutrophil trafficking. J. Biol. Chem. 286 (9), 7348–7358. doi:10.1074/jbc.M110.171819
An, D., Na, C., Bielawski, J., Hannun, Y. A., and Kasper, D. L. (2011). Membrane sphingolipids as essential molecular signals for Bacteroides survival in the intestine. Proc. Natl. Acad. Sci. U. S. A. 108 (1), 4666–4671. doi:10.1073/pnas.1001501107
Arimura, K., Takagi, H., Uto, T., Fukaya, T., Nakamura, T., Choijookhuu, N., et al. (2017). Crucial role of plasmacytoid dendritic cells in the development of acute colitis through the regulation of intestinal inflammation. Mucosal Immunol. 10 (4), 957–970. doi:10.1038/mi.2016.96
Baeyens, A. A. L., and Schwab, S. R. (2020). Finding a way out: S1P signaling and immune cell migration. Annu. Rev. Immunol. 38, 759–784. doi:10.1146/annurev-immunol-081519-083952
Baur, P., Martin, F.-P., Gruber, L., Bosco, N., Brahmbhatt, V., Collino, S., et al. (2011). Metabolic phenotyping of the Crohn's disease-like IBD etiopathology in the TNF(ΔARE/WT) mouse model. J. Proteome Res. 10 (12), 5523–5535. doi:10.1021/pr2007973
Bernardo, D., Chaparro, M., and Gisbert, J. P. (2018). Human intestinal dendritic cells in inflammatory bowel diseases. Mol. Nutr. Food Res. 62 (7), e1700931. doi:10.1002/mnfr.201700931
Camm, J., Hla, T., Bakshi, R., and Brinkmann, V. (2014). Cardiac and vascular effects of fingolimod: Mechanistic basis and clinical implications. Am. Heart J. 168 (5), 632–644. doi:10.1016/j.ahj.2014.06.028
Chen, T., Gu, K., Lin, R., Liu, Y., and Shan, Y. (2022). The function of Sphingosine-1-phosphate receptor 2 (S1PR2) in maintaining intestinal barrier and inducing ulcerative colitis. Bioengineered 13 (5), 13703–13717. doi:10.1080/21655979.2022.2076500
Chen, T., Huang, Z., Liu, R., Yang, J., Hylemon, P. B., and Zhou, H. (2017). Sphingosine-1 phosphate promotes intestinal epithelial cell proliferation via S1PR2. Front. Biosci. (Landmark Ed. 22 (4), 596–608. doi:10.2741/4505
Chen, T., Lin, R., Jin, S., Chen, R., Xue, H., Ye, H., et al. (2018). The sphingosine-1-phosphate/sphingosine-1-phosphate receptor 2 Axis in intestinal epithelial cells regulates intestinal barrier function during intestinal epithelial cells-cd4+t-cell interactions. Cell. Physiology Biochem. 48 (3), 1188–1200. doi:10.1159/000491985
Chihab, R., Pörn-Ares, M. I., Alvarado-Kristensson, M., and Andersson, T. (2003). Sphingosine 1-phosphate antagonizes human neutrophil apoptosis via p38 mitogen-activated protein kinase. Cell. Mol. Life Sci. CMLS 60 (4), 776–785. doi:10.1007/s00018-003-2357-8
Danese, S., Furfaro, F., and Vetrano, S. (2018). Targeting S1P in inflammatory bowel disease: New avenues for modulating intestinal leukocyte migration. J. Crohn's Colitis 12 (2), S678–S686. doi:10.1093/ecco-jcc/jjx107
Degagné, E., Pandurangan, A., Bandhuvula, P., Kumar, A., Eltanawy, A., Zhang, M., et al. (2014). Sphingosine-1-phosphate lyase downregulation promotes colon carcinogenesis through STAT3-activated microRNAs. J. Clin. Investigation 124 (12), 5368–5384. doi:10.1172/JCI74188
Deguchi, Y., Andoh, A., Yagi, Y., Bamba, S., Inatomi, O., Tsujikawa, T., et al. (2006). The S1P receptor modulator FTY720 prevents the development of experimental colitis in mice. Oncol. Rep. 16 (4), 699–703. doi:10.3892/or.16.4.699
Deng, F., Peng, L., Li, Z., Tan, G., Liang, E., Chen, S., et al. (2018). YAP triggers the Wnt/β-catenin signalling pathway and promotes enterocyte self-renewal, regeneration and tumorigenesis after DSS-induced injury. Cell Death Dis. 9 (2), 153. doi:10.1038/s41419-017-0244-8
Deng, F., Yan, J., Lu, J., Luo, M., Xia, P., Liu, S., et al. (2021). M2 macrophage-derived exosomal miR-590-3p attenuates DSS-induced mucosal damage and promotes epithelial repair via the LATS1/YAP/β-catenin signalling Axis. J. Crohn's Colitis 15 (4), 665–677. doi:10.1093/ecco-jcc/jjaa214
Deng, Z., Mu, J., Tseng, M., Wattenberg, B., Zhuang, X., Egilmez, N. K., et al. (2015). Enterobacteria-secreted particles induce production of exosome-like S1P-containing particles by intestinal epithelium to drive Th17-mediated tumorigenesis. Nat. Commun. 6, 6956. doi:10.1038/ncomms7956
Dillmann, C., Mora, J., Olesch, C., Brüne, B., and Weigert, A. (2015). S1PR4 is required for plasmacytoid dendritic cell differentiation. Biol. Chem. 396 (6-7), 775–782. doi:10.1515/hsz-2014-0271
Dong, J., Wang, H., Zhao, J., Sun, J., Zhang, T., Zuo, L., et al. (2015). SEW2871 protects from experimental colitis through reduced epithelial cell apoptosis and improved barrier function in interleukin-10 gene-deficient mice. Immunol. Res. 61 (3), 303–311. doi:10.1007/s12026-015-8625-5
Donoviel, M. S., Hait, N. C., Ramachandran, S., Maceyka, M., Takabe, K., Milstien, S., et al. (2015). Spinster 2, a sphingosine-1-phosphate transporter, plays a critical role in inflammatory and autoimmune diseases. FASEB J. 29 (12), 5018–5028. doi:10.1096/fj.15-274936
Drouillard, A., Mathieu, A.-L., Marçais, A., Belot, A., Viel, S., Mingueneau, M., et al. (2018). S1PR5 is essential for human natural killer cell migration toward sphingosine-1 phosphate. J. Allergy Clin. Immunol. 141 (6), 2265–2268. doi:10.1016/j.jaci.2017.11.022
Drury, B., Hardisty, G., Gray, R. D., and Ho, G.-T. (2021). Neutrophil extracellular traps in inflammatory bowel disease: Pathogenic mechanisms and clinical translation. Cell. Mol. Gastroenterology Hepatology 12 (1), 321–333. doi:10.1016/j.jcmgh.2021.03.002
El-Mahdy, N. A., El-Sayad, M. E.-S., El-Kadem, A. H., and Abu-Risha, S. E.-S. (2021). Metformin alleviates inflammation in oxazolone induced ulcerative colitis in rats: Plausible role of sphingosine kinase 1/sphingosine 1 phosphate signaling pathway. Immunopharmacol. Immunotoxicol. 43 (2), 192–202. doi:10.1080/08923973.2021.1878214
Feagan, B. G., Sandborn, W. J., Danese, S., Wolf, D. C., Liu, W. J., Hua, S. Y., et al. (2020). Ozanimod induction therapy for patients with moderate to severe crohn's disease: A single-arm, phase 2, prospective observer-blinded endpoint study. Lancet. Gastroenterology Hepatology 5 (9), 819–828. doi:10.1016/S2468-1253(20)30188-6
Funes, S. C., Rios, M., Escobar-Vera, J., and Kalergis, A. M. (2018). Implications of macrophage polarization in autoimmunity. Immunology 154 (2), 186–195. doi:10.1111/imm.12910
Fyrst, H., and Saba, J. D. (2010). An update on sphingosine-1-phosphate and other sphingolipid mediators. Nat. Chem. Biol. 6 (7), 489–497. doi:10.1038/nchembio.392
Giakountis, A., Moulos, P., Zarkou, V., Oikonomou, C., Harokopos, V., Hatzigeorgiou, A. G., et al. (2016). A positive regulatory loop between a wnt-regulated non-coding RNA and ASCL2 controls intestinal stem cell fate. Cell Rep. 15 (12), 2588–2596. doi:10.1016/j.celrep.2016.05.038
Gomez-Larrauri, A., Presa, N., Dominguez-Herrera, A., Ouro, A., Trueba, M., and Gomez-Muñoz, A. (2020). Role of bioactive sphingolipids in physiology and pathology. Essays Biochem. 64 (3), 579–589. doi:10.1042/EBC20190091
González-Fernández, B., Sánchez, D. I., Crespo, I., San-Miguel, B., Álvarez, M., Tuñón, M. J., et al. (2017). Inhibition of the SphK1/S1P signaling pathway by melatonin in mice with liver fibrosis and human hepatic stellate cells. BioFactors Oxf. Engl. 43 (2), 272–282. doi:10.1002/biof.1342
Greenspon, J., Li, R., Xiao, L., Rao, J. N., Marasa, B. S., Strauch, E. D., et al. (2009). Sphingosine-1-phosphate protects intestinal epithelial cells from apoptosis through the Akt signaling pathway. Dig. Dis. Sci. 54 (3), 499–510. doi:10.1007/s10620-008-0393-9
Greenspon, J., Li, R., Xiao, L., Rao, J. N., Sun, R., Strauch, E. D., et al. (2011). Sphingosine-1-phosphate regulates the expression of adherens junction protein E-cadherin and enhances intestinal epithelial cell barrier function. Dig. Dis. Sci. 56 (5), 1342–1353. doi:10.1007/s10620-010-1421-0
He, J., Song, Y., Li, G., Xiao, P., Liu, Y., Xue, Y., et al. (2019). Fbxw7 increases CCL2/7 in CX3CR1hi macrophages to promote intestinal inflammation. J. Clin. Investigation 129 (9), 3877–3893. doi:10.1172/JCI123374
Hirten, R. P., and Sands, B. E. (2021). New therapeutics for ulcerative colitis. Annu. Rev. Med. 72, 199–213. doi:10.1146/annurev-med-052919-120048
Hla, T., Venkataraman, K., and Michaud, J. (2008). The vascular S1P gradient-cellular sources and biological significance. Biochimica Biophysica Acta 1781 (9), 477–482. doi:10.1016/j.bbalip.2008.07.003
Huang, W.-C., Liang, J., Nagahashi, M., Avni, D., Yamada, A., Maceyka, M., et al. (2016). Sphingosine-1-phosphate phosphatase 2 promotes disruption of mucosal integrity, and contributes to ulcerative colitis in mice and humans. FASEB J. 30 (8), 2945–2958. doi:10.1096/fj.201600394R
Jiang, P., Smith, A. D., Li, R., Rao, J. N., Liu, L., Donahue, J. M., et al. (2013). Sphingosine kinase 1 overexpression stimulates intestinal epithelial cell proliferation through increased c-Myc translation. Am. J. Physiology. Cell Physiology 304 (12), C1187–C1197. doi:10.1152/ajpcell.00271.2012
Jo, E., Sanna, M. G., Gonzalez-Cabrera, P. J., Thangada, S., Tigyi, G., Osborne, D. A., et al. (2005). S1P1-selective in vivo-active agonists from high-throughput screening: Off-the-shelf chemical probes of receptor interactions, signaling, and fate. Chem. Biol. 12 (6), 703–715. doi:10.1016/j.chembiol.2005.04.019
Jung, I. D., Lee, J. S., Kim, Y. J., Jeong, Y.-I., Lee, C.-M., Baumruker, T., et al. (2007). Sphingosine kinase inhibitor suppresses a Th1 polarization via the inhibition of immunostimulatory activity in murine bone marrow-derived dendritic cells. Int. Immunol. 19 (4), 411–426. doi:10.1093/intimm/dxm006
Kaminsky, L. W., Al-Sadi, R., and Ma, T. Y. (2021). IL-1β and the intestinal epithelial tight junction barrier. Front. Immunol. 12, 767456. doi:10.3389/fimmu.2021.767456
Karuppuchamy, T., Behrens, E. H., González-Cabrera, P., Sarkisyan, G., Gima, L., Boyer, J. D., et al. (2017). Sphingosine-1-phosphate receptor-1 (S1P1) is expressed by lymphocytes, dendritic cells, and endothelium and modulated during inflammatory bowel disease. Mucosal Immunol. 10 (1), 162–171. doi:10.1038/mi.2016.35
Karuppuchamy, T., Tyler, C. J., Lundborg, L. R., Pérez-Jeldres, T., Kimball, A. K., Clambey, E. T., et al. (2020). Sphingosine-1-Phosphate lyase inhibition alters the S1P gradient and ameliorates crohn's-like ileitis by suppressing thymocyte maturation. Inflamm. Bowel Dis. 26 (2), 216–228. doi:10.1093/ibd/izz174
Kucharzik, T., Koletzko, S., Kannengiesser, K., and Dignass, A. (2020). Ulcerative colitis-diagnostic and therapeutic algorithms. Dtsch. Arzteblatt Int. 117 (33-34), 564–574. doi:10.3238/arztebl.2020.0564
Kühl, A. A., Erben, U., Kredel, L. I., and Siegmund, B. (2015). Diversity of intestinal macrophages in inflammatory bowel diseases. Front. Immunol. 6, 613. doi:10.3389/fimmu.2015.00613
Kurokawa, K., Hayakawa, Y., and Koike, K. (2020). Plasticity of intestinal epithelium: Stem cell niches and regulatory signals. Int. J. Mol. Sci. 22 (1), 357. doi:10.3390/ijms22010357
Kusters, P., Seijkens, T., Bürger, C., Legein, B., Winkels, H., Gijbels, M., et al. (2017). Constitutive CD40 signaling in dendritic cells limits atherosclerosis by provoking inflammatory bowel disease and ensuing cholesterol malabsorption. Am. J. Pathology 187 (12), 2912–2919. doi:10.1016/j.ajpath.2017.08.016
Li, H., Xing, X., Zhang, X., Li, L., Jiang, Z., Wang, T., et al. (2020). Effects of triptolide on the sphingosine kinase - sphingosine-1-phosphate signaling pathway in colitis-associated colon cancer. Int. Immunopharmacol. 88, 106892. doi:10.1016/j.intimp.2020.106892
Li, Q., Li, Y., Lei, C., Tan, Y., and Yi, G. (2021). Sphingosine-1-phosphate receptor 3 signaling. Clin. Chimica Acta; Int. J. Clin. Chem. 519, 32–39. doi:10.1016/j.cca.2021.03.025
Liang, L., Liu, L., Zhou, W., Yang, C., Mai, G., Li, H., et al. (2022). Gut microbiota-derived butyrate regulates gut mucus barrier repair by activating the macrophage/WNT/ERK signaling pathway. Clin. Sci. Lond. Engl. 1979) 136 (4), 291–307. doi:10.1042/CS20210778
Liao, C.-Y., Song, M. J., Gao, Y., Mauer, A. S., Revzin, A., and Malhi, H. (2018). Hepatocyte-derived lipotoxic extracellular vesicle sphingosine 1-phosphate induces macrophage chemotaxis. Front. Immunol. 9, 2980. doi:10.3389/fimmu.2018.02980
Lin, W.-C., Lin, C.-F., Chen, C.-L., Chen, C.-W., and Lin, Y.-S. (2011). Inhibition of neutrophil apoptosis via sphingolipid signaling in acute lung injury. J. Pharmacol. Exp. Ther. 339 (1), 45–53. doi:10.1124/jpet.111.181560
Liu, B., Piao, X., Niu, W., Zhang, Q., Ma, C., Wu, T., et al. (2020). Kuijieyuan decoction improved intestinal barrier injury of ulcerative colitis by affecting TLR4-dependent PI3K/AKT/NF-κB oxidative and inflammatory signaling and gut microbiota. Front. Pharmacol. 11, 1036. doi:10.3389/fphar.2020.01036
Liu, H., Dasgupta, S., Fu, Y., Bailey, B., Roy, C., Lightcap, E., et al. (2019). Subsets of mononuclear phagocytes are enriched in the inflamed colons of patients with IBD. BMC Immunol. 20 (1), 42. doi:10.1186/s12865-019-0322-z
Liu, J., and Jiang, B. (2020). Sphk1 promotes ulcerative colitis via activating JAK2/STAT3 signaling pathway. Hum. Cell 33 (1), 57–66. doi:10.1007/s13577-019-00283-z
Lloyd-Price, J., Arze, C., Ananthakrishnan, A. N., Schirmer, M., Avila-Pacheco, J., Poon, T. W., et al. (2019). Multi-omics of the gut microbial ecosystem in inflammatory bowel diseases. Nature 569 (7758), 655–662. doi:10.1038/s41586-019-1237-9
Maceyka, M., Harikumar, K. B., Milstien, S., and Spiegel, S. (2012). Sphingosine-1-phosphate signaling and its role in disease. Trends Cell Biol. 22 (1), 50–60. doi:10.1016/j.tcb.2011.09.003
Maines, L. W., Fitzpatrick, L. R., Green, C. L., Zhuang, Y., and Smith, C. D. (2010). Efficacy of a novel sphingosine kinase inhibitor in experimental Crohn's disease. Inflammopharmacology 18 (2), 73–85. doi:10.1007/s10787-010-0032-x
Matloubian, M., Lo, C. G., Cinamon, G., Lesneski, M. J., Xu, Y., Brinkmann, V., et al. (2004). Lymphocyte egress from thymus and peripheral lymphoid organs is dependent on S1P receptor 1. Nature 427 (6972), 355–360. doi:10.1038/nature02284
Mbongue, J. C., Nieves, H. A., Torrez, T. W., and Langridge, W. H. R. (2017). The role of dendritic cell maturation in the induction of insulin-dependent diabetes mellitus. Front. Immunol. 8, 327. doi:10.3389/fimmu.2017.00327
Miao, R.-R., Zhan, S., Cui, S.-X., and Qu, X.-J. (2022). Intestinal aberrant sphingolipid metabolism shaped-gut microbiome and bile acids metabolome in the development of hepatic steatosis. FASEB J. 36 (8), e22398. doi:10.1096/fj.202200148RR
Michaud, J., Im, D.-S., and Hla, T. (2010). Inhibitory role of sphingosine 1-phosphate receptor 2 in macrophage recruitment during inflammation. J. Immunol. Baltim. Md, 1950) 184 (3), 1475–1483. doi:10.4049/jimmunol.0901586
Misselwitz, B., Juillerat, P., Sulz, M. C., Siegmund, B., and Brand, S.Swiss IBDnet, an official working group of the Swiss Society of Gastroenterology (2020). Emerging treatment options in inflammatory bowel disease: Janus kinases, stem cells, and more. Digestion 101 (1), 69–82. doi:10.1159/000507782
Mohammed, S., Vineetha, N. S., James, S., Aparna, J. S., Babu Lankadasari, M., Maeda, T., et al. (2020). Regulatory role of SphK1 in TLR7/9-dependent type I interferon response and autoimmunity. FASEB J. 34 (3), 4329–4347. doi:10.1096/fj.201902847R
Montrose, D. C., Zhou, X. K., McNally, E. M., Sue, E., Wang, H., Nishiguchi, R., et al. (2018). Colonoscopic-guided pinch biopsies in mice as a useful model for evaluating the roles of host and luminal factors in colonic inflammation. Am. J. Pathology 188 (12), 2811–2825. doi:10.1016/j.ajpath.2018.08.016
Murphy, C. T., Hall, L. J., Hurley, G., Quinlan, A., MacSharry, J., Shanahan, F., et al. (2012). The sphingosine-1-phosphate analogue FTY720 impairs mucosal immunity and clearance of the enteric pathogen Citrobacter rodentium. Infect. Immun. 80 (8), 2712–2723. doi:10.1128/IAI.06319-11
Nagahashi, M., Takabe, K., Terracina, K. P., Soma, D., Hirose, Y., Kobayashi, T., et al. (2014). Sphingosine-1-phosphate transporters as targets for cancer therapy. BioMed Res. Int. 2014, 651727. doi:10.1155/2014/651727
Nagahashi, M., Yamada, A., Katsuta, E., Aoyagi, T., Huang, W.-C., Terracina, K. P., et al. (2018). Targeting the SphK1/S1P/S1PR1 Axis that links obesity, chronic inflammation, and breast cancer metastasis. Cancer Res. 78 (7), 1713–1725. doi:10.1158/0008-5472.CAN-17-1423
Narula, N., Kassam, Z., Yuan, Y., Colombel, J.-F., Ponsioen, C., Reinisch, W., et al. (2017). Systematic review and meta-analysis: Fecal microbiota transplantation for treatment of active ulcerative colitis. Inflamm. Bowel Dis. 23 (10), 1702–1709. doi:10.1097/MIB.0000000000001228
Ng, M. L., Yarla, N. S., Menschikowski, M., and Sukocheva, O. A. (2018). Regulatory role of sphingosine kinase and sphingosine-1-phosphate receptor signaling in progenitor/stem cells. World J. Stem Cells 10 (9), 119–133. doi:10.4252/wjsc.v10.i9.119
Ng, S. C., Shi, H. Y., Hamidi, N., Underwood, F. E., Tang, W., Benchimol, E. I., et al. (2017). Worldwide incidence and prevalence of inflammatory bowel disease in the 21st century: A systematic review of population-based studies. Lancet (London, Engl. 390 (10114), 2769–2778. doi:10.1016/S0140-6736(17)32448-0
Nielsen, O. H., Li, Y., Johansson-Lindbom, B., and Coskun, M. (2017). Sphingosine-1-Phosphate signaling in inflammatory bowel disease. Trends Mol. Med. 23 (4), 362–374. doi:10.1016/j.molmed.2017.02.002
Odenwald, M. A., and Turner, J. R. (2017). The intestinal epithelial barrier: A therapeutic target? Nat. Rev. Gastroenterology Hepatology 14 (1), 9–21. doi:10.1038/nrgastro.2016.169
Öhman, L., Törnblom, H., and Simrén, M. (2015). Crosstalk at the mucosal border: Importance of the gut microenvironment in IBS. Nat. Rev. Gastroenterology Hepatology 12 (1), 36–49. doi:10.1038/nrgastro.2014.200
Oz-Arslan, D., Rüscher, W., Myrtek, D., Ziemer, M., Jin, Y., Damaj, B. B., et al. (2006). IL-6 and IL-8 release is mediated via multiple signaling pathways after stimulating dendritic cells with lysophospholipids. J. Leukoc. Biol. 80 (2), 287–297. doi:10.1189/jlb.1205751
Park, S.-J., and Im, D.-S. (2019). Blockage of sphingosine-1-phosphate receptor 2 attenuates allergic asthma in mice. Br. J. Pharmacol. 176 (7), 938–949. doi:10.1111/bph.14597
Patankar, J. V., and Becker, C. (2020). Cell death in the gut epithelium and implications for chronic inflammation. Nat. Rev. Gastroenterology Hepatology 17 (9), 543–556. doi:10.1038/s41575-020-0326-4
Perez, D. A., Galvão, I., Athayde, R. M., Rezende, B. M., Vago, J. P., Silva, J. D., et al. (2019). Inhibition of the sphingosine-1-phosphate pathway promotes the resolution of neutrophilic inflammation. Eur. J. Immunol. 49 (7), 1038–1051. doi:10.1002/eji.201848049
Pérez-Jeldres, T., Tyler, C. J., Boyer, J. D., Karuppuchamy, T., Bamias, G., Dulai, P. S., et al. (2019). Cell trafficking interference in inflammatory bowel disease: Therapeutic interventions based on basic pathogenesis concepts. Inflamm. Bowel Dis. 25 (2), 270–282. doi:10.1093/ibd/izy269
Pulkoski-Gross, M. J., Uys, J. D., Orr-Gandy, K. A., Coant, N., Bialkowska, A. B., Szulc, Z. M., et al. (2017). Novel sphingosine kinase-1 inhibitor, LCL351, reduces immune responses in murine DSS-induced colitis. Prostagl. Other Lipid Mediat. 130, 47–56. doi:10.1016/j.prostaglandins.2017.03.006
Pyne, S., Lee, S. C., Long, J., and Pyne, N. J. (2009). Role of sphingosine kinases and lipid phosphate phosphatases in regulating spatial sphingosine 1-phosphate signalling in health and disease. Cell. Signal. 21 (1), 14–21. doi:10.1016/j.cellsig.2008.08.008
Sandborn, W. J., Feagan, B. G., D'Haens, G., Wolf, D. C., Jovanovic, I., Hanauer, S. B., et al. (2021). Ozanimod as induction and maintenance therapy for ulcerative colitis. N. Engl. J. Med. 385 (14), 1280–1291. doi:10.1056/NEJMoa2033617
Sandborn, W. J., Peyrin-Biroulet, L., Zhang, J., Chiorean, M., Vermeire, S., Lee, S. D., et al. (2020). Efficacy and safety of etrasimod in a phase 2 randomized trial of patients with ulcerative colitis. Gastroenterology 158 (3), 550–561. doi:10.1053/j.gastro.2019.10.035
Sandborn, W. J., Vermeire, S., Peyrin-Biroulet, L., Dubinsky, M. C., Panes, J., Yarur, A., et al. (2023). Etrasimod as induction and maintenance therapy for ulcerative colitis (ELEVATE): Two randomised, double-blind, placebo-controlled, phase 3 studies. Lancet (London, Engl. 401 (10383), 1159–1171. doi:10.1016/S0140-6736(23)00061-2
Sartor, R. B. (2004). Therapeutic manipulation of the enteric microflora in inflammatory bowel diseases: Antibiotics, probiotics, and prebiotics. Gastroenterology 126 (6), 1620–1633. doi:10.1053/j.gastro.2004.03.024
Sears, C. L. (2009). Enterotoxigenic Bacteroides fragilis: A rogue among symbiotes. Clin. Microbiol. Rev. 22 (2), 349–369. doi:10.1128/CMR.00053-08
Seo, Y.-J., Pritzl, C. J., Vijayan, M., Blake, C. R., McClain, M. E., and Hahm, B. (2012). Sphingosine analogue AAL-R increases TLR7-mediated dendritic cell responses via p38 and type I IFN signaling pathways. J. Immunol. Baltim. Md, 1950) 188 (10), 4759–4768. doi:10.4049/jimmunol.1102754
Shafik, N. M., Gaber, R. A., Mohamed, D. A., and Ebeid, A. M. (2019). Hesperidin modulates dextran sulfate sodium-induced ulcerative colitis in rats: Targeting sphingosine kinase-1- sphingosine 1 phosphate signaling pathway, mitochondrial biogenesis, inflammation, and apoptosis. J. Biochem. Mol. Toxicol. 33 (6), e22312. doi:10.1002/jbt.22312
Skoura, A., Michaud, J., Im, D.-S., Thangada, S., Xiong, Y., Smith, J. D., et al. (2011). Sphingosine-1-phosphate receptor-2 function in myeloid cells regulates vascular inflammation and atherosclerosis. Arteriosclerosis, Thrombosis, Vasc. Biol. 31 (1), 81–85. doi:10.1161/ATVBAHA.110.213496
Sleutjes, J. A. M., de Vries, A. C., and van der Woude, C. J. (2020). Ozanimod in crohn's disease: A promising new player. Lancet. Gastroenterology Hepatology 5 (9), 791–792. doi:10.1016/S2468-1253(20)30189-8
Smirnov, A., Pohlmann, S., Nehring, M., Ali, S., Mann-Nüttel, R., Scheu, S., et al. (2017). Sphingosine 1-phosphate- and C-C chemokine receptor 2-dependent activation of CD4+ plasmacytoid dendritic cells in the bone marrow contributes to signs of sepsis-induced immunosuppression. Front. Immunol. 8, 1622. doi:10.3389/fimmu.2017.01622
Snider, A. J., Kawamori, T., Bradshaw, S. G., Orr, K. A., Gilkeson, G. S., Hannun, Y. A., et al. (2009). A role for sphingosine kinase 1 in dextran sulfate sodium-induced colitis. FASEB J. 23 (1), 143–152. doi:10.1096/fj.08-118109
Snippert, H. J., van der Flier, L. G., Sato, T., van Es, J. H., van den Born, M., Kroon-Veenboer, C., et al. (2010). Intestinal crypt homeostasis results from neutral competition between symmetrically dividing Lgr5 stem cells. Cell 143 (1), 134–144. doi:10.1016/j.cell.2010.09.016
Spiegel, S., Maczis, M. A., Maceyka, M., and Milstien, S. (2019). New insights into functions of the sphingosine-1-phosphate transporter SPNS2. J. Lipid Res. 60 (3), 484–489. doi:10.1194/jlr.S091959
Spiegel, S., and Milstien, S. (2003). Sphingosine-1-phosphate: An enigmatic signalling lipid. Nat. Rev. Mol. Cell Biol. 4 (5), 397–407. doi:10.1038/nrm1103
Suh, J. H., and Saba, J. D. (2015). Sphingosine-1-phosphate in inflammatory bowel disease and colitis-associated colon cancer: The fat's in the fire. Transl. Cancer Res. 4 (5), 469–483. doi:10.3978/j.issn.2218-676X.2015.10.06
Sun, M., Du, B., Shi, Y., Lu, Y., Zhou, Y., and Liu, B. (2019a). Combined signature of the fecal microbiome and plasma metabolome in patients with ulcerative colitis. Med. Sci. Monit. Int. Med. J. Exp. Clin. Res. 25, 3303–3315. doi:10.12659/MSM.916009
Sun, M., Zhou, Y., Shi, Y., and Liu, B. (2019b). Effect of the sphingosine kinase 1 selective inhibitor, PF543 on dextran sodium sulfate-induced colitis in mice. DNA Cell Biol. 38 (11), 1338–1345. doi:10.1089/dna.2019.4737
Tang, X., and Brindley, D. N. (2020). Lipid phosphate phosphatases and cancer. Biomolecules 10 (9), 1263. doi:10.3390/biom10091263
Thamilselvan, V., Li, W., Sumpio, B. E., and Basson, M. D. (2002). Sphingosine-1-phosphate stimulates human Caco-2 intestinal epithelial proliferation via p38 activation and activates ERK by an independent mechanism. Vitro Cell. Dev. Biol. Animal 38 (4), 246–253. doi:10.1290/1071-2690(2002)038<0246:SPSHCI>2.0.CO;2
Tran, H. B., Jersmann, H., Truong, T. T., Hamon, R., Roscioli, E., Ween, M., et al. (2017). Disrupted epithelial/macrophage crosstalk via Spinster homologue 2-mediated S1P signaling may drive defective macrophage phagocytic function in COPD. PloS One 12 (11), e0179577. doi:10.1371/journal.pone.0179577
Ueno, H., Schmitt, N., Palucka, A. K., and Banchereau, J. (2010). Dendritic cells and humoral immunity in humans. Immunol. Cell Biol. 88 (4), 376–380. doi:10.1038/icb.2010.28
Vermeire, S., Chiorean, M., Panés, J., Peyrin-Biroulet, L., Zhang, J., Sands, B. E., et al. (2021). Long-term safety and efficacy of etrasimod for ulcerative colitis: Results from the open-label extension of the OASIS study. J. Crohn's Colitis 15 (6), 950–959. doi:10.1093/ecco-jcc/jjab016
Verstockt, B., Vetrano, S., Salas, A., Nayeri, S., Duijvestein, M., Vande Casteele, N., et al. (2022). Sphingosine 1-phosphate modulation and immune cell trafficking in inflammatory bowel disease. Nat. Rev. Gastroenterology Hepatology 19 (6), 351–366. doi:10.1038/s41575-021-00574-7
Wang, J., Goren, I., Yang, B., Lin, S., Li, J., Elias, M., et al. (2022a). Review article: The sphingosine 1 phosphate/sphingosine 1 phosphate receptor axis - a unique therapeutic target in inflammatory bowel disease. Alimentary Pharmacol. Ther. 55 (3), 277–291. doi:10.1111/apt.16741
Wang, X., Chen, S., Xiang, H., Wang, X., Xiao, J., Zhao, S., et al. (2022b). S1PR2/RhoA/ROCK1 pathway promotes inflammatory bowel disease by inducing intestinal vascular endothelial barrier damage and M1 macrophage polarization. Biochem. Pharmacol. 201, 115077. doi:10.1016/j.bcp.2022.115077
Weichand, B., Weis, N., Weigert, A., Grossmann, N., Levkau, B., and Brüne, B. (2013). Apoptotic cells enhance sphingosine-1-phosphate receptor 1 dependent macrophage migration. Eur. J. Immunol. 43 (12), 3306–3313. doi:10.1002/eji.201343441
Xavier, R. J., and Podolsky, D. K. (2007). Unravelling the pathogenesis of inflammatory bowel disease. Nature 448 (7152), 427–434. doi:10.1038/nature06005
Xie, J., Liu, Y., Chen, B., Zhang, G., Ou, S., Luo, J., et al. (2019). Ganoderma lucidum polysaccharide improves rat DSS-induced colitis by altering cecal microbiota and gene expression of colonic epithelial cells. Food and Nutr. Res. 63. doi:10.29219/fnr.v63.1559
Xiong, M., Li, L., Liu, Y., Zhou, F., Shi, N., Huang, H., et al. (2019). The sphingosine 1-phosphate receptor agonist FTY720 interfered the distribution of dendritic cell and induced the maternal-fetal immune tolerance. J. Cell. Biochem. 120 (2), 1869–1877. doi:10.1002/jcb.27501
Xu, Y., Krause, A., Limberis, M., Worgall, T. S., and Worgall, S. (2013). Low sphingosine-1-phosphate impairs lung dendritic cells in cystic fibrosis. Am. J. Respir. Cell Mol. Biol. 48 (2), 250–257. doi:10.1165/rcmb.2012-0021OC
Yang, J., Yang, L., Tian, L., Ji, X., Yang, L., and Li, L. (2018). Sphingosine 1-phosphate (S1P)/S1P receptor2/3 Axis promotes inflammatory M1 polarization of bone marrow-derived monocyte/macrophage via G(α)i/o/PI3K/JNK pathway. Cell. Physiology Biochem. 49 (5), 1677–1693. doi:10.1159/000493611
Yao, J., Liu, T., Chen, R.-J., Liang, J., Li, J., and Wang, C.-G. (2020). Sphingosine-1-phosphate signal transducer and activator of transcription 3 signaling pathway contributes to baicalein-mediated inhibition of dextran sulfate sodium-induced experimental colitis in mice. Chin. Med. J. 133 (3), 292–300. doi:10.1097/CM9.0000000000000627
You, Y., Zhou, C., Li, D., Cao, Z.-L., Shen, W., Li, W.-Z., et al. (2016). Sorting nexin 10 acting as a novel regulator of macrophage polarization mediates inflammatory response in experimental mouse colitis. Sci. Rep. 6, 20630. doi:10.1038/srep20630
Yousefi-Ahmadipour, A., Rashidian, A., Mirzaei, M. R., Farsinejad, A., PourMohammadi-Nejad, F., Ghazi-Khansari, M., et al. (2019). Combination therapy of mesenchymal stromal cells and sulfasalazine attenuates trinitrobenzene sulfonic acid induced colitis in the rat: The S1P pathway. J. Cell. Physiology 234 (7), 11078–11091. doi:10.1002/jcp.27944
Zaidi, D., Huynh, H. Q., Carroll, M. W., Mandal, R., Wishart, D. S., and Wine, E. (2020). Gut microenvironment and bacterial invasion in paediatric inflammatory bowel diseases. J. Pediatr. Gastroenterology Nutr. 71 (5), 624–632. doi:10.1097/MPG.0000000000002848
Zhao, X., Yang, L., Chang, N., Hou, L., Zhou, X., Dong, C., et al. (2021). Neutrophil recruitment mediated by sphingosine 1-phosphate (S1P)/S1P receptors during chronic liver injury. Cell. Immunol. 359, 104243. doi:10.1016/j.cellimm.2020.104243
Keywords: sphingosine-1-phosphate, S1P-S1PR signaling pathway, intestinal epithelial barrier, immune dysfunction, inflammatory bowel disease
Citation: Zou F, Wang S, Xu M, Wu Z and Deng F (2023) The role of sphingosine-1-phosphate in the gut mucosal microenvironment and inflammatory bowel diseases. Front. Physiol. 14:1235656. doi: 10.3389/fphys.2023.1235656
Received: 06 June 2023; Accepted: 17 July 2023;
Published: 25 July 2023.
Edited by:
Priyankar Dey, Thapar Institute of Engineering and Technology, IndiaCopyright © 2023 Zou, Wang, Xu, Wu and Deng. This is an open-access article distributed under the terms of the Creative Commons Attribution License (CC BY). The use, distribution or reproduction in other forums is permitted, provided the original author(s) and the copyright owner(s) are credited and that the original publication in this journal is cited, in accordance with accepted academic practice. No use, distribution or reproduction is permitted which does not comply with these terms.
*Correspondence: Feihong Deng, dfh411@csu.edu.cn