- 1Education and Training Integration Center, Nanjing Sport Institute, Nanjing, China
- 2School of Sports Medicine and Health, Chengdu Sport University, Chengdu, China
- 3Department of Physical Education, Gdańsk University of Physical Education and Sport, Gdańsk, Poland
From the perspective of energy expenditure, this study investigated the effects of whole-body vibration training on the energy metabolism of deep squats with different weights. Twenty-two healthy male college students with sports experiences were selected and randomly assigned to perform resistance exercise vibration (REV) or resistance exercise (RE) with varying loads two times per week for 4 weeks. Oxygen uptake and heart rate were measured before, during, and after exercises using a gas analyzer, and energy expenditure was calculated. The results showed the following: 1) the oxygen uptake and energy expenditure of the REV group were significantly higher than those of the RE group during and 30 min after exercise (p < 0.01), respectively, and the excess post-exercise oxygen consumption (EPOC) was also significantly higher than that of the RE group (p < 0.01). 2) Changes in the oxygen uptake and energy expenditure were stable with increasing exercise in both vibration and non-vibration conditions. There was no difference in energy expenditure per unit of body mass versus additional energy per kilogram of body weight (p > 0.05). 3) No significant differences in changing exercise intensity were observed in the REV group compared to those in the RE group during the adjacent incremental load phases of △ (40%–0%) and △ (80%–40%) of load during and 30 min after exercise (p > 0.05). Our results suggest that 1) vibration training can increase energy expenditure during low-intensity training and excess post-exercise oxygen consumption, and improve the exercise intensity. 2) The effects on energy expenditure were the same for both weight-bearing and non-weight-bearing deep squats, up to 40% of body mass.
1 Introduction
At the present stage, whole-body vibration training (Van Heuvelen et al., 2021) has been widely used in the field of sports training and rehabilitation, which is an efficient, fast, non-invasive, and easy-to-use training tool that not only effectively increases muscle strength (Cormie et al., 2006; Bogaerts et al., 2007; Minhaj et al., 2022) and power (Pamukoff et al., 2016) and improves body composition (da Cunha de Sá-Caputo et al., 2021; Rubio-Arias et al., 2021; Reis-Silva et al., 2022) but also rapidly increases energy expenditure (Alizadeh et al., 2020; Jiménez-Pérez et al., 2020). Da et al. (2007) showed that vibration training increased oxygen uptake by 17% compared to non-vibration training. The squat, as an essential compound exercise in strength training, is crucial to the physiological function and athletic performance of athletes (Salmanpour, 2022), which can increase muscle strength (Taheri, 2018), endurance, and explosive power, and also effectively improve the body’s reaction time (Taheri, 2017) and adaptive ability and prevent sports injuries (Al-Azzawi et al., 2023). Therefore, by understanding the effects of vibration training on energy metabolism in squatting (Hibino et al., 2023), we can integrate vibration training into athletes’ strength training programs and the general public’s sports and fitness (Dos Santos et al., 2023), and maximize the optimization of athletes’ training effects and the development of more personalized training programs by enhancing energy expenditure. Meanwhile, Serravite et al. also found, in their study, that oxygen uptake (VO2) consumption was significantly greater during high-frequency 20% vibration training than during non-vibration training (Serravite et al., 2008).
The metabolic rate of the body can be increased by vibration training (Cristi-Montero et al., 2013). The study by Dionisio VC and others found that significant changes in body composition occurred with relatively low-intensity vibration training three times a week (Dionisio et al., 2021). It accelerates the effect of fat loss. It also provides a new avenue for sports enthusiasts with fat loss needs. Fat reduction in public sports mainly relies on energy consumption during exercise. The more activity and the longer the exercise time, the more energy the body consumes (Jiménez-Pérez et al., 2020). In a study by DaSilva et al., it was concluded that the vibration group had a higher energy expenditure and RPE than the non-vibration group during the exercise and recovery periods (Da et al., 2007). When the intensity of exercise is higher, the body consumes more and needs more time to recover, and the duration of excess post-exercise oxygen consumption (EPOC) is also longer, indicating that during the recovery process, the body needs to consume more oxygen to return to the resting state (Tsolakis et al., 2020). This is one of the main reasons that EPOC is significantly higher after high-intensity resistive training than lower-intensity resistive training.
Studies suggest that the effect of exercise intensity on EPOC is more important than the time spent exercising (Dionisio et al., 2021). It has been found that exercise intensity can affect the amount and duration of EPOC. However, exercise duration can only affect the duration of EPOC. In addition to exercise intensity and exercise duration, EPOC is also affected by some physiological factors (Cochrane et al., 2008). It has also been shown that there is a linear relationship between oxygen uptake and amplitude, which makes it clear that regardless of population, type of exercise, or whether it is weight-bearing or not, as long as the amplitude changes, oxygen uptake will change and metabolic capacity may not increase, but greater energy expenditure can be realized (Rittweger et al., 2001). There is also the removal of lactic acid from muscle tissue during exercise, the hemoglobin load in blood and the myoglobin load in muscle tissue, oxygen load, oxygen load of myoglobin in muscle tissue, gas exchange, body temperature, heart rate, and hormone maintenance at high levels (Gomes-Neto et al., 2021).
The purpose of this experimental study was to investigate the difference in energy expenditure between the resistance exercise vibration (REV) and resistance exercise (RE) groups under different weighted squatting conditions (Dionisio et al., 2021). Does vibration training alter energy expenditure during deep squats? Does vibration training have different effects on energy metabolism under different weight-bearing conditions? Experiments by Bertucci W M and others demonstrated that in terms of energy expenditure and metabolic equivalent values, energy expenditure was significantly greater with REV than with RE, but energy expenditure was greater for low amplitude than for high amplitude and no vibration (Bertucci et al., 2015).
There are two main intervention factors in this experiment: one is the mechanical factor. According to Rittweger J et al., 30 Hz is a relatively suitable frequency stimulus for the human body during vibration training (Rittweger et al., 2001). Bertucci believes that the experimental results in the case of frequency 30 Hz are average and that 50 Hz should be used for best results (Bertucci et al., 2015). The conventional parameter selection is about 26–45 Hz and 2∼6 mm; and too low and too high vibration stimulation have some adverse effects on the human body, so our research parameters are constant in the mechanical vibration amplitude of 2 mm and vibration frequency of 30 Hz. The other factor is the external resistance load change factor. Three loads were selected in the study by Serravite et al., which were 0, 20, and 40% of body weight (Serravite et al., 2008); meanwhile, in our study, the loads were finally set at 0, 20, 40, and 80% in order to try to understand more about the effect of loads on energy consumption.
In this study, our results were statistically analyzed to demonstrate that vibration stimulation can increase additional energy consumption and deepen EPOC; with the increase in load, the intensity of energy consumption increased by vibration training is more than that increased by non-vibration training (Avelar et al., 2011). On the other hand, vibration training can increase the energy metabolic rate and ability to oxidize sugar during exercise, thus achieving the effect of accelerating fat loss (Rittweger et al., 2001). Therefore, for the athletic training and mass fitness fat loss crowd, this can also serve as an efficient, more scientific, and effective training guide.
2 Materials and methods
2.1 Experimental approach to study
According to previous studies (Garatachea et al., 2007), when the frequency is below 20 Hz, the resonance phenomenon with each organ can be easily triggered, which will cause harm to the human body. However, the conventional frequency choice for vibration training is 30 Hz, which proved to be a relatively appropriate body frequency stimulus (Rittweger et al., 2001), and the choice of the 2-mm amplitude exercise condition was a more appropriate intervention stimulus, with the lower amplitude producing more EE (energy expenditure) and RPE (respiratory exchange rate) compared to the higher amplitude of 4 mm (Serravite et al., 2008). So, in our experiment, the vibration frequency is set at 30 Hz, the amplitude is set at 2 mm, and the frequency of each vibration training is about 3 days (Rehn et al., 2007). We randomly sorted each person before the experiment into two groups, vibration and no vibration, respectively, according to body mass 0, 20, 40, and 80% of the load. A total of eight experiments were conducted, comprising a total experimental period of about 4 weeks, while the data before and after the experiment were used to compare and analyze.
2.2 Subjects
To calculate the sample size for the experiment, we used G*Power software. We set one tail, effect size to 0.5, α to 0.05, and power to 0.8. We calculated that we needed to recruit at least 21 subjects. Therefore, we selected 22 healthy male college students as subjects (Table 1), and the Research Ethics Committee of Nanjing Sport Institute approved this study protocol (registration number RT-2023-08). Participants in this study must meet the following criteria: 1) have at least 3 years of exercise experience; 2) have no history of cardiovascular disease, injury, or discomfort during vibration stimulation training; and 3) be able to perform at least 10 repetitions of squats at body weight. We asked them to refrain from other strenuous exercises for 2 days before each experiment to avoid interference with the results of the experiment, and they had to sign a consent form.
2.3 Training program and prescription
In order to prevent stress reactions during the formal experiment, 1 week before the start of the experiment, each subject was subjected to shaking table and oxygen mask use, and pre-experimental simulations were conducted to further familiarize them with the whole process of the experiment. To reduce the effect of abnormal food thermogenesis and resting metabolism on the experimental results, 6–8 h of adequate sleep was ensured, eating should be completed 2 h before the start of the experiment, and the time of each experiment was consistent with the pre-experimental diet.
We used the Power Plate vibration trainer. Before each experiment, the participant entered the laboratory, sat and rested, wore an oxygen mask, and was monitored using a cortex gas metabolism analyzer. When stabilized to basal metabolism, it was recorded in the resting condition 10 min before exercise and in exercise conditions. Six groups of deep squats were performed, 10 per group, according to the rhythm of the metronome, 3 s every time, with each group interval of 60 s (Van Heuvelen et al., 2021). Immediately after exercise, the basal metabolic values were collected during the complete return to the resting condition as a complete experimental procedure (Figure 1).
The formula (Goudarzian et al., 2021) is then used to calculate the total energy expenditure during and after the exercise.
CHO = (4.585*CO2) − (3.2255*CO2)2
FAT = (1.6946*CO2) − (1.7012*CO2)2
EE = (CHO*4) + (FAT*9)
To obtain the actual energy expended during exercise, the energy produced by resting metabolism during exercise (EE-REE) was subtracted from the total energy expenditure in each phase (Alizadeh et al., 2020).
REE = 88.362+(13.397*body weight)+(4.799*height)−(5.677*age)
2.4 Statistical analysis
SPSS 18.0 was used for statistical analysis, and results were expressed as mean ± standard deviation (X ± SD). To test whether energy expenditure, oxygen uptake, and other indices were statistically different under different loads in the vibration and non-vibration conditions, two-factor repeated measures ANOVA was used. Whenever two factors interacted, separate effects were identified, and when they did not, the main effects were identified and effect sizes were reported to highlight the separate effects. Each group’s data were tested by Shapiro–Wilk’s method and the p-value was greater than 0.05, indicating that they followed a normal distribution. The p-value of Levene’s chi-square test was greater than 0.05, and the data were consistent with equal variance. Then, according to the sphericity test, if the p-value is greater than 0.05, it means that there is no correlation between the data on repeated measurements. If the p-value was less than 0.05, the correction was performed with Greenhouse–Geisser correction. The statistically significant level was p < 0.05. The highly significant level was p < 0.01. Then, we defined the “∆” notation to indicate the change in relative oxygen uptake between two different loading levels. For example, ∆ (40%–0%) denotes the change in relative oxygen uptake between 0% and 40% loads, while ∆ (80%–40%) denotes the change in relative oxygen uptake between 40% and 80% loads.
3 Research results
3.1 Changes in oxygen uptake during different phases of vibration training
Statistical analysis of the changes in oxygen uptake in different phases of the exercise process and the reliability of its data was conducted, according to confidence intervals (Roelants et al., 2004). Total oxygen uptake in 10 min during the rest phase before exercise, “vibration stimulation” (F = 2.496, p = 0.149), “load” (F = 0.018, p = 0.997), and no difference obtained from a statistical test due to “vibration stimulation” * “load” p = 0.143 is shown in Figure 2.
Total oxygen uptake during exercise in 8 min, “vibration stimulation” (F = 31.972, p = 0.000), “load” (F = 170.784, p = 0.000), and by “vibration stimulation"*"load” (F = 3.896, p = 0.020) had an interaction effect according to a statistical test (p < 0. 05). The oxygen uptake of the body gradually increased with the increase in load in both REV and RE groups, respectively, and there was a significant difference (p < 0.01) between the groups (Table 2).
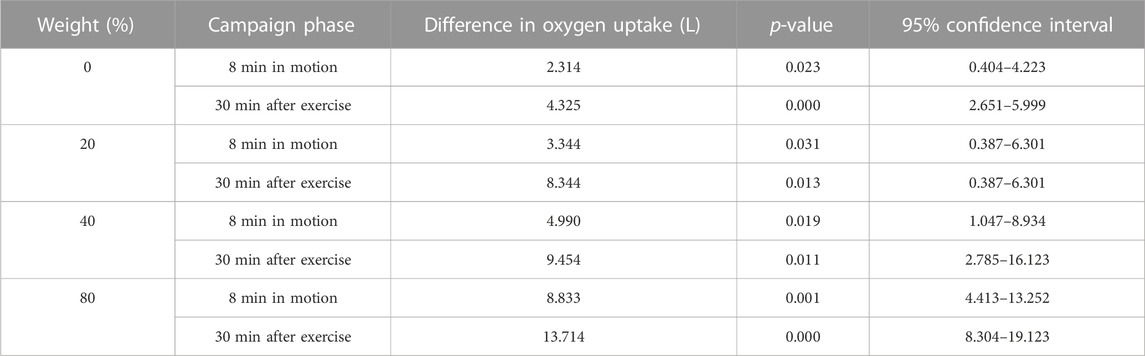
TABLE 2. Analysis of the difference in oxygen uptake between the two phases with significant differences.
Total oxygen uptake within 30 min after exercise, “vibration stimulation” (F = 49.503, p = 0.000), “load” (F = 25.805, p = 0.000), and due to “vibration stimulation“*”load” (F = 6.203, p = 0.002) had an interaction effect, according to a statistical test (p < 0.05). Under the premise of controlling vibration stimulation, the oxygen uptake of the organism gradually increased with increasing load in both REV and RE groups, and there was a significant difference between them (p < 0.01). This indicates that vibration stimulation has an effect on oxygen uptake, and the oxygen uptake at 80% load was significantly greater than that at 40% and 20%.
Total oxygen uptake 30–60 min after exercise, ‘vibration stimulation’ (F = 15.810, p = 0.003), ‘load’ (F = 8.284, p = 0.000), and by ‘vibration stimulation‘*’load’ (F = 0.390, p = 0.761) had no interaction effect, according to a statistical test (p > 0.05). After 30 min since the end of the exercise, there was no significant difference in the oxygen uptake at 0%, 20%, 40%, and 80%, indicating a basic return to a resting state and the end of EPOC.
3.2 Changes in total energy consumption under different phases of vibration training
Statistical analysis of total energy expenditure in different phases of the exercise process was performed (shown in Figure 3). The total energy expenditure in the resting phase for 10 min before exercise, “vibration stimulation” (F = 1.126, p = 0.316), “load” (F = 0.157, p = 0.924), and by "vibration stimulation” * “load” (F = 1.393, p = 0.266) was not different according to a statistical test. This indicates that during the resting phase, all groups were at the same basal metabolic level as each other, and therefore, there was no significant difference.
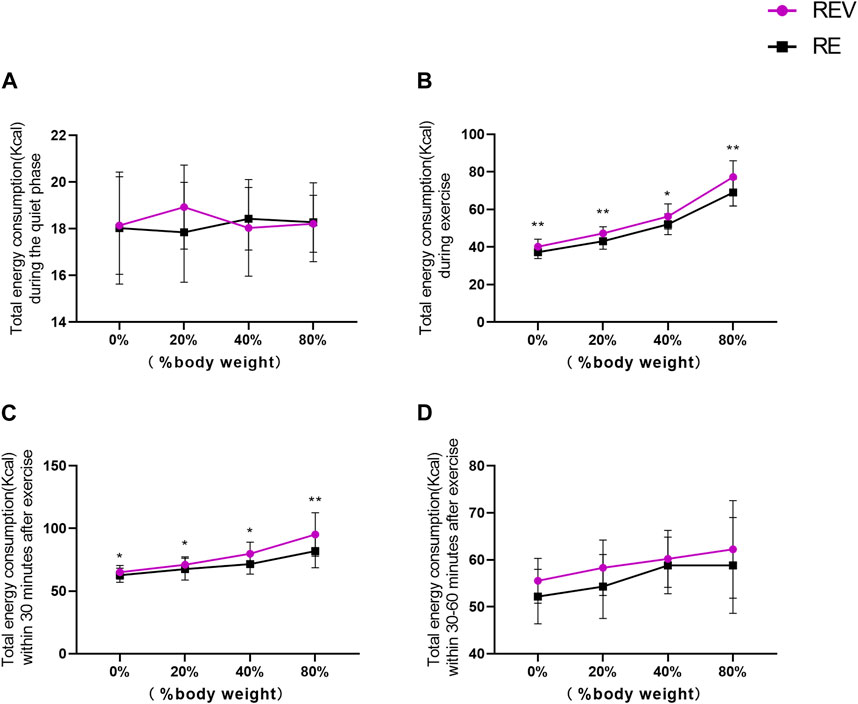
FIGURE 3. Changes in total energy consumption during different stages of exercise (*p < 0.05; **p < 0.01).
Total energy expenditure during exercise for 8 min, “vibration stimulation” (F = 55.538, p = 0.000), “load” (F = 171.713, p = 0.000), and due to “vibration stimulation“*”load” (F = 3.012, p = 0.047) had an interaction effect according to a statistical test (p < 0.05). The energy expenditure of the body gradually increased with the increase in load in both REV and RE groups, respectively, and there was a significant difference between them (p < 0.01) (Table 3).
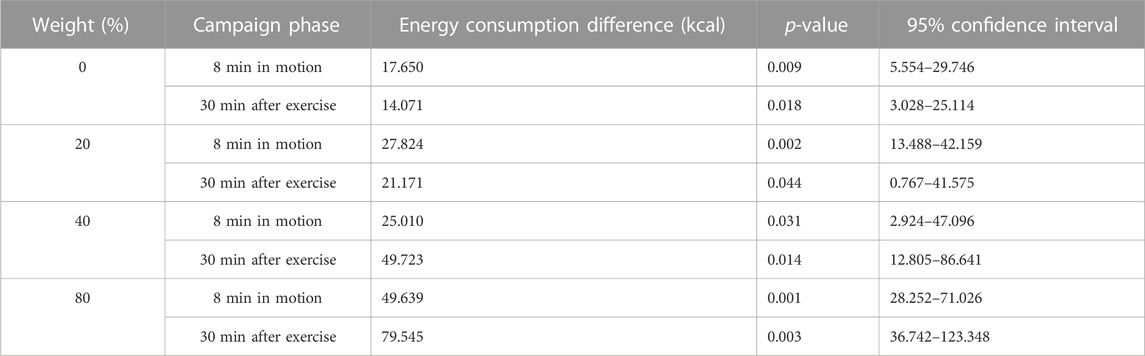
TABLE 3. Analysis of the difference in energy consumption between the two phases with significant differences.
Total energy expenditure within 30 min after exercise, “vibration stimulation” (F = 31.508, p = 0.000), “load” (F = 23.754, p = 0.000), and due to “vibration stimulation“*”load” (F = 5.04, p = 0.007) had an interaction effect according to a statistical test (p < 0.05). The energy expenditure of the body gradually increased with the increase in load in both REV and RE groups, respectively, and there was a significant difference between them (p < 0.01). It indicates that the effect of high-intensity vibration exercise is significantly greater than that of low- and medium-intensity exercise.
Total energy expenditure 30–60 min after exercise, “vibration stimulation” (F = 15.837, p = 0.003), “load” (F = 5.986, p = 0.003), and by “vibration stimulation” * “load” (F = 0.986, p = 0.422) had no interaction effect, according to a statistical test (p > 0.05). It means that vibration training with different weights after 30 min of exercise did not meet statistical differences in energy expenditure.
3.3 Differences in oxygen uptake and energy expenditure between the REV and RE groups during incremental loading
To investigate the change in relative oxygen uptake between adjacent incremental loads during exercise (shown in Figure 4), a paired-samples t-test was performed in both the △ (40%–0%) and △ (80%–40%) incremental load phases, and the statistical results showed no significant difference between the two groups (p > 0.05). When comparing within the groups, the REV (p = 0.263) and RE groups (p = 0.219) showed no significant effect of the “weight” factor on the relative oxygen uptake (p > 0.05). When comparing between the △ (40%–0%) (p = 0.135) and △ (80%–40%) groups (p = 0.128), there was no significant difference in relative oxygen uptake by “vibration stimulation” (p > 0.05). With the increase in load and the application of vibration stimulation, the intensity of the change in oxygen uptake was not significant.
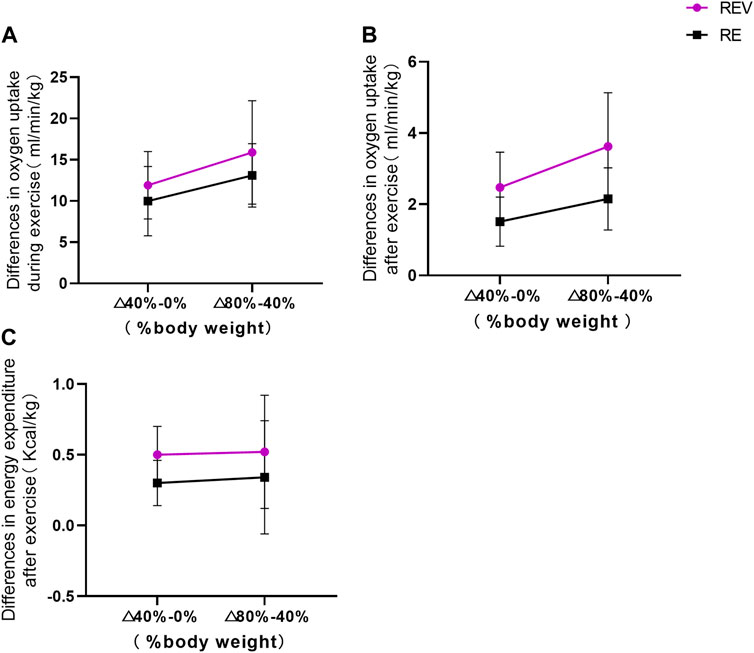
FIGURE 4. Changes in oxygen uptake and energy consumption during progressive loading (*p < 0.05; **p < 0.01).
To investigate the change in relative oxygen uptake between adjacent incremental loads after exercise, a paired-samples t-test was performed in both the △ (40%–0%) and △ (80%–40%) incremental load phases, and the statistical results showed no significant difference between the two groups (p > 0.05). When comparing within the groups, the REV (p = 0.303) and RE groups (p = 0.559) showed no significant effect of the “weight” factor on the relative oxygen uptake (p > 0.05). Comparison between the △(40%–0%) (p = 0.134) and △(80%–40%) groups (p = 0.157) showed no significant difference between “vibration stimulation” and relative oxygen uptake (p > 0.05).
To investigate the change in relative energy expenditure between adjacent incremental loads after exercise, a paired-samples t-test was performed in the incremental load △ (40%–0%) and △ (80%–40%) phases, and the statistical results showed that there was no significant difference between the two groups (p > 0.05). When comparing within the groups, the REV (p = 0.846) and RE groups (p = 0.772) showed that the “weight” factor did not have a significant effect on relative energy expenditure (p > 0.05). When comparing between the △ (40%–0%) (p = 0.055) and △ (80%–40%) groups (p = 0.207), it showed that there is no significant difference between “vibration stimulation” and relative energy expenditure (p > 0.05).
4 Discussion
4.1 The effect of weighted vibration training on the body’s oxygen uptake
The purpose of this study was to investigate the effect of whole-body vibration training on energy metabolism during deep squats of different intensities from the perspective of energy expenditure. Our results showed that vibration training can increase energy expenditure and post-exercise excess oxygen consumption during low-intensity training and improve exercise intensity.
Related experimental results show that the average VO of individuals performing resistance half-squat training with vibration stimulation intervention increases by 4.5 mL/kg compared to non-vibration training, which corresponds to 20 Kcal of energy from oxygen consumption and is approximately equal to the exercise intensity at a walking speed of 0.4 m/s, while increasing with vibration frequency, amplitude, and weight (Dallas et al., 2015). In addition, studies have shown that VO2 of the REV group was significantly higher than that of the RE group (Serravite et al., 2008), which were 16.6% and 18.9%; it indicates that vibration has an effect on oxygen uptake by stimulation, and the effect of higher-intensity vibration exercise is significantly better than that of low-intensity exercise. When resistance vibration training was performed under different load conditions, VO2 of the REV group was also higher than that of the RE group by about 17% (Bertucci et al., 2015). During the phase of weight-bearing exercise, VO2 of the REV group during the training period was also significantly higher than that of the RE group because the intensity of the vibration weight-bearing squatting was significantly higher than that of normal squatting; at the same time, the paper corresponds to Serravite’s experiment, on the basis of which the subjects involved were male college students with athletic experience and all of them had proficient experience in squatting practice (Serravite et al., 2008), which could minimize the error of the experiment due to the differences in movements and individual athletic ability issues, thus minimizing the experimental error. At four different loads (0%, 20%, 40%, and 80%), the increase in oxygen uptake in the REV group compared to that in the RE group was 9%, 12%, 8%, and 12%, respectively.
The results of the study by Milanese demonstrated that vibration training may be beneficial in increasing the energy metabolism rate during low weight-bearing deep squats (Milanese et al., 2018). However, the results of this experiment showed that in the △ (40%–0%) and △ (80%–40%) phases, although the mean of the REV group was higher than that of the RE group, there was no difference in oxygen consumption per unit body weight versus extra per kilogram at low and medium intensities, resulting in a non-significant difference in the trend of intensity change between REV and RE in incremental loading.
Our study also found that the recovery period becomes longer after a certain intensity of resistance exercise; during the recovery period, high-intensity exercise requires more fat oxidation for energy than low-intensity exercise (Osterberg and Melby, 2000); therefore, oxygen uptake increases. A study also found that vibration training increased the level of lactate in the blood after a deep squat exercise without increasing the exercise load (Amasay et al., 2020; Miokovic et al., 2019). Similarly, studies have also shown that vibration training significantly reduces body fat and insulin levels, which is expected to improve metabolic health, which is very beneficial for the body (Bautmans et al., 2005; Zeigler and Swan, 2016).
The results of this experiment showed that VO2 was significantly higher in the REV group than in the RE group in the 0–30 min phase at the end of the post-exercise period, but there was no significant difference in the results in this phase from 30 min to 60 min. The reason for this is that this experiment is different from most experiments because the load selection is lighter and not designed according to 1 RM proportional weight bearing, but selecting small- and medium-intensity loads proportional to body weight for training, coupled with the fact that the subjects are experienced in sports and have a higher recovery ability, so the difference is not significant. There are also differences in gravitational acceleration generated by different shakers during vibration, and the mass of the human body and the external load on the vibration platform are also different. These differences affect the intensity of training, resulting in differences in oxygen uptake and EPOC. Another point is that at the end of vibration training with increasing load, no significant difference was found in the change in oxygen uptake between the REV and RE groups, and the change in exercise intensity tended to be the same after two incremental exercise phases, △ (40%–0%) and △ (80%–40%). This may be because the subjects were a group of people who were experienced in exercise and had a high recovery capacity of the body. Therefore, different individuals have different sensitivities to the load stimulus due to the difference in the strength level, which is also an important factor affecting the experimental results.
4.2 The effect of weighted vibration training on the body’s energy expenditure
The main finding of this experiment is that the trend of energy expenditure is stable in each weight-bearing relative energy expenditure and does not cause a difference between the energy consumed per unit of body weight and the additional energy consumed per kilogram. The amount of energy consumed per kilogram per unit of body weight and external load is approximately equal, whether under vibrating or non-vibrating conditions. From this, it can be found that in addition to the energy generated by the active muscle firing contraction during work, there is also a large amount of energy consumption generated by other muscle groups in static contraction to maintain body stability (Hazell and Lemon, 2012). Because the process of standing force involves the muscles of the entire body, indicating that the muscles are continuously contracting, the tibialis anterior, rectus femoris, gluteus maximus, and hamstrings of the extensor group are very active (Rittweger et al., 2000; Patrícia et al., 2021), and the muscles around the calf are mainly contracted statically, as a form of stable support to connect the ground to the body. The energy consumed by the contraction of the stabilizing muscles is not less than that of the active muscles as the plantar flexors, femoral, iliopsoas, and longissimus muscles are highly active during the force process, indicating that these stabilizing muscles also play an important role in postural control (Lima et al., 2020).
The experimental results showed that the energy expenditure of the REV group was significantly higher than that of the RE group. The experiments concluded that the energy expenditure of continuous weighted deep squats with 40 Hz vibration training at 50% of the weighted body weight was greater for 2 mm amplitude than for no amplitude and at 4 mm amplitude (Bertucci et al., 2015). Therefore, it is not necessary to increase the intensity of the vibration to improve energy expenditure during exercise. In other studies, it was also found that the energy expenditure of the vibration group was greater than 15% compared to the non-vibration group during 30 min of continuous dynamic vibration exercise (Vissers et al., 2009). In addition, we found that the energy expenditure of the vibration group was also significantly higher than that of the non-vibration group at 30% of body weight during 4 mm, 30 Hz vibration training (Rittweger et al., 2001).
The results of the present experiment are also in line with the conclusion of the aforementioned authors’ that as the weight becomes heavier, the intensity of change in the energy consumed by the body becomes greater (Dionisio et al., 2021). Even under vibration intervention, it cannot be contrary to common sense. However, the results of the present experiment showed some differences in the loads △ (40%–0%) and △ (80%–40%) REV groups compared to the RE group in the two increasing phases of loads 40%–0% and 80%–40%, but no significant differences were found after statistical tests. So, we inferred that, on one hand, the energy expenditure due to the additional load caused by the vibration stimulation was not as high as expected. On the other hand, individuals with a high level of strength and coordinated movement completion will use less energy compared to others. In particular, at low and medium intensities, the magnitude of the change in energy expenditure with incremental load was also not significant.
4.3 Effect of post-exercise vibration on energy expenditure with different weights
Regarding the effect of energy expenditure, researchers found that the REV group had significantly higher energy expenditure than the RE group (Tsolakis et al., 2020). In this study, it was found that the total energy expenditure in the REV group was significantly greater than that in the RE group during and 0–30 min after exercise (Da et al., 2007; Osterberg and Melby, 2000). During phases of the exercise and at the end of the exercise, the energy expenditure of the REV group was significantly higher than that of the RE group. The reason for this is that the intensity of vibration training is greater than that of single resistance training, and it further increases energy expenditure (Alizadeh et al., 2020). Over time, there was no significant difference in energy expenditure in the REV group compared to that in the RE group at the end of the 30–60-min exercise phase (Jiménez-Pérez et al., 2020), suggesting that 1 hour is sufficient to restore the metabolic capacity of the function in low-to-moderate intensity training (Torvinen et al., 2002; Sitjà-Rabert et al., 2012).
From our experimental results, it is found that as the load weight increases, the energy expenditure also increases (Minhaj et al., 2022), but the trend of energy expenditure change intensity does not change much in the REV group compared with that in the RE group as the load increases. The reason for this is probably due to the arrangement of load intensity, where the load is too low, which reduces the intensity of training and is not conducive to deepening the post-exercise excess oxygen consumption, so there is not much difference between the REV and RE groups in terms of the post-exercise change in energy consumption. It shows that during training, a bigger load is not better (Lee and Kim, 2020), and the results are not always accurate when trying to increase the energy expenditure by increasing the load. It should be explored which load interval causes the individual to consume the most energy to improve the training efficiency.
4.4 Research limitations
First, the generalizability to different populations and training levels is limited by the relatively small sample size of this study. Further research could be conducted by increasing the sample size and including more subjects from different backgrounds to better understand the effects of whole-body vibration training in different populations. Second, the duration of this study was relatively short. It only covered certain training cycles. Future studies may consider designing interventions of longer duration to understand the ongoing effects of long-term training in order to more fully evaluate the effects of whole-body vibration training. Additionally, measurements of physiological metrics, such as energy expenditure and excess oxygen consumption, were limited in this study. The effects of whole-body vibration training on other physiological measures and athletic performance, such as cardiorespiratory fitness, strength performance, and musculoskeletal adaptations, could be further investigated in future studies. Finally, only one specific vibration parameter and load variation was addressed in this study. Further studies could investigate the effects of different vibration parameters and loading modes on energy metabolism and training effects. This would help optimize whole-body vibration training protocols.
4.5 Research advantages
First, the present study was conducted under controlled laboratory conditions with a rigorous experimental design and reliable data collection methods. This adds credibility to the study. Second, we examined the effects of vibration training from multiple perspectives by conducting a comprehensive analysis of the differences in energy expenditure between the REV and RE groups for different weight-bearing deep squat situations. In addition, the intervening factors in this study included both mechanical and extrinsic resistance load changes. This added to the complexity and scientific validity of the study. Finally, this study provides an efficient and easy-to-use exercise modality for the population wanting to lose fat. This provides practicality and guidance for real-world applications.
5 Conclusion
Whole-body vibration training may increase energy expenditure during low-intensity deep squat exercise and excess oxygen consumption within 30 min of exercise. However, when performing weighted squat training under vibration or non-vibration conditions within 40% of body mass, the energy consumption effect per unit of body weight is consistent with that caused by additional weight per kilogram carried. In addition, vibration training can be implemented through simple operations that improve our exercise efficiency through ease of use. The vibration stimulation of the wobbly platform also creates an adaptive stimulus that is psychologically conducive to withstanding greater training loads. However, increasing intensity requires caution and gradual progress. Therefore, in daily exercise activities, for people trying to lose fat through mass sports, if they are tired of regular running and weight training, they can try vibration training, through which the lean body mass and fat loss compared to the traditional means of exercise will be better.
Data availability statement
The original contributions presented in the study are included in the article/Supplementary Material; further inquiries can be directed to the corresponding author.
Ethics statement
The studies involving humans were approved by the Nanjing Sport Institute’s Research Ethics Committee (registration number RT-2023-08). The studies were conducted in accordance with the local legislation and institutional requirements. The participants provided their written informed consent to participate in this study. Written informed consent was obtained from the individual(s) for the publication of any potentially identifiable images or data included in this article.
Author contributions
Conceptualization: XH, ZY, and XQ. Data curation and writing–original draft: XH and ZY. Formal analysis and investigation: XH, ZY, and XQ. Writing–review and editing: XH, ZY, and XQ. All authors contributed to the article and approved the submitted version.
Funding
This work was supported by the major sports research projects of Jiangsu Provincial Sports Bureau (ST211109).
Acknowledgments
The authors would thank the volunteers for their time, enthusiasm, and feedback, and the School of Athletic Training of Nanjing Sports Institute for providing experimental materials.
Conflict of interest
The authors declare that the research was conducted in the absence of any commercial or financial relationships that could be construed as a potential conflict of interest.
Publisher’s note
All claims expressed in this article are solely those of the authors and do not necessarily represent those of their affiliated organizations, or those of the publisher, the editors, and the reviewers. Any product that may be evaluated in this article, or claim that may be made by its manufacturer, is not guaranteed or endorsed by the publisher.
References
Al-Azzawi D. M., Halouani J., Al-Gertani A. O., Chtourou H. (2023). Effect of three months specific training on physical capacities of Iraq futsal players. Int. J. Sport Stud. Health 6 (1). doi:10.5812/intjssh-135037
Alizadeh M. H., Goudarzian M., Rahimi R., Gharaat M. (2020). The acute effects of whole-body vibration on energy expenditure and respiratory exchange ratio during squatting exercise. J. Exerc. Sci. Fit. 18 (2), 47–51. doi:10.1016/j.jesf.2020.07.002
Amasay T., Aquino C. F., Gosselin L. E. (2020). Whole-body vibration and blood lactate response to squat exercise. Clin. Physiol. Funct. Imaging 40 (4), 253–257. doi:10.1111/cpf.12611
Avelar N. C., Simão A. P., Tossige-Gomes R., Neves C. D., Mezencio B., Szmuchrowski L., et al. (2011). Oxygen consumption and heart rate during repeated squatting exercises with or without whole-body vibration in the elderly. J. Strength Cond. Res. 25 (12), 3495–3500. doi:10.1519/JSC.0b013e3182176664
Bautmans I., Van Hees E., Lemper J. C., Mets T. (2005). The feasibility of whole body vibration in institutionalised elderly persons and its influence on muscle performance, balance and mobility: A randomised controlled trial [ISRCTN62535013]. BMC Geriatr. 5, 17. doi:10.1186/1471-2318-5-17
BertucciArfaouiDuc W. M. A. S., Letellier T., Brikci A., Brikci A. (2015). Effect of whole body vibration in energy expenditure and perceived exertion during intense squat exercise. Acta Bioeng. Biomechanics 17 (1), 87–93.
Bogaerts A., Verschueren S., Delecluse C., Claessens A. L., Boonen S. (2007). Effects of whole body vibration training on postural control in older individuals: A 1 year randomized controlled trial. Gait Posture 26 (2), 309–316. doi:10.1016/j.gaitpost.2006.09.078
Cochrane D. J., Stannard S. R., Sargeant A. J., Rittweger J. (2008). The rate of muscle temperature increase during acute whole-body vibration exercise. Eur. J. Appl. Physiol. 103 (4), 441–448. doi:10.1007/s00421-008-0736-4
Cormie P., Deane R. S., Triplett N. T., McBride J. M. (2006). Acute effects of whole-body vibration on muscle activity, strength, and power. J. Strength Cond. Res. 20 (3), 257–261. doi:10.1519/R-17835.1
Cristi-Montero C., Cuevas M. J., Collado P. S. (2013). Whole-body vibration training as complement to programs aimed at weight loss. Nutr. Hosp. 28 (5), 1365–1371. doi:10.3305/nh.2013.28.5.6656
Da Cunha de Sá-Caputo D., Sonza A., Coelho-Oliveira A. C., Pessanha-Freitas J., Reis A. S., Francisca-Santos A., et al. (2021). Evaluation of the relationships between simple anthropometric measures and bioelectrical impedance assessment variables with multivariate linear regression models to estimate body composition and fat distribution in adults: preliminary results. Biol. (Basel) 10 (11), 1209. doi:10.3390/biology10111209
Da S. M., Fernandez J. M., Castillo E., Nuñez V. M., Vaamonde D. M., Poblador M. S., et al. (2007). Influence of vibration training on energy expenditure in active men. J. Strength and Cond. Res. 21 (2), 470–475. doi:10.1519/R-19025.1
Dallas G., Paradisis G., Kirialanis P., Mellos V., Argitaki P., Smirniotou A. (2015). The acute effects of different training loads of whole body vibration on flexibility and explosive strength of lower limbs in divers. Biol. Sport 32 (3), 235–241. doi:10.5604/20831862.1163373
Dionisio V. C., Altimari L. R., Altimari J. M., Fernandes A. C., Orsatti F. L. (2021). Acute effects of whole-body vibration at 3 different frequencies on lower limb muscle activation and energy expenditure in healthy men. J. Strength Cond. Res. 35 (9), 2491–2497. doi:10.1519/JSC.0000000000002841
Dos Santos J. M., Taiar R., Ribeiro V. G. C., da Silva Lage V. K., Scheidt Figueiredo P. H., Costa H. S., et al. (2023). Whole-body vibration training on oxidative stress markers, irisin levels, and body composition in women with fibromyalgia: A randomized controlled trial. Bioeng. (Basel) 10 (2), 260. doi:10.3390/bioengineering10020260
Garatachea N., Jiménez A., Bresciani G., Mariño N. A., González-Gallego J., de Paz J. A. (2007). The effects of movement velocity during squatting on energy expenditure and substrate utilization in whole-body vibration. J. Strength Cond. Res. 21 (2), 594–598. doi:10.1519/R-20566.1
Gomes-Neto M., Durigan J. L. Q., Bittencourt N. F. N., de Oliveira Gonçalves I. D. S., Silva M. F. D., Amorim P. R. S., et al. (2021). Effects of whole-body vibration on energy metabolism: A systematic review. J. Musculoskelet. Neuronal Interact. 21 (2), 256–266.
Goudarzian M., Rahimi R., Alizadeh M. H., Gharaat M. (2021). Acute effect of whole-body vibration with resistance exercise on postexercise energy expenditure and respiratory exchange ratio in untrained men. J. Sport Rehabilitation 30 (3), 349–353. doi:10.1123/jsr.2020-0317
Hazell T. J., Lemon P. W. (2012). Synchronous whole-body vibration increases VO2 during and following acute exercise. Eur. J. Appl. Physiology. 112 (2), 413–420. doi:10.1007/s00421-011-1984-2
Hibino I., Takeda C., Takahashi K., Aoyama T. (2023). Effects of short-term whole-body vibration training on muscle strength, balance performance, and body composition. J. Phys. Ther. Sci. 35 (6), 414–420. doi:10.1589/jpts.35.414
Jiménez-Pérez I., Sánchez-Muñoz C., Gil-Gómez J., Pardo P. J., Ponce-González J. G. (2020). High consumption of soft drinks is associated with an increased risk of fracture: A 7-year follow-up study. Nutrients 12 (2), 530. doi:10.3390/nu12020530
Lee K., Kim H., Menting J., Sattoe J. N. T. (2020). Patterns of social participation among older adults with disabilities and the relationship with well-being: A latent class analysis. Archives Gerontology Geriatrics 86, 103933. doi:10.1016/j.archger.2019.103933
Lima C. D. S., Herzog W., Von Zuben F. J. (2020). Whole-body vibration affects squatting kinematics in young resistance-trained women. Hum. Mov. Sci. 72, 102643. doi:10.1016/j.humov.2020.102643
Milanese C., Cavedon V., Sandri M., Tam E., Piscitelli F., Boschi F., et al. (2018). Metabolic effect of bodyweight whole-body vibration in a 20-min exercise session: A crossover study using verified vibration stimulus. PLoS One 13 (1), e0192046. doi:10.1371/journal.pone.0192046
Minhaj T., Sharma S., Hayat Z. (2022). Effects of whole-body vibration on sports performance: A systematic review and meta-analysis. Sci. Sports 37 (4), 231–243. doi:10.1016/j.scispo.2021.06.015
Miokovic T., Arandelovic M., Radovanovic D. (2019). Whole-body vibration during squatting exercise increases lactate concentration without affecting performance in young male athletes. J. Strength Cond. Res. 33 (7), 1878–1883. doi:10.1519/JSC.0000000000003260
Osterberg K., Melby C. L. (2000). Effect of acute resistance exercise on postexercise oxygen consumption and resting metabolic rate in young women. Int. J. Sport Nutr. Exerc Metab. 10 (1), 71–81. doi:10.1123/ijsnem.10.1.71
Pamukoff D. N., Pietrosimone B., Lewek M. D., Ryan E. D., Weinhold P. S., Lee D. R., et al. (2016). Whole-body and local muscle vibration immediately improve quadriceps function in individuals with anterior cruciate ligament reconstruction. Arch. Phys. Med. Rehabil. 97 (7), 1121–1129. doi:10.1016/j.apmr.2016.01.021
Patrícia E. M., Lívia G. R., José C., Araújo F., Anna X. P., do Amparo Andrade M., et al. (2021). Effects of whole-body vibration on muscle strength, quadriceps muscle thickness and functional capacity in kidney transplant recipients: A randomized controlled trial. J. Bodyw. Mov. Ther. 26, 101–107. doi:10.1016/j.jbmt.2020.10.005
Rehn B., Lidström J., Skoglund J., Lindström B. (2007). Effects on leg muscular performance from whole-body vibration exercise: A systematic review. Scand. J. Med. Sci. Sports 17 (4), 2–11. doi:10.1111/j.1600-0838.2006.00578.x
Reis-Silva A., Coelho-Oliveira A. C., Martins-Anjos E., Moura-Fernandes M. C., Xavier V. L., Mendonça V. A., et al. (2022). Impact of two whole-body vibration exercise protocols on body composition of patients with metabolic syndrome: A randomized controlled trial. Int. J. Environ. Res. Public Health 20 (1), 436. doi:10.3390/ijerph20010436
Rittweger J., Beller G., Felsenberg D. (2000). Acute physiological effects of exhaustive whole-body vibration exercise in man. Clin. Physiol. 20 (2), 134–142. doi:10.1046/j.1365-2281.2000.00238.x
Rittweger J., Schiessl H., Felsenberg D. (2001). Oxygen uptake during whole-body vibration exercise: comparison with squatting as a slow voluntary movement. Eur. J. Appl. Physiol. 86 (2), 169–173. doi:10.1007/s004210100511
Roelants M., Delecluse C., Goris M., Verschueren S. (2004). Effects of 24 weeks of whole body vibration training on body composition and muscle strength in untrained females. Int. J. Sports Med. 25 (4), 1–5. doi:10.1055/s-2003-45238
Rubio-Arias J. Á., Martínez-Aranda L. M., Andreu-Caravaca L., Sanz G., Benito P. J., Ramos-Campo D. J. (2021). Effects of whole-body vibration training on body composition, cardiometabolic risk, and strength in the population who are overweight and obese: A systematic review with meta-analysis. Arch. Phys. Med. Rehabil. 102 (12), 2442–2453. doi:10.1016/j.apmr.2021.03.037
Salmanpour M., Soori R., H'mida C., Halouani J., Clarck C. T., Pournemati P., et al. (2022). The effect of 8 weeks of combined interval (resistance-interval training) and combined endurance (endurance-resistance training) on plasma levels of adropin and nitric oxide in males with high blood pressure. Ir. J. Med. Sci. 191 (6), 2559–2568. doi:10.1007/s11845-021-02873-z
Serravite D., Edwards D., Elizabeth S., Signorile J. (2008). Acute effect of frequency, amplitude and load changes on oxygen consumption during whole body vibration: 1231: board #4 may 29 8:00 AM - 10:00 AM. Med. Sci. Sports Exerc. 40 (5), S161. doi:10.1249/01.mss.0000322166.47176.41
Sitjà-Rabert M., Rigau D., Fort Vanmeerghaeghe A., Romero-Rodríguez D., Bonastre Subirana M., Bonfill X. (2012). Efficacy of whole body vibration exercise in older people: A systematic review. Disabil. Rehabil. 34 (11), 883–893. doi:10.3109/09638288.2011.626486
Taheri B., Barati A., Norasteh A. A., Madadi-Shad M. (2018). EMG analysis of trunk and lower limb muscles in three different squat exercises in athletes and non-athletes. Int. J. Sport Stud. Health 1 (2), e79463. doi:10.5812/intjssh.79463
Taheri M., Irandoost K., Yousefi S., Jamali A. (2017). Effect of 8-week lower extremity weight-bearing exercise protocol and acute caffeine consumption on reaction time in postmenopausal women. Iran. J. Ageing 12 (1), 16–27. doi:10.21859/SIJA-120116
Torvinen S., Kannus P., Sievänen H., Järvinen T. A., Pasanen M., Kontulainen S., et al. (2002). Effect of 4-min vertical whole body vibration on muscle performance and body balance: A randomized cross-over study. Int. J. Sports Med. 23 (5), 374–379. doi:10.1055/s-2002-33148
Tsolakis C., Vrabas I. S., Vamvakoudis E., Christou C., Spengos K. (2020). Acute effects of whole-body vibration on oxygen uptake during and after different high-intensity squat protocols in resistance-trained men. J. Strength Cond. Res. 34 (11), 3204–3212. doi:10.1519/JSC.0000000000003106
Van Heuvelen M. J. G., Rittweger J., Judex S., Sañudo B., Seixas A., Fuermaier A. B. M., et al. (2021). Reporting guidelines for whole-body vibration studies in humans, animals and cell cultures: A consensus statement from an international group of experts. Biol. (Basel) 10 (10), 965. doi:10.3390/biology10100965
Vissers D., Baeyens J. P., Truijen S., Ides K., Vercruysse C. C., Van Gaal L. (2009). The effect of whole body vibration short-term exercises on respiratory gas exchange in overweight and obese women. Physician &Sportsmedicine 37 (37), 88–94. doi:10.3810/psm.2009.10.1733
Keywords: resistance exercise vibration, resistance exercise, oxygen uptake, energy metabolism, weight-bearing squats
Citation: Huang X, Ye Z and Qin X (2023) Effects of 4 weeks of whole-body vibration training on energy expenditure during deep squats of male well-trained students. Front. Physiol. 14:1232045. doi: 10.3389/fphys.2023.1232045
Received: 31 May 2023; Accepted: 26 September 2023;
Published: 11 October 2023.
Edited by:
Danúbia Da Cunha De Sá Caputo, Rio de Janeiro State University, BrazilReviewed by:
Morteza Taheri, University of Tehran, IranKhadijeh Irandoust, Imam Khomeini International University, Iran
Mario Bernardo-Filho, Rio de Janeiro State University, Brazil
Copyright © 2023 Huang, Ye and Qin. This is an open-access article distributed under the terms of the Creative Commons Attribution License (CC BY). The use, distribution or reproduction in other forums is permitted, provided the original author(s) and the copyright owner(s) are credited and that the original publication in this journal is cited, in accordance with accepted academic practice. No use, distribution or reproduction is permitted which does not comply with these terms.
*Correspondence: Xuelin Qin, cXh1ZWxpbkAxMjYuY29t
†These authors share first authorship