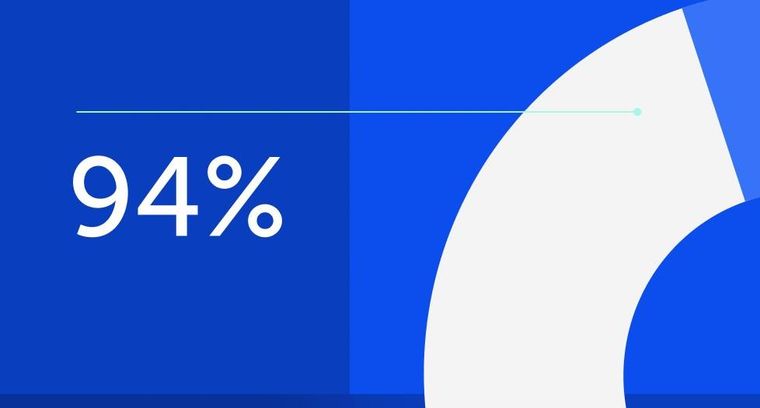
94% of researchers rate our articles as excellent or good
Learn more about the work of our research integrity team to safeguard the quality of each article we publish.
Find out more
REVIEW article
Front. Physiol., 01 June 2023
Sec. Membrane Physiology and Membrane Biophysics
Volume 14 - 2023 | https://doi.org/10.3389/fphys.2023.1213866
This article is part of the Research TopicTargeting Signals in Protein Trafficking and TransportView all 15 articles
The photosynthetic capacity of chloroplasts is vital for autotrophic growth in algae and plants. The origin of the chloroplast has been explained by the endosymbiotic theory that proposes the engulfment of a cyanobacterium by an ancestral eukaryotic cell followed by the transfer of many cyanobacterial genes to the host nucleus. As a result of the gene transfer, the now nuclear-encoded proteins acquired chloroplast targeting peptides (known as transit peptides; transit peptide) and are translated as preproteins in the cytosol. Transit peptides contain specific motifs and domains initially recognized by cytosolic factors followed by the chloroplast import components at the outer and inner envelope of the chloroplast membrane. Once the preprotein emerges on the stromal side of the chloroplast protein import machinery, the transit peptide is cleaved by stromal processing peptidase. In the case of thylakoid-localized proteins, cleavage of the transit peptides may expose a second targeting signal guiding the protein to the thylakoid lumen or allow insertion into the thylakoid membrane by internal sequence information. This review summarizes the common features of targeting sequences and describes their role in routing preproteins to and across the chloroplast envelope as well as the thylakoid membrane and lumen.
The chloroplast is a member of the plastid organelle family known mostly for its photosynthetic activity though it does perform a vast array of other metabolic activities essential to plant survival, development, and stress responses (Jarvis and López-Juez, 2013). Plastids are the result of an endosymbiotic process that started over a billion years ago (Zimorski et al., 2014). Since that time, most plastid genes have either been lost or transferred to the nucleus (Timmis et al., 2004). Of the 2,000 plus chloroplast proteins only about 10% remain encoded by the chloroplast genome. The nuclear-encoded chloroplast preproteins contain an N-terminal transit peptide (TP). The TP can be compared to a molecular zip code of preproteins to be targeted to the chloroplast and imported via the chloroplast protein import machinery (Lee and Hwang, 2021).
The import mechanism involves multiple steps at different (sub-)organellar locations. Initially, the preprotein is guided through the cytosol accompanied by a chaperone complex until it is handed off at the outer envelope of the chloroplast where the transit peptide makes first contact with the TOC (Translocon at the Outer envelope of the Chloroplast) complex (Flores-Pérez and Jarvis, 2013). This involves the action of the two GTP-binding receptors TOC159 and TOC34. In a process that requires GTP and low concentrations of ATP (0.1 mM), the preprotein is inserted across the large hybrid outer membrane protein-conducting channel that consists of the C-terminal ß-barrel membrane (M-) domain of TOC159 and that of TOC75 (Richardson et al., 2014; Schnell, 2019). At this stage already, the transit peptide is in contact with the intermembrane POTRA-domains of TOC75 and initiates contact with components of the TIC (translocon at the Inner envelope of the chloroplast) complex, namely, TIC22 and TIC20, however, without traversing the inner membrane (Kouranov et al., 1998). In the presence of high concentrations of ATP (> 1 mM) the preprotein crosses the TIC20 inner membrane protein-conducting channel and enters the chloroplast stroma assisted by ATP-dependent motor components (Richardson et al., 2018).
Once inside the stroma, the transit peptide is cleaved by the Stromal Processing Peptidase (SPP) (Richter and Lamppa, 1998). Many imported, mature proteins remain in the stroma and are folded with the help of chaperones. Some proteins, however, are targeted further to the thylakoid membrane or lumen. Thylakoid lumen targeted proteins possess bipartite targeting sequences consisting of a transit peptide followed by a thylakoid targeting signal that engages one of two pathways leading to the thylakoid lumen: The ΔpH-dependent TAT (twin arginine targeting) and SEC (secretory) pathways. However, the Signal Recognition Particle (SRP) pathway inserting proteins into the thylakoid membrane relies on targeting information residing within the mature sequence. Each of these pathways relies on a distinct set of protein components. Thylakoid targeting signals of preproteins are removed by a thylakoid processing peptidase (TPP) which promotes final assembly of the mature proteins leading to functional chloroplasts (Mori and Cline, 2001; Albiniak et al., 2012; Teixeira and Glaser, 2013).
“Signal Peptide” refers to an endoplasmic reticulum targeting sequence, “pre-sequence” to a mitochondrial one, and “transit peptide” is specific for chloroplast-targeted proteins (Bruce, 2000). In the late 1970s, after the signal hypothesis had been proposed, a study showed that in vitro translated Rubisco small unit (RbcS) protein had a higher molecular mass than mature RbcS in plant extracts. It was therefore considered a putative precursor (Dobberstein et al., 1977). The RbcS cDNA was cloned and revealed an N-terminal extension that was not present in the mature RbcS. It was identified as the chloroplast targeting sequence and coined “transit peptide” (Broglie et al., 1981; Coruzzi et al., 1983). Later studies demonstrated that the putative precursor of RbcS was transported into isolated chloroplasts and processed to its mature form (Highfield and Ellis, 1976; Chua and Schmidt, 1978). It has been proposed that transit peptides evolved from antimicrobial amphipathic peptides derived from host cells during endosymbiotic events, an intriguing hypothesis that is supported by experimental evidence (Caspari et al., 2023).
A motif study has shown that transit peptides contain three regions, a N-terminal region lacking charged amino acids, a central one containing hydroxylated amino acids and C-terminal one containing an arginine rich motif. This domain structure may be common to most preproteins (Karlin-Neumann and Tobin, 1986; von Heijne et al., 1989; Bruce, 2001). A later study, reporting extensive mutagenesis of the RbcS transit peptide, provided clues to the existence of FP/RK and MLM motifs in the transit peptide and their vital role in chloroplast protein import (Lee et al., 2006). Site-specific cross-linking experiments with the RbcS transit peptide, demonstrated that the FP/RK motif is important for interaction not only with components of the TOC complex, but also with the TIC20 component of the TIC complex (Richardson et al., 2018). In addition, FGLK is a transit peptide motif that has been characterized as being recurrent in transit peptides and playing an important role in the preprotein recognition by TOC34. The deletion of the FGLK sequence by mutagenesis prevented the preprotein from being translocated into the chloroplast (Chotewutmontri et al., 2012; Holbrook et al., 2016).
Based on a synthetic transit peptide, a study demonstrated that FGLK and FP/RK motifs are essential for RbcS transit peptide function and preprotein targeting of the chloroplast (Lee et al., 2015). Moderate hydrophobicity at the N-terminal region of the transit peptide is important for preprotein recognition, (Bhushan et al., 2006; Lee et al., 2006; Lee et al., 2008). Exchange of basic amino acids (N-terminal region) to acidic amino acids negatively affected preprotein import into chloroplasts (Razzak et al., 2017; Lee and Hwang, 2019). Twin-positive (positively charged amino acids) motifs in the TP appear to play a key role in preprotein import into old versus young chloroplasts (Teng et al., 2012). In addition, large scale in silico analysis and experimental evidence revealed that the twin-positive motif is important for preprotein import into leucoplasts (Chu et al., 2020).
The importance of proline residues in transit peptides has been demonstrated by comparing the import of preproteins containing proline-rich transit peptides with those lacking proline residues. The mutation of transit peptides by the replacement of prolines by alanines resulted in reduced efficiency of translocation into the chloroplast, specifically concerning transmembrane proteins and proteins prone to aggregation (Lee et al., 2018; Jeong et al., 2021). Proline is an amino acid that tends to disrupt the secondary structures of polypeptides (Guzzo, 1965). As preproteins are believed to be translocated across the TOC complex an unstructured transit peptide as described by the “perfect random coil hypothesis” may be advantageous to initiate the early stages of protein import (von Heijne and Nishikawa, 1991).
The energy requirement of preprotein transport across the chloroplast envelopes was first analyzed in an in vitro import assay using isolated chloroplasts that were either light- or dark-adapted. The study showed that import into dark-adapted chloroplasts was compromised (Grossman et al., 1980). Exogenously added ATP rescued imports into dark-adapted chloroplasts, demonstrating that ATP was the primary energy source (Cline et al., 1985). Later studies demonstrated that import of preproteins into chloroplasts was driven by the hydrolysis of ATP inside the chloroplast (Flügge and Hinz, 1986; Pain and Blobel, 1987; Theg et al., 1989). It was then revealed that distinct concentrations of ATP in different compartments defined separate steps of chloroplast protein import. Low concentrations of ATP (50–100 µM) were sufficient for preprotein binding to the surface of the chloroplast, whereas high concentrations of ATP (1 mM or more) were required for protein translocation across the chloroplast envelope (Olsen et al., 1989). The RbcS preprotein could be chemically crosslinked to chloroplast envelope component in an ATP-dependent manner (Perry and Keegstra, 1994).
The energetics findings were exploited to generate preprotein translocation intermediates and isolate the first components of the protein import machinery from isolated pea chloroplasts (Schnell and Blobel, 1993). In these experiments, recombinant preprotein of RbcS fused to two IgG-binding domains of Staphylococcus aureus ProteinA (resulting in pS-ProtA) was used as a tool. When incubated at low ATP concentrations, pS-ProtA is stably bound to isolated chloroplasts. pS-ProtA remained sensitive to exogenous protease and the transit peptide was not cleaved. This state defines the “early translocation intermediate”. When incubated at high ATP concentrations, pS-ProtA was fully imported. However, its import could be arrested by chilling on ice. At this stage, pS-ProtA was both accessible to exogenous protease and the transit peptide partially cleaved resulting in mature S-ProtA. Thus, pS-ProtA and S-ProtA had traversed and were now spanning both the outer and inner envelope membrane. This state defines the “late translocation intermediate”. It is important to note that the formation of both the “early” and “late” translocation intermediates critically depended on the presence of the transit peptide in pS-ProtA (Schnell and Blobel, 1993). The production of “early” and “late” translocation intermediates was upscaled from analytical to biochemical quantities allowing their isolation by IgG-affinity chromatography. The “early” translocation intermediate pS-ProtA was associated with three visible bands on a SDS-PAGE gel. These first three proteins were molecularly cloned and sequenced and are now known as TOC159, TOC75 and TOC34 (Kessler et al., 1994; Schnell et al., 1994). The three form the core of the TOC-complex as it is widely accepted today. In addition to the three core components of the TOC-complex, the “late” translocation intermediate pS-ProtA and S-ProtA associated with two more bands. One is known today as TIC110 while the second one, named IAP36 at the time, was never identified (Schnell et al., 1994). To this day, the role of TIC110 in chloroplast protein import remains contested and is notably absent from algal protein import complexes (Ramundo et al., 2020).
The large majority of chloroplast proteins are imported via the TOC-TIC complexes (Figure 1). The first components were identified in the beginning of 1990s as a result of studies on isolated pea chloroplasts and revealed three components of the outer and one at the inner envelope membranes (namely, Import intermediate Associated Proteins or Outer Envelope Protein), IAP/OEP34, IAP/OEP75, IAP/OEP86 and Inner Envelope Protein IAP100/IEP110 (Hirsch et al., 1994; Kessler et al., 1994; Perry and Keegstra, 1994; Schnell et al., 1994). These translocon components were renamed according to the TOC–TIC nomenclature as Toc34, Toc75, and Toc159 (for IAP/OEP86) and Tic110 (Schnell et al., 1997). The initial characterization revealed that TOC159 and TOC34 were homologous GTP-binding proteins exposed at the chloroplast surface. They were both sensitive to the addition of exogenous thermolysin protease fulfilling an important criterium for preprotein receptors at the chloroplast surface (Kessler et al., 1994). TOC75 was insensitive to exogenous thermolysin, bore homology to cyanobacterial ß-barrel solute channels related to the β-barrel assembly machinery A (BamA) family fulfilling criteria for a protein-conducting channel at the outer chloroplast membrane. TIC110 had two N-terminal alpha-helices and a large stromal domain suggesting that it may function as scaffold coordinating late translocation functions such as recruitment of chaperones for protein folding and assembly (Kessler and Blobel, 1996).
FIGURE 1. Preproteins translocation into chloroplast membrane systems. General scheme of chloroplast import of nuclear-encoded preproteins containing a transit peptide (TP) followed by a thylakoid Signal sequence (SS). Preprotein translocation passes through the Translocons at the Outer envelope (OE) of the Chloroplast (TOC) and the Inner envelope (IE) of the chloroplast (TIC). Upon entry, the transit peptide is cleaved by the Stromal Processing Peptidase (SPP) and processed by the PreP protease for recycling/degradation. The thylakoid-targeted proteins pass through either the Twin Arginine Transport (Tat) pathway requiring the Proton Motive Force (PMF), the Sec requiring ATP, or the Signal Recognition Particle (SRP) pathway. Upon thylakoid membrane insertion, the thylakoid signal sequence (SS) is cleaved by the Thylakoid Processing Peptidase (TPP), completing the final import step.
The presence of GTP-binding proteins in the TOC complex encouraged further energetics experimentation. Preprotein binding to chloroplasts does not only require low concentrations of ATP but also implicates GTP as non- and slowly-hydrolyzable GTP analogs inhibited import. These findings supported the importance of the role of TOC GTPase receptors (Olsen and Keegstra, 1992; Kessler et al., 1994). Apart from the irreversible energy-dependent interactions, the transit peptide is also reversibly bound to TOC159 and TOC75 in an energy-independent way as demonstrated by chemical cross-linking (Ma et al., 1996).
In Arabidopsis as well as other species, both of the GTP-binding TOCs are encoded by multigene families and consequently several isoforms of each have been discovered. The structure of TOC34 as well as those of its homologs consists of two main features, a N-terminal GTPase domain and a single C-terminal alpha-helical membrane-spanning domain followed by a short hydrophilic tail (Jarvis et al., 1998). TOC159 and its three homologs in Arabidopsis (atTOC120, −132, −90) possess a central GTPase (G-) domain, a C-terminal membrane-anchoring (M-) domain, and a N-terminal acidic (A-) domain at the N-terminus (Kubis et al., 2004). The M-domain has now been shown to take on a ß-barrel structure and associate with TOC75 to form a large hybrid channel at the outer chloroplast membrane (Jin et al., 2022; Liu et al., 2023). The A-domains in the four Arabidopsis isoforms of TOC159 are much more divergent than the G- and M-domains and appear to play a role in pre-protein specificity (Agne et al., 2010). It, however, is not clear how the various A-domains distinguish the transit peptides of different classes of preproteins (i.e., photosynthesis-associated versus house-keeping) (Bauer et al., 2000; Ivanova et al., 2004). TOC75 belongs to the BamA family with homologs in Gram-negative bacteria as well as mitochondria and plastids (Schleiff and Becker, 2011). Based on these similarities, TOC75 was proposed to function as the protein-conducting channel at the outer membrane of the chloroplast. TOC75 is encoded by a single orthologous gene in the genomes of all plant species sequenced so far. In addition to forming a ß-barrel channel, TOC75 has three N-terminal POTRA (polypeptide transport-associated) domains (Sánchez-Pulido et al., 2003; Srinivasan et al., 2023). The POTRA domain contributes to preprotein recognition and has chaperone-like activity to guide the incoming preprotein across the intermembrane space (Kouranov and Schnell, 1997; Paila et al., 2016; O'Neil et al., 2017).
At the inner envelope membrane, at least two models have been proposed for the TIC complex, the first consisting of the TIC20 (channel) TIC214 (plastid-encoded), TIC100, TIC56, TIC21 and TIC12 forming a 1 MDa complex (Kikuchi et al., 2013) the second consisting of TIC110 and TIC40. Currently, it is not clear whether the second complex functions together with or independently from the 1 MDa TIC complex in land plants. Cryo-EM structures of the Chlamydomonas TOC-TIC holocomplexes, however, did not contain homologs of TIC40 or TIC110 (Jin et al., 2022; Liu et al., 2023). In addition to the aforementioned components the intermembrane space component TIC236 constitutes a physical link between the TOC and TIC complexes (Chen et al., 2018). TIC22, another intermembrane space component, has been proposed to promote preprotein import across both envelope membranes and the intermembrane space besides its function as a chaperone (Kouranov et al., 1999). As preprotein import requires ATP, the existence of ATP-dependent motors has been proposed. However, the exact nature of such stromal import motor(s) is currently contested. On the one hand biochemical and genetic information provide support for a chaperone network consisting of cpHsp70, Hsp90C, and Hsp93) consuming the ATP and energizing translocation (Su and Li, 2010; Inoue et al., 2013; Huang et al., 2016). On the other hand, an alternative stromal motor has been proposed that consists of a 2-MDa ycf2/FtsH1 complex that also has predicted ATP hydrolysis activity (Kikuchi et al., 2018). However, the respective significance of the two proposed motor systems has not been evaluated so far, and neither of the two systems were observed in the currently available Cryo-EM structures (Jin et al., 2022; Liu et al., 2023).
Upon entry into the chloroplast stroma and possibly before complete translocation, the transit peptide is cleaved by Stromal Processing Peptidase (SPP) (Figure 1) (Richter and Lamppa, 2002; Richter and Lamppa, 2003). SPP is an M16 metallopeptidase carrying out a function comparable to that of the Mitochondrial Processing Peptidase (MPP), a metalloprotease, involved in the maturation of nuclear encoded proteins imported into mitochondria (Pollock et al., 1988; Braun et al., 1992). SPP, cleaves at a semiconserved motif ((I/V)-X-(A/C)-↓-A (arrow marks cleavage site) at the C-terminus of the transit peptide (von Heijne et al., 1989). Thereby initiating the final steps of preprotein maturation. After transit peptide cleavage, these may include folding and/or assembly in the stroma or insertion into or translocation across the thylakoid membrane. SPP is an essential component of the import mechanism as demonstrated by the aborted seed phenotype observed in the spp homozygous knockout mutants (Trösch and Jarvis, 2011). Once cleaved, transit peptides are further degraded by presequence peptidases (PrePs) (Figure 1) (Ståhl et al., 2005).
The thylakoid membrane is home to the light reactions of photosynthesis. For thylakoid biogenesis, assembly of thylakoid luminal and integral membrane proteins is essential. For a considerable number of proteins, the journey therefore is not finished upon arrival inside the chloroplast. Cleavage of the transit peptide may expose a secondary targeting sequence that will engage one of at least two entry pathways to the thylakoids. Two routes exist for entering the thylakoid lumen: the twin-arginine translocase (TAT) that may accommodate folded proteins, or the SEC translocase for unfolded proteins (Figure 1) (Yuan et al., 1994; Mori et al., 1999). In addition, integral thylakoid membrane proteins require the signal recognition particle (SRP) pathway for alternative insertion (Figure 1) (Schünemann, 2007). Interestingly, all three pathways have been conserved from the cyanobacterial ancestor and exist in bacteria and, in the case of the SEC and SRP pathways, in animals to this day.
The SEC pathway is well-known for its evolutionary conserved mechanism (Dalbey and Chen, 2004). In thylakoid targeting signals, the SEC-specific signal sequence has been described as containing three domains, a charged domain at the N-terminal part, a hydrophobic mid-section and C-terminal cleavage domain containing an A-x-A motif set for interaction with the thylakoid processing peptidase (TPP) (Hsu et al., 2011; Celedon and Cline, 2013). SEC1 is the SEC translocase at the thylakoid membrane (Fernandez, 2018). The SEC1 complex contains SCY1 and SECE1 thylakoid membrane protein channels associated with the stromal motor protein SECA1 (Nakai et al., 1994). Nuclear-encoded lumenal proteins are translocated in an unfolded form across the SEC translocase. The N-terminal part of the signal peptide interacts with SECA1 translocation motor and its ATPase activity provides the energy for translocation across the SCY1/SECE1 channel. Subsequently, the signal sequence is cleaved in the thylakoid lumen (Figure 1) (Albiniak et al., 2012). HSP90C may also assist the SEC1 translocation pathway in translocating thylakoid precursor proteins from the stroma to the lumen (Jiang et al., 2020). Surprisingly, a SEC2 translocase system also exists that is similar to SEC1, but SCY2 and SECE2 are inner envelope membrane protein channels using the stromal motor protein SECA2 (Skalitzky et al., 2011). The known examples of SEC2-dependent translocation of inner envelope proteins are TIC40 and FTSH12 (Li et al., 2017). However, the SEC2 translocase system is poorly understood compared to SEC1 due to a lack of studies.
The Twin Arginine Transport (Tat) pathway is so called because the corresponding targeting sequences contain two neighbouring arginine residues (Cline et al., 1992). The TAT pathway is distinct from others in that it is able to transport fully folded protein across the thylakoid membrane and into the lumen. The TAT-specific signal sequence features are similar to those of SEC with the exception of the N-terminal part that contains the twin arginine (RR) motif. The RR motif is responsible for SEC avoidance response in thylakoid targeting (New et al., 2018). The Tat pathway is estimated to be responsible for the import of an estimated 50% of the thylakoid lumen proteins (Robinson and Bolhuis, 2004). The characteristic twin-arginine motif is essential for translocation and is disabled by mutation to other combinations of amino acids. The TAT pathway requires only the proton motive force (pmf) as energy source in order to achieve protein transport and has therefore also been called the ∆pH pathway (Mould and Robinson, 1991). Three proteins named TatC, Hcf106 and Tha4 form a complex that binds to the precursor protein’s RRXFLK motif in the N-terminal part of the signal sequence in order to facilitate translocation (Figure 1). Liquid-liquid phase separation by Hcf106-ankyrin-repeat proteins (STT) interaction facilitates the TAT dependent translocation of the luminal proteins (Ouyang et al., 2020). Several models of translocation have been proposed for the plant TAT pathway. However, no proven model exists to date (New et al., 2018).
The chloroplast signal recognition particle (cpSRP) pathway, which is derived from prokaryotes and known as cpSRP pathway, targets and inserts abundant thylakoid membrane proteins, for example, light-harvesting chlorophyll-binding proteins (LHCPs) (Ziehe et al., 2018). Unlike SEC and TAT pathways, no conserved motif or domain is present at the N-terminal of the protein for thylakoid targeting. Several studies address LHCP recognition by the cpSRP pathway. The L18 motif (18 amino acids within the second and third transmembrane helices) of LHCP is crucial for recognition by cpSRP transit complex (Tu et al., 2000). Once nuclear-encoded LHCP is imported into the chloroplast via the TOC-TIC complex and processed by SPP, it forms the stromal transit complex together with cpSRP54 (GTPase) and cpSRP43 (Schuenemann et al., 1998). The cpSRP transit complex containing LHCP binds to cpSRP receptor cpFtsY (GTPase) (Kogata et al., 1999; Tu et al., 1999; Nguyen et al., 2011) and docks to Alb3 (insertase at thylakoid membrane) via cpSRP43, promoting precursor/LHCP insertion into thylakoid membrane (Figure 1) (Moore et al., 2000; Bals et al., 2010). cpSRP43 has two distinct chaperone activities for i) LHCP insertion and ii) tetrapyrrole biosynthesis enzymes. The chaperone activity towards tetrapyrolle biosynthesis activity allows to coordinate LHCP insertion with chlorophyll biosynthesis and assembly into LHCP. Interestingly, cpSRP54 activates the cpSRP43 chaperone function towards LHCP insertion and inhibits the chaperone activity towards tetrapyrrole biosynthesis enzymes (Wang et al., 2018; Ji et al., 2021). However, except for LHCP, there is a lack of information about how the SRP targets are recognized by the components of the SRP pathway.
The thylakoid proteins are translocated into the thylakoid lumen by either the Sec or Tat pathways and, in a final step the N-terminal thylakoid targeting sequence is cleaved by Thylakoid Processing Peptidase (TPP) (Figure 1) (Hsu et al., 2011). TPP is a member of the membrane-bound proteases belonging to the type I signal peptidase (SPase I) family in both prokaryotes and eukaryotes. Plsp1 and Plsp2A/B are the two TPPs present in the thylakoids (Hsu et al., 2011). Plsp1 is known to be involved in the SEC and TAT dependent signal sequence cleavage and, surprisingly also, processing of TOC75 at the envelope membrane, suggesting that at least the Plsp1 protease is found not only in the thylakoid membrane but also in the envelopes. Plsp1 is essential for chloroplast biogenesis, its mutation resulting in a very pale green phenotype (Shipman and Inoue, 2009). Currently, the physiological and functional roles of Plsp2A/B in signal peptide processing are unclear.
In the last years, significant progress has been made with regard to the understanding of the molecular and mechanistic details of chloroplast import of nuclear-encoded proteins by the TOC-TIC complex. The recent cryo-EM structural studies reveal how the TOC-TIC components are arranged in detail and provide some information on the likely path of the preprotein and its transit peptide across the chloroplast envelope. It would now be highly interesting to study the cryo-EM structure of the TOC-TIC complex in association with a preprotein and its transit peptide to gain a complete understanding of the import process. Also, the recent cryo-EM structures failed to reveal the cytosolic GTPase domains of the TOC34 and −159 (Jin et al., 2022; Liu et al., 2023) that play a central role in transit peptide recognition. The GTPase domains should remain a key target in future structural work. Recent advances in the chloroplast transit peptide field reveal that specific motifs, i.e., the proline-rich motif, have vital roles in the preprotein interaction with the TOC-TIC translocon. However, fundamental knowledge concerning the recognition and distinction of transit peptides belonging to different classes of preproteins (i.e., photosynthesis-associated vs. nonphotosynthetic housekeeping) is still lacking. In the future, the identification and investigation of particular motifs playing essential roles in tissue- and plastid-specific protein import pathways are predicted to be important research questions. Last but not least, many questions regarding second targeting sequences and their role in processing and assembly of the all-important photosystems remain open and should be addressed in the future.
FK and VS conceived this review article. GB, MF, FK, and VS participated in the writing of the manuscript. GB prepared the figure. All authors contributed to the article and approved the submitted version.
This work was supported by the Swiss National Science Foundation (SNSF) grant 310030_208000 to FK.
We thank the BioRender program for the image.
The authors declare that the research was conducted in the absence of any commercial or financial relationships that could be construed as a potential conflict of interest.
All claims expressed in this article are solely those of the authors and do not necessarily represent those of their affiliated organizations, or those of the publisher, the editors and the reviewers. Any product that may be evaluated in this article, or claim that may be made by its manufacturer, is not guaranteed or endorsed by the publisher.
Agne, B., Andrès, C., Montandon, C., Christ, B., Ertan, A., Jung, F., et al. (2010). The acidic A-domain of Arabidopsis TOC159 occurs as a hyperphosphorylated protein. Plant Physiol. 153, 1016–1030. doi:10.1104/pp.110.158048
Albiniak, A. M., Baglieri, J., and Robinson, C. (2012). Targeting of lumenal proteins across the thylakoid membrane. J. Exp. Bot. 63, 1689–1698. doi:10.1093/jxb/err444
Bals, T., Dünschede, B., Funke, S., and Schünemann, D. (2010). Interplay between the cpSRP pathway components, the substrate LHCP and the translocase Alb3: An in vivo and in vitro study. FEBS Lett. 584, 4138–4144. doi:10.1016/j.febslet.2010.08.053
Bauer, J., Chen, K., Hiltbunner, A., Wehrli, E., Eugster, M., Schnell, D., et al. (2000). The major protein import receptor of plastids is essential for chloroplast biogenesis. Nature 403, 203–207. doi:10.1038/35003214
Bhushan, S., Kuhn, C., Berglund, A. K., Roth, C., and Glaser, E. (2006). The role of the N-terminal domain of chloroplast targeting peptides in organellar protein import and miss-sorting. FEBS Lett. 580, 3966–3972. doi:10.1016/j.febslet.2006.06.018
Braun, H. P., Emmermann, M., Kruft, V., and Schmitz, U. K. (1992). The general mitochondrial processing peptidase from potato is an integral part of cytochrome c reductase of the respiratory chain. Embo J. 11, 3219–3227. doi:10.1002/j.1460-2075.1992.tb05399.x
Broglie, R., Bellemare, G., Bartlett, S. G., Chua, N. H., and Cashmore, A. R. (1981). Cloned DNA sequences complementary to mRNAs encoding precursors to the small subunit of ribulose-1,5-bisphosphate carboxylase and a chlorophyll a/b binding polypeptide. Proc. Natl. Acad. Sci. U. S. A. 78, 7304–7308. doi:10.1073/pnas.78.12.7304
Bruce, B. D. (2000). Chloroplast transit peptides: Structure, function and evolution. Trends Cell Biol. 10, 440–447. doi:10.1016/s0962-8924(00)01833-x
Bruce, B. D. (2001). The paradox of plastid transit peptides: Conservation of function despite divergence in primary structure. Biochim. Biophys. Acta 1541, 2–21. doi:10.1016/s0167-4889(01)00149-5
Caspari, O. D., Garrido, C., Law, C. O., Choquet, Y., Wollman, F. A., and Lafontaine, I. (2023). Converting antimicrobial into targeting peptides reveals key features governing protein import into mitochondria and chloroplasts. Plant Commun. 2023, 100555. doi:10.1016/j.xplc.2023.100555
Celedon, J. M., and Cline, K. (2013). Intra-plastid protein trafficking: How plant cells adapted prokaryotic mechanisms to the eukaryotic condition. Biochim. Biophys. Acta 1833, 341–351. doi:10.1016/j.bbamcr.2012.06.028
Chen, Y. L., Chen, L. J., Chu, C. C., Huang, P. K., Wen, J. R., and Li, H. M. (2018). TIC236 links the outer and inner membrane translocons of the chloroplast. Nature 564, 125–129. doi:10.1038/s41586-018-0713-y
Chotewutmontri, P., Reddick, L. E., Mcwilliams, D. R., Campbell, I. M., and Bruce, B. D. (2012). Differential transit peptide recognition during preprotein binding and translocation into flowering plant plastids. Plant Cell 24, 3040–3059. doi:10.1105/tpc.112.098327
Chu, C. C., Swamy, K., and Li, H. M. (2020). Tissue-specific regulation of plastid protein import via transit-peptide motifs. Plant Cell 32, 1204–1217. doi:10.1105/tpc.19.00702
Chua, N. H., and Schmidt, G. W. (1978). In vitro synthesis, transport, and assembly of ribulose 1,5-bisphosphate carboxylase subunits. Basic Life Sci. 11, 325–347. doi:10.1007/978-1-4684-8106-8_20
Cline, K., Ettinger, W. F., and Theg, S. M. (1992). Protein-specific energy requirements for protein transport across or into thylakoid membranes. Two lumenal proteins are transported in the absence of ATP. J. Biol. Chem. 267, 2688–2696. doi:10.1016/s0021-9258(18)45935-2
Cline, K., Werner-Washburne, M., Lubben, T. H., and Keegstra, K. (1985). Precursors to two nuclear-encoded chloroplast proteins bind to the outer envelope membrane before being imported into chloroplasts. J. Biol. Chem. 260, 3691–3696. doi:10.1016/s0021-9258(19)83678-5
Coruzzi, G., Broglie, R., Cashmore, A., and Chua, N. H. (1983). Nucleotide sequences of two pea cDNA clones encoding the small subunit of ribulose 1,5-bisphosphate carboxylase and the major chlorophyll a/b-binding thylakoid polypeptide. J. Biol. Chem. 258, 1399–1402. doi:10.1016/s0021-9258(18)32995-8
Dalbey, R. E., and Chen, M. (2004). Sec-translocase mediated membrane protein biogenesis. Biochim. Biophys. Acta 1694, 37–53. doi:10.1016/j.bbamcr.2004.03.009
Dobberstein, B., Blobel, G., and Chua, N. H. (1977). In vitro synthesis and processing of a putative precursor for the small subunit of ribulose-1,5-bisphosphate carboxylase of Chlamydomonas reinhardtii. Proc. Natl. Acad. Sci. U. S. A. 74, 1082–1085. doi:10.1073/pnas.74.3.1082
Fernandez, D. E. (2018). Two paths diverged in the stroma: Targeting to dual SEC translocase systems in chloroplasts. Photosynth Res. 138, 277–287. doi:10.1007/s11120-018-0541-9
Flores-Pérez, Ú., and Jarvis, P. (2013). Molecular chaperone involvement in chloroplast protein import. Biochim. Biophys. Acta 1833, 332–340. doi:10.1016/j.bbamcr.2012.03.019
Flügge, U. I., and Hinz, G. (1986). Energy dependence of protein translocation into chloroplasts. Eur. J. Biochem. 160, 563–570. doi:10.1111/j.1432-1033.1986.tb10075.x
Grossman, A. R., Bartlett, S. G., Schmidt, G. W., and Chua, N. H. (1980). Post-translational uptake of cytoplasmically synthesized proteins by intact chloroplasts in vitro. Ann. N. Y. Acad. Sci. 343, 266–274. doi:10.1111/j.1749-6632.1980.tb47257.x
Guzzo, A. V. (1965). The influence of amino-acid sequence on protein structure. Biophys. J. 5, 809–822. doi:10.1016/S0006-3495(65)86753-4
Highfield, P. E., and Ellis, R. J. (1976). Protein synthesis in chloroplasts. VII. Initiation of protein synthesis in isolated intact pea chloroplasts. Biochim. Biophys. Acta 447, 20–27. doi:10.1016/0005-2787(76)90091-5
Hirsch, S., Muckel, E., Heemeyer, F., Von Heijne, G., and Soll, J. (1994). A receptor component of the chloroplast protein translocation machinery. Science 266, 1989–1992. doi:10.1126/science.7801125
Holbrook, K., Subramanian, C., Chotewutmontri, P., Reddick, L. E., Wright, S., Zhang, H., et al. (2016). Functional analysis of semi-conserved transit peptide motifs and mechanistic implications in precursor targeting and recognition. Mol. Plant 9, 1286–1301. doi:10.1016/j.molp.2016.06.004
Hsu, S. C., Endow, J. K., Ruppel, N. J., Roston, R. L., Baldwin, A. J., and Inoue, K. (2011). Functional diversification of thylakoidal processing peptidases in Arabidopsis thaliana. PLoS One 6, e27258. doi:10.1371/journal.pone.0027258
Huang, P. K., Chan, P. T., Su, P. H., Chen, L. J., and Li, H. M. (2016). Chloroplast Hsp93 directly binds to transit peptides at an early stage of the preprotein import process. Plant Physiol. 170, 857–866. doi:10.1104/pp.15.01830
Inoue, H., Li, M., and Schnell, D. J. (2013). An essential role for chloroplast heat shock protein 90 (Hsp90C) in protein import into chloroplasts. Proc. Natl. Acad. Sci. U. S. A. 110, 3173–3178. doi:10.1073/pnas.1219229110
Ivanova, Y., Smith, M. D., Chen, K., and Schnell, D. J. (2004). Members of the Toc159 import receptor family represent distinct pathways for protein targeting to plastids. Mol. Biol. Cell 15, 3379–3392. doi:10.1091/mbc.e03-12-0923
Jarvis, P., Chen, L. J., Li, H., Peto, C. A., Fankhauser, C., and Chory, J. (1998). An Arabidopsis mutant defective in the plastid general protein import apparatus. Science 282, 100–103. doi:10.1126/science.282.5386.100
Jarvis, P., and López-Juez, E. (2013). Biogenesis and homeostasis of chloroplasts and other plastids. Nat. Rev. Mol. Cell Biol. 14, 787–802. doi:10.1038/nrm3702
Jeong, J., Hwang, I., and Lee, D. W. (2021). Functional organization of sequence motifs in diverse transit peptides of chloroplast proteins. Front. Physiol. 12, 795156. doi:10.3389/fphys.2021.795156
Ji, S., Siegel, A., Shan, S. O., Grimm, B., and Wang, P. (2021). Chloroplast SRP43 autonomously protects chlorophyll biosynthesis proteins against heat shock. Nat. Plants 7, 1420–1432. doi:10.1038/s41477-021-00994-y
Jiang, T., Mu, B., and Zhao, R. (2020). Plastid chaperone HSP90C guides precursor proteins to the SEC translocase for thylakoid transport. J. Exp. Bot. 71, 7073–7087. doi:10.1093/jxb/eraa399
Jin, Z., Wan, L., Zhang, Y., Li, X., Cao, Y., Liu, H., et al. (2022). Structure of a TOC-TIC supercomplex spanning two chloroplast envelope membranes. Cell 185, 4788–4800.e13. doi:10.1016/j.cell.2022.10.030
Karlin-Neumann, G. A., and Tobin, E. M. (1986). Transit peptides of nuclear-encoded chloroplast proteins share a common amino acid framework. Embo J. 5, 9–13. doi:10.1002/j.1460-2075.1986.tb04170.x
Kessler, F., and Blobel, G. (1996). Interaction of the protein import and folding machineries of the chloroplast. Proc. Natl. Acad. Sci. U. S. A. 93, 7684–7689. doi:10.1073/pnas.93.15.7684
Kessler, F., Blobel, G., Patel, H. A., and Schnell, D. J. (1994). Identification of two GTP-binding proteins in the chloroplast protein import machinery. Science 266, 1035–1039. doi:10.1126/science.7973656
Kikuchi, S., Asakura, Y., Imai, M., Nakahira, Y., Kotani, Y., Hashiguchi, Y., et al. (2018). A ycf2-FtsHi heteromeric AAA-ATPase complex is required for chloroplast protein import. Plant Cell 30, 2677–2703. doi:10.1105/tpc.18.00357
Kikuchi, S., Bédard, J., Hirano, M., Hirabayashi, Y., Oishi, M., Imai, M., et al. (2013). Uncovering the protein translocon at the chloroplast inner envelope membrane. Science 339, 571–574. doi:10.1126/science.1229262
Kogata, N., Nishio, K., Hirohashi, T., Kikuchi, S., and Nakai, M. (1999). Involvement of a chloroplast homologue of the signal recognition particle receptor protein, FtsY, in protein targeting to thylakoids. FEBS Lett. 447, 329–333. doi:10.1016/s0014-5793(99)00305-1
Kouranov, A., Chen, X., Fuks, B., and Schnell, D. J. (1998). Tic20 and Tic22 are new components of the protein import apparatus at the chloroplast inner envelope membrane. J. Cell Biol. 143, 991–1002. doi:10.1083/jcb.143.4.991
Kouranov, A., and Schnell, D. J. (1997). Analysis of the interactions of preproteins with the import machinery over the course of protein import into chloroplasts. J. Cell Biol. 139, 1677–1685. doi:10.1083/jcb.139.7.1677
Kouranov, A., Wang, H., and Schnell, D. J. (1999). Tic22 is targeted to the intermembrane space of chloroplasts by a novel pathway. J. Biol. Chem. 274, 25181–25186. doi:10.1074/jbc.274.35.25181
Kubis, S., Patel, R., Combe, J., Bédard, J., Kovacheva, S., Lilley, K., et al. (2004). Functional specialization amongst the Arabidopsis Toc159 family of chloroplast protein import receptors. Plant Cell 16, 2059–2077. doi:10.1105/tpc.104.023309
Lee, D. W., and Hwang, I. (2019). Protein import into chloroplasts via the Tic40-dependent and -independent pathways depends on the amino acid composition of the transit peptide. Biochem. Biophys. Res. Commun. 518, 66–71. doi:10.1016/j.bbrc.2019.08.009
Lee, D. W., and Hwang, I. (2021). Understanding the evolution of endosymbiotic organelles based on the targeting sequences of organellar proteins. New Phytol. 230, 924–930. doi:10.1111/nph.17167
Lee, D. W., Kim, J. K., Lee, S., Choi, S., Kim, S., and Hwang, I. (2008). Arabidopsis nuclear-encoded plastid transit peptides contain multiple sequence subgroups with distinctive chloroplast-targeting sequence motifs. Plant Cell 20, 1603–1622. doi:10.1105/tpc.108.060541
Lee, D. W., Lee, S., Lee, G. J., Lee, K. H., Kim, S., Cheong, G. W., et al. (2006). Functional characterization of sequence motifs in the transit peptide of Arabidopsis small subunit of rubisco. Plant Physiol. 140, 466–483. doi:10.1104/pp.105.074575
Lee, D. W., Woo, S., Geem, K. R., and Hwang, I. (2015). Sequence motifs in transit peptides act as independent functional units and can Be transferred to new sequence contexts. Plant Physiol. 169, 471–484. doi:10.1104/pp.15.00842
Lee, D. W., Yoo, Y. J., Razzak, M. A., and Hwang, I. (2018). Prolines in transit peptides are crucial for efficient preprotein translocation into chloroplasts. Plant Physiol. 176, 663–677. doi:10.1104/pp.17.01553
Li, Y., Martin, J. R., Aldama, G. A., Fernandez, D. E., and Cline, K. (2017). Identification of putative substrates of SEC2, a chloroplast inner envelope translocase. Plant Physiol. 173, 2121–2137. doi:10.1104/pp.17.00012
Liu, H., Li, A., Rochaix, J. D., and Liu, Z. (2023). Architecture of chloroplast TOC-TIC translocon supercomplex. Nature 615, 349–357. doi:10.1038/s41586-023-05744-y
Ma, Y., Kouranov, A., Lasala, S. E., and Schnell, D. J. (1996). Two components of the chloroplast protein import apparatus, IAP86 and IAP75, interact with the transit sequence during the recognition and translocation of precursor proteins at the outer envelope. J. Cell Biol. 134, 315–327. doi:10.1083/jcb.134.2.315
Moore, M., Harrison, M. S., Peterson, E. C., and Henry, R. (2000). Chloroplast Oxa1p homolog albino3 is required for post-translational integration of the light harvesting chlorophyll-binding protein into thylakoid membranes. J. Biol. Chem. 275, 1529–1532. doi:10.1074/jbc.275.3.1529
Mori, H., and Cline, K. (2001). Post-translational protein translocation into thylakoids by the Sec and DeltapH-dependent pathways. Biochim. Biophys. Acta 1541, 80–90. doi:10.1016/s0167-4889(01)00150-1
Mori, H., Summer, E. J., Ma, X., and Cline, K. (1999). Component specificity for the thylakoidal Sec and Delta pH-dependent protein transport pathways. J. Cell Biol. 146, 45–56. doi:10.1083/jcb.146.1.45
Mould, R. M., and Robinson, C. (1991). A proton gradient is required for the transport of two lumenal oxygen-evolving proteins across the thylakoid membrane. J. Biol. Chem. 266, 12189–12193. doi:10.1016/s0021-9258(18)98879-4
Nakai, M., Goto, A., Nohara, T., Sugita, D., and Endo, T. (1994). Identification of the SecA protein homolog in pea chloroplasts and its possible involvement in thylakoidal protein transport. J. Biol. Chem. 269, 31338–31341. doi:10.1016/s0021-9258(18)31698-3
New, C. P., Ma, Q., and Dabney-Smith, C. (2018). Routing of thylakoid lumen proteins by the chloroplast twin arginine transport pathway. Photosynth Res. 138, 289–301. doi:10.1007/s11120-018-0567-z
Nguyen, T. X., Chandrasekar, S., Neher, S., Walter, P., and Shan, S. O. (2011). Concerted complex assembly and GTPase activation in the chloroplast signal recognition particle. Biochemistry 50, 7208–7217. doi:10.1021/bi200742a
O'neil, P. K., Richardson, L. G. L., Paila, Y. D., Piszczek, G., Chakravarthy, S., Noinaj, N., et al. (2017). The POTRA domains of Toc75 exhibit chaperone-like function to facilitate import into chloroplasts. Proc. Natl. Acad. Sci. U. S. A. 114, E4868–e4876. doi:10.1073/pnas.1621179114
Olsen, L. J., and Keegstra, K. (1992). The binding of precursor proteins to chloroplasts requires nucleoside triphosphates in the intermembrane space. J. Biol. Chem. 267, 433–439. doi:10.1016/s0021-9258(18)48513-4
Olsen, L. J., Theg, S. M., Selman, B. R., and Keegstra, K. (1989). ATP is required for the binding of precursor proteins to chloroplasts. J. Biol. Chem. 264, 6724–6729. doi:10.1016/s0021-9258(18)83489-5
Ouyang, M., Li, X., Zhang, J., Feng, P., Pu, H., Kong, L., et al. (2020). Liquid-liquid phase transition drives intra-chloroplast cargo sorting. Cell 180, 1144–1159. doi:10.1016/j.cell.2020.02.045
Paila, Y. D., Richardson, L. G., Inoue, H., Parks, E. S., Mcmahon, J., Inoue, K., et al. (2016). Multi-functional roles for the polypeptide transport associated domains of Toc75 in chloroplast protein import. Elife 5, e12631. doi:10.7554/eLife.12631
Pain, D., and Blobel, G. (1987). Protein import into chloroplasts requires a chloroplast ATPase. Proc. Natl. Acad. Sci. U. S. A. 84, 3288–3292. doi:10.1073/pnas.84.10.3288
Perry, S. E., and Keegstra, K. (1994). Envelope membrane proteins that interact with chloroplastic precursor proteins. Plant Cell 6, 93–105. doi:10.1105/tpc.6.1.93
Pollock, R. A., Hartl, F. U., Cheng, M. Y., Ostermann, J., Horwich, A., and Neupert, W. (1988). The processing peptidase of yeast mitochondria: The two co-operating components MPP and PEP are structurally related. Embo J. 7, 3493–3500. doi:10.1002/j.1460-2075.1988.tb03225.x
Ramundo, S., Asakura, Y., Salomé, P. A., Strenkert, D., Boone, M., Mackinder, L. C. M., et al. (2020). Coexpressed subunits of dual genetic origin define a conserved supercomplex mediating essential protein import into chloroplasts. Proc. Natl. Acad. Sci. U. S. A. 117, 32739–32749. doi:10.1073/pnas.2014294117
Razzak, M. A., Lee, D. W., Yoo, Y. J., and Hwang, I. (2017). Evolution of rubisco complex small subunit transit peptides from algae to plants. Sci. Rep. 7, 9279. doi:10.1038/s41598-017-09473-x
Richardson, L. G. L., Small, E. L., Inoue, H., and Schnell, D. J. (2018). Molecular topology of the transit peptide during chloroplast protein import. Plant Cell 30, 1789–1806. doi:10.1105/tpc.18.00172
Richardson, L. G., Paila, Y. D., Siman, S. R., Chen, Y., Smith, M. D., and Schnell, D. J. (2014). Targeting and assembly of components of the TOC protein import complex at the chloroplast outer envelope membrane. Front. Plant Sci. 5, 269. doi:10.3389/fpls.2014.00269
Richter, S., and Lamppa, G. K. (1998). A chloroplast processing enzyme functions as the general stromal processing peptidase. Proc. Natl. Acad. Sci. U. S. A. 95, 7463–7468. doi:10.1073/pnas.95.13.7463
Richter, S., and Lamppa, G. K. (2002). Determinants for removal and degradation of transit peptides of chloroplast precursor proteins. J. Biol. Chem. 277, 43888–43894. doi:10.1074/jbc.M206020200
Richter, S., and Lamppa, G. K. (2003). Structural properties of the chloroplast stromal processing peptidase required for its function in transit peptide removal. J. Biol. Chem. 278, 39497–39502. doi:10.1074/jbc.M305729200
Robinson, C., and Bolhuis, A. (2004). Tat-dependent protein targeting in prokaryotes and chloroplasts. Biochim. Biophys. Acta 1694, 135–147. doi:10.1016/j.bbamcr.2004.03.010
Sánchez-Pulido, L., Devos, D., Genevrois, S., Vicente, M., and Valencia, A. (2003). Potra: A conserved domain in the FtsQ family and a class of beta-barrel outer membrane proteins. Trends Biochem. Sci. 28, 523–526. doi:10.1016/j.tibs.2003.08.003
Schleiff, E., and Becker, T. (2011). Common ground for protein translocation: Access control for mitochondria and chloroplasts. Nat. Rev. Mol. Cell Biol. 12, 48–59. doi:10.1038/nrm3027
Schnell, D. J. (2019). The TOC GTPase receptors: Regulators of the fidelity, specificity and substrate profiles of the general protein import machinery of chloroplasts. Protein J. 38, 343–350. doi:10.1007/s10930-019-09846-3
Schnell, D. J., and Blobel, G. (1993). Identification of intermediates in the pathway of protein import into chloroplasts and their localization to envelope contact sites. J. Cell Biol. 120, 103–115. doi:10.1083/jcb.120.1.103
Schnell, D. J., Blobel, G., Keegstra, K., Kessler, F., Ko, K., and Soll, J. (1997). A consensus nomenclature for the protein-import components of the chloroplast envelope. Trends Cell Biol. 7, 303–304. doi:10.1016/S0962-8924(97)01111-2
Schnell, D. J., Kessler, F., and Blobel, G. (1994). Isolation of components of the chloroplast protein import machinery. Science 266, 1007–1012. doi:10.1126/science.7973649
Schuenemann, D., Gupta, S., Persello-Cartieaux, F., Klimyuk, V. I., Jones, J. D., Nussaume, L., et al. (1998). A novel signal recognition particle targets light-harvesting proteins to the thylakoid membranes. Proc. Natl. Acad. Sci. U. S. A. 95, 10312–10316. doi:10.1073/pnas.95.17.10312
Schünemann, D. (2007). Mechanisms of protein import into thylakoids of chloroplasts. Biol. Chem. 388, 907–915. doi:10.1515/BC.2007.111
Shipman, R. L., and Inoue, K. (2009). Suborganellar localization of plastidic type I signal peptidase 1 depends on chloroplast development. FEBS Lett. 583, 938–942. doi:10.1016/j.febslet.2009.02.016
Skalitzky, C. A., Martin, J. R., Harwood, J. H., Beirne, J. J., Adamczyk, B. J., Heck, G. R., et al. (2011). Plastids contain a second sec translocase system with essential functions. Plant Physiol. 155, 354–369. doi:10.1104/pp.110.166546
Srinivasan, K., Erramilli, S. K., Chakravarthy, S., Gonzalez, A., Kossiakoff, A., and Noinaj, N. (2023). Characterization of synthetic antigen binding fragments targeting Toc75 for the isolation of TOC in A. thaliana and P. sativum. Structure 31, 595–606. doi:10.1016/j.str.2023.03.002
Ståhl, A., Nilsson, S., Lundberg, P., Bhushan, S., Biverståhl, H., Moberg, P., et al. (2005). Two novel targeting peptide degrading proteases, PrePs, in mitochondria and chloroplasts, so similar and still different. J. Mol. Biol. 349, 847–860. doi:10.1016/j.jmb.2005.04.023
Su, P. H., and Li, H. M. (2010). Stromal Hsp70 is important for protein translocation into pea and Arabidopsis chloroplasts. Plant Cell 22, 1516–1531. doi:10.1105/tpc.109.071415
Teixeira, P. F., and Glaser, E. (2013). Processing peptidases in mitochondria and chloroplasts. Biochim. Biophys. Acta 1833, 360–370. doi:10.1016/j.bbamcr.2012.03.012
Teng, Y. S., Chan, P. T., and Li, H. M. (2012). Differential age-dependent import regulation by signal peptides. PLoS Biol. 10, e1001416. doi:10.1371/journal.pbio.1001416
Theg, S. M., Bauerle, C., Olsen, L. J., Selman, B. R., and Keegstra, K. (1989). Internal ATP is the only energy requirement for the translocation of precursor proteins across chloroplastic membranes. J. Biol. Chem. 264, 6730–6736. doi:10.1016/s0021-9258(18)83490-1
Timmis, J. N., Ayliffe, M. A., Huang, C. Y., and Martin, W. (2004). Endosymbiotic gene transfer: Organelle genomes forge eukaryotic chromosomes. Nat. Rev. Genet. 5, 123–135. doi:10.1038/nrg1271
Trösch, R., and Jarvis, P. (2011). The stromal processing peptidase of chloroplasts is essential in Arabidopsis, with knockout mutations causing embryo arrest after the 16-cell stage. PLoS One 6, e23039. doi:10.1371/journal.pone.0023039
Tu, C. J., Peterson, E. C., Henry, R., and Hoffman, N. E. (2000). The L18 domain of light-harvesting chlorophyll proteins binds to chloroplast signal recognition particle 43. J. Biol. Chem. 275, 13187–13190. doi:10.1074/jbc.c000108200
Tu, C. J., Schuenemann, D., and Hoffman, N. E. (1999). Chloroplast FtsY, chloroplast signal recognition particle, and GTP are required to reconstitute the soluble phase of light-harvesting chlorophyll protein transport into thylakoid membranes. J. Biol. Chem. 274, 27219–27224. doi:10.1074/jbc.274.38.27219
Von Heijne, G., and Nishikawa, K. (1991). Chloroplast transit peptides. The perfect random coil? FEBS Lett. 278, 1–3. doi:10.1016/0014-5793(91)80069-f
Von Heijne, G., Steppuhn, J., and Herrmann, R. G. (1989). Domain structure of mitochondrial and chloroplast targeting peptides. Eur. J. Biochem. 180, 535–545. doi:10.1111/j.1432-1033.1989.tb14679.x
Wang, P., Liang, F. C., Wittmann, D., Siegel, A., Shan, S. O., and Grimm, B. (2018). Chloroplast SRP43 acts as a chaperone for glutamyl-tRNA reductase, the rate-limiting enzyme in tetrapyrrole biosynthesis. Proc. Natl. Acad. Sci. U. S. A. 115, E3588–e3596. doi:10.1073/pnas.1719645115
Yuan, J., Henry, R., Mccaffery, M., and Cline, K. (1994). SecA homolog in protein transport within chloroplasts: Evidence for endosymbiont-derived sorting. Science 266, 796–798. doi:10.1126/science.7973633
Ziehe, D., Dünschede, B., and Schünemann, D. (2018). Molecular mechanism of SRP-dependent light-harvesting protein transport to the thylakoid membrane in plants. Photosynth Res. 138, 303–313. doi:10.1007/s11120-018-0544-6
Keywords: chloroplasts, preprotein, transit peptides, TOC-TIC, thylakoid
Citation: Ballabani G, Forough M, Kessler F and Shanmugabalaji V (2023) The journey of preproteins across the chloroplast membrane systems. Front. Physiol. 14:1213866. doi: 10.3389/fphys.2023.1213866
Received: 28 April 2023; Accepted: 22 May 2023;
Published: 01 June 2023.
Edited by:
Inhwan Hwang, Pohang University of Science and Technology, Republic of KoreaReviewed by:
Dong Wook Lee, Chonnam National University, Republic of KoreaCopyright © 2023 Ballabani, Forough, Kessler and Shanmugabalaji. This is an open-access article distributed under the terms of the Creative Commons Attribution License (CC BY). The use, distribution or reproduction in other forums is permitted, provided the original author(s) and the copyright owner(s) are credited and that the original publication in this journal is cited, in accordance with accepted academic practice. No use, distribution or reproduction is permitted which does not comply with these terms.
*Correspondence: Felix Kessler, ZmVsaXgua2Vzc2xlckB1bmluZS5jaA==; Venkatasalam Shanmugabalaji, c2hhbm11Z2FiYWxhamkudmVua2F0YXNhbGFtQHVuaW5lLmNo
†These authors have contributed equally to this work
Disclaimer: All claims expressed in this article are solely those of the authors and do not necessarily represent those of their affiliated organizations, or those of the publisher, the editors and the reviewers. Any product that may be evaluated in this article or claim that may be made by its manufacturer is not guaranteed or endorsed by the publisher.
Research integrity at Frontiers
Learn more about the work of our research integrity team to safeguard the quality of each article we publish.