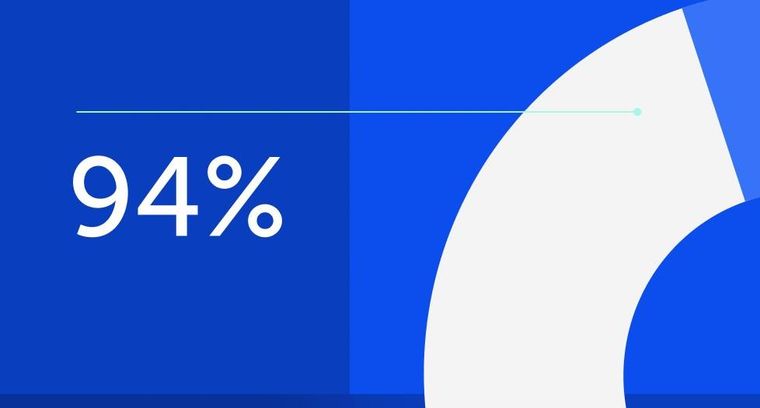
94% of researchers rate our articles as excellent or good
Learn more about the work of our research integrity team to safeguard the quality of each article we publish.
Find out more
ORIGINAL RESEARCH article
Front. Physiol., 04 August 2023
Sec. Respiratory Physiology and Pathophysiology
Volume 14 - 2023 | https://doi.org/10.3389/fphys.2023.1204531
Background. Global and regional transpulmonary pressure (PL) during one-lung ventilation (OLV) is poorly characterized. We hypothesized that global and regional PL and driving PL (ΔPL) increase during protective low tidal volume OLV compared to two-lung ventilation (TLV), and vary with body position.
Methods. In sixteen anesthetized juvenile pigs, intra-pleural pressure sensors were placed in ventral, dorsal, and caudal zones of the left hemithorax by video-assisted thoracoscopy. A right thoracotomy was performed and lipopolysaccharide administered intravenously to mimic the inflammatory response due to thoracic surgery. Animals were ventilated in a volume-controlled mode with a tidal volume (VT) of 6 mL kg−1 during TLV and of 5 mL kg−1 during OLV and a positive end-expiratory pressure (PEEP) of 5 cmH2O. Global and local transpulmonary pressures were calculated. Lung instability was defined as end-expiratory PL<2.9 cmH2O according to previous investigations. Variables were acquired during TLV (TLVsupine), left lung ventilation in supine (OLVsupine), semilateral (OLVsemilateral), lateral (OLVlateral) and prone (OLVprone) positions randomized according to Latin-square sequence. Effects of position were tested using repeated measures ANOVA.
Results. End-expiratory PL and ΔPL were higher during OLVsupine than TLVsupine. During OLV, regional end-inspiratory PL and ΔPL did not differ significantly among body positions. Yet, end-expiratory PL was lower in semilateral (ventral: 4.8 ± 2.9 cmH2O; caudal: 3.1 ± 2.6 cmH2O) and lateral (ventral: 1.9 ± 3.3 cmH2O; caudal: 2.7 ± 1.7 cmH2O) compared to supine (ventral: 4.8 ± 2.9 cmH2O; caudal: 3.1 ± 2.6 cmH2O) and prone position (ventral: 1.7 ± 2.5 cmH2O; caudal: 3.3 ± 1.6 cmH2O), mainly in ventral (p ≤ 0.001) and caudal (p = 0.007) regions. Lung instability was detected more often in semilateral (26 out of 48 measurements; p = 0.012) and lateral (29 out of 48 measurements, p < 0.001) as compared to supine position (15 out of 48 measurements), and more often in lateral as compared to prone position (19 out of 48 measurements, p = 0.027).
Conclusion. Compared to TLV, OLV increased lung stress. Body position did not affect stress of the ventilated lung during OLV, but lung stability was lowest in semilateral and lateral decubitus position.
One-lung ventilation (OLV) for thoracic surgery leads to pronounced changes in respiratory system mechanics. When the thorax is opened and lung isolation achieved, not only absolute values, but also the distribution of intrathoracic pressure may change importantly. Furthermore, lateral decubitus position, which is commonly used during thoracic surgery, can promote atelectasis formation, and reduce the total lung volume. As a result, both global and regional mechanical stress on lung parenchyma may increase and lead to ventilator-induced lung injury (VILI) (Slutsky and Ranieri, 2013). In fact, the risk of VILI is considered to be higher during OLV than two-lung ventilation (TLV) (Lohser and Slinger, 2015).
Currently, respiratory system mechanics and mechanical power (MP), as well as global regional end-inspiratory and end-expiratory transpulmonary pressure (PL), and driving PL (ΔPL) during OLV are poorly defined. Theoretically, higher end-inspiratory PL might increase stress and overdistend the lungs, whereas negative end-expiratory PL might cause instability with tidal collapse and reopening of lung units. Also, excessive transpulmonary driving pressure (ΔPL) could further increase lung stress and injury (Grieco et al., 2017). To prevent this, VT during OLV should not exceed 6 mL kg−1 predicted body weight (Batchelor et al., 2019).
In this study, we aimed at determining PL and ΔPL in different lung regions, as well as the elastance, resistance and MP of the respiratory system during OLV in anesthetized pigs. We hypothesized that, compared to TLV, OLV increases PL and ΔPL and impairs respiratory system mechanics and MP. Also, we hypothesized that the gravity gradient is higher in OLV and, thus, OLV in lateral position increases stress and instability of the ventilated lung compared to semi-lateral, supine and prone positions.
This study was conducted as a secondary protocol of a previous experiment from our group (Wittenstein et al., 2021), and was approved by the Institutional Animal Care and Welfare Committee and the Government of the State of Saxony, Germany (DD24.1-5131/449/71). In the primary study, we determined the distribution of pulmonary blood flow during commonly used body positions for thoracic surgery during intravascular normo- and hypovolemia (n per group = 8) in pigs undergoing OLV (Wittenstein et al., 2021). All animals received care in compliance with the Principles of Laboratory Animal Care formulated by the National Society for Medical Research and the US National Academy of Sciences Guide for the Care and Use of Laboratory Animals, and complied with relevant aspects of the ARRIVE guidelines. Animals were kept at controlled temperature and light-dark cycle with free access to water and food.
The animal preparations were described in detail elsewhere (Wittenstein et al., 2021). Briefly, intravenous anesthesia and muscle paralysis were induced and maintained with midazolam (1 mg kg-1 h-1), ketamine (15 mg kg-1 h-1), and atracurium (3 mg kg− 1 h-1) in sixteen female pigs (German landrace, weighing 35–49 kg, Danish Specific Pathogen Free Certification (Health Status Management, 2022)). The intravascular volume was maintained with a crystalloid solution at a rate of 5 mL kg-1 h-1. Norepinephrine was used to maintain a mean arterial pressure of at least 60 mmHg throughout the experiments. Lungs were ventilated in volume-controlled mode: fraction of inspired oxygen (FIO2) of 1.0, tidal volume (VT) of 6 mL kg-1, positive end-expiratory pressure (PEEP) of 5 cmH2O, inspiratory: expiratory (I:E) ratio of 1:1, the constant gas flow of 25 L min-1, and respiratory rate (RR) adjusted to achieve arterial pH > 7.3 (Evita XL, Drägerwerk AG & Co. KGaA, Lübeck, Germany).
All skin incisions were preceded by infiltration with 2–5 mL lidocaine 2%. A 20 cm PiCCO catheter was inserted in the right internal carotid artery. A 7.5 Fr. pulmonary artery catheter was advanced through an 8.5 Fr. sheath, placed in the right external jugular vein until typical pulmonary arterial pressure waveforms were observed. Urine was collected with a bladder catheter inserted through a median mini-laparotomy.
Regional pleural pressures (PPl) were measured using three custom-made pressure sensors, calibrated and placed as previously described by our group (Kiss et al., 2019). Briefly, pressure sensors were made from a glued (Silicon-based clue, MBFZ toolcraft GmbH, Georgensgmünd, Germany) double layer of thin latex to build a sealed air-filled chamber (30 × 30 × 3 mm3). A silicon tube (50 cm length, 500 µm inner diameter, 1.2 mm outer diameter) was introduced into the chamber using seldinger technique and connected to the respective pressure transducers (163PC01D48-PCB, FirstSensors AG, Berlin, Germany) via Luer-lock. Before implantation, each sensor was inflated with 0.05 mL of room air and calibrated within an air-sealed chamber using pressures in the range of −50 to 50 cmH2O within 30 s, yielding a pressure rate of 6.7 cmH2O s-1. For calibration, a linear correction was used.
For placement of the sensors, lungs were separated introducing a left-sided double-lumen tube (39 Fr., Silbroncho Fuji, Tokyo, Japan) through a tracheotomy, with the bronchial tip placed into the left main bronchus under fiberoptic control (Ambu aScope 3 and Ambu aView, Ambu GmbH, Bad Nauheim, Germany). Animals were positioned in the right lateral decubitus position, and OLV of the dependent lung (VT = 5 mL kg-1, RR = 35 min-1) was initiated. Using video-assisted thoracoscopy (Thoracoscopy Set, Karl Storz, Tuttlingen, Germany), three pressure sensors were attached to the parietal pleura by staples in the following regions of the left hemithorax: 1) ventral (4–5th rib parasternal); 2) dorsal (4–5th rib paravertebral); and 3) caudal (8–9th rib paravertebral) (Figure 1). A thoracic drain was placed, and surgical wounds were sutured and sealed.
FIGURE 1. Position of the intrapleural pressure sensors. To measure regional pleural pressure, three pressure sensors were custom-made, calibrated, and placed via video-assisted thoracoscopy. The sensors were attached to the parietal pleura by staples in the following regions of the left hemithorax: 1) ventral (4–5th rib parasternal); 2) dorsal (4–5th rib paravertebral); and 3) caudal (8–9th rib paravertebral).
To mimic thoracic surgery conditions, a right-sided thoracotomy was performed between the medial-clavicular and the anterior axillary line in the fourth-fifth intercostal space, and a rib spreader was placed. Furthermore, systemic inflammation was induced by continuous administration of 0.5 μg kg-1 h-1 Lipopolysaccharides (LPS) from Escherichia coli O111:B4 (SIGMA Aldrich, St. Louis, United States) through the central venous line.
According to the protocol of the primary study, animals were randomized to either normo- or the hypovolemia group. For induction of hypovolemia, 25% of the calculated blood volume, estimated as 70 mL kg-1. However, in this substudy all animals were analysed independently from their volume status. Animals were randomly submitted to one of four sequences during OLV according to a Latin square cross-over design: 1) a-b-c-d, 2) b-d-a-c, 3) d-c-b-a, and 4) c-a-d-b (with a = supine; b = left semilateral; c = left lateral; d = prone position; 30 min each). For protective low tidal volume OLV, volume-controlled mode was used (VT = 5 mL kg-1; FIO2 = 1.0, PEEP = 5 cmH2O, I:E = 1:1, RR = 30–35 min-1 to achieve arterial pH > 7.25, and gas flow = 25 L min-1). To reset lung history between the interventions, animals were placed in the supine position and disconnected from the ventilator (20 s), TLV resumed with baseline settings until normalization of gas exchange, and an alveolar recruitment maneuver performed before the start of OLV in each position.
PL and respiratory variables were first collected after completing instrumentation (Baseline). Measurements were then repeated 1 h after starting the LPS infusion (TLVsupine) and 30 min after turning the animal into each body position during OLV (OLVsupine, OLVsemilateral, OLVlateral, and OLVprone, 30 min each).
Signals were recorded for 5 minutes at each time point. Air flow and airway pressure was obtained from internal sensors of the ventilator. An additional airway pressure signal and the three pleural pressure signals were measured using analogue pressure transducers. Pleural pressure signals were synchronized during post-processing with airway pressure, flow and volume using the redundantly measured airway pressure signal. Regional PL in the three regions were calculated by subtracting the respective pleural pressure from airway pressure. Global PL was calculated as mean PL of the three sensors (ventral, dorsal and caudal). End-expiratory PL<2.9 cmH2O was defined as lung instability, as suggested elsewhere (Hedenstierna et al., 1981). In anaesthetized subjects in supine position, end-expiratory PL of the left lung at closing capacity was 2.9 cmH2O.
Respiratory system elastance and resistance were determined using multiple linear regression of the linear equation of motion to airway flow, volume and pressure signals. Respiratory system MP was calculated by deriving mechanical energy (ME) per breath by numerical integration of the tidal pressure-volume-curve (PV curve) using a trapezoidal rule (Huhle et al., 2018).
All animals of the primary study were included in this analysis. Data are presented as mean and standard deviation (SD) if not stated otherwise. The statistical analysis was conducted with SPSS (Version 27, IBM Corp, United States). Significance was accepted at p < 0.05. Differences between TLVsupine and OLVsupine were tested using a t-test for paired samples. Differences between the respective body position during OLV and sequences of interventions were compared using a repeated measures linear mixed-effects model with OLVsupine, OLVsemilateral, OLVlateral, and OLVprone as within-subjects factor and with latin-square sequence as between-subjects factor. Pairwise post hoc multiple comparisons were performed when appropriate and corrected for multiple comparisons according to Šidák. Differences of the binary outcome lung instability, defined as end-expiratory PL < 2.9 cmH2O (Hedenstierna et al., 1981) were tested using the χ2 test and pair-wise binomial post hoc test corrected according to Bonferroni.
Global transpulmonary pressures are depicted in Table 1. Global end-expiratory, end-inspiratory and ΔPL were lower during TLVsupine than during OLVsupine. During OLV, end-expiratory PL was lower in lateral as compared to supine and prone position, while end-inspiratory and ΔPL did not differ among body positions.
Regional end-expiratory PL is depicted in Table 2. During TLVsupine, ventral and dorsal end-expiratory PL were lower than during OLVsupine, while caudal end-expiratory PL was not different.
During OLV, dorsal end-expiratory PL was not different among the body positions during OLV. Ventral end-expiratory PL was higher in OLVsupine as compared to all other positions and higher in OLVsemilateral as compared with OLVlateral and OLVprone. Caudal end-expiratory PL was lower in the semilateral position as compared to OLVlateral and OLVprone. Lung instability was detected more often in semilateral (26 out of 48 measurements; p = 0.012) and lateral (29 out of 48 measurements, p < 0.001) as compared to supine position (15 out of 48 measurements) and more often in lateral as compared to prone position (19 out of 48 measurements, p = 0.027).
Regional end-inspiratory PL is depicted in Table 2. Ventral, dorsal, and caudal end-inspiratory PL were lower during TLVsupine as compared with OLVsupine. During OLV, ventral, dorsal and caudal end-inspiratory PL were not different between the different positions. During TLVsupine, ΔPL was lower in all regions as compared with OLVsupine (Figure 2). During OLV, ΔPL was not different among the body positions.
FIGURE 2. Regional transpulmonary driving pressure. Mean ± SD; ΔPL, transpulmonary driving pressure. Significance was accepted at p < 0.05. BL: Baseline. Differences between TLVsupine and OLVsupine were tested using a t-test for paired samples. Differences between the respective body position during OLV and sequences of interventions were compared using a linear mixed-effects model with repeated measures with OLVsupine, OLVsemilateral, OLVlateral, and OLVprone as within-subjects factor and with the sequence as between-subjects factor. Pairwise post hoc multiple comparisons were performed when appropriate and corrected for multiple comparisons according to Šidák (#p < 0.05).
Respiratory variables are represented in Table 3. Respiratory rate, plateau, and mean airway pressure, as well as elastance and resistance, were lower during TLVsupine compared with OLVsupine, while there were no differences between positions during OLV. Respiratory system MW and MP were lower during TLVsupine as compared with OLVsupine. During OLV, MW and MP were not significantly different between the different body positions.
The main findings of the present study were that, in anesthetized pigs, mean end-inspiratory and end-expiratory PL and ΔPL were higher during OLVsupine than TLVsupine as well as respiratory system elastance, resistance and MP. During OLV, only end-expiratory PL was lower in semilateral and lateral compared to supine and prone position, mainly in ventral and caudal regions.
To our knowledge, this is the first comprehensive investigation on regional PL during TLV and OLV in different body positions. Differently from esophageal manometry, which may overestimate PPl of non-dependent lung zones and underestimates PPl of dependent regions (Pelosi et al., 2001; Pelosi et al., 2011), we measured PPl locally. Thereby, the distribution of PL in ventral, dorsal, and caudal region of the left hemithorax could be determined in presence of a contra-lateral open chest. Of note, mechanical ventilation settings both during TLV as well as OLV followed closely the clinical standard recommended by an expert panel-based consensus (Batchelor et al., 2019; Young et al., 2019).
Our finding that end-expiratory PL increased when switching from TLV to OLV in supine position might be explained by the fact that the pressure difference over the ribcage is reduced during pneumothorax as well as a shift of the mediastinal content towards the side of pneumothorax, reducing intrathoracic pressure. During OLV, ventral and caudal end-expiratory PL differed depending on body position, with the lowest values in lateral and semilateral position, suggesting increased lung instability in this position. This finding is line with previous investigations in healthy volunteers during two lung ventilation (Ferris et al., 1959; Milic-Emili et al., 1964; Washko et al., 2006) and in thoracic surgery patients (Chiumello et al., 2020) using esophageal balloon manometry. Our finding can be explained by the fact that in the lateral position, the ventilated lung is compressed by the weight of the mediastinum, which shifts to the side of ventilation following gravity once the thorax is opened. More pronounced lung compression may increase the risk of end-expiratory lung collapse. As a consequence, potentially higher PEEP values are necessary to keep the lung open in lateral position. However, it is a matter of ongoing debate on which PEEP should be chosen during OLV (Battaglini et al., 2020). In addition to the set PEEP, auto-PEEP can influence end-expiratory PL. In our study however, auto-PEEP did not differ among the investigated body positions.
End-inspiratory PL, as well ΔPL, markedly increased in all regions of the lung during OLV as compared to TLV, theoretically indicating increased lung stress and tidal overdistension. High alveolar stress and overdistension are the driving forces of VILI (Güldner et al., 2016; Bates and Smith, 2018). We used a low VT during OLV, which is considered to be protective. However, even this low VT resulted in high end-inspiratory PL and high ΔPL, independently of the body position, putting the ventilated lung at risk of VILI during OLV. Our results might explain why the risk of postoperative pulmonary complications is increased after thoracic anesthesia (Uhlig et al., 2020).
The fact that, during OLV, MP was almost twice as high as during TLV is in line with the literature and likely explained by increased elastance. In a prospective, observational, single-center study in 30 patients, MP increased in the lateral position with OLV as compared to TLV (Chiumello et al., 2020). Interestingly, MP exceeded 12 J/min during OLV in our animals, a threshold that was associated with formation of lung edema during TLV in pigs (Cressoni et al., 2016). Furthermore, OLV was accompanied by an increase of elastance in our study, which means that the stress exerted on the parenchymal tissue increased, potentially leading to increased distension of the septal walls and VILI (Bates and Smith, 2018). The fact that elastance did not differ significantly among body positions contrasts with results reported during TLV elsewhere (Tanskanen et al., 1997). A likely explanation for this discrepancy is that, in our animals, elastance almost doubled during OLV compared to TLV, possibly masking less pronounced effects of body position. Furthermore, VT adjusted to body weight during left lung ventilation was almost twice when adjusted for the relative size of the ventilated lung (assuming that the left lung accounts for approximately 45% of the total lung size), which may explain the increased elastance, despite the pneumothorax.
The findings of this study suggest that the added risk of VILI during of OLV, as compared to TLV, is explained by increased MP and lung distending pressures. Importantly, this was not amenable by protective low tidal volumes that are commonly used in clinical practice. It is worth noting that lateral and semi-lateral decubitus positon, the most common positions used during thoracic surgery with OLV, led to the highest risk of VILI, since they were accompanied by increased lung instability.
The present study knows several limitations. First, the study was conducted as an exploratory sub-study of another trial and lacked formal sample size estimation. Second, we did not investigate the effects of right lateral and semilateral decubitus positions. A previous study reported lower end-expiratory PPl, as estimated by oesophageal manometry, in the right lateral position compared to the left lateral position during OLV, which was explained by the lower volume of the left lung, thus leading to a smaller decrease in PPl during right decubitus compared to left decubitus position due to less traction of the smaller left lung on the pleural surface (Chiumello et al., 2020). Third, the cross-over design precluded assessment of lung injury. Therefore, we do not know whether lower end-inspiratory PL during OLV in the lateral position increases the risk of VILI. Fourth, the thoracotomy limited the direct comparison of respiratory mechanics between TLV and OLV since the latter did not include assessment of chest wall mechanics. Fourth, the different shape of the rib cage and anatomical distribution of intrathoracic organs in pigs may lead to different amplitudes and distribution of pressures in humans.
In anesthetized pigs, protective low tidal volume OLV, as compared to TLV, increased lung stress and respiratory system MP and impaired respiratory system mechanics. Body position did not affect lung stress, or respiratory system mechanics and MP during OLV, but end-expiratory PL was lowest in semilateral and lateral decubitus position.
The raw data supporting the conclusion of this article will be made available by the authors, without undue reservation.
The animal study was reviewed and approved by Landesdirektion Sachsen, Referat 25, Veterinärwesen und Lebensmittelüberwachung.
JW, MG, and RH planed and designed the study. JW, MS, XY, and RH conducted the experiments. JW, MS, XY, TB, TK, MS, PR, PP, MG, and RH were involved in the analyses of the data. JW and XY wrote the manuscript draft. RH designed, build and validated the custom-made pleural pressure sensors. All authors contributed to the article and approved the submitted version.
This work was supported by departmental sources. This study was funded by institutional funds, a grant by the local Faculty of Medicine Carl Gustav Carus, Dresden (MeDDrive #60485), and a national grant of the German Research Council (Deutsche Forschungsgemeinschaft, DFG; Grant number GA 1256/8-1).
We thank the members of the Pulmonary Engineering Group, University Hospital Carl Gustav Carus Dresden at Technische Universität Dresden, Dresden, for their assistance in conducting the experiments. Finally, we express our gratitude to PP, who recently passed away, for his contribution, his genuine inspiration, and his pioneering work in local pleural pressure measurement in experimental research: “To the next...”!
The authors declare that the research was conducted in the absence of any commercial or financial relationships that could be construed as a potential conflict of interest.
All claims expressed in this article are solely those of the authors and do not necessarily represent those of their affiliated organizations, or those of the publisher, the editors and the reviewers. Any product that may be evaluated in this article, or claim that may be made by its manufacturer, is not guaranteed or endorsed by the publisher.
ANOVA, analysis of variance; FIO2, inspired fraction of oxygen; I:E, inspiratory to expiratory time ratio; LPS, Lipopolysaccharide; MP, mechanical power; MW, mechanical work; OLV, one-lung ventilation; PEEP, positive end-expiratory pressure; PL, transpulmonary pressure; PPl, pleural pressure; ΔPL, driving transpulmonary pressure; RR, respiratory rate; SD, standard deviation; TLV, two-lung ventilation; VILI, ventilator induced lung injury; VT, tidal volume.
Batchelor, T. J. P., Rasburn, N. J., Abdelnour-Berchtold, E., Brunelli, A., Cerfolio, R. J., Gonzalez, M., et al. (2019). Guidelines for enhanced recovery after lung surgery: Recommendations of the enhanced recovery after surgery (ERAS®) society and the European society of thoracic surgeons (ESTS). Eur. J. Cardio-Thorac Surg. Off. J. Eur. Assoc. Cardio-Thorac Surg. 55 (1), 91–115. doi:10.1093/ejcts/ezy301
Bates, J. H. T., and Smith, B. J. (2018). Ventilator-induced lung injury and lung mechanics. Ann. Transl. Med. 6 (19), 378. doi:10.21037/atm.2018.06.29
Battaglini, D., Ball, L., Wittenstein, J., Cohen, E., Gama de Abreu, M., and Pelosi, P. (2020). PEEP in thoracic anesthesia: PROS and CONS. Minerva Anestesiol. 87, 223–229. doi:10.23736/S0375-9393.20.14797-7
Chiumello, D., Formenti, P., Bolgiaghi, L., Mistraletti, G., Gotti, M., Vetrone, F., et al. (2020). Body position alters mechanical power and respiratory mechanics during thoracic surgery. Anesth. Analg. 130 (2), 391–401. doi:10.1213/ANE.0000000000004192
Cressoni, M., Gotti, M., Chiurazzi, C., Massari, D., Algieri, I., Amini, M., et al. (2016). Mechanical power and development of ventilator-induced lung injury. Anesthesiology 124 (5), 1100–1108. doi:10.1097/ALN.0000000000001056
Ferris, B. G., Mead, J., and Frank, N. R. (1959). Effect of body position on esophageal pressure and measurement of pulmonary compliance. J. Appl. Physiol. 14 (4), 521–524. doi:10.1152/jappl.1959.14.4.521
Grieco, D. L., Chen, L., and Brochard, L. (2017). Transpulmonary pressure: Importance and limits. Ann. Transl. Med. 5 (14), 285. doi:10.21037/atm.2017.07.22
Güldner, A., Braune, A., Ball, L., Silva, P. L., Samary, C., Insorsi, A., et al. (2016). Comparative effects of volutrauma and atelectrauma on lung inflammation in experimental acute respiratory distress syndrome. Crit. Care Med. 44 (9), e854–e865. doi:10.1097/CCM.0000000000001721
Health Status Management (2022). RaaTec AB approved producer of heat treated sphagnum. Home [Internet] Available at: https://spfsus.dk/en (Accessed November 28, 2022).
Hedenstierna, G., Bindslev, L., and Santesson, J. (1981). Pressure-volume and airway closure relationships in each lung in anaesthetized man. Clin. Physiol. Oxf Engl. 1 (5), 479–493. doi:10.1111/j.1475-097x.1981.tb00915.x
Huhle, R., Serpa Neto, A., Schultz, M. J., and Gama de Abreu, M. (2018). Is mechanical power the final word on ventilator-induced lung injury?-no. Ann. Transl. Med. 6 (19), 394. doi:10.21037/atm.2018.09.65
Kiss, T., Bluth, T., Braune, A., Huhle, R., Denz, A., Herzog, M., et al. (2019). Effects of positive end-expiratory pressure and spontaneous breathing activity on regional lung inflammation in experimental acute respiratory distress syndrome. Crit. Care Med. 47 (4), e358–e365. doi:10.1097/CCM.0000000000003649
Lohser, J., and Slinger, P. (2015). Lung injury after one-lung ventilation: A review of the pathophysiologic mechanisms affecting the ventilated and the collapsed lung. Anesth. Analg. 121 (2), 302–318. doi:10.1213/ANE.0000000000000808
Milic-Emili, J., Mead, J., and Turner, J. M. (1964). Topography of esophageal pressure as a function of posture in man. J. Appl. Physiol. 19, 212–216. doi:10.1152/jappl.1964.19.2.212
Pelosi, P., Goldner, M., McKibben, A., Adams, A., Eccher, G., Caironi, P., et al. (2001). Recruitment and derecruitment during acute respiratory failure: An experimental study. Am. J. Respir. Crit. Care Med. 164 (1), 122–130. doi:10.1164/ajrccm.164.1.2007010
Pelosi, P., Luecke, T., and Rocco, P. R. M. (2011). Chest wall mechanics and abdominal pressure during general anaesthesia in normal and obese individuals and in acute lung injury. Curr. Opin. Crit. Care 17 (1), 72–79. doi:10.1097/MCC.0b013e3283427213
Slutsky, A. S., and Ranieri, V. M. (2013). Ventilator-induced lung injury. N. Engl. J. Med. 369 (22), 2126–2136. doi:10.1056/nejmra1208707
Tanskanen, P., Kyttä, J., and Randell, T. (1997). The effect of patient positioning on dynamic lung compliance. Acta Anaesthesiol. Scand. 41 (5), 602–606. doi:10.1111/j.1399-6576.1997.tb04750.x
Uhlig, C., Neto, A. S., van der Woude, M., Kiss, T., Wittenstein, J., Shelley, B., et al. (2020). Intraoperative mechanical ventilation practice in thoracic surgery patients and its association with postoperative pulmonary complications: Results of a multicenter prospective observational study. BMC Anesthesiol. 20 (1), 179. doi:10.1186/s12871-020-01098-4
Washko, G. R., O’Donnell, C. R., and Loring, S. H. (2006). Volume-related and volume-independent effects of posture on esophageal and transpulmonary pressures in healthy subjects. J. Appl. Physiol. 100(3), 753–758. doi:10.1152/japplphysiol.00697.2005
Wittenstein, J., Scharffenberg, M., Ran, X., Zhang, Y., Keller, D., Tauer, S., et al. (2021). Effects of body position and hypovolemia on the regional distribution of pulmonary perfusion during one-lung ventilation in endotoxemic pigs. Front. Physiol. 12, 717269. doi:10.3389/fphys.2021.717269
Keywords: OLV, VILI, thoracic surgery, local transpulmonary pressure, local pleural pressure, mechanical power, open pneumothorax
Citation: Wittenstein J, Scharffenberg M, Yang X, Bluth T, Kiss T, Schultz MJ, Rocco PRM, Pelosi P, Gama de Abreu M and Huhle R (2023) Distribution of transpulmonary pressure during one-lung ventilation in pigs at different body positions. Front. Physiol. 14:1204531. doi: 10.3389/fphys.2023.1204531
Received: 12 April 2023; Accepted: 13 July 2023;
Published: 04 August 2023.
Edited by:
Gaetano Perchiazzi, Uppsala University, SwedenReviewed by:
Lucia Mirabella, University of Foggia, ItalyCopyright © 2023 Wittenstein, Scharffenberg, Yang, Bluth, Kiss, Schultz, Rocco, Pelosi, Gama de Abreu and Huhle. This is an open-access article distributed under the terms of the Creative Commons Attribution License (CC BY). The use, distribution or reproduction in other forums is permitted, provided the original author(s) and the copyright owner(s) are credited and that the original publication in this journal is cited, in accordance with accepted academic practice. No use, distribution or reproduction is permitted which does not comply with these terms.
*Correspondence: Marcelo Gama de Abreu, Z2FtYWRlbUBjY2Yub3Jn
Disclaimer: All claims expressed in this article are solely those of the authors and do not necessarily represent those of their affiliated organizations, or those of the publisher, the editors and the reviewers. Any product that may be evaluated in this article or claim that may be made by its manufacturer is not guaranteed or endorsed by the publisher.
Research integrity at Frontiers
Learn more about the work of our research integrity team to safeguard the quality of each article we publish.