- 1Laboratoire de Biomécanique et Physiologie et la Locomotion, Institute of Neuroscience, Louvain-la-Neuve, Belgium
- 2Exercise Science Laboratory, School of Kinesiology, Faculty of Medicine, Universidad Finis Terrae, Santiago, Chile
With extended life expectancy, the quality of life of elders is a priority. Loss of mobility, increased morbidity and risks of falls have dramatic individual and societal impacts. Here we consider the age-related modifications of gait, from a biomechanical and neurophysiological perspective. Among the many factors of frailty involved (e.g., metabolic, hormonal, immunological), loss of muscle strength and neurodegenerative changes inducing slower muscle contraction may play a key role. We highlight that the impact of the multifactorial age-related changes in the neuromuscular systems results in common features of gait in the immature gait of infants and older adults. Besides, we also consider the reversibility of age-related neuromuscular deterioration by, on the one hand, exercise training, and the other hand, novel techniques such as direct spinal stimulation (tsDCS).
1 Introduction
With extended life expectancy, the quality of life of elders is a priority. Loss of mobility, increased morbidity and risks of falls have dramatic individual and societal impacts. Among the many factors of frailty involved, loss of muscle mass and strength (Akima et al., 2001) and neurodegenerative changes (Rygiel et al., 2016) play a key role. Whether changes in the neural control precede or follow the decline of muscle mass and strength and how they both are related to gait alteration remains yet to be established. More than ever, this needs to be elucidated to implement interventions that can maintain or improve neuromuscular function in older adults.
Biomechanical changes with age have garnered considerable scientific attention for nearly 50 years. The scientific community (Winter et al., 1990; Delabastita et al., 2021) most often points to a reduction in mechanical power generated by the plantar flexor muscles during the push-off phase of walking as the hallmark biomechanical ageing features of gait. However, the 11%–35% decline in force or power-generating capacity of propulsive leg muscles cannot fully explain the age-related modification of gait in older adults. Indeed, (i) many old adults underutilize their available muscular capacity for generating propulsive power in walking and are able to increase it during slope walking or using biofeedbacks (Waanders et al., 2021), and (ii) age-related changes in kinematics have been found prior to the appearance of propulsion decline with increasing age (Sloot et al., 2021). Taken together, it suggests that the decline of propulsive power generation is thus not only due to a reduced muscular capacity, but neural factors are likely to contribute as well.
For instance, with aging motor weakness is due in part to neuromuscular degeneration, but also to degenerative changes in the central nervous system. Thus, reduction in grey matter volume (Good et al., 2001), number of motor cortical (Henderson et al., 1980) and spinal motor neurons (Doherty, 2003), synaptic density (Haug and Eggers, 1991), white matter integrity (Davis et al., 2009), and descending commands for motor activation (Yue et al., 1999) are some of the factors that may contribute to age-related motor impairment. Another determinant of functional capacity and autonomy is the integrity of other components of the neuromuscular system, which wires the brain and skeletal muscles via motor neurons and the neuromuscular junction. However, despite its obvious importance for rhythm generation, the potential involvement of the spinal cord in age-related modification of locomotion has received little attention.
Using an electrophysiological approach, a way to get insight into spinal cord functioning is to look at the spatiotemporal organization of the total locomotor output by mapping multi-muscles EMG onto the spinal cord in approximate rostro-caudal locations of the motoneuron (MN) pools (Ivanenko et al., 2008; Cappellini et al., 2010; Ivanenko et al., 2013; La Scaleia et al., 2014; Yokoyama et al., 2017; Dewolf et al., 2019a). By studying the spinal motor output across various walking conditions in older adults (walking at different speeds, backward, upslope, downslope, upstairs, downstairs), similar age-related differences in muscle activations have been observed despite the various biomechanical constraints (Dewolf et al., 2021a; Dewolf et al., 2021b). In particular, the activity profiles of the muscles innervated from the sacral segments were significantly wider in older adults in all conditions. Interestingly, similar modification has been observed in young children (Ivanenko et al., 2013; Dewolf et al., 2020b).
The major consideration of this review is the age-related remodeling of both the neural and muscular system and its relationship with locomotion changes with age, to shed light on the multifactorial age-related changes of gait. The alterations of the gait pattern in older adults are then compared to immature gait. Besides, we also consider the reversibility of age-related neuromuscular deterioration by, on the one hand, exercise training, and the other hand, novel techniques such as direct spinal stimulation (tsDCS) to mitigate the reduction of intrinsic spinal motoneuron excitability in older adults (Orssatto et al., 2021a), and how it could potentially lead to improved strategies for promoting locomotor function recovery.
2 Neuromuscular modification with aging
Aging is a natural and gradual process where the alterations in motor control and physical fitness are multifactorial (Borzuola et al., 2020). Strength capacity and muscle mass decrease during aging, in great part due to sarcopenia. Aging-related sarcopenia is the most common type of atrophy in humans. Specifically, sarcopenia is a progressive skeletal muscle disorder identified by low muscle strength, low muscle quantity or quality, and low physical performance (Cruz-Jentoft et al., 2019). It is associated with an increased risk of adverse outcomes, such as functional disability, poor quality of life, and a higher risk of mortality (Batsis et al., 2014; Beaudart et al., 2017; Kelley and Kelley, 2017; Liu et al., 2017). In older adults, the loss of strength seriously affects independence associated with activities of daily living but also leads to a greater risk of falls, which is strongly related to mortality (Suzuki et al., 1992; Landi et al., 2012). From a clinical perspective, it is essential to understand the mechanisms underlying the modifications in skeletal muscle morphology and function, which are evident during aging (Fiatarone et al., 1990). Muscle strength begins to decline after 30 years of age and continues to decline with advancing age (Gava et al., 2015). Changes related to muscle morphology and its electrophysiology generally appear after the age of ∼40, and it also continues to decrease progressively (Stålberg and Fawcett, 1982; Oertel, 1986; Murton, 2015). Therefore, changes in strength appear to precede changes associated with skeletal muscle morphology. Also, the effect of aging on skeletal muscles depends on muscle location and function, since leg muscles are more affected than arm muscles (Stålberg and Fawcett, 1982; Oertel, 1986).
Several age-related modifications of muscle tissue have been described, such as loss of muscle fibers (Lexell et al., 1983; Lexell, 1995; McPhee et al., 2018) or substantial loss of contractile proteins (Larsson et al., 1996), such as myosin heavy chain (Siparsky et al., 2014). Not only the reduction in the total number of fibres occurs, but also in their cross-sectional area (Lexell and Taylor, 1991). It should be noted that a differential response is reported in fibre loss depending on the type of muscle, with a faster decrease mainly observed in type II fibres (Cade and Yarasheski, 2006; Domingues-Faria et al., 2016). Also, a change from the fast myosin isoform to the slow isoform has also been observed, which has a lower capacity to generate force. This change in fibre type could contribute to both slowing movement and decreased maximal strength, and in turn induce age-related changes of gait.
Even the pathogenesis of sarcopenia is not yet fully understood, multiple etiological factors seem to be involved, including alteration of muscle proteostasis (Lecker et al., 2004), mitochondrial dysfunction and mitochondrial DNA deletions (Bua et al., 2006), deregulation of satellite cells (Shefer et al., 2006), and accumulation of extracellular matrix called fibrosis and fat infiltration into skeletal muscle (Song et al., 2004). The cause of sarcopenia cannot be solely attributed to alterations in skeletal muscles. In fact, the nerve responsible for stimulating muscle fibers plays a significant role in sarcopenia. As the skeletal muscles experience degeneration with age, the decline in neuromuscular function emerges as a crucial contributing factor (Aagaard et al., 2010). Evidence has shown alterations associated with a reduction in motor units. Studies have specifically compared the amount of motor neurons in young and elderly subjects, with the latter showing a 50% decrease (Doherty et al., 1993). In addition, the motor neurons begin to exhibit alterations in firing frequency and rate. The maximum firing frequency of motor neurons is lower compared to young subjects (Klass et al., 2008). Other changes have also been detected during aging related to a decrease in axonal conduction velocity, which is explained by reduced myelination and internodal length (Scaglioni et al., 2002). Therefore, evidence suggests that the compromised nervous system function may also be one of the important contributors to functional decline described in sarcopenia (Rygiel et al., 2016; Kwon and Yoon, 2017). Indeed, normal innervation and its corresponding regular activation are necessary to maintain muscle mass through muscle contraction. For example, there is an association between the loss of muscle fibres and the loss of motor units in older people (McNeil et al., 2005; Piasecki et al., 2016; Piasecki et al., 2018). In addition, slow motor neurons may be more adapted to reinnervation, leading to the loss of fast motor neurons with age. This could respond to the change in fibre type that occurs with aging (Larsson et al., 1978; Kadhiresan et al., 1996; Andersen, 2003).
While the causes of the age-associated loss of motor neurons are still unsettled, the neuromuscular junction integrity, and in particular the mitochondrial dysfunction at the neuromuscular junction, may have an important role (Shigemoto et al., 2010). The changes in the neuromuscular junction have been reported to be related to morphological alterations of the pre- and post-synaptic regions and to the reduction of synaptic vesicles (Jang and Van Remmen, 2011). The loss of motoneurons also plays an important role in the alterations of the excitation-contraction coupling process during aging (Payne and Delbono, 2004). Indeed, the decrease in isometric strength and contraction velocity appears before the reduction in muscle mass. Therefore, it has been proposed that the decrease in the number of motor units occurs before the loss of muscle function (Deschenes et al., 2010; Sheth et al., 2018). However, there is still insufficient evidence and more studies are required to complement the current hypothesis.
3 Gait during development and aging: a brief overview of the two sides of life
The multifactorial age-related changes in the neuromuscular system, summarized in the last section, are rather well documented. As people age, those changes result in alterations in gait patterns. In this section, we present different aspect of gait (presented in Figure 1) that are affected by age, and that interestingly resemble those seen in younger infants.
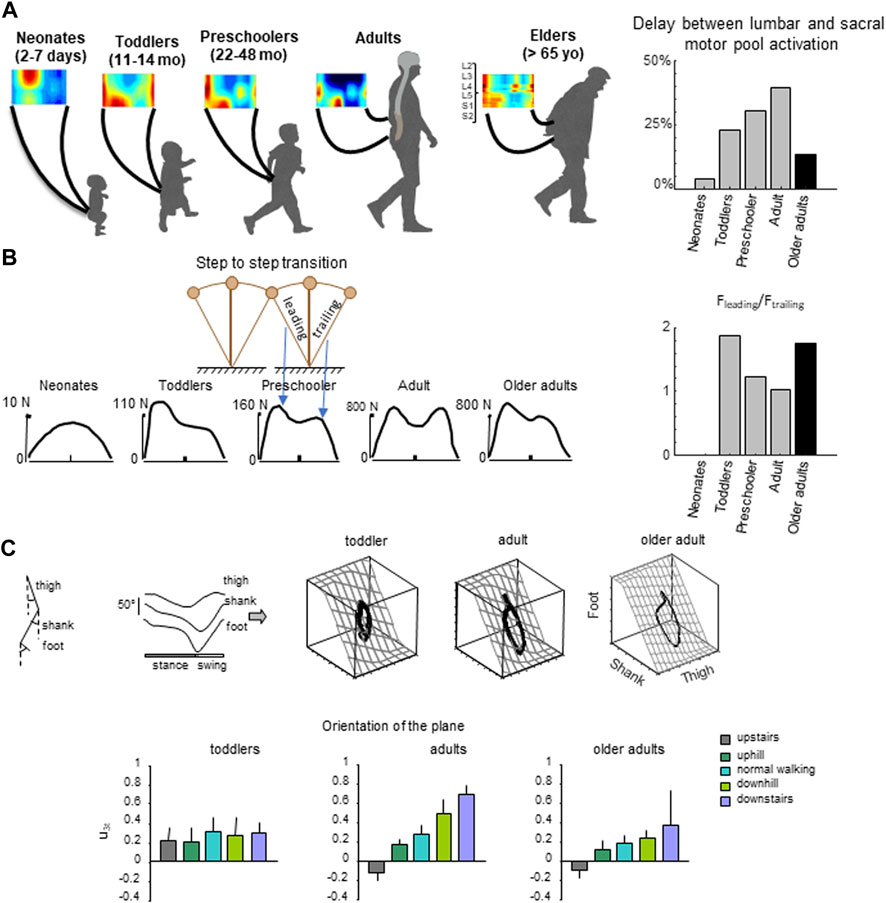
FIGURE 1. General features of gait in older adults. (A) spatiotemporal maps of motoneuron activity of the lumbosacral enlargement in neonates, toddlers, preschoolers, adults and older adults, and the delay between the activation of lumbar and sacral activation (data from Ivanenko et al. (2013) for neonates, toddlers, preschoolers and adults, and from Dewolf et al. (2021a) for older adults). (B) Schematic representation of walking like an inverted-pendulum. Below, representative vertical loading force during stepping in neonates, toddlers, preschoolers, adults and in older adults. The characteristic force profile was evaluated using the ratio between the leading limb and the tailing limb. (C) Intersegmental coordination assessed by principal component analysis (PCA) of limb segment elevation angles during walking. From left to right: thigh, shank, and foot elevation angles (relative to the vertical), corresponding 3D trajectory in segment angle space along with the interpolated plane (modified from Ivanenko et al. (2008)). Three examples of gait loops are presented (one toddler, one adult and one older adults). Below, changes in the orientation of the covariance plane during walking over different surfaces in toddlers, adults and older adults (modified from Dominici et al. (2010) and from Dewolf et al. (2021b)).
In humans, when EMG activity patterns are mapped onto the spinal cord in approximate rostrocaudal locations of the motoneuron (MN) pools, the activation of MNs tends to occur in bursts that can be associated with the major kinetic events of the gait cycle (Ivanenko et al., 2008; Cappellini et al., 2010; La Scaleia et al., 2014; Yokoyama et al., 2017; Dewolf et al., 2019a). In particular, the first burst occurs around foot contact and is mainly localized on the lumbar segment whereas the second burst occurs during the second part of the stance mainly and is localized on the sacral segment (Figure 1A). This approach provides information about pattern output in terms of lumbosacral segmental control (from L2 to S2) rather than in terms of individual muscle control.
In older adults, across different forms of walking gait, age-related differences were observed (Monaco et al., 2010; Santuz et al., 2020; Dewolf et al., 2021a; Dewolf et al., 2021b), suggesting specific adjustments of the pattern generation circuitries. In particular, the sacral output was significantly wider in older adults and occurred earlier in the stance (Figure 1A). Interestingly, this result does not simply reflect the documented distal-to-proximal modification of kinetics since the human spinal topography does not reflect the muscle topography on the lower limbs (Kendall, 2005). Instead, this potentially highlights a distal to proximal degeneration of the motor system. Accordingly, when the spinal excitability is estimated using the Hoffmann reflex technique, no difference is found between young and older adults on vastus medialis muscle (Mau-Moeller et al., 2013), whereas age-related modulations of the reflex response have been reported in soleus muscle (Baudry et al., 2015). Interestingly, while the craniocaudal gradient of corticospinal development in infancy is well established (Payne and Isaacs, 2017), less is known about the differential degeneration of different portions of the corticospinal tract with aging.
Development and aging can be seen as two opposite but complementary phenomena (Feltes et al., 2015). For example, it appears that projection tracts, such as the corticospinal tract, which develop earlier than association tracts in infancy, degenerate later than association tracts in older subjects. Also, primitive reflexes, which are commonly present in normal infants and disappear during development, reappear in patients with diseases of the nervous system but also in healthy older adults with an incidence increasing with age (Gossman and Jacobs, 1980; Jacobs and Gossman, 1980; Damasceno et al., 2005; van Boxtel et al., 2006; Hobo et al., 2014). Indeed, attempts to elicit primitive reflexes are a routine part of the standard neurological examination in the elderly, with the following reflexes tested: e.g., snout, suck, palmomenral, and hand grasp (described in detail by Koller (Koller, 1984)). Another reflex observed at the beginning of life is the stepping reflex: human newborns step on the ground if supported (Thelen and Fisher, 1982; Forssberg, 1985; Yang et al., 1998; Dominici et al., 2011; Dewolf et al., 2020b; Dewolf et al., 2020a), and stepping generally disappears a few weeks after birth unless trained. The relationship between this reflex and mature walking gait has been argued (Andre-Thomas and Autgaerden, 1966; Dominici et al., 2011; Sylos-Labini et al., 2022), with lower ow complexity and higher variability of neuromuscular signals in neonates. Because of the less complex and more variable control of muscle in older adults (Allen and Franz, 2018), and based on the common features of gait between infants and older adult presented in the present section (Figure 1), One may speculate about the potential greater similarities between neonatal stepping and older adult’s gait pattern.
Also from a kinematic point of view, a simpler coordination pattern among the lower limb segments can be observed both during childhood and agedness (Ivanenko et al., 2004; Noble and Prentice, 2008; Dominici et al., 2010; Bleyenheuft and Detrembleur, 2012; Dewolf et al., 2019b; Gueugnon et al., 2019). One way to unravel the multi-segmental coordinative law is the so-called coplanar variation (Borghese et al., 1996; Bianchi et al., 1998b). During walking, each lower-limb segment oscillates back and forth relative to the vertical with a similar waveform, time-shifted across different segments (Figure 1C). The lower limb segment angles do not evolve independently of each other, but they are tightly coupled: when plotted one vs. the others, they co-vary along a plane, describing a characteristic loop over each stride (Figure 1C). The specific shape and orientation of the plane reflects the phase relationship between segments and therefore the timing of the intersegmental coordination (Barliya et al., 2009). Even if the intersegmental coordination in toddlers rapidly evolves toward the adult shape with experience (Cheron et al., 2001; Ivanenko et al., 2004; Dominici et al., 2010), when toddlers step in various conditions (slope, stairs, backward), they do not adapt their segmental coordination as adults do. Instead, they keep constant phase relationships (Dominici et al., 2010) (Figure 1C). In older adults, the modification of plane orientation across gait conditions is less adapted than in young adults (Dewolf et al., 2019b; Dewolf et al., 2021a; Dewolf et al., 2021b). Since the changes in planar covariation are thought to reflect the ability to adapt to different gait conditions (Bianchi et al., 1998b; Martino et al., 2014; Dewolf et al., 2018), the lack of changes observed in toddlers and to a lesser extent in older adults suggest reduced ability to adapt gait to environment or specific constraits (Dominici et al., 2010).
Because a link between center of mass (COM) trajectory and functional spinal cord topography has been previously highlighted (Cappellini et al., 2010; Dewolf et al., 2019a; Dewolf et al., 2020b), one may expect comparable COM dynamics in older adults and young infants. Center of mass (COM) mechanics is a fundamental concept in biomechanics that describes the movement and balance of an individual’s body. In young adults, during walking the COM vaults over a relatively stiff limb with the heel well in front of the hip at the beginning of the stance, and the heel lift with maintained toe contact at the end of the stance. One of the direct consequences of such a heel-to-toe roll-over pattern is that the extension of distal joints is delayed relative to proximal joints, leading to the typical double hump shape (so-called ≪m − pattern≫) of the vertical ground reaction force (Hallemans et al., 2006) (Figure 1A). In both older adults and younger infants, the walking gait lacks the specific m-pattern shape of adult heel-to-toe roll-over walking pattern (Forssberg, 1985; Dominici et al., 2011; Sylos-Labini et al., 2017), due to the lack of late push-off from the trailing leg (Dominici et al., 2011, 201; Dewolf et al., 2019b; Gueugnon et al., 2019). Another similarity is that both young infants and older adults have limited control over their COM (Foster et al., 2019; Malloggi et al., 2019). In the next section, we discussed the potential cause of modification of gait in older adults, resulting in kinematics, kinetics and neural similarities with the gait observed in children.
4 Could we counteract the age-related modification of gait?
Based on the well-documented change in muscle strength with aging described in the last section, a lot of efforts have been made to counteract it using exercise (Christie, 2011; Hortobágyi et al., 2015; Keating et al., 2021; Wu et al., 2021). Physical training is reported as an effective treatment for maintaining muscular function (Kraemer et al., 2002; Chen et al., 2021; el Hadouchi et al., 2022; Markov et al., 2022). Guidelines recommend high physical activity levels to increase health benefits in older adults (Boyer et al., 2012; Taylor, 2014), since it is supposed to enhance daily activities like gait. Resistance or power training not only increases/maintains muscle mass, strength, power and functional capacity in older adults, but it also induces several neuromuscular adaptations, such as an increase in peak firing frequencies of motoneurons. Also, older adults still practicing long-distance running reduce the decline in muscle function with age by enhancing the neural drive to the muscle (Cogliati et al., 2020).
Resistance training (RT) positively affects walking speed (Keating et al., 2021). For example, Hortobágyi et al. (2015) found that RT significantly increases the habitual gait speed of healthy old adults by 8.4% as a long-term effect. Power training also impacts gait velocity (Beijersbergen et al., 2017a; Beijersbergen et al., 2017b; Beijersbergen et al., 2017c), changing the rate of force development, which is moderately correlated with gait speed (Stock et al., 2019), and improving the functional performance (Radaelli et al., 2023). The main related effect of muscular training is a higher ‘habitual walking speed’ after the exercise sessions. However, training fails to directly translate to improved propulsive power generation in walking (Beijersbergen et al., 2013). For example, a higher level of physical activity in older adults did not mitigate the age-related modification of kinematic coordination and distal-to-proximal redistribution (Boyer et al., 2012). Also, greater muscular power (more than muscle strength) has been reported to have a strong influence on mobility (Bean et al., 2003), but without a clear change in gait pattern. Indeed, the biomechanical, physiological, and motor control adaptations in gait with training are still unknown, and physical trainers or physiotherapists lack consistent biomechanical data to understand the adaptation mechanism (Beijersbergen et al., 2017c). Based on lower limb coordination, Bianchi et al. (Bianchi et al., 1998a) showed that trained young subjects can exploit better the dynamic coupling between segments to save mechanical energy than untrained young subjects. It is, therefore, plausible that training in older adults may affect the lower limb intersegmental coordination to allow optimised gait mechanics.
While walking speed has been suggested to predict frailty and disability in older adults (Guralnik et al., 2000), we believe that evaluation of spontaneous walking speed is not the best outcome to evaluate the age-related decline of gait. Spontaneous gait speed, if not performed after period of familiarization sessions and following standardized instructions, may vary with the mood, motivation, stimuli of the experimenters, etc. For example, in a classical paper, Bornstein and Borstein (Bornstein and Bornstein, 1976) showed that the ‘pace of life’, measured as the spontaneous speed, varies with the size of the local population, regardless of the cultural setting, suggesting that immediate social and physical environment exert strong control over individual habitual speed (Levine and Norenzayan, 1999). Therefore, we believe that there’s an imperative need to understand the role of physical exercise in the process of age-related modification of neuromuscular control of gait. In particular, the data to interpret the mechanism needs to be more quantitative.
Enhancing physical capacity alone may not be sufficient to mitigate the age-related decline of the neuro-muscular system, such as the distal to proximal degeneration of the motor system highlighted above. A recent rehabilitation approach is the use of real-time biofeedback to encourage favorable biomechanical adaptations. For example, it has been showed that the propulsive power can be increased during walking in older adults using ankle power biofeedback (Browne and Franz, 2019), resulting in a reduction of distal-to-proximal redistribution of joint efforts. Based on the age-related modification of gait highlighted in Figure 1, one may expect that other parameters, such as the center of mass trajectory, can be manipulated using real-time biofeedbacks (e.g., as in Massaad et al., 2007). In particular, using real-time biofeedback to cue an acute change in the peak of vertical ground reaction force or in the limb loading may be an effective gait training intervention to mitigate the effect of age in older adults. Such approach has been developed in gait retraining following anterior cruciate ligament reconstruction (Luc-Harkey et al., 2018; Armitano-Lago et al., 2020) but not, to the best of our knowledge, with older adults.
Also, not only muscles but also the firing characteristics of our spinal motoneurons play a critical role in producing force, and so, performing daily activities. Motoneuron firing is determined by complex factors, such as ionotropic synaptic input and persistent inward currents (PICs) (Orssatto et al., 2021b). PICs are depolarizing currents generated by voltage-sensitive sodium and calcium channels. Hassan et al. (Hassan et al., 2021) (2021) found weaker estimates of PICs in older adults than in their younger counterparts, and propose that this weakening is an underlying mechanism for the slowing of motoneuron firing with ageing. Interestingly, the similarities observed between infants’ and older adults’ locomotor patterns (Figure 1) might be related to the slower and weaker firing characteristics (Dayanidhi et al., 2013).
As described by Hassan et al. (Hassan et al., 2021), the PICs weakening might result from a multitude of factors: (1) deterioration within the monoaminergic systems, (2) imbalance between excitatory and inhibitory synaptic inputs, or (3) changes in the function of monoaminergic receptors or voltage-gated channels. The question that need to be answered is now: how can we counteract the age-related decline in PICs? Our proposed answer for a future research question in this context is spinal neuromodulation, a promising strategy to augment spinal cord activity. In particular, non-invasive trans-spinal cord direct current stimulation (tsDCS) may improve spinal motor circuit function and motor output (Jankowska, 2017; Song and Martin, 2017) in older adults, because of the increase in firing frequencies of motoneuron (Bączyk et al., 2019) its specific effect of augmenting PIC-like responses induced by c-tsDCS, L-type Ca2C channel activation (Song and Martin, 2022).
The tsDCS has been increasingly used over recent years in the rehabilitation of patients following neurological injuries (Levins and Moritz, 2017; Gad et al., 2021; Taylor et al., 2021) or as an addition to physical training in sports (Berry et al., 2017). The effect of tsDCS on the gait patterns of older adults has not been studied yet. However, enhancing the PIC-like response of motor units would be well-suited to mitigate the effect of aging on spinal motor output. We hope that the ideas presented here help to motivate future efforts in understanding the quantitative modification of gait with aging and in evaluating a promising method that could be used as a supplementary tool in the management of geriatric patients.
5 Concluding remarks
This review outlines great similarities between the ‘first steps’ of infants and the ‘last steps’ of older adults. While part of the modifications observed in older adults may emerge from a lack of propulsive power, other neurodegenerative changes play a key role. In particular, slower muscle contraction is observed, resulting from the change in fiber type, the greater reinnervation of slow motor neurons or the lower motoneuron firing frequency with ageing, which is also an important peripheral contributor to the lack of adult-like locomotor patterns in early infancy (Dewolf et al., 2020b). Gaining insights into the age-related changes in human gaits may provide important clinical implications. For instance, we propose a novel intervention to enhance the PIC-like response of the motor unit, and in turn, mitigate the effect of aging.
Author contributions
All authors listed have made a substantial, direct, and intellectual contribution to the work and approved it for publication.
Funding
This research was supported by the Fonds National de la Recherche Scientifique (CDR 40013847), the FSR-UCLouvain and by Wallonie-Bruxelles International.
Conflict of interest
The authors declare that the research was conducted in the absence of any commercial or financial relationships that could be construed as a potential conflict of interest.
The handling editor LP-T declared a past co-authorship with the author AD.
Publisher’s note
All claims expressed in this article are solely those of the authors and do not necessarily represent those of their affiliated organizations, or those of the publisher, the editors and the reviewers. Any product that may be evaluated in this article, or claim that may be made by its manufacturer, is not guaranteed or endorsed by the publisher.
References
Aagaard, P., Suetta, C., Caserotti, P., Magnusson, S. P., and Kjaer, M. (2010). Role of the nervous system in sarcopenia and muscle atrophy with aging: Strength training as a countermeasure. Scand. J. Med. Sci. Sports 20, 49–64. doi:10.1111/j.1600-0838.2009.01084.x
Akima, H., Kano, Y., Enomoto, Y., Ishizu, M., Okada, M., Oishi, Y., et al. (2001). Muscle function in 164 men and women aged 20-84 yr. Med. Sci. Sports Exerc. 33, 220–226. doi:10.1097/00005768-200102000-00008
Allen, J. L., and Franz, J. R. (2018). The motor repertoire of older adult fallers may constrain their response to balance perturbations. J. Neurophysiol. 120, 2368–2378. doi:10.1152/jn.00302.2018
Andersen, J. L. (2003). Muscle fibre type adaptation in the elderly human muscle. Scand. J. Med. Sci. Sports 13, 40–47. doi:10.1034/j.1600-0838.2003.00299.x
Andre-Thomas, Y., and Autgaerden, S. (1966). Locomotion from pre-to post-natal life. Lavenham, Suffolk, England: Spastics Society Medical Education and Information Unit and William Heinemann Medical Books.
Armitano-Lago, C., Pietrosimone, B., Davis-Wilson, H. C., Evans-Pickett, A., Franz, J. R., Blackburn, T., et al. (2020). Biofeedback augmenting lower limb loading alters the underlying temporal structure of gait following anterior cruciate ligament reconstruction. Hum. Mov. Sci. 73, 102685. doi:10.1016/j.humov.2020.102685
Bączyk, M., Drzymała-Celichowska, H., Mrówczyński, W., and Krutki, P. (2019). Motoneuron firing properties are modified by trans-spinal direct current stimulation in rats. J. Appl. Physiology 126, 1232–1241. doi:10.1152/japplphysiol.00803.2018
Barliya, A., Omlor, L., Giese, M. A., and Flash, T. (2009). An analytical formulation of the law of intersegmental coordination during human locomotion. Exp. Brain Res. 193, 371–385. doi:10.1007/s00221-008-1633-0
Batsis, J. A., Mackenzie, T. A., Barre, L. K., Lopez-Jimenez, F., and Bartels, S. J. (2014). Sarcopenia, sarcopenic obesity and mortality in older adults: Results from the national health and nutrition examination survey III. Eur. J. Clin. Nutr. 68, 1001–1007. doi:10.1038/ejcn.2014.117
Baudry, S., Collignon, S., and Duchateau, J. (2015). Influence of age and posture on spinal and corticospinal excitability. Exp. Gerontol. 69, 62–69. doi:10.1016/j.exger.2015.06.006
Bean, J. F., Leveille, S. G., Kiely, D. K., Bandinelli, S., Guralnik, J. M., and Ferrucci, L. (2003). A comparison of leg power and leg strength within the InCHIANTI study: Which influences mobility more? J. Gerontol. A Biol. Sci. Med. Sci. 58, 728–733. doi:10.1093/gerona/58.8.m728
Beaudart, C., Zaaria, M., Pasleau, F., Reginster, J.-Y., and Bruyère, O. (2017). Health outcomes of sarcopenia: A systematic review and meta-analysis. Plos One 12, e0169548. doi:10.1371/journal.pone.0169548
Beijersbergen, C. M. I., Granacher, U., Gäbler, M., DeVita, P., and Hortobágyi, T. (2017a). Hip mechanics underlie lower extremity power training-induced increase in old adults’ fast gait velocity: The Potsdam Gait Study (POGS). Gait Posture 52, 338–344. doi:10.1016/j.gaitpost.2016.12.024
Beijersbergen, C. M. I., Granacher, U., Gäbler, M., Devita, P., and Hortobágyi, T. (2017b). Kinematic mechanisms of how power training improves healthy old adults’ gait velocity. Med. Sci. Sports Exerc. 49, 150–157. doi:10.1249/MSS.0000000000001082
Beijersbergen, C. M. I., Granacher, U., Gäbler, M., Devita, P., and Hortobágyi, T. (2017c). Power training-induced increases in muscle activation during gait in old adults. Med. Sci. Sports Exerc. 49, 2198–2025. doi:10.1249/MSS.0000000000001345
Beijersbergen, C. M. I., Granacher, U., Vandervoort, A. A., DeVita, P., and Hortobágyi, T. (2013). The biomechanical mechanism of how strength and power training improves walking speed in old adults remains unknown. Ageing Res. Rev. 12, 618–627. doi:10.1016/j.arr.2013.03.001
Berry, H. R., Tate, R. J., and Conway, B. A. (2017). Transcutaneous spinal direct current stimulation induces lasting fatigue resistance and enhances explosive vertical jump performance. PLOS ONE 12, e0173846. doi:10.1371/journal.pone.0173846
Bianchi, L., Angelini, D., and Lacquaniti, F. (1998a). Individual characteristics of human walking mechanics. Pflügers Archiv Eur. J. Physiology 436, 343–356. doi:10.1007/s004240050642
Bianchi, L., Angelini, D., Orani, G. P., and Lacquaniti, F. (1998b). Kinematic coordination in human gait: Relation to mechanical energy cost. J. Neurophysiol. 79, 2155–2170. doi:10.1152/jn.1998.79.4.2155
Bleyenheuft, C., and Detrembleur, C. (2012). Kinematic covariation in pediatric, adult and elderly subjects: Is gait control influenced by age? Clin. Biomech. 27, 568–572. doi:10.1016/j.clinbiomech.2012.01.010
Borghese, N. A., Bianchi, L., and Lacquaniti, F. (1996). Kinematic determinants of human locomotion. J. Physiol. 494, 863–879. doi:10.1113/jphysiol.1996.sp021539
Bornstein, M. H., and Bornstein, H. G. (1976). The pace of life. Nature 259, 557–559. doi:10.1038/259557a0
Borzuola, R., Giombini, A., Torre, G., Campi, S., Albo, E., Bravi, M., et al. (2020). Central and peripheral neuromuscular adaptations to ageing. J. Clin. Med. 9, 741. doi:10.3390/jcm9030741
Boyer, K. A., Andriacchi, T. P., and Beaupre, G. S. (2012). The role of physical activity in changes in walking mechanics with age. Gait Posture 36, 149–153. doi:10.1016/j.gaitpost.2012.02.007
Browne, M. G., and Franz, J. R. (2019). Ankle power biofeedback attenuates the distal-to-proximal redistribution in older adults. Gait Posture 71, 44–49. doi:10.1016/j.gaitpost.2019.04.011
Bua, E., Johnson, J., Herbst, A., Delong, B., McKenzie, D., Salamat, S., et al. (2006). Mitochondrial DNA-deletion mutations accumulate intracellularly to detrimental levels in aged human skeletal muscle fibers. Am. J. Hum. Genet. 79, 469–480. doi:10.1086/507132
Cade, W. T., and Yarasheski, K. E. (2006). “Metabolic and molecular aspects of sarcopenia,” in Principles of molecular medicine. Editors M. S. Runge,, and C. Patterson (Totowa, NJ: Humana Press), 529–534. doi:10.1007/978-1-59259-963-9_50
Cappellini, G., Ivanenko, Y. P., Dominici, N., Poppele, R. E., and Lacquaniti, F. (2010). Migration of motor pool activity in the spinal cord reflects body mechanics in human locomotion. J. Neurophysiol. 104, 3064–3073. doi:10.1152/jn.00318.2010
Chen, N., He, X., Feng, Y., Ainsworth, B. E., and Liu, Y. (2021). Effects of resistance training in healthy older people with sarcopenia: A systematic review and meta-analysis of randomized controlled trials. Eur. Rev. Aging Phys. Activity 18, 23–19. doi:10.1186/s11556-021-00277-7
Cheron, G., Bouillot, E., Dan, B., Bengoetxea, A., Draye, J. P., and Lacquaniti, F. (2001). Development of a kinematic coordination pattern in toddler locomotion: Planar covariation. Exp. Brain Res. 137, 455–466. doi:10.1007/s002210000663
Christie, J. (2011). Progressive resistance strength training for improving physical function in older adults. Int. J. Older People Nurs. 6, 244–246. doi:10.1111/j.1748-3743.2011.00291.x
Cogliati, M., Cudicio, A., Martinez-Valdes, E., Tarperi, C., Schena, F., Orizio, C., et al. (2020). Half marathon induces changes in central control and peripheral properties of individual motor units in master athletes. J. Electromyogr. Kinesiol. 55, 102472. doi:10.1016/j.jelekin.2020.102472
Cruz-Jentoft, A. J., Bahat, G., Bauer, J., Boirie, Y., Bruyère, O., Cederholm, T., et al. (2019). Sarcopenia: Revised European consensus on definition and diagnosis. Age Ageing 48, 16–31. doi:10.1093/ageing/afy169
Damasceno, A., Delicio, A. M., Mazo, D. F. C., Zullo, J. F. D., Scherer, P., Ng, R. T. Y., et al. (2005). Primitive reflexes and cognitive function. Arq. Neuropsiquiatr. 63, 577–582. doi:10.1590/s0004-282x2005000400004
Davis, S. W., Dennis, N. A., Buchler, N. G., White, L. E., Madden, D. J., and Cabeza, R. (2009). Assessing the effects of age on long white matter tracts using diffusion tensor tractography. NeuroImage 46, 530–541. doi:10.1016/j.neuroimage.2009.01.068
Dayanidhi, S., Kutch, J. J., and Valero-Cuevas, F. J. (2013). Decrease in muscle contraction time complements neural maturation in the development of dynamic manipulation. J. Neurosci. 33, 15050–15055. doi:10.1523/JNEUROSCI.1968-13.2013
Delabastita, T., Hollville, E., Catteau, A., Cortvriendt, P., De Groote, F., and Vanwanseele, B. (2021). Distal-to-proximal joint mechanics redistribution is a main contributor to reduced walking economy in older adults. Scand. J. Med. Sci. Sports 31, 1036–1047. doi:10.1111/sms.13929
Deschenes, M. R., Roby, M. A., Eason, M. K., and Harris, M. B. (2010). Remodeling of the neuromuscular junction precedes sarcopenia related alterations in myofibers. Exp. Gerontol. 45, 389–393. doi:10.1016/j.exger.2010.03.007
Dewolf, A. H., Ivanenko, Y. P., Zelik, K. E., Lacquaniti, F., and Willems, P. A. (2019a). Differential activation of lumbar and sacral motor pools during walking at different speeds and slopes. J. Neurophysiol. 122, 872–887. doi:10.1152/jn.00167.2019
Dewolf, A. H., Ivanenko, Y., Zelik, K. E., Lacquaniti, F., and Willems, P. A. (2018). Kinematic patterns while walking on a slope at different speeds. J. Appl. Physiol. 125, 642–653. doi:10.1152/japplphysiol.01020.2017
Dewolf, A. H., Meurisse, G. M., Schepens, B., and Willems, P. A. (2019b). Effect of walking speed on the intersegmental coordination of lower-limb segments in elderly adults. Gait Posture 70, 156–161. doi:10.1016/j.gaitpost.2019.03.001
Dewolf, A. H., Sylos Labini, F., Ivanenko, Y., and Lacquaniti, F. (2020a). Development of locomotor-related movements in early infancy. Front. Cell Neurosci. 14, 623759. doi:10.3389/fncel.2020.623759
Dewolf, A. H., Sylos-Labini, F., Cappellini, G., Ivanenko, Y., and Lacquaniti, F. (2021a). Age-related changes in the neuromuscular control of forward and backward locomotion. PLOS ONE 16, e0246372. doi:10.1371/journal.pone.0246372
Dewolf, A. H., Sylos-Labini, F., Cappellini, G., Lacquaniti, F., and Ivanenko, Y. (2020b). Emergence of different gaits in infancy: Relationship between developing neural circuitries and changing biomechanics. Front. Bioeng. Biotechnol. 8, 473. doi:10.3389/fbioe.2020.00473
Dewolf, A. H., Sylos-Labini, F., Cappellini, G., Zhvansky, D., Willems, P. A., Ivanenko, Y., et al. (2021b). Neuromuscular age-related adjustment of gait when moving upwards and downwards. Front. Hum. Neurosci. 15, 749366. doi:10.3389/fnhum.2021.749366
Doherty, T. J. (2003). Invited review: Aging and sarcopenia. J. Appl. Physiol. 95, 1717–1727. doi:10.1152/japplphysiol.00347.2003
Doherty, T. J., Vandervoort, A. A., Taylor, A. W., and Brown, W. F. (1993). Effects of motor unit losses on strength in older men and women. J. Appl. Physiol. 74, 868–874. doi:10.1152/jappl.1993.74.2.868
Domingues-Faria, C., Vasson, M.-P., Goncalves-Mendes, N., Boirie, Y., and Walrand, S. (2016). Skeletal muscle regeneration and impact of aging and nutrition. Ageing Res. Rev. 26, 22–36. doi:10.1016/j.arr.2015.12.004
Dominici, N., Ivanenko, Y. P., Cappellini, G., d’Avella, A., Mondì, V., Cicchese, M., et al. (2011). Locomotor primitives in newborn babies and their development. Science 334, 997–999. doi:10.1126/science.1210617
Dominici, N., Ivanenko, Y. P., Cappellini, G., Zampagni, M. L., and Lacquaniti, F. (2010). Kinematic strategies in newly walking toddlers stepping over different support surfaces. J. Neurophysiol. 103, 18–1684. doi:10.1152/jn.00945.2009
el Hadouchi, M., Kiers, H., de Vries, R., Veenhof, C., and van Dieën, J. (2022). Effectiveness of power training compared to strength training in older adults: A systematic review and meta-analysis. Eur. Rev. Aging Phys. Activity 19, 1–15. doi:10.1186/s11556-022-00297-x
Feltes, B. C., Poloni, J. de F., and Bonatto, D. (2015). Development and aging: Two opposite but complementary phenomena. Aging Health - A Syst. Biol. Perspective 40, 74–84. doi:10.1159/000364932
Fiatarone, M. A., Marks, E. C., Ryan, N. D., Meredith, C. N., Lipsitz, L. A., and Evans, W. J. (1990). High-intensity strength training in nonagenarians. Effects on skeletal muscle. JAMA 263, 3029–3034. doi:10.1001/jama.263.22.3029
Forssberg, H. (1985). Ontogeny of human locomotor control I. Infant stepping, supported locomotion and transition to independent locomotion. Exp. Brain Res. 57, 480–493. doi:10.1007/BF00237835
Foster, R. J., Maganaris, C. N., Reeves, N. D., and Buckley, J. G. (2019). Centre of mass control is reduced in older people when descending stairs at an increased riser height. Gait Posture 73, 305–314. doi:10.1016/j.gaitpost.2019.08.004
Gad, P., Hastings, S., Zhong, H., Seth, G., Kandhari, S., and Edgerton, V. R. (2021). Transcutaneous spinal neuromodulation reorganizes neural networks in patients with cerebral palsy. Neurotherapeutics 18, 1953–1962. doi:10.1007/s13311-021-01087-6
Gava, P., Kern, H., and Carraro, U. (2015). Age-associated power decline from running, jumping, and throwing male masters world records. Exp. Aging Res. 41, 115–135. doi:10.1080/0361073X.2015.1001648
Good, C. D., Johnsrude, I. S., Ashburner, J., Henson, R. N., Friston, K. J., and Frackowiak, R. S. (2001). A voxel-based morphometric study of ageing in 465 normal adult human brains. Neuroimage 14, 21–36. doi:10.1006/nimg.2001.0786
Gossman, M. D., and Jacobs, L. (1980). Three primitive reflexes in parkinsonism patients. Neurology 30, 189–192. doi:10.1212/wnl.30.2.189
Gueugnon, M., Stapley, P. J., Gouteron, A., Lecland, C., Morisset, C., Casillas, J.-M., et al. (2019). Age-related adaptations of lower limb intersegmental coordination during walking. Front. Bioeng. Biotechnol. 7, 173. doi:10.3389/fbioe.2019.00173
Guralnik, J. M., Ferrucci, L., Pieper, C. F., Leveille, S. G., Markides, K. S., Ostir, G. V., et al. (2000). Lower extremity function and subsequent disability: Consistency across studies, predictive models, and value of gait speed alone compared with the short physical performance battery. Journals Gerontology - Ser. A Biol. Sci. Med. Sci. 55, 221–231. doi:10.1093/gerona/55.4.M221
Hallemans, A., De Clercq, D., Dongen, S. V., and Aerts, P. (2006). Changes in foot-function parameters during the first 5 months after the onset of independent walking: A longitudinal follow-up study. Gait Posture 23, 142–148. doi:10.1016/j.gaitpost.2005.01.003
Hassan, A. S., Fajardo, M. E., Cummings, M., McPherson, L. M., Negro, F., Dewald, J. P. A., et al. (2021). Estimates of persistent inward currents are reduced in upper limb motor units of older adults. J. Physiology 599, 4865–4882. doi:10.1113/JP282063
Haug, H., and Eggers, R. (1991). Morphometry of the human cortex cerebri and corpus striatum during aging. Neurobiol. Aging 12, 336–338. doi:10.1016/0197-4580(91)90013-A
Henderson, G., Tomlinson, B. E., and Gibson, P. H. (1980). Cell counts in human cerebral cortex in normal adults throughout life using an image analysing computer. J. Neurological Sci. 46, 113–136. doi:10.1016/0022-510X(80)90048-9
Hobo, K., Kawase, J., Tamura, F., Groher, M., Kikutani, T., and Sunakawa, H. (2014). Effects of the reappearance of primitive reflexes on eating function and prognosis. Geriatr. Gerontol. Int. 14, 190–197. doi:10.1111/ggi.12078
Hortobágyi, T., Lesinski, M., Gäbler, M., VanSwearingen, J. M., Malatesta, D., and Granacher, U. (2015). Effects of three types of exercise interventions on healthy old adults’ gait speed: A systematic review and meta-analysis. Sports Med. 45, 1627–1643. doi:10.1007/s40279-015-0371-2
Ivanenko, Y. P., Cappellini, G., Poppele, R. E., and Lacquaniti, F. (2008). Spatiotemporal organization of alpha-motoneuron activity in the human spinal cord during different gaits and gait transitions. Eur. J. Neurosci. 27, 3351–3368. doi:10.1111/j.1460-9568.2008.06289.x
Ivanenko, Y. P., Dominici, N., Cappellini, G., Dan, B., Cheron, G., and Lacquaniti, F. (2004). Development of pendulum mechanism and kinematic coordination from the first unsupported steps in toddlers. J. Exp. Biol. 207, 3797–3810. doi:10.1242/jeb.01214
Ivanenko, Y. P., Dominici, N., Cappellini, G., Di Paolo, A., Giannini, C., Poppele, R. E., et al. (2013). Changes in the spinal segmental motor output for stepping during development from infant to adult. J. Neurosci. 33, 3025–336a. doi:10.1523/JNEUROSCI.2722-12.2013
Jacobs, L., and Gossman, M. D. (1980). Three primitive reflexes in normal adults. Neurology 30, 184–188. doi:10.1212/wnl.30.2.184
Jang, Y. C., and Van Remmen, H. (2011). Age-associated alterations of the neuromuscular junction. Exp. Gerontol. 46, 193–198. doi:10.1016/j.exger.2010.08.029
Jankowska, E. (2017). Spinal control of motor outputs by intrinsic and externally induced electric field potentials. J. Neurophysiology 118, 1221–1234. doi:10.1152/jn.00169.2017
Kadhiresan, V. A., Hassett, C. A., and Faulkner, J. A. (1996). Properties of single motor units in medial gastrocnemius muscles of adult and old rats. J. Physiol. 493, 543–552. doi:10.1113/jphysiol.1996.sp021402
Keating, C. J., Cabrera-Linares, J. C., Párraga-Montilla, J. A., Latorre-Román, P. A., del Castillo, R. M., and García-Pinillos, F. (2021). Influence of resistance training on gait and balance parameters in older adults: A systematic review. Int. J. Environ. Res. Public Health 18, 1759. doi:10.3390/ijerph18041759
Kelley, G. A., and Kelley, K. S. (2017). Is sarcopenia associated with an increased risk of all-cause mortality and functional disability? Exp. Gerontol. 96, 100–103. doi:10.1016/j.exger.2017.06.008
Kendall, F. P. (2005). Muscles: Testing and function with posture and pain. Philadelphia: Lippincott Williams and Wilkins.
Klass, M., Baudry, S., and Duchateau, J. (2008). Age-related decline in rate of torque development is accompanied by lower maximal motor unit discharge frequency during fast contractions. J. Appl. Physiol. 104, 739–746. doi:10.1152/japplphysiol.00550.2007
Koller, W. C. (1984). Primitive reflexes in the evaluation of the aging patient. Clin. Gerontol. 3, 19–22. doi:10.1300/J018v03n02_04
Kraemer, W. J., Adams, K., Cafarelli, E., Dudley, G. A., Dooly, C., Feigenbaum, M. S., et al. (2002). American College of Sports Medicine position stand. Progression models in resistance training for healthy adults. Med. Sci. Sports Exerc. 34, 364–380. doi:10.1097/00005768-200202000-00027
Kwon, Y. N., and Yoon, S. S. (2017). Sarcopenia: Neurological point of view. J. Bone Metab. 24, 83–89. doi:10.11005/jbm.2017.24.2.83
La Scaleia, V., Ivanenko, Y. P., Zelik, K. E., and Lacquaniti, F. (2014). Spinal motor outputs during step-to-step transitions of diverse human gaits. Front. Hum. Neurosci. 8, 305. doi:10.3389/fnhum.2014.00305
Landi, F., Liperoti, R., Russo, A., Giovannini, S., Tosato, M., Capoluongo, E., et al. (2012). Sarcopenia as a risk factor for falls in elderly individuals: Results from the ilSIRENTE study. Clin. Nutr. 31, 652–658. doi:10.1016/j.clnu.2012.02.007
Larsson, L., Li, X., Berg, H. E., and Frontera, W. R. (1996). Effects of removal of weight-bearing function on contractility and myosin isoform composition in single human skeletal muscle cells. Pflugers Arch. 432, 320–328. doi:10.1007/s004240050139
Larsson, L., Sjödin, B., and Karlsson, J. (1978). Histochemical and biochemical changes in human skeletal muscle with age in sedentary males, age 22-65 years. Acta Physiol. Scand. 103, 31–39. doi:10.1111/j.1748-1716.1978.tb06187.x
Lecker, S. H., Jagoe, R. T., Gilbert, A., Gomes, M., Baracos, V., Bailey, J., et al. (2004). Multiple types of skeletal muscle atrophy involve a common program of changes in gene expression. FASEB J. 18, 39–51. doi:10.1096/fj.03-0610com
Levine, R. V., and Norenzayan, A. (1999). The pace of life in 31 countries. J. Cross-Cultural Psychol. 30, 178–205. doi:10.1177/0022022199030002003
Levins, A., and Moritz, C. T. (2017). Therapeutic stimulation for restoration of function after spinal cord injury. Physiology 32, 391–398. doi:10.1152/physiol.00010.2017
Lexell, J., Henriksson-Larsén, K., Winblad, B., and Sjöström, M. (1983). Distribution of different fiber types in human skeletal muscles: Effects of aging studied in whole muscle cross sections. Muscle Nerve 6, 588–595. doi:10.1002/mus.880060809
Lexell, J. (1995). Human aging, muscle mass, and fiber type composition. J. Gerontol. A Biol. Sci. Med. Sci. 50, 11–16. doi:10.1093/gerona/50a.special_issue.11
Lexell, J., and Taylor, C. C. (1991). Variability in muscle fibre areas in whole human quadriceps muscle: Effects of increasing age. J. Anat. 174, 239–249.
Liu, P., Hao, Q., Hai, S., Wang, H., Cao, L., and Dong, B. (2017). Sarcopenia as a predictor of all-cause mortality among community-dwelling older people: A systematic review and meta-analysis. Maturitas 103, 16–22. doi:10.1016/j.maturitas.2017.04.007
Luc-Harkey, B. A., Franz, J. R., Blackburn, J. T., Padua, D. A., Hackney, A. C., and Pietrosimone, B. (2018). Real-time biofeedback can increase and decrease vertical ground reaction force, knee flexion excursion, and knee extension moment during walking in individuals with anterior cruciate ligament reconstruction. J. Biomech. 76, 94–102. doi:10.1016/j.jbiomech.2018.05.043
Malloggi, C., Rota, V., Catino, L., Malfitano, C., Scarano, S., Soranna, D., et al. (2019). Three-dimensional path of the body centre of mass during walking in children: An index of neural maturation. Int. J. Rehabil. Res. 42, 112–119. doi:10.1097/MRR.0000000000000345
Markov, A., Hauser, L., and Chaabene, H. (2022). Effects of concurrent strength and endurance training on measures of physical fitness in healthy middle-aged and older adults: A systematic review with meta-analysis. Sports Med. 53, 437–455. doi:10.1007/s40279-022-01764-2
Martino, G., Ivanenko, Y. P., Serrao, M., Ranavolo, A., d’Avella, A., Draicchio, F., et al. (2014). Locomotor patterns in cerebellar ataxia. J. Neurophysiol. 112, 2810–2821. doi:10.1152/jn.00275.2014
Massaad, F., Lejeune, T. M., and Detrembleur, C. (2007). The up and down bobbing of human walking: A compromise between muscle work and efficiency. J. Physiology 582, 789–799. doi:10.1113/jphysiol.2007.127969
Mau-Moeller, A., Behrens, M., Lindner, T., Bader, R., and Bruhn, S. (2013). Age-related changes in neuromuscular function of the quadriceps muscle in physically active adults. J. Electromyogr. Kinesiol 23, 640–648. doi:10.1016/j.jelekin.2013.01.009
McNeil, C. J., Doherty, T. J., Stashuk, D. W., and Rice, C. L. (2005). Motor unit number estimates in the tibialis anterior muscle of young, old, and very old men. Muscle Nerve 31, 461–467. doi:10.1002/mus.20276
McPhee, J. S., Cameron, J., Maden-Wilkinson, T., Piasecki, M., Yap, M. H., Jones, D. A., et al. (2018). The contributions of fiber atrophy, fiber loss, in situ specific force, and voluntary activation to weakness in sarcopenia. J. Gerontol. A Biol. Sci. Med. Sci. 73, 1287–1294. doi:10.1093/gerona/gly040
Monaco, V., Ghionzoli, A., and Micera, S. (2010). Age-related modifications of muscle synergies and spinal cord activity during locomotion. J. Neurophysiol. 104, 2092–2102. doi:10.1152/jn.00525.2009
Murton, A. J. (2015). Muscle protein turnover in the elderly and its potential contribution to the development of sarcopenia. Proc. Nutr. Soc. 74, 387–396. doi:10.1017/S0029665115000130
Noble, J. W., and Prentice, S. D. (2008). Intersegmental coordination while walking up inclined surfaces: Age and ramp angle effects. Exp. Brain Res. 189, 249–255. doi:10.1007/s00221-008-1464-z
Oertel, G. (1986). Changes in human skeletal muscles due to ageing. Histological and histochemical observations on autopsy material. Acta Neuropathol. 69, 309–313. doi:10.1007/BF00688309
Orssatto, L. B. R., Borg, D. N., Blazevich, A. J., Sakugawa, R. L., Shield, A. J., and Trajano, G. S. (2021a). Intrinsic motoneuron excitability is reduced in soleus and tibialis anterior of older adults. Geroscience 43, 2719–2735. doi:10.1007/s11357-021-00478-z
Orssatto, L. B. R., Mesquita, R. N. O., and Phillips, K. M. (2021b). Looking at the bigger PICture: Understanding and counteracting the decline of persistent inward currents in older adults. J. Physiology 599, 5137–5139. doi:10.1113/JP282370
Payne, A. M., and Delbono, O. (2004). Neurogenesis of excitation-contraction uncoupling in aging skeletal muscle. Exerc Sport Sci. Rev. 32, 36–40. doi:10.1097/00003677-200401000-00008
Payne, V. G., and Isaacs, L. D. (2017). Human motor development: A lifespan approach. Oxfordshire: Routledge.
Piasecki, M., Ireland, A., Coulson, J., Stashuk, D. W., Hamilton-Wright, A., Swiecicka, A., et al. (2016). Motor unit number estimates and neuromuscular transmission in the tibialis anterior of master athletes: Evidence that athletic older people are not spared from age-related motor unit remodeling. Physiol. Rep. 4, e12987. doi:10.14814/phy2.12987
Piasecki, M., Ireland, A., Piasecki, J., Stashuk, D. W., McPhee, J. S., and Jones, D. A. (2018). The reliability of methods to estimate the number and size of human motor units and their use with large limb muscles. Eur. J. Appl. Physiol. 118, 767–775. doi:10.1007/s00421-018-3811-5
Radaelli, R., Trajano, G. S., Freitas, S. R., Izquierdo, M., Cadore, E. L., and Pinto, R. S. (2023). Power training prescription in older individuals: Is it safe and effective to promote neuromuscular functional improvements? Sports Med. 53, 569–576. doi:10.1007/s40279-022-01758-0
Rygiel, K. A., Picard, M., and Turnbull, D. M. (2016). The ageing neuromuscular system and sarcopenia: A mitochondrial perspective. J. Physiology 594, 4499–4512. doi:10.1113/JP271212
Santuz, A., Brüll, L., Ekizos, A., Schroll, A., Eckardt, N., Kibele, A., et al. (2020). Neuromotor dynamics of human locomotion in challenging settings. iScience 23, 100796. doi:10.1016/j.isci.2019.100796
Scaglioni, G., Ferri, A., Minetti, A. E., Martin, A., Van Hoecke, J., Capodaglio, P., et al. (2002). Plantar flexor activation capacity and H reflex in older adults: Adaptations to strength training. J. Appl. Physiology 92, 2292–2302. doi:10.1152/japplphysiol.00367.2001
Shefer, G., Van de Mark, D. P., Richardson, J. B., and Yablonka-Reuveni, Z. (2006). Satellite-cell pool size does matter: Defining the myogenic potency of aging skeletal muscle. Dev. Biol. 294, 50–66. doi:10.1016/j.ydbio.2006.02.022
Sheth, K. A., Iyer, C. C., Wier, C. G., Crum, A. E., Bratasz, A., Kolb, S. J., et al. (2018). Muscle strength and size are associated with motor unit connectivity in aged mice. Neurobiol. Aging 67, 128–136. doi:10.1016/j.neurobiolaging.2018.03.016
Shigemoto, K., Kubo, S., Mori, S., Yamada, S., Akiyoshi, T., and Miyazaki, T. (2010). Muscle weakness and neuromuscular junctions in aging and disease. Geriatrics Gerontology Int. 10, S137–S147. doi:10.1111/j.1447-0594.2010.00608.x
Siparsky, P. N., Kirkendall, D. T., and Garrett, W. E. (2014). Muscle changes in aging: Understanding sarcopenia. Sports Health 6, 36–40. doi:10.1177/1941738113502296
Sloot, L. H., Malheiros, S., Truijen, S., Saeys, W., Mombaur, K., Hallemans, A., et al. (2021). Decline in gait propulsion in older adults over age decades. Gait Posture 90, 475–482. doi:10.1016/j.gaitpost.2021.09.166
Song, M.-Y., Ruts, E., Kim, J., Janumala, I., Heymsfield, S., and Gallagher, D. (2004). Sarcopenia and increased adipose tissue infiltration of muscle in elderly African American women. Am. J. Clin. Nutr. 79, 874–880. doi:10.1093/ajcn/79.5.874
Song, W., and Martin, J. H. (2017). Spinal cord direct current stimulation differentially modulates neuronal activity in the dorsal and ventral spinal cord. J. Neurophysiology 117, 1143–1155. doi:10.1152/jn.00584.2016
Song, W., and Martin, J. H. (2022). Trans-spinal direct current stimulation targets Ca2+ channels to induce persistent motor unit responses. Front. Neurosci. 16, 856948. doi:10.3389/fnins.2022.856948
Stålberg, E., and Fawcett, P. R. (1982). Macro EMG in healthy subjects of different ages. J. Neurol. Neurosurg. Psychiatry 45, 870–878. doi:10.1136/jnnp.45.10.870
Stock, M. S., Thompson, B. J., and Sobolewski, E. J. (2019). Age-related differences in the predictability of fast gait speed with absolute and rapid squat strength. J. Sci. Sport Exerc. 1, 273–280. doi:10.1007/s42978-019-00032-6
Suzuki, M., Okamura, T., Shimazu, Y., Takahashi, H., Eguchi, K., Kano, K., et al. (1992). A study of falls experienced by institutionalized elderly. Nihon Koshu Eisei Zasshi 39, 927–940.
Sylos-Labini, F., La Scaleia, V., Cappellini, G., Dewolf, A., Fabiano, A., Solopova, I. A., et al. (2022). Complexity of modular neuromuscular control increases and variability decreases during human locomotor development. Commun. Biol. 5, 1256. doi:10.1038/s42003-022-04225-8
Sylos-Labini, F., Magnani, S., Cappellini, G., La Scaleia, V., Fabiano, A., Picone, S., et al. (2017). Foot placement characteristics and plantar pressure distribution patterns during stepping on ground in neonates. Front. Physiol. 8, 784. doi:10.3389/fphys.2017.00784
Taylor, C., McHugh, C., Mockler, D., Minogue, C., Reilly, R. B., and Fleming, N. (2021). Transcutaneous spinal cord stimulation and motor responses in individuals with spinal cord injury: A methodological review. PLoS ONE 16, e0260166. doi:10.1371/journal.pone.0260166
Taylor, D. (2014). Physical activity is medicine for older adults. Postgrad. Med. J. 90, 26–32. doi:10.1136/postgradmedj-2012-131366
Thelen, E., and Fisher, D. M. (1982). Newborn stepping: An explanation for a “disappearing” reflex. Dev. Psychol. 18, 760–775. doi:10.1037/0012-1649.18.5.760
van Boxtel, M. P. J., Bosma, H., Jolles, J., and Vreeling, F. W. (2006). Prevalence of primitive reflexes and the relationship with cognitive change in healthy adults: A report from the maastricht aging study. J. Neurol. 253, 935–941. doi:10.1007/s00415-006-0138-7
Waanders, J. B., Murgia, A., DeVita, P., Franz, J. R., and Hortobágyi, T. (2021). Age does not affect the relationship between muscle activation and joint work during incline and decline walking. J. Biomechanics 124, 110555. doi:10.1016/j.jbiomech.2021.110555
Winter, D. A., Patla, A. E., Frank, J. S., and Walt, S. E. (1990). Biomechanical walking pattern changes in the fit and healthy elderly. Phys. Ther. 70, 340–347. doi:10.1093/ptj/70.6.340
Wu, J., Wang, X., Ye, M., Wang, L., and Zheng, G. (2021). Effect of regular resistance training on memory in older adults: A systematic review. Exp. Gerontol. 150, 111396. doi:10.1016/j.exger.2021.111396
Yang, J. F., Stephens, M. J., and Vishram, R. (1998). Infant stepping: A method to study the sensory control of human walking. J. Physiol. 507, 927–937. doi:10.1111/j.1469-7793.1998.927bs.x
Yokoyama, H., Ogawa, T., Shinya, M., Kawashima, N., and Nakazawa, K. (2017). Speed dependency in α-motoneuron activity and locomotor modules in human locomotion: Indirect evidence for phylogenetically conserved spinal circuits. Proc. Biol. Sci. 284, 20170290. doi:10.1098/rspb.2017.0290
Keywords: neuromechanics, gait, physio-mechanics, direct current spinal stimulation, walking pattern
Citation: Nùñez-Lisboa M, Valero-Breton M and Dewolf AH (2023) Unraveling age-related impairment of the neuromuscular system: exploring biomechanical and neurophysiological perspectives. Front. Physiol. 14:1194889. doi: 10.3389/fphys.2023.1194889
Received: 27 March 2023; Accepted: 14 June 2023;
Published: 22 June 2023.
Edited by:
Leonardo Alexandre Peyré-Tartaruga, Federal University of Rio Grande do Sul, BrazilReviewed by:
Clarissa Brusco, Edith Cowan University, AustraliaValeria Feijo Martins, Federal University of Rio Grande do Sul, Brazil
Copyright © 2023 Nùñez-Lisboa, Valero-Breton and Dewolf. This is an open-access article distributed under the terms of the Creative Commons Attribution License (CC BY). The use, distribution or reproduction in other forums is permitted, provided the original author(s) and the copyright owner(s) are credited and that the original publication in this journal is cited, in accordance with accepted academic practice. No use, distribution or reproduction is permitted which does not comply with these terms.
*Correspondence: A. H. Dewolf, Arthur.dewolf@uclouvain.be