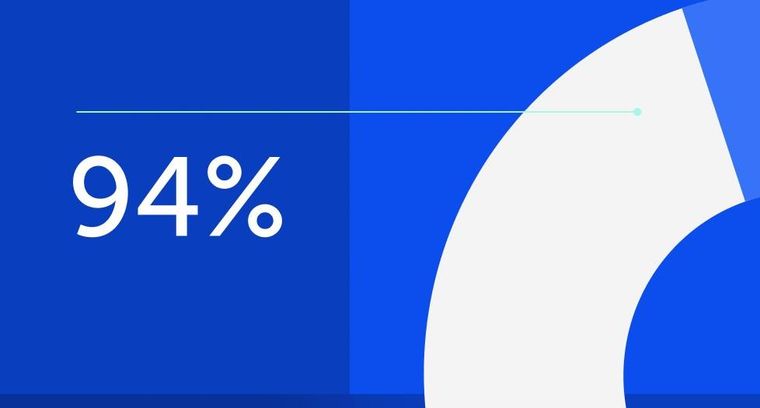
94% of researchers rate our articles as excellent or good
Learn more about the work of our research integrity team to safeguard the quality of each article we publish.
Find out more
ORIGINAL RESEARCH article
Front. Physiol., 17 August 2023
Sec. Exercise Physiology
Volume 14 - 2023 | https://doi.org/10.3389/fphys.2023.1182833
This article is part of the Research TopicA Physiologically-Based Approach to Study Different Types of Locomotion in Association with Core PerformanceView all 14 articles
Background: During competition and training, exercises involving the lungs may occur throughout the sport, and fatigue is a major injury risk factor in sport, before and after fatigue studies of changes in the lungs are relatively sparse. This study is to investigate into how fatigue affects the lower limb’s biomechanics during a forward lunge.
Methods: 15 healthy young men participate in this study before and after to exposed to a fatigue protocol then we tested the forward lunge to obtain kinematic, kinetic changing during the task, and to estimate the corresponding muscles’ strength changes in the hip, knee, and ankle joints. The measurement data before and after the fatigue protocol were compared with paired samples t-test.
Results: In the sagittal and horizontal planes of the hip and knee joints, in both, the peak angles and joint range of motion (ROM) increased, whereas the moments in the sagittal plane of the knee joint smaller. The ankle joint’s maximum angle smaller after fatigue. Peak vertical ground reaction force (vGRF) and peak contact both significantly smaller after completing the fatigue protocol and the quadriceps mean and maximum muscular strength significantly increased.
Conclusion: After completing a fatigue protocol during lunge the hip, knee, and ankle joints become less stable in both sagittal and horizontal planes, hip and knee range of motion becomes greater. The quadriceps muscles are more susceptible to fatigue and reduced muscle force. Trainers should focus more on the thigh muscle groups.
Lunge is an important part of a lower-limb muscle strength training and rehabilitation programs. This task is typically performed to enhance the lower limb muscles'-ability to generate higher force, especially in the quadriceps, and to minimize the risk of joint injury (Keogh, 1999), and develop functional postural balance (Ebben et al., 2009). Lunge requires more balance than the more commonly used deep squat thus it can be used more safely to strengthen the biarticular quadriceps and hamstrings muscles, which are necessary for appropriate rehabilitation of gait and daily living activities (Wilson et al., 2008; Dregney et al., 2023). Also, this task often makes sure that the athlete’s lower body and trunk become strong, flexible, and stable enough to support safe and efficient augmentation training (Tippett and Voight, 1995).
While modest knee loads support the health of the joint cartilage (Griffin and Guilak, 2005), high-intensity loads or extended exercise might cause injuries in the knee (Ebben et al., 2009; Richmond et al., 2013). Repeated work exposure to bow steps may also raise the incidence of joint degenerations, especially in the knee joint (Amin et al., 2008). For instance, repetitive knee flexion during work duties can lead to excessive fatigue (Richmond et al., 2013). Furthermore, the risk of injury increases when the intensity of exercise increases and activation of certain muscles increases or even exceeds the activation of other muscles (Irish et al., 2010).
Muscle fatigue, which is characterized by decreased muscular efficiency and force production capacity after extended exposure to activity (Gandevia et al., 2006; Yamane et al., 2023), has repeatedly been proven to have a negative impact on performance (Pincivero et al., 2000). In female athletes doing single-leg landings, neuromuscular fatigue has been demonstrated to alter knee flexion, knee abduction, and hip internal rotation (McLean and Samorezov, 2009). Moreover, the muscles’ diminished capacity for force production may affect their capacity to function as dynamic joint dampers (Pincivero et al., 2000) and may contribute to damage (Du and Fan, 2020). For instance, epidemiological research shows that greater incidence of badminton injuries are discovered during trainings because of muscle strength decreased (Shariff et al., 2009; Kondrič et al., 2011). In badminton, lunge is frequently employed as a fundamental step, and when players grow tired, they are more likely to suffer ankle injuries in addition to knee injuries during practice or competition (Herbaut et al., 2018). Garcia et al. (2012), however, showed the lunge squat is an exercise that accurately represents the tiredness process and enhances knee stability by equally activating the quadriceps and hamstring complexes, and this co-activation Weir et al. (1998) observed to occur simultaneously with fatigue. The fatigue process reduces the contraction force and muscle activation time of the quadriceps, which triggers an increase in hamstring muscle activity during fatigue (Garcia et al., 2012). By preventing external joint rotation, the quadriceps, hamstrings, and gastrocnemius muscles stabilize the knee (Winby et al., 2009). On the other hand, fatigue fractures are a common injury that typically arise from running exercise. Consequently, a vital initial step in guiding injury prevention research and informing injury screening is understanding how fatigue affects movement patterns in standard musculoskeletal screening procedures (Weeks et al., 2015).
Some studies have illustrated lunge and squat EMG and kinematic changes under different loading conditions (Stastny et al., 2015a; Stastny et al., 2015b), but fewer studies have been conducted after prolonged exercise or even fatigue. The muscle performance during extended exercise is unknown, although experienced gym athletes show greater muscular strength and better joint coordination compared to novice, which helps to minimize redundancy in force production through joint coordination and employing muscle power effectively (Phomsoupha and Laffaye, 2015).
There is a lack of many studies focusing on how fatigue induce kinematic and kinetics changing in lunge. There is a lack of information on the kinematics, kinetics, and changes in the force generation of the relevant muscle groups in the lunge before and after fatigue, despite the kinematic performance of the lunge in various directions (Comfort et al., 2015; Goulette et al., 2021; Park et al., 2021) and of closed chain exercises of the lower limb after fatigue (Gribble et al., 2007; Weeks et al., 2015; Du and Fan, 2020). Therefore, the aim of this study was to investigate the effects of fatigue on lower limb biomechanics during the anterior lunge before and after completing a fatigue protocol. Fatigue is a major contributor to injury risk, and relevant objective data provide the required understanding for understanding the cause of injury and to help improve injury prevention programs.
The sample size was determined using data from previous studies (Nielsen et al., 2018; Liu et al., 2023). At least 15 participants were selected using G*Power3.1, Statistical test choosing Means: Wilcoxon signed-rank test (matched pairs) with tails of 2 and an alpha value of 0.05 and a power value of 0.80 and effect size of 0.80 (Faul et al., 2009). 15 young healthy males were recruited for this study, and relevant basic information is shown in Figure 1. The dominant leg was identified by self-declaration of the preferred leg during kicking which was defined as the dominant limb. Participants had no lower limb muscle or bone illness or injury 6 months prior to this testing session. Before the experiment, participants fasted for 2 h and were not allowed to drink any alcohol or caffeine for the following 24 h. To prevent shoes from influencing the experiment’s outcomes, they all wore shoes of the same design. All participants signed an informed permission form before the experiment and were made aware of the importance and goal of the study as well as the testing methods. The Ethics Committee of Ningbo University Research Institute approved the experiment. (NO: RAGH202303073005.2).
FIGURE 1. (A) Basic information of participants; (B) Back, front, and side view of the participant’s reflective markers; (C) Forward lunge process and corresponding vGRF; a, Initial impact peak; b, Drive-off peak.
An eight-camera Vicon motion capture system (Vicon Metrics Ltd., Oxford UK) was used to capture the markers motion trajectories with a sampling frequency of 200 Hz. Simultaneously with motion capture an embedded Kistler 3D force plate (Kistler, Switzerland) was used to record ground reaction forces with 1000 Hz. Before the experiment, 38 reflective markers (diameter: 14 mm) were mounted onto their bodies (Figure 1).
The EMG signals of the rectus femoris (RF), biceps longus (BF), tibialis anterior (TA), and gastrocnemius (GA) were recorded simultaneously at 1000 Hz with a Delsys EMG test system (Delsys, Boston, MA, and United States) to validate the results of the model. Maximum voluntary isometric contractions (MVIC) were performed for the rectus femoris, biceps femoris long head, tibialis anterior, and gastrocnemius muscles using a dynamometer (CON-TREX MJ System, CMV, Dübendorf, Switzerland).
First, participants warmed up with 5 min run on a stationary motorized treadmill (h/p/cosmos sports and medical GmbH, Nussdorf-Traunstein, Germany). The leg length of each participant was measured manually with a metric ruler. We defined step length as the 70% of the leg length. The lunging leg position was marked with tape on the force platform for both side and starting position was also marked with tape with the corresponding distance for each participant leg length.
To obtain maximal values of EMG signals, participants performed a 5-s MVIC on a dynamometer, and each participant performed 3 consecutive measurements for each muscle group with 1-min rest interval. The MVIC was tested according to the recommended setting of the dynamometer, which allows participants to power up to the maximum force possible (Bliss and Dekerle, 2019). Professionals were on hand to guide and protect the participants throughout the test and secured the athlete’s trunk with a strap to avoid pronation. The participants were provided with concurrent visual feedback in the form of an isokinetic strength curve displayed on the dynamometer monitor. Verbal encouragement was also provided.
After skin preparation, shave off the body and clean the skin with alcohol. Bipolar surface electrodes were attached over four lower limb muscles. The sensor was placed over the muscle belly of the muscle parallel to the direction of the muscle fascicles. Bipolar surface electrodes (adhesive disposable electrode, 10 mm interelectrode distance) were taped parallel to muscle fascicles. Electrode placements was in accordance with the SENIAM recommendations (Hermens et al., 2000). The EMG signals of the RF, BF, TA, and GA muscles were collected at 1000 Hz using a wireless Delsys EMG test system.
Before the experiment, participants received specific instructions from an expert coach with several years of experience to make sure that participants were familiar with the task and that can perform the task properly. Proper executions had to meet the following criteria: 1) the body must remain upright and perpendicular to the horizontal at all times, with the arms crossed at the sides of the body; 2) the dominant leg’s thigh must be perpendicular to the ground; and 3) the non-dominant leg’s knee must be close to the ground but not touch it. Lunge started in a standing position with feet parallel to each other, at the previously marked starting location of the experiment. Then lunge was initiated by a verbal command. Based on the lunge forward of the dominant leg (right leg) to the mark on the force platform, participants were asked to moved their body weight downward to the upright leg and to maintain an upright posture. After the deepest position the non-dominant leg followed the dominant leg forward and settles parallel to the upright foot and stood on the corresponding ground marking, i.e., the body back into a standing position at the end. When a lunge is completed, the expert will indicate the quality of this movement, whether it reached the standard and how it can be improved. Unqualified movements are not included in the data.
Prior completing the fatigue protocol participants were familiarized with the fatigue protocol and instructed in the use of the Borg Scale RPE 6–20 scale (Borg, 1982), to obtain the level of the subjective fatigue. In addition, heart rate was also measured with a portable detector (Polar RS100, Polar Electro Oy, Woodbury, NY, and United States) to monitor the changes in heart rate (HR) changes during the fatigue protocol. In to imitate lower limb movement patterns that might be seen in a sporting or recreational situation (Weeks et al., 2015), fatigue protocol consisted 20 repetitions of forward lunges (alternating forward lunges with the dominant and non-dominant leg) and a 400-m of running at an individually maximal running speed on a motorized treadmill. If fatigue level was minimal after one set of fatigue protocols, then the following set of procedures were carried out, and so on. HR and RPE data are used to quantify fatigue (Du and Fan, 2020). When the participants were unable to continue the fatigue protocol due to exhaustion, or their heart rates reached 90% of the maximum heart rate (HRmax = 220-age) predicted for their age, or if their Borg scale scores reached RPE >17 (very difficult), fatigue was considered to be occurred. Participants were not informed about the number of repetitions to avoid intentional halting to save energy.
After the fatigue protocol was completed, we repeated the measured lung test. 5 min were given for placing reflective markers on to the participants body. For each person, six repetition was requested, three trial included in the analysis, the flow chart is shown in Figure 2.
One lunge data period was defined as starting from the previous frame of the dominant leg contacting the force table to the end of the next stance position. By collecting kinematic and kinetic data from the hip, knee, and ankle joints in the sagittal and horizontal planes, as well as vertical ground reaction forces (vGRF), joint changes under fatigue were examined. Lunge can be divided lunges into five phases recommended by Kuntze et al. (2010). In this study we used the first and last peak of the ground reaction force as initial impact peak and drive-off peak (Figure 1).
Joint moment, vGRF, and muscular strength data were normalized to body weight, respectively. Joint angular data, moment, and vGRF data were time-normalized to lunge strides (1–101 points), then these strides were averaged in a group level.
The original EMG signal is first pre-processed, including 50 Hz trapping to remove the industrial frequency interference, then 30 Hz zero-phase-shift high-pass filtering to remove motion artefacts; finally, the signal is taken to its absolute value, and the negative half-axis of the signal is flipped to the positive half-axis. Processing EMG signals in the 10–500 Hz frequency range using a bandpass fourth-order Butterworth filter (Zhang et al., 2010). The amplitude analysis is carried out using root mean square (RMS) calculation. RMS values of the EMG signals were calculated with a window size of 50 m. Using the same method for the EMG signal at MVIC, EMG values during lunge were normalized to the peak MVIC activity for each corresponding muscles. The MVIC and standardized activity value of each action was output. The EMG activity was calculated from 0 (0, completely inactive) to 1 (100%, fully activated) through the test root mean square amplitude/MVIC root mean square amplitude.
Marker trajectories and ground reaction force were filtered by zero-latency fourth-order Butterworth low-pass filters at 12 and 30 Hz, respectively. A threshold of 20 N was used, and ground reaction force data to determine the instant of the ground contact. The filtered marker coordinate data were imported into OpenSim for data processing after being converted in Matlab R2018a (The MathWorks, MA, United States) to formats recognized by OpenSim 4.3 (.mot and. trc) (Zhou et al., 2021). The model Mei et al. (2022) used in this experiment was adapted from the original OpenSim model (Rajagopal et al., 2016) by Mei et al. (2022). The model was upgraded with additional ranges of motion in the coronal and horizontal planes of the knee joint. First, the model was scaled to the static calibration measurement data using the positions and weights of the subjects’ marker points. Marker positions were manually corrected to reduce RMS error value (less than 0.02) between the experimental and virtual markers in the model. In order to reduce the error between the experimental and virtual markers, the joint angles were estimated using the inverse kinematics (IK) calculation tool in OpenSim. The findings were then optimized using least squares. The net moments of the hip, knee, and ankle joints are determined calculating inverse dynamics (ID) with a built in OpenSim algorithm. In order to determine the net forces and torques for each joint that creates the motion, the ID tool solves the force and acceleration mathematical equations of classical mechanics in an inverse dynamics sense (Yamane et al., 2023). The next step involved estimating muscle forces and muscle activation using static optimization (SO) and minimization of the sum of squared muscle activity. The Delsys EMG test system measurements of standardized muscle electrical signals were used to validate the model, which was then compared to the OpenSim static optimization algorithm’s output of the degree of muscle activation. The quadriceps, hamstrings, gastrocnemius, and tibialis anterior muscles were among the major muscle groups whose forces were estimated using static optimization.
Data are presented as the Mean Difference and Standard Deviations (MD±SD). To check the normality of each dataset we used Shapiro-Wilk normality test. Paired samples t-tests were used to compare joint ROM, peak joint angles, peak joint moments, peak vGRF, and two peaks (A and B) of the lunge test before and after the fatigue protocol. The direction of the movement was also marked as follows: 1; flexion (+)-extension (−) in the sagittal plane and internal rotation (+)-external rotation (−) in the horizontal plane, 2; knee joint was flexion (+)-extension (−) in the sagittal plane and internal rotation (+)-external rotation (−) in the horizontal plane. 3; ankle sagittal plane was dorsiflexion (+) and plantarflexion (−). IBM SPSS Statistics 26 (IBM Corporation, Armonk, NY) was used to calculate all statistical analysis. The alpha level was set at 5%.
As can be seen in Table 1, there was a significant difference in the hip’s maximum angle both in the sagittal plane and horizontal plane before and after fatigue (p = 0.007 and p = 0.017), representing an increase of 4.38° and 3.35°. And hip minimum peak joint angle was significantly lower (p = 0.011) in the horizontal plane after fatigue. There was a 5.75° increase in ROM and a significant difference in maximal and ROM at the knee joint (both p = 0.001) in the sagittal plane. And for the knee in the horizontal plane after fatigue, the maximum value has, increased by 11.99°, a significantly higher (p < 0.001), ROM also differed significantly before and after fatigue (p = 0.001), with an increase of 10.32°. The maximum and minimum values in the ankle were significantly different (both p < 0.001). The ROM was nearly unchanged, while the maximum value decreased by 7.49° and the minimum value dropped by 7.74°.
TABLE 1. Hip, knee and ankle range of motion and maximum and minimum peak angles in the Sagittal plane and Horizontal plane before and after fatigue protocol.
As shown in Table 2, there was a significant difference in the peak sagittal plane moment at the hip joint before and after fatigue (p < 0.001), with an increase in moment of 0.39 Nm/kg. There was also a significant increase in the horizontal plane (p = 0.003) as well. The moment at the knee joint significantly decreased in the sagittal plane by 0.24 Nm/kg (p = 0.004). At the ankle joint, the sagittal plane moment was statistically significant after fatigue (p = 0.023). At the ankle joint, the post-fatigue sagittal moment was statistically significant (p = 0.023).
Table 3 shows that only the initial contact peak (A) for vGRF before and after fatigue had a statistically significant decline of 0.49 N/kg (p = 0.014). The mean peak and the drive-off peak (B) were not statistically significant, but the mean peak increases while the drive-off peak decreases.
According to Figure 3, muscle activity during lunge using the OpenSim SO tool matched the experimentally obtained EMG activity signal trends, proving that the data from the OpenSim model in this study is reliable (Hamner and Delp, 2013). When compared to muscle forces before fatigue (Figure 4), the biceps femoris and gastrocnemius both had lower average and maximal muscle force after fatigue protocol. The quadriceps and tibialis anterior muscles generated more force on average and at their maximal force output was also higher. The average muscle force of the tibialis anterior muscle was increased by 1.56 N/kg compared to after fatigue, it has a significant difference (p = 0.001); its maximum muscle force increased by 3.00 N/kg, also a significant difference, (p = 0.046). The mean muscle force of the biceps femoris decreased by 4.19 N/kg (p < 0.001). The maximum muscle force was significantly different, decreasing by 12.76 N/kg (p = 0.005). For the quadriceps, mean muscle force significantly increased by 13.16 N/kg (p = 0.002), and maximum muscle force increased by 19.78 N/kg (p < 0.001). Of the muscles explored, the quadriceps had the most significant increase in mean and maximum muscle force.
FIGURE 3. The comparison of gastrocnemius (A), biceps femoris (B), rectus femoris (C), and tibialis anterior (D) EMG activity levels obtained using the EMG signal and the estimated values from the OpenSim optimization algorithm.
FIGURE 4. Comparison of the average (A) and maximal (B) estimated muscle force during lunge before and after fatigue protocol. GA, gastrocnemius; TA, tibialis anterior; BF, biceps femoris; QF, quadriceps femoris. *, p < 0.05.
The aim of this study is to investigate the changes of lunge biomechanics before and after completing a fatigue protocol in healthy young men. Our results provide the necessary insights by using our objective data to understand which aspects of the lower limb are specifically affected by fatigue, and help to prevent the injuries associated with fatigue based on previous research. Fatigue needs to be taken into account as part of a risk assessment for injuries where internal workload, cardiovascular fitness, and fatigue interact (Benjaminse et al., 2019; Song et al., 2023). Running and forward lunge a chosen as the fatigue protocol for this study because it more accurately simulates the fatigue brought on by performing exercise repeatedly.
In both the sagittal and horizontal planes of the hip joint, according to our research, the peak angle increased in the sagittal plane and horizontal plane. On both planes, ROM also increased differently. Peak angle and ROM in the sagittal plane both significantly increased in the knee. The action of the knee joint and the motion of the hip joint during lunging are very similar. Greater knee flexion and forward trunk movement as a result of tiredness can also be used to explain increased hip flexion (Lin et al., 2015). The upper trunk’s swing is enhanced by fatigue, and limb movements are necessarily less effective as maintaining the trunk becomes difficult. Similar findings were made by Weeks et al. (2015) who hypothesized that fatigue increases hip flexion, pelvic tilt, inclination, and rotation as well as trunk flexion and lateral rotation. As a result of fatigue, fatigue causes the trunk and pelvis ROM become greater. The position of the body’s center of mass and the subsequent loading of the lower limb joints may be dramatically altered by the trunk because of its greater relative mass (Park et al., 2021). The peak knee joint angle and ROM increased significantly after the fatigue protocol compared to pre-fatigue in the sagittal plane. This shows that when we are exhausted or when the intensity of the exercise builds up over time, the knee joint tends to move farther forward from a vertical ground position. According to Zellmer et al. (2019), increased knee flexion may also increase the knee’s tendency to move farther forward. And he also finds, peak knee joint stress, quadriceps force, knee moment, and knee flexion are higher when the knee is brought in front of the toes during the lunge. Less stretching force is placed on the patellar tendon when the knee is kept behind the toes (Escamilla et al., 2008). The plantar flexor and foot muscles involved more to take transfer the additional stress required to maintain the joint output when the hip and knee muscles are working less efficiently due to the fatigue effect. In the horizontal plane, the knee joint found a considerable shift as well, showing an increase in peak angle and ROM. This outcome, in our opinion, may be a result of greater toe external rotation movement in the upright foot during landing and more forward movement of the knee. Because fatigue has been shown to lead to reduced control of the stabilizing muscles in the hip and knee joints, resulting in altered joint kinematics (Webster et al., 2012). Different degrees of knee flexion may result in different levels of sports performance and joint impact forces. Athletes with knee injuries increase knee flexion during the lunge to reduce the impact of landing on the joint and increase their dynamic stability center of mass by reducing their knee height (Huang et al., 2014). And Huang et al. (2014) noted that to reduce the likelihood of damage, athletes may flex their knees more and conduct less physically demanding activities. This may be a neuromuscular protective mechanism. If athletes want to improve performance, adding greater weights is the most popular and important method of resistance training (Comfort et al., 2015). In addition, the increased external weight causes more mechanical work to be done at the hip and ankle joints (Riemann et al., 2012), muscle activation was significantly higher in the weight-bearing condition than in the self-weight condition.
While joint ROM did not vary significantly in the ankle joint, the peak angle did, with the maximum joint angle falling by almost 7.49° and the minimal angle increasing by 7.74°. This indicates that following fatigue, the ankle joint is more likely to be at a more plantarflexed position before landing. According to previous study, a considerable 10° increase in ankle plantarflexion increases the risk of injury owing to calf muscle fatigue and foot overuse and may also produce fatigue and injury to the Achilles tendon and anterior heel ligaments (Mei et al., 2017). Participants showed reduced dorsiflexion, possibly as a result of fatigue, leading to an insufficient dorsiflexion muscular force generation and relatively high plantarflexion muscle force. Additionally, the vGRF can also be used as a measure for resistance training, which can be used to improve performance, reduce injury risk, and increase strength (Dempster et al., 2021). According to our finding, peak vGRF falls down after fatigue, and peak contact reduces down to around 0.49 N/kg. A larger portion of the heel contact the ground and a better transmission of forces across the ankle muscles when the heel touches the ground are made possible by higher ankle plantarflexion, which can possibly reduce the risk of injury.
In terms of joint moment, the peak hip moment increased significantly in the sagittal plane and horizontal plane, while at the knee joint it decreased in the sagittal plane and increased in the horizontal plane. The ankle joint showed a significant reduction in the moment. According to previous research (Kovács et al., 2023), an increase in moment at the hip joint was linked to a change in kinematics, including an increase in flexion angle brought on by forward body lean. Fatigue reduces the activation of the vastus lateralis muscles during the lunge and the knee moment in the upright leg during the lunge. The quadriceps, hamstrings, and gastrocnemius are the primary tibiofemoral joint motor muscles. These muscle groups make up nearly all of the cross-sectional area of the knee muscular tissue, or 98% (Wilson et al., 2008). The co-activation of the quadriceps and hamstrings, as well as the activation of the biceps femoris and rectus femoris, were unaffected by fatigue. When becoming fatigued, lateral femoral muscle activation may decrease and hamstring co-activation may rise. This strategy may be intended to reduce knee flexion by increasing resistance to diagonal joint motion because co-activation between the lateral femoral and biceps femoris muscles has a tendency to increase after fatigue (Longpré et al., 2015). (Longpré et al., 2015) speculated that a significant decrease in knee moments may result from the vastus lateralis and biceps femoris muscles’ ability to cooperate more when they are subjected to fatigued. An increase in plantarflexion and a decrease in the dorsiflexor muscles’ force may be responsible for the drop in ankle net moments. Du’s reported Du and Fan (2020) that there was a tendency for ankle plantarflexion to increase after fatigue, although the foot landed more flat position (i.e., greater contact area with the ground) at the ground. The findings of this study also indicate that better-performing athletes had stronger muscle strength and displayed higher knee and ankle moments, suggesting that the results of his experiment may be related to the participants’ varying levels of activity.
The forward lunge is a great exercise to stimulate lower limb extensor muscles, including the hip and knee extensor muscles (Ross, 1997). The average and maximum muscle force of the biceps femoris and gastrocnemius muscles decreased based on the estimation of the OpenSim SO calculation. The quadriceps and tibialis anterior muscles experienced significant increases in both their average and maximal muscular forces. The quadriceps muscle showed the highest increase in maximum force increase by a 19.78 N/kg. This demonstrates that the quadriceps muscle gets the most work done and it is the most active muscle during forward lunge. Running fatigue throughout the fatigue protocol may have been the source of the decline in biceps femoris muscle strength. Moreover, the maximum muscle force of the biceps femoris decreased by 12.76 N/kg. In contrast, the increased force in the tibialis anterior muscle may be the result of the knee joint moving more forward after exhaustion. For the aim of inducing localized tiredness in the quadriceps and other lower limb muscles, the forward lunge in the fatigue protocol was used, and this is what the study explored. The results of this study help us to determine which of the investigated muscles that are heavily involved in the lunge movement during the post-fatigue and furthermore it can help us to design more specific training exercise for these muscles. According to Baker et al. (1998), a variety of exercises at a moderate speed should be performed than for a high resistance strength training can be started in order to gain additional muscle strength and muscle mass. Although high level of fatigue is believed to enhance these aspects of muscle function, thus increasing the amount of fatigue that working muscles experience may benefit in improving strength and hypertrophy (Rooney et al., 1994).
The front lunge has the advantages of increasing safety when it is properly executed, preventing potential knee injuries, and building strong postural muscles (Wilson et al., 2008). Certain forms of the lunge can also be added into the fitness regime if you want to improve your fitness. These movements can help to improve the dynamic flexibility of the lower limb joints and aiding in muscle hypertrophy and force development. Importantly, they can also be used to prevent injuries so that augmentation training can be carried out effectively and safely (Tippett and Voight, 1995). In general, it is important to perform all relevant exercises according to your condition to avoid unnecessary injuries caused by fatigue.
The methods we used in this study has limitation which needs to be addressed. First of all, although the biomechanical alterations in the forward lunge before and after fatigue protocol were investigated, they were not compared between different fatigue states. Secondly, The SO uses known movements of the model to solve for muscle activation forces, so the results may differ from the EMG test system, this means that there are limitations of the muscle force estimation we used. Thirdly, there is regional difference in muscle EMG activity and the location of measurements is not always the same for each participant, so EMG is also limited. Finally, we only tested the healthy young men, and we did not test other populations such as the athletic and injured populations, besides the protocol is limited.
In this study, we discovered that forward lung is affected by fatigue. After fatigue, the hip and knee joints have a greater ROM in the sagittal plane and an increased offset in the horizontal plane, with a tendency towards forward leaning. In addition to an increased amount of plantarflexion muscle activity in the ankle and unstable body postural performance. Additionally, the quadriceps muscles are the most affected after fatigue. To avoid injuries due to instability after fatigue, training people should focus more on strength training of the hip and knee muscles groups as a way to control joint motion more effectively.
The raw data supporting the conclusion of this article will be made available by the authors, without undue reservation.
The studies involving human participants were reviewed and approved by the Ningbo University. The patients/participants provided their written informed consent to participate in this study.
All the authors contributed substantially to the manuscript. LG, JY, KB, and YG were responsible for the conceptualization. LG, JY, ZM, and ZR were responsible for investigation and methodology. LG, JY, KB, and YG were responsible for formal analysis and writing–original draft. JY, ZR, ZM, and YG were responsible for writing–review and editing and supervision. All authors contributed to the article and approved the submitted version.
This study was sponsored by the Zhejiang Provincial Natural Science Foundation of China for Distinguished Young Scholars (LR22A020002), Zhejiang Provincial Key Research and Development Program of China (2021C03130), Zhejiang Provincial Natural Science Foundation (LTGY23H040003), Ningbo key R&D Program (2022Z196), Research Academy of Medicine Combining Sports, Ningbo (No. 2023001), the Project of NINGBO Leading Medical and Health Discipline (No. 2022-F15 and No. 2022-F22), Ningbo Natural Science Foundation (20221JCGY010532 and 20221JCGY010607), Public Welfare Science and Technology Project of Ningbo, China (2021S134), and K. C. Wong Magna Fund in Ningbo University, Zhejiang Rehabilitation Medical Association Scientific Research Special Fund (ZKKY2023001).
The authors declare that the research was conducted in the absence of any commercial or financial relationships that could be construed as a potential conflict of interest.
All claims expressed in this article are solely those of the authors and do not necessarily represent those of their affiliated organizations, or those of the publisher, the editors and the reviewers. Any product that may be evaluated in this article, or claim that may be made by its manufacturer, is not guaranteed or endorsed by the publisher.
Amin, S., Goggins, J., Niu, J., Guermazi, A., Grigoryan, M., Hunter, D. J., et al. (2008). Occupation-related squatting, kneeling, and heavy lifting and the knee joint: a magnetic resonance imaging-based study in men. J. rheumatology 35 (8), 1645–1649.
Baker, D., Coach, H. S., League, A. U., and Team, P. (1998). Designing, implementing and coaching strength training programs for beginners and intermediate level athletes-part 1: designing the program. Strength Cond. Coach Champaign 5, 11–20.
Benjaminse, A., Webster, K. E., Kimp, A., Meijer, M., and Gokeler, A. (2019). Revised approach to the role of fatigue in anterior cruciate ligament injury prevention: a systematic review with meta-analyses. Sports Med. 49, 565–586. doi:10.1007/s40279-019-01052-6
Bliss, A., and Dekerle, J. (2019). Reciprocal versus nonreciprocal assessment of knee flexors and extensors in concentric actions using the CON-trex multijoint isokinetic dynamometer: a reliability study. Meas. Phys. Educ. Exerc. Sci. 23 (2), 118–123. doi:10.1080/1091367x.2018.1550087
Borg, G. A. (1982). Psychophysical bases of perceived exertion. Med. Sci. Sports Exerc 14, 377–381. doi:10.1249/00005768-198205000-00012
Comfort, P., Jones, P. A., Smith, L. C., and Herrington, L. (2015). Joint kinetics and kinematics during common lower limb rehabilitation exercises. J. Athl. Train. 50 (10), 1011–1018. doi:10.4085/1062-6050-50.9.05
Dempster, J., Dutheil, F., and Ugbolue, U. C. (2021). The prevalence of lower extremity injuries in running and associated risk factors: a systematic review. Phys. Activity Health 5 (1), 133–145. doi:10.5334/paah.109
Dregney, T. M., Thul, C. M., Linde, J. A., and Lewis, B. A. (2023). Affective responses to high intensity interval training relative to moderate intensity continuous training. Phys. Activity Health 7 (1), 229–238. doi:10.5334/paah.271
Du, Y., and Fan, Y. (2020). Changes in the kinematic and kinetic characteristics of lunge footwork during the fatiguing process. Appl. Sci. 10 (23), 8703. doi:10.3390/app10238703
Ebben, W., Feldmann, C., Dayne, A., Mitsche, D., Alexander, P., and Knetzger, K. (2009). Muscle activation during lower body resistance training. Int. J. sports Med. 30 (01), 1–8. doi:10.1055/s-2008-1038785
Escamilla, R. F., Zheng, N., MacLeod, T. D., Edwards, W. B., Hreljac, A., Fleisig, G. S., et al. (2008). Patellofemoral joint force and stress between a short-and long-step forward lunge. J. Orthop. sports Phys. Ther. 38 (11), 681–690. doi:10.2519/jospt.2008.2694
Faul, F., Erdfelder, E., Buchner, A., and Lang, A. G. (2009). Statistical power analyses using G* power 3.1: tests for correlation and regression analyses. Behav. Res. methods 41 (4), 1149–1160. doi:10.3758/BRM.41.4.1149
Gandevia, S., Allen, G., and McKenzie, D. (2006). Central fatigue. Fatigue: Springer, 281–294. doi:10.2165/00007256-200636100-00006
Garcia, G. R. L., Sousa, N. M. F. d., Arakelian, V. M., Garcia, J. F., Marson, R. A., Perez, S. E. D. A., et al. (2012). Thigh muscles electromyographic activity during lunge exercise to fatigue. Rev. Bras. de Cineantropometria Desempenho Hum. 14, 83–92. doi:10.5007/1980-0037.2012v14n1p83
Goulette, D., Griffith, P., Schiller, M., Rutherford, D., and Kernozek, T. W. (2021). Patellofemoral joint loading during the forward and backward lunge. Phys. Ther. Sport 47, 178–184. doi:10.1016/j.ptsp.2020.12.001
Gribble, P., Hertel, J., and Denegar, C. (2007). Chronic ankle instability and fatigue create proximal joint alterations during performance of the Star Excursion Balance Test. Int. J. sports Med. 28 (03), 236–242. doi:10.1055/s-2006-924289
Griffin, T. M., and Guilak, F. (2005). The role of mechanical loading in the onset and progression of osteoarthritis. Exerc. sport Sci. Rev. 33 (4), 195–200. doi:10.1097/00003677-200510000-00008
Hamner, S. R., and Delp, S. L. (2013). Muscle contributions to fore-aft and vertical body mass center accelerations over a range of running speeds. J. biomechanics 46 (4), 780–787. doi:10.1016/j.jbiomech.2012.11.024
Herbaut, A., Delannoy, J., and Foissac, M. (2018). Injuries in French and Chinese regular badminton players. Sci. Sports 33 (3), 145–151. doi:10.1016/j.scispo.2018.02.001
Hermens, H. J., Freriks, B., Disselhorst-Klug, C., and Rau, G. (2000). Development of recommendations for SEMG sensors and sensor placement procedures. J. Electromyogr. Kinesiol. 10 (5), 361–374. doi:10.1016/s1050-6411(00)00027-4
Huang, M. T., Lee, H. H., Lin, C. F., Tsai, Y. J., and Liao, J. C. (2014). How does knee pain affect trunk and knee motion during badminton forehand lunges? J. sports Sci. 32 (7), 690–700. doi:10.1080/02640414.2013.848998
Irish, S. E., Millward, A. J., Wride, J., Haas, B. M., and Shum, G. L. (2010). The effect of closed-kinetic chain exercises and open-kinetic chain exercise on the muscle activity of vastus medialis oblique and vastus lateralis. J. Strength and Cond. Res. 24 (5), 1256–1262. doi:10.1519/JSC.0b013e3181cf749f
Keogh, J. (1999). Lower-body resistance training: increasing functional performance with lunges. Strength and Cond. J. 21 (1), 67. doi:10.1519/1533-4295(1999)021<0067:lbrtif>2.0.co;2
Kondrič, M., Matković, B., Furjan-Mandić, G., Hadžić, V., and Dervišević, E. (2011). Injuries in racket sports among Slovenian players. Coll. Antropol. 35 (2), 413–417.
Kovács, B., Csala, D., Sebestyén, Ö., Matlák, J., Groszmann, Á., Tihanyi, J., et al. (2023). Arm swing during vertical jumps does not increase EMG activity of the lower limb muscles. Phys. Activity Health 7 (1), 132–142. doi:10.5334/paah.263
Kuntze, G., Mansfield, N., and Sellers, W. (2010). A biomechanical analysis of common lunge tasks in badminton. J. sports Sci. 28 (2), 183–191. doi:10.1080/02640410903428533
Lin, C. F., Hua, S. H., Huang, M. T., Lee, H. H., and Liao, J. C. (2015). Biomechanical analysis of knee and trunk in badminton players with and without knee pain during backhand diagonal lunges. J. Sports Sci. 33 (14), 1429–1439. doi:10.1080/02640414.2014.990492
Liu, Z., Yang, C., Yu, J., Zhao, X., Wu, J., Zhang, Y., et al. (2023). The effect of muscles fatigue on the knee’s kinetics and kinematics characteristics. Sustainability 15 (4), 3029. doi:10.3390/su15043029
Longpré, H. S., Acker, S. M., and Maly, M. R. (2015). Muscle activation and knee biomechanics during squatting and lunging after lower extremity fatigue in healthy young women. J. Electromyogr. Kinesiol. 25 (1), 40–46. doi:10.1016/j.jelekin.2014.08.013
McLean, S. G., and Samorezov, J. E. (2009). Fatigue-induced ACL injury risk stems from a degradation in central control. Med. Sci. Sports Exerc. 41 (8), 1661–1672. doi:10.1249/MSS.0b013e31819ca07b
Mei, Q., Fernandez, J., Xiang, L., Gao, Z., Yu, P., Baker, J. S., et al. (2022). Dataset of lower extremity joint angles, moments and forces in distance running. Heliyon 8 (11), e11517. doi:10.1016/j.heliyon.2022.e11517
Mei, Q., Gu, Y., Fu, F., and Fernandez, J. (2017). A biomechanical investigation of right-forward lunging step among badminton players. J. Sports Sci. 35 (5), 457–462. doi:10.1080/02640414.2016.1172723
Nielsen, M. H., Lund, J. N., Lam, W. K., and Kersting, U. G. (2018). Differences in impact characteristics, joint kinetics and measurement reliability between forehand and backhand forward badminton lunges. Sports Biomech. 19, 547–560. doi:10.1080/14763141.2018.1501086
Park, S., Huang, T., Song, J., Lee, M., Lee, Y. S., Kim, J. H., et al. (2021). Diversity and antimicrobial resistance in the Streptococcus bovis/Streptococcus equinus complex (SBSEC) isolated from Korean domestic ruminants. Phys. Ther. Rehabilitation Sci. 10 (2), 98–105. doi:10.3390/microorganisms9010098
Phomsoupha, M., and Laffaye, G. (2015). The science of badminton: game characteristics, anthropometry, physiology, visual fitness and biomechanics. Sports Med. 45 (4), 473–495. doi:10.1007/s40279-014-0287-2
Pincivero, D. M., Aldworth, C., Dickerson, T., Petry, C., and Shultz, T. (2000). Quadriceps-hamstring EMG activity during functional, closed kinetic chain exercise to fatigue. Eur. J. Appl. physiology 81 (6), 504–509. doi:10.1007/s004210050075
Rajagopal, A., Dembia, C. L., DeMers, M. S., Delp, D. D., Hicks, J. L., and Delp, S. L. (2016). Full-body musculoskeletal model for muscle-driven simulation of human gait. IEEE Trans. Biomed. Eng. 63 (10), 2068–2079. doi:10.1109/TBME.2016.2586891
Richmond, S. A., Fukuchi, R. K., Ezzat, A., Schneider, K., Schneider, G., and Emery, C. A. (2013). Are joint injury, sport activity, physical activity, obesity, or occupational activities predictors for osteoarthritis? A systematic review. J. Orthop. sports Phys. Ther. 43 (8), B515–B519. doi:10.2519/jospt.2013.4796
Riemann, B. L., Lapinski, S., Smith, L., and Davies, G. (2012). Biomechanical analysis of the anterior lunge during 4 external-load conditions. J. Athl. Train. 47 (4), 372–378. doi:10.4085/1062-6050-47.4.16
Rooney, K. J., Herbert, R. D., and Balnave, R. J. (1994). Fatigue contributes to the strength training stimulus. Med. Sci. sports Exerc. 26 (9), 1160–1164. doi:10.1249/00005768-199409000-00014
Ross, M. D. (1997). Using closed kinetic chain tests to determine functional performance levels. Strength and Cond. J. 19 (5), 66–69. doi:10.1519/1073-6840(1997)019<0066:uckctt>2.3.co;2
Shariff, A., George, J., and Ramlan, A. (2009). Musculoskeletal injuries among Malaysian badminton players. Singap. Med. J. 50 (11), 1095–1097.
Song, Y., Cen, X., Chen, H., Sun, D., Munivrana, G., Bálint, K., et al. (2023). The influence of running shoe with different carbon-fiber plate designs on internal foot mechanics: a pilot computational analysis. J. Biomech. 153, 111597. doi:10.1016/j.jbiomech.2023.111597
Stastny, P., Lehnert, M., Zaatar, A. M., Svoboda, Z., and Xaverova, Z. (2015a). Does the dumbbell-carrying position change the muscle activity in split squats and walking lunges? J. strength Cond. Res. 29 (11), 3177–3187. doi:10.1519/JSC.0000000000000976
Stastny, P., Tufano, J. J., Lehnert, M., Golas, A., Zaatar, A., Xaverova, Z., et al. (2015b). Hip abductors and thigh muscles strength ratios and their relation to electromyography amplitude during split squat and walking lunge exercises. Acta Gymnica 45, 51–59. doi:10.5507/ag.2015.011
Tippett, S. R., and Voight, M. L. (1995). Functional progressions for sport rehabilitation. Champaign, IL, United States: Human Kinetics.
Webster, K. E., Santamaria, L. J., McClelland, J. A., and Feller, J. A. (2012). Effect of fatigue on landing biomechanics after anterior cruciate ligament reconstruction surgery. Med. Sci. sports Exerc. 44 (5), 910–916. doi:10.1249/MSS.0b013e31823fe28d
Weeks, B. K., Carty, C. P., and Horan, S. A. (2015). Effect of sex and fatigue on single leg squat kinematics in healthy young adults. BMC Musculoskelet. Disord. 16 (1), 271–279. doi:10.1186/s12891-015-0739-3
Weir, J. P., Keefe, D. A., Eaton, J. F., Augustine, R. T., and Tobin, D. M. (1998). Effect of fatigue on hamstring coactivation during isokinetic knee extensions. Eur. J. Appl. physiology Occup. physiology 78, 555–559. doi:10.1007/s004210050460
Wilson, D. J., Gibson, K., and Masterson, G. L. (2008). Kinematics and kinetics of 2 styles of partial forward lunge. J. sport rehabilitation 17 (4), 387–398. doi:10.1123/jsr.17.4.387
Winby, C. R., Lloyd, D. G., Besier, T. F., and Kirk, T. B. (2009). Muscle and external load contribution to knee joint contact loads during normal gait. J. biomechanics 42 (14), 2294–2300. doi:10.1016/j.jbiomech.2009.06.019
Yamane, T., Yamasaki, Y., Nakashima, W., and Morita, M. (2023). Tri-axial accelerometer-based recognition of daily activities causing shortness of breath in COPD patients. Phys. Activity Health 7 (1), 64–75. doi:10.5334/paah.224
Zellmer, M., Kernozek, T. W., Gheidi, N., Hove, J., and Torry, M. (2019). Patellar tendon stress between two variations of the forward step lunge. J. Sport Health Sci. 8 (3), 235–241. doi:10.1016/j.jshs.2016.12.005
Zhang, X., Wang, Y., and Han, R. P. (2010). “Wavelet transform theory and its application in EMG signal processing,” in 2010 Seventh International Conference on Fuzzy Systems and Knowledge Discovery, Yantai, China, 10-12 August 2010 (IEEE), 2234–2238. doi:10.1109/FSKD.2010.5569532
Keywords: forward lunge, kinetics, kinematics, fatigue, lower limb
Citation: Gao L, Ye J, Bálint K, Radak Z, Mao Z and Gu Y (2023) Biomechanical effects of exercise fatigue on the lower limbs of men during the forward lunge. Front. Physiol. 14:1182833. doi: 10.3389/fphys.2023.1182833
Received: 09 March 2023; Accepted: 25 July 2023;
Published: 17 August 2023.
Edited by:
Erika Zemková, Comenius University, SlovakiaReviewed by:
Petr Stastny, Charles University, CzechiaCopyright © 2023 Gao, Ye, Bálint, Radak, Mao and Gu. This is an open-access article distributed under the terms of the Creative Commons Attribution License (CC BY). The use, distribution or reproduction in other forums is permitted, provided the original author(s) and the copyright owner(s) are credited and that the original publication in this journal is cited, in accordance with accepted academic practice. No use, distribution or reproduction is permitted which does not comply with these terms.
*Correspondence: Zhuqing Mao, bWFvemh1cWluZ0BuYnUuZWR1LmNu; Yaodong Gu, Z3V5YW9kb25nQG5idS5lZHUuY24=
Disclaimer: All claims expressed in this article are solely those of the authors and do not necessarily represent those of their affiliated organizations, or those of the publisher, the editors and the reviewers. Any product that may be evaluated in this article or claim that may be made by its manufacturer is not guaranteed or endorsed by the publisher.
Research integrity at Frontiers
Learn more about the work of our research integrity team to safeguard the quality of each article we publish.