- 1State Key Laboratory of Crop Gene Exploration and Utilization in Southwest China, Sichuan Agricultural University, Chengdu, China
- 2College of Agriculture, Sichuan Agricultural University, Chengdu, China
- 3College of Plant Protection, Nanjing Agricultural University, Nanjing, China
- 4Key Laboratory of Integrated Pest Management on Crops in Southwest, Sichuan Academy of Agricultural Sciences, Ministry of Agriculture, Institute of Plant Protection, Chengdu, China
- 5Talent Development Service Center, Sichuan Provincial Department of Agriculture and Rural Affairs, Chengdu, China
- 6Department of Entomology, China Agricultural University, Beijing, China
The fall armyworm, Spodoptera frugiperda (Noctuidae: Lepidoptera), is a wide-reaching notorious insect pest of important cereal crops, which has developed resistance to multiple classes of insecticides. It invaded the Sichuan Province of China in 2019. In this study, we performed resistance monitoring of insecticides for 11 field-collected populations from Sichuan, and all the populations were susceptible to emamectin benzoate and chlorpyrifos. The variations in resistance level to indoxacarb (resistance ratio (RR), 9.23–45.53-fold), spinetoram (RR, 4.32–18.05-fold), and chlorantraniliprole (RR, 2.02–10.39-fold) were observed among these populations. To investigate the resistance mechanism of chlorantraniliprole, synergism tests were performed and showed that piperonyl butoxide had a slight synergistic effect on chlorantraniliprole for the QJ-20 population (1.43-fold) in moderate resistance (RR, 10.39-fold) compared with the treatment group without synergist. Furthermore, the expression scanning for resistance-related genes showed that five P450 genes (CYP6AE43, CYP321A8, CYP305A1, CYP49A1, and CYP306A1) and the ryanodine receptor gene (Ryr, chlorantraniliprole target) were overexpressed in the QJ-20 population. These results indicated that the fall armyworm in Sichuan has exhibited diverse susceptibilities to several classes of insecticides, and the overexpression of Ryr and several P450 genes may contribute to the development of resistance in S. frugiperda to chlorantraniliprole.
1 Introduction
The fall armyworm (FAW), Spodoptera frugiperda (Noctuidae: Lepidoptera), is an instinctive pest of the tropical regions of the continents of North and South America, which has rapidly spread over a large area (Sparks et al., 1979). Being polyphagous, it is a notorious pest to more than 350 plant species, including maize, cotton, sugarcane, wheat, sorghum, rice, tomato, beet, and pasture grasses (Montezano et al., 2018). There are two host plant strains of this insect pest, the “corn-biotype” (C-biotype) mostly feeds on maize, sorghum, and cotton, while the “rice-biotype” (R-biotype) mostly targets rice and many other pasture grasses (Dumas et al., 2015).
Among the control methods for agricultural insect pests, chemical control is widely preferable in the farming community across the world. Common pesticides include organophosphorus, carbamate, pyrethroid, and benzoylurea insecticides, which have been supplemented by newer insecticides (indoxacarb, chlorantraniliprole, and emamectin benzoate) in recent years. Owing to the overuse of insecticide, the resistance ratios (RRs) of several FAW populations collected from Mexico and Puerto Rico to permethrin, chlorpyrifos, chlorantraniliprole, and flubendiamide were up to 500-fold in 2016 and were similar for methomyl, deltamethrin, and cypermethrin (Gutierrez et al., 2019). It was reported that the FAW populations invading China carried resistance to the pyrethroids and organophosphates insecticides (Zhang B. et al., 2020). However, most FAW populations in China were found to be susceptible to emamectin benzoate, chlorantraniliprole, spinetoram, indoxacarb, lambda-cyhalothrin, and acephate (Wang et al., 2022). The FAW had no previous record of being resistant to chlorantraniliprole during 2019–2021 (Liu et al., 2022; Wang et al., 2022). However, compared with the 2019 field-collected population, several FAW field populations (collected during 2020) showed slightly reduced toxicity in chlorantraniliprole (Wang et al., 2022), which is a broad spectrum, has high insecticidal efficacy, and is capable of controlling lepidopteran pests. Meanwhile, using less-harmful insecticides inappropriately for a brief period of time in the field would enhance the selection pressure on the target pests and raise the probability of resistance development (Li et al., 2021). The key method by which the FAW develops insecticide resistance is the enhanced detoxifying metabolism of pesticides, which is primarily caused by inadequate pesticide treatment. Previous studies on the FAW revealed that the overexpression of glutathione S-transferases (GSTs), cytochrome P450s (P450s), and esterases (ESTs) was mainly involved in developing resistance against pyrethroids, organophosphorus, and carbamate pesticides (Liu et al., 2022). In this regard, the application of different synergists can also be helpful to compare their activities under different conditions as these are crucial in the development of resistance against insecticides (Mohan and Gujar, 2003). There are numerous studies on general P450 functions in pesticide resistance. According to reports, insecticide adaptability is associated with known detoxification families, like P450 monooxygenases (Feyereisen et al., 2012). Comparative genomics analysis displayed that the cytochrome P450 gene family has vastly expanded to 425 members in the FAW, of which 283 genes are specific to the FAW (Gui et al., 2022). A comparison of the expression levels of several cytochrome P450 genes using quantitative real-time PCR (qRT-PCR) in the field-collected populations could also be helpful to understand the nature of the resistance mechanism (Lao et al., 2015). According to certain studies, the overexpression of some P450 genes may be the primary cause of the increase in P450 activity, which can contribute to the FAW’s resistance to chlorantraniliprole (Gong et al., 2013; Elzaki et al., 2016). The transcriptomic and genomic studies of the FAW revealed that approximately 117–425 P450 genes in the FAW, including CYP321A8, CYP321A9, and CYP321B1, may play a critical role in insecticide detoxification and, thus, might be involved in pesticide resistance (Zhang D. D. et al., 2020; Chen and Palli, 2021).
There is currently limited literature on resistance monitoring in the FAW from China. It is urgently necessary to gain scientific and practical knowledge about resistance monitoring and to comprehend the underlying mechanisms of insecticide resistance in the FAW to design Integrated Pest Management (IPM) or Insect Resistance Management (IRM). In this study, we examined the resistance levels of 11 S. frugiperda field populations from Sichuan Province against emamectin benzoate, chlorpyrifos, spinetoram, chlorantraniliprole, and indoxacarb. In addition, we determined the synergistic effects of three different synergists and compared the detoxification enzymes’ activities under different treatments. Moreover, we observed the relative expression levels for 26 resistance-related genes (one ryanodine receptor gene (Ryr) and 25 P450 genes) in the field population by qRT-PCR. Therefore, the results of our study may be useful in planning appropriate management strategies for the resistant field populations of the FAW, mainly against chlorantraniliprole, and provide a theoretical basis for exploring the extent of resistance and its mechanism against different insecticides used for controlling S. frugiperda.
2 Materials and methods
2.1 Insects
The 11 populations of the FAW were collected from different places in Sichuan Province, China, in 2019–2022 (Figure 1). The larvae were reared on artificially prepared food. The newly hatched larvae were shifted into small boxes containing food, and when they reached the third instar, they were shifted individually into small glass tubes with artificial food to avoid cannibalism, which is highly reported in the FAW (Chapman et al., 2001). The adults were reared on a 10% honey water solution. The newly laid eggs and pupae were treated with sodium hypochlorite disinfection solution (0.2–0.3%) to avoid any contamination (Wang et al., 2018). All the developmental stages were kept under the controlled conditions of 70–80% relative humidity (RH), 26 ± 1 °C temperature, and a 16:8 h (L:D) photoperiod.
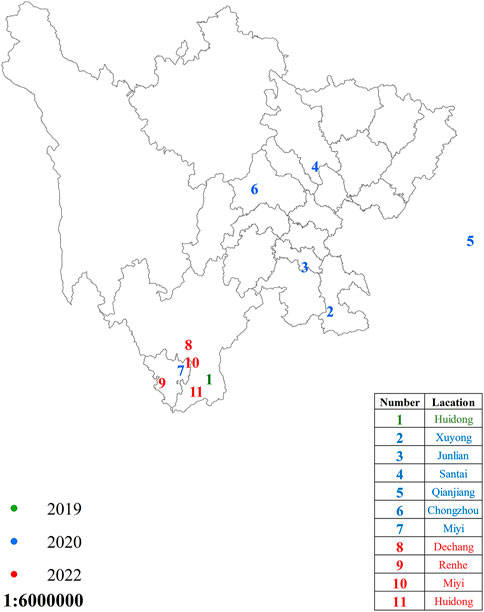
FIGURE 1. Spodoptera frugiperda sampling regions in Sichuan Province, China. The dots in green, blue, and red represent areas where S. frugiperda were sampled during the years 2019, 2020, and 2022, respectively.
2.2 Insecticides and chemicals
Five different insecticides were used in this research, including 74% emamectin benzoate (Nanjing Red Sun Co., Ltd., Gaochun, China), 97% chlorpyrifos (Hubei Sharonda Co., Ltd., Jingzhou, China), 95% chlorantraniliprole (Corteva Agriscience, Indianapolis, United States), 95% indoxacarb (Jiangsu Flag Chemical Industry Co., Ltd., Nanjing, China), and 99% spinetoram (Wuhan Xiyu Biotechnology Co., Ltd., Hubei, China). The synergists included diethyl maleate (DEM), piperonyl butoxide (PBO), and triphenyl phosphate (TPP), which were bought from Aladdin Shanghai Co., Ltd. (Nanjing, China). α-Naphthyl acetate (α-NA), 1-chloro-2,4-dinitrobenzene (CDNB), and fast blue salt B were purchased from Chengdu Ai Keda Chemical Technology Co., Ltd. (Chengdu, China). Bovine serum albumin (BSA), NADPH, DTT, PMSF, and reduced glutathione (GSH) were purchased from Beijing Solarbio Science and Technology Co., Ltd. (Beijing, China). The Coomassie brilliant blue G 250, EDTA Na2, and sodium dodecyl sulfate (SDS) were bought from Chengdu Kelong Chemical Reagent Co., Ltd. (Chengdu, China).
2.3 Bioassays
For the bioassays, the topical application method was used for 2 days after the third instar larva molting (Wang et al., 2021). There were three replications for each concentration with 12 larvae per replication. The stock solutions for all insecticides were prepared using acetone or dimethyl sulfoxide and then serially diluted to make five or six concentrations of each, to cover mortality from 0 to 100%. A 50 μL microsyringe (Hamilton company, Reno, NV) coupled with a microapplicator (PB600-1 Repeating Dispenser, Hamilton Company) was used to apply 1 μL of each prepared concentration over the dorsal side of the frontal thorax of the third instar larva, as reported in previous reports (Brewer and Trumble, 1989; Mccaffery et al., 1991). We selected the 23rd instar larvae in the same condition to weigh and finally figure out 0.006 g/larva. One microliter of acetone per larva was applied or dimethyl sulfoxide was used for the control treatment. The treated larvae were transferred in 12-compartment culture plates, containing a small quantity of food. Each culture plate was considered one replication. The mortality was assessed 24 h after treatment. If they showed severe intoxication symptoms, including feeding cessation, severe growth inhibition or slow movement, and twitching when touched with a small brush, the larvae were considered dead.
The toxicity of synergists including DEM, TPP, and PBO with chlorantraniliprole was determined for the QJ-20 population using topical application, as previously mentioned. All these synergists were dissolved in acetone at a concentration of 1000 mg/L (Sun et al., 2018). After this, a 1.0 µL droplet was applied over the thoracic dorsum of each third instar larva using a microsyringe coupled with a microapplicator (PB600-1 Repeating Dispenser, Hamilton Company). After an hour of synergist application, the different concentrations of insecticide were applied over larvae, as described previously, and mortality was observed 24 h after treatment.
2.4 Enzymatic assays
The activities of CarEs, GSTs, and P450s were determined for the three experimental groups including the QJ-20 population, the QJ-20 population treated only with a synergist, and the QJ-20 population treated with LD25 of chlorantraniliprole plus a synergist (TPP or DEM or PBO) (Liu et al., 2022). The fifth instar larvae were selected, and their midguts were dissected over dry ice packs (Wang et al., 2018). These midguts were first put in 1.15% ice-cold KCl solution to isolate the excessive fatty material and were then transferred to a 1.5 mL centrifuge tube, labeled, and stored at −80 °C as quickly as possible.
The determination of CarEs activity was made according to Van Asperen (1962). First, 1 mL of buffer solution (0.04 mol/L phosphate buffer, pH 7.0) was added in a 5 mL glass homogenizer to homogenize the midguts on ice, and then, centrifugation (10000 ×g for 10 min at 4 °C) was performed, using a 5417R centrifuge (Eppendorf, Germany). Later, the supernatant was transferred to a clean tube, considered an enzyme source, and then, 1.8 mL of α-NA solution (having 3 × 10−4 mol/L physostigmine), 0.45 mL of buffer solution (0.04 mol/L, pH 7.0), and 50 μL of enzyme source were put in the 4 mL centrifuge tube and incubated in a water bath for 15 min at 30 °C. Next, 0.9 mL of staining solution (with 0.2 g of fast blue salt B in 20 mL distilled water plus 50 mL of 5% SDS) was added to stop the reaction. The changes in absorbance values were recorded (after 5 min of calibration with blank) at a wavelength of 600 nm, using the UV 2000 spectrophotometer (Unic Instruments Incorporated, Shanghai, China). The CarEs activity was expressed as millimoles of naphthol per minute per milligram of protein and calculated from the production of α-naphthol by referring to an experimentally determined standard curve.
The GST activity was determined according to Habig and Jakoby (1981), for which the midguts of the fifth instar larvae were first homogenized over ice using 1 mL of buffer (0.1 mol/L phosphate buffer, having 1 mmol/L EDTA, pH 6.5), and the supernatant was transferred to the new tube after centrifugation at 10800 ×g for 10 min at 4 °C, the supernatant was considered as an enzyme source. Next, 90 µL of CDNB (15 mmol/L), 90 µL of reduced GSH (30 mmol/L), 2470 µL of phosphate buffer (0.1 mol/L, pH 6.5), and 50 µL of enzyme source were mixed in a 4 mL tube. The OD value was recorded for 2 min at 340 nm and calculated as △A340/min.
The method of Rose et al. (1995) was used to determine the activity of cytochrome P450s ethoxycoumarin O-deethylase (ECOD), with some modifications. The enzyme source was the supernatant of the homogenized midguts of the fifth instar larvae, obtained by adding 1 mL of buffer (0.1 mol/L phosphate buffer, pH 7.5, having 20% glycerol, 0.1 mmol/L DTT, 0.1 mmol/L EDTA, and 0.4 mmol/L PMSF), in a 5 mL glass homogenizer over the ice, and centrifugation was conducted at 10000 ×g for 15 min at 4 °C. Later, 90 μL of enzyme source was mixed with 100 μL of 2 mmol/L 4-nitroanisole and put into the culture plate and incubated at 27 °C for 3 min in the water bath. After this, 10 μL of 9.6 mmol/L NADPH was added to initiate the reaction, and the absorbance values at 405 nm for 2 min with 20 s intervals at 27 °C were determined using the microplate reader (Model 680 Microplate Reader, Bio-Rad).
The method of Bradford (1976) was used to determine the total protein content, considering BSA as a standard. A test solution of 3 mL was prepared by adding a certain prepared concentration of BSA with 2.5 mL of Coomassie Brilliant blue to measure the OD value at a wavelength of 595 nm using the UV 2000 Spectrophotometer (Unic Instruments Incorporated, Shanghai, China) to get the standard curve.
2.5 qRT-PCR
A total of 26 genes were chosen from Giraudo et al. (2014) and the NCBI database to determine which genes contribute to the development of the FAW’s resistance to chlorantraniliprole, and we designed their corresponding primers using Primer-BLAST on the NCBI website (Supplementary Table S1). The midgut of the fifth instar larvae was obtained by dissection on dry ice packs (Wang et al., 2018). RNA was examined, and cDNA synthesis and sequencing of genes were carried out. Due to the lack of a susceptible strain, the relative expressions of all the genes were later discovered in the QJ-20 population, a moderately resistant population (among the 11 populations), using the comparatively sensitive population (HD-19) of S. frugiperda as the control. Six higher-expression genes were screened out and were further determined in the six field populations collected in 2020 using qRT-PCR.
The fifth instar larvae were selected for the extraction of RNA using RNase Easy Water (Vazyme Biotech Ltd., China) according to the manufacturer’s instructions, and later, the concentrations of RNA were measured using the Micro-Drop ultra microspectrophotometer (Baoyide Scientific Ltd., Shanghai, China). The Novoscript® plus all-in-one reagent kit (Novoprotein Scientific Inc., Shanghai, China) was used to prepare cDNA from 1 μg total RNA. The Applied Biosystems 7500 RT-PCR system (Applied Biosystems, Foster City, CA, United States) was used to perform qRT-PCR using the 2 × NovoStart® SYBRqPCR SuperMix Plus kit (Novoprotein Scientific Inc.). The total volume of the reaction mixture was 20 μL with 10 μL of 2 ×SuperMix, 1.5 μL of each primer, 1 μL of cDNA template, and 6 μL of RNase-free water. The protocol program was as follows: 95 °C for 1 min, followed by 40 cycles of 95°C for 20 s, 60°C for 20 s, and 72°C for 30 s. The reference gene was the elongation factor 1 alpha (EF1α) for S. frugiperda (Shu et al., 2020), and the experiment was performed for three technical replicates with three independent biological replicates. The 2−ΔΔCt method (Livak and Schmittgen, 2001) was used for calculating the relative expression levels of target genes.
2.6 Data analysis
The POLO 2.0 program (Leora Software, www.leorasoftware.com) was used to calculate the slope, LD50, 95% confidence intervals (CI), and chi-square (χ2) value of each insecticide 24 h after treatment (Wang et al., 2018). RRs were calculated using the susceptible baseline values for indoxacarb, emamectin benzoate, chlorantraniliprole, and spinetoram as the factor divisor from the result by Wang et al. (2022), while for chlorpyrifos, the most susceptible population was selected as the reference baseline. The resistance levels for insecticides were classified as susceptible (RR ˂ 5.0), low level of resistance (5.0 ≤ RR ˂ 10.0), moderate level of resistance (10.0 ≤ RR ˂ 100.0), and high level of resistance (RR ≥ 100.0) (Lu et al., 2017; Zhang et al., 2017). The activities of CarEs, GSTs, and P450s along with the relative normalized expression of resistance-related genes were expressed as the mean ± standard error (SE), compared using the analysis of variance (ANOVA) followed by Tukey’s test (p ˂ 0.05) with the SPSS version 17.0 software package (IBM), while the graphs were prepared using the mean with SE in the Sigma Plot 10.0 software. Correlation analysis between the relative expressions of Ryr, P450 genes, and chlorantraniliprole resistance in the field populations of S. frugiperda in 2020 was calculated according to the Pearson method using the SPSS Statistics software package (Lu et al., 2017). A p-value of p < 0.05 or p < 0.01 was considered to be statistically significant or extremely significant.
3 Results
3.1 Susceptibility status and variations in field populations
The toxicities of five insecticides toward the field populations are given in Table 1. Field populations displayed low levels of resistance to chlorpyrifos. For chlorpyrifos, their LD50 values ranged from 106.680 μg/g (RH-22) to 339.518 μg/g (MIYI-22). For chlorantraniliprole, there was a deviation among the field populations whose RR values ranged from 2.02-fold (HD-19) to 10.39-fold (QJ-20), and their resistance levels ranged from susceptible to moderate level, and the QJ-20 population had the highest LD50 value (4.260 μg/g). For spinetoram, their RR values were ranging from 4.32-fold (DC-22) to 18.05-fold (RH-22). The LD50 values of all the different populations to emamectin benzoate were distributed between 0.242 μg/g (HD-19) and 0.886 μg/g (MIYI-22), and their RR values were ranging from 0.68-fold to 2.44-fold. According to insecticides of the sensitive baseline, the resistance of spinetoram was monitored in 2022, and only four populations were calculated. The LD50 values ranged from 2.197 μg/g to 10.837 μg/g with an RR of 9.23-fold (HD-22) to 45.53-fold (DC-22).
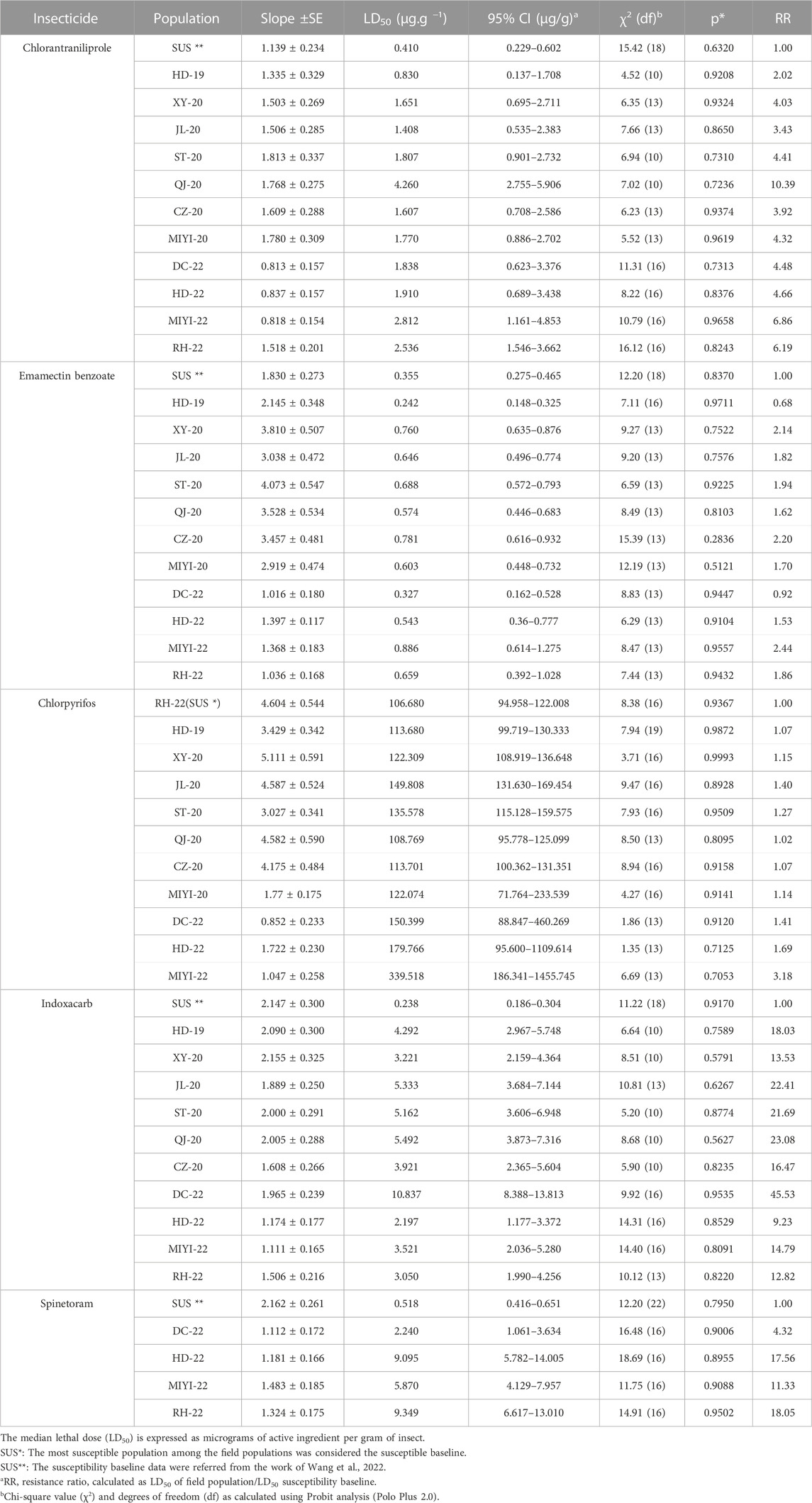
TABLE 1. Resistance levels of the field-collected populations of fall armyworm Spodoptera frugiperda to several insecticides in the topical bioassay method.
3.2 Synergism of TPP, PBO, and DEM
The effects of TPP, PBO, and DEM on the toxicity of chlorantraniliprole toward the QJ-20 population (RR = 10.39-fold) are given in Table 2. PBO had the highest synergistic of chlorantraniliprole for the QJ-20 population in moderate resistance (RR = 10.39-fold) compared with the treatment group without a synergist. The synergists PBO and DEM showed a slight increase in the toxicity of chlorantraniliprole, among which PBO enhanced the efficacy up to 1.43-fold and its LD50 treated by PBO decreased from 0.716 μg/g to 0.500 μg/g, while for DEM (LD50 = 0.667 μg/g) and TPP (LD50 = 0.767 μg/g), the synergistic ratios were only 1.08- and 0.93-fold, respectively.
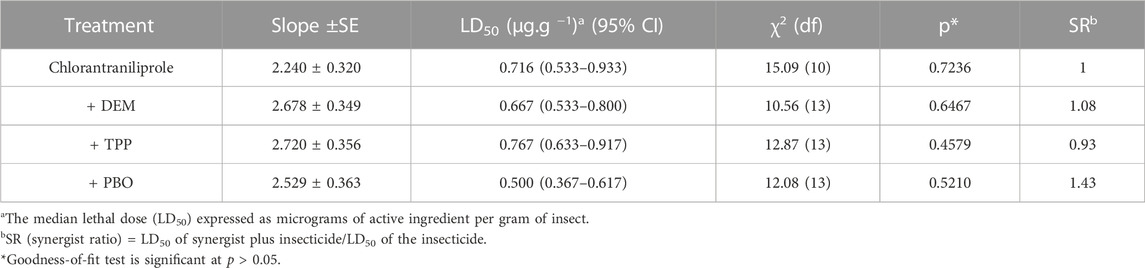
TABLE 2. Synergism of DEM, TPP, and PBO with chlorantraniliprole in the QJ-20 population of S. frugiperda.
3.3 Detoxification enzyme activities
To evaluate the role of these three detoxification enzymes in the development of resistance in S. frugiperda against chlorantraniliprole, the activities of CarEs, GSTs, and P450s were determined. As shown in Table 3, there was no significant difference among the activities of CarEs for all treatments (from 0.282 to 0.519 mmol/min. mg pro) (p ˃ 0.05). Similarly, no significant difference was observed among the activities of GSTs with the values of 0.106–0.166 mmol/min. mg pro, respectively (p ˃ 0.05). Meanwhile, for cytochrome P450s, there was significant difference in the activities between the QJ-20 population (0.629 nmol/min. mg pro) and PBO synergist application treatment (0.273 nmol/min. mg pro) (p < 0.05), but no significant difference with the treatment of PBO plus LD25 of chlorantraniliprole (0.439 nmol/min. mg pro) (p ˃ 0.05).
3.4 Relative expressions of Ryr and P450 genes
The mRNA expression levels of Ryr and P450 genes were detected in the QJ-20 population by qRT-PCR (Figure 2). Among these genes, six showed variation with the maximum relative expression for the Ryr gene (5.5-fold), followed by CYP49A1, CYP305A1, CYP6AE43, CYP306A1, and CYP321A8, with values of 3.9-, 3.3-, 2.4-, 2-, and 0.9-fold, respectively (Figure 2). Meanwhile, the relative expressions of these five CYP450 genes and the Ryr gene were also calculated in the tested field populations, in which CYP6AE43, CYP321A8, CYP305A1, CYP49A1, and CYP306A1 showed relative expression values ranging from 0.009- to 2.4-fold, 0.005- to 0.9-fold, 0.24- to 3.3-fold, 0.12- to 3.9-fold, and 0.9- to 2.0-fold, respectively (Figure 3).
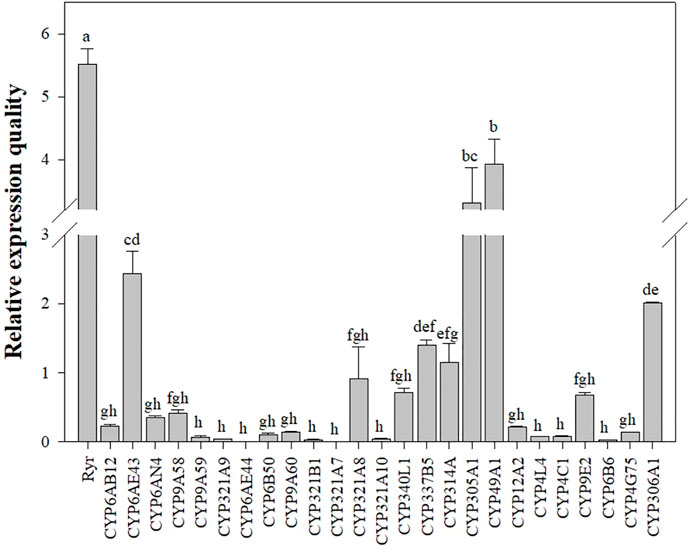
FIGURE 2. Relative expression levels of the CYP450 and Ryr gene in the QJ-20 population of FAW. The relative expression levels were compared using one-way ANOVA followed by Tukey’s test, at 0.05 level of significance, in SPSS version 17.0 software (IBM). Letters above the bars indicate significant differences (p ˂ 0.05), and means followed by the same letters did not differ significantly (p ˃ 0.05).
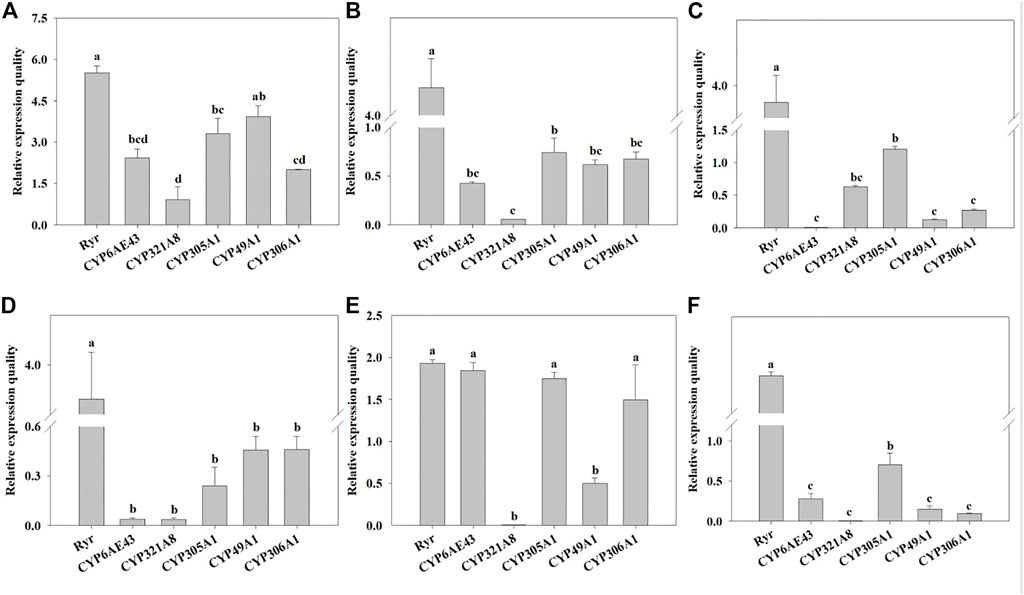
FIGURE 3. Relative normalized expressions of six resistance-related genes in all field populations. (A) QJ-20 field population, (B) XY-20 field population, (C) JL-20 field population, (D) ST-20 field population, (E) MIYI-20 field population, and (F) CZ-20 field population. Letters above the bars indicate significant differences (p ˂ 0.05), and means followed by the same letters did not differ significantly (p ˃ 0.05) according to Tukey’s test with SPSS version 17.0 software package (IBM).
Specifically, the Ryr gene showed significantly high relative expression levels, ranging from 1.93- to 5.5-fold, in all field populations (p < 0.05), compared with hd-19. Among them, QJ-20 showed the highest expression level (Figure 3). In the populations of XY-20, CZ-20, and JL-20, Ryr showed the highest relative expression, followed by CYP305A1, with the least significant relative expression of CYP321A8 in XY-20 (Figure 3B) and CZ-20 (Figure 3F), but in the JL-20 population, the CYP6AE43 expressed the least significant relative expression (p < 0.05) (Figure 3C). For the MIYI-20 population, Ryr showed the highest relative expression, followed by CYP6AE43, while CYP321A8 expressed the lowest relative expression (p < 0.05) (Figure 3E). Moreover, in the ST-20 population, Ryr showed significant overexpression while CYP321A8 expressed the lowest relative expression (p < 0.05) (Figure 3D).
To further verify the relationship between resistance to chlorantraniliprole and the relative gene expressions, we analyzed their correlation for six field populations in 2020. The Ryr expression had a correlation with chlorantraniliprole resistance but in a correlation value of 0.810 (p > 0.05), and the correlation coefficient of CYP49A1 and CYP305A1 reached 0.895 and 0.857 (p < 0.05), respectively, while for others, it was <0.8 (p > 0.05) (Table 4).
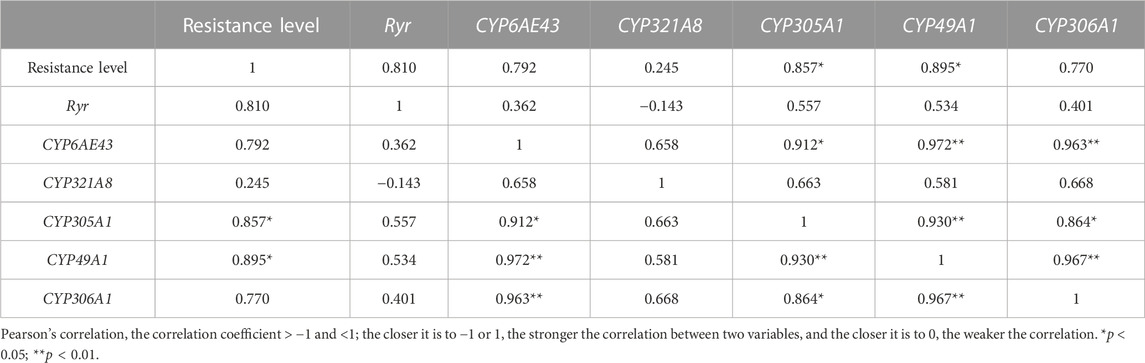
TABLE 4. Correlation analysis between the relative expressions of Ryr, P450 genes, and chlorantraniliprole resistance in the field populations of S. frugiperda in 2020.
4 Discussion
Spodoptera frugiperda is an invasive pest, not only does the unscientific use of chemical insecticides in the field result in the outbreak of resistance but invasive pests themselves may also carry resistance to certain insecticides (Gao et al., 2013). In our study, most tested FAW populations were susceptible to emamectin benzoate, which was consistent with previous reports (Liu et al., 2022; Wang et al., 2022). However, indoxacarb and spinetoram showed medium toxicity and posed a high risk for the evolution of resistance, and the sensitivity to chlorpyrifos had dropped substantially. It might be that those insecticides had still been used in the field, and S. frugiperda was also exposed to such agents, resulting in a large LD50 value in the field population (Zhang et al., 2021; Zhang et al., 2022). Among them, three field populations of the FAW in Sichuan were in low or moderate resistance to chlorantraniliprole; the resistance level was also increasing with time, and there were potential resistance risks, as reported in previous reports (Wang et al., 2022). This might be due to using unreasonable pesticides, the field selection of the pest, or its migration from other places with high resistance levels.
The resistance mechanism of insects toward insecticides is comprised of two main aspects, including the detoxification enzyme activity upregulation and the target-induced decreased sensitivity (Zhang B. et al., 2020). Low-dose chlorantraniliprole treatment can increase resistance to the same class of insecticides, which suggests that this is the consequence of the induction of particular detoxification enzymes (Riaz et al., 2009). An increase in the detoxification enzymes’ activities, such as CarEs, GSTs, and P450s, is one of the important reasons for the development of resistance toward insecticides (Pedersen et al., 2019), among which enhanced P450s activity plays an essential role (Liu et al., 2006; Mao et al., 2019). Our results also prove that the synergist PBO significantly increased the efficacy of chlorantraniliprole by reducing the P450 enzyme activities in our synergist experiment, and analogous results were also reported by Xu et al. (2019) and Tan et al. (2022), who suggested that P450 might play a primary role in the development of resistance toward chlorantraniliprole. Meanwhile, our result regarding enzyme activity determination also shows that the increase in P450 activity plays an important role in the resistance of S. frugiperda to chlorantraniliprole, which is consistent with the previous reports by Zhang D. D. et al. (2020), who reported that the increased activity of P450s enzymes might also be secondarily involved in the resistance mechanism of S. frugiperda against chlorantraniliprole.
The overexpression of some P450 genes may be the main reason for the increase in P450 activity, which can referee the resistance or induction of S. frugiperda to chlorantraniliprole (Gong et al., 2013; Elzaki et al., 2016). Our results show that four P450 genes CYP49A1, CYP305A1, CYP6AE43, and CYP306A1 were upregulated in the chlorantraniliprole-resistant populations and when exposed to the sublethal concentrations of chlorantraniliprole, CYP6AE43 in S. frugiperda was also upregulated (Xiao and Lu, 2022), while the downregulation of CYP6AE43 would enhance the susceptibility of S. frugiperda to some insecticides (Zhang B. et al., 2020). However, the association analysis between gene expression and chlorantraniliprole resistance revealed that CYP305A and CYP49A1 had the highest correlation with chlorantraniliprole resistance, reaching a significant level. Some studies have reported that pesticides, such as azadirachtin (Li et al., 2015) and phoxim (Yu et al., 2022), could induce the upregulation of CYP305A and CYP49A1 expression. In addition to P450 genes, we also found that the Ryr expression was highly correlated with resistance to chlorantraniliprole, which was in support of reports published by Sun et al. (2012) and Qin et al. (2018), who considered that the diamide-resistant populations have higher mRNA expression levels of the Ryr gene in Plutella xylostella. However, it has also been reported that chlorantraniliprole-resistant populations have lower expression levels of the Ryr gene in P. xylostella and Chilo suppressalis (Gong et al., 2014; Wei et al., 2019). Thus, to investigate whether the aforementioned genes are associated with chlorantraniliprole resistance, in the future, we would design a suitable dsRNA for executing RNA interference (RNAi) or RNA knockout assays to fully elaborate their functions in the development of resistance toward chlorantraniliprole.
Our results show that the resistance of the field populations of S. frugiperda in Sichuan toward emamectin benzoate and chlorpyrifos was still at a sensitive level. However, they exhibited a low or moderate level of resistance to chlorantraniliprole. The synergist and detoxification enzyme activity experiment showed that the resistance of S. frugiperda against chlorantraniliprole might cause the upregulated activities of the detoxification enzymes. The result of qRT-PCR and the association analysis between gene expression and chlorantraniliprole resistance revealed that the upregulation of CYP305A1, CYP49A1, and Ryr might be related to the upregulated activities of the detoxification enzymes and the induction of particular detoxification enzymes. However, further investigation is imperative to provide functional evidence for a catalytic interaction of chlorantraniliprole, which could include the expression of the corresponding genes in vivo or in vitro and functional verification by molecular technologies such as RNAi or CRISP-cas9, and so on. Nonetheless, our results provide a foundation for subsequent efforts to control S. frugiperda with integrated pest management strategies.
Data availability statement
The original contributions presented in the study are included in the article/Supplementary Material; further inquiries can be directed to the corresponding authors.
Author contributions
H-LC and AH: conceptualization, writing original draft, investigation, data curation, and formal analysis. Q-HC: project administration and resources. L-JX: funding acquisition and resources. Y-HC, RH, and C-WG: investigation. X-ML: supervision, funding, and acquisition. JP: supervision and project administration. LZ: formal analysis and investigation. X-GW: conceptualization, methodology, review and editing, supervision, visualization, and funding acquisition.
Acknowledgments
The authors gratefully acknowledge the financial support of the Science and Technology Program of Sichuan, China (2022YFSY0034).
Conflict of interest
The authors declare that the research was conducted in the absence of any commercial or financial relationships that could be construed as a potential conflict of interest.
Publisher’s note
All claims expressed in this article are solely those of the authors and do not necessarily represent those of their affiliated organizations, or those of the publisher, the editors, and the reviewers. Any product that may be evaluated in this article, or claim that may be made by its manufacturer, is not guaranteed or endorsed by the publisher.
Supplementary material
The Supplementary Material for this article can be found online at: https://www.frontiersin.org/articles/10.3389/fphys.2023.1180655/full#supplementary-material
References
Asperen, K. V. (1962). A study of housefly esterase by means of a sensitive colorimetric method. Insect Physiol. 8, 401–414. doi:10.1016/0022-1910(62)90074-4
Bradford, M. M. (1976). A rapid and sensitive method for the quantitation of microgram quantities of protein utilizing the principle of protein-dye binding. Anal. Biochem. 72, 248–254. doi:10.1006/abio.1976.9999
Brewer, M. J., and Trumble, J. T. (1989). Field monitoring for insecticide resistance in beet armyworm (Lepidoptera: Noctuidae). J. Econ. Entomol. 82, 1520–1526. doi:10.1093/jee/82.6.1520
Chapman, J. W., Williams, T., Escribano, A., Caballero, P., Cave, R. D., and Goulson, D. E. (2001). Age-related cannibalism and horizontal transmission of a nuclear polyhedrosis virus in larval Spodoptera frugiperda. Ecol. Entomol. 24, 268–275. doi:10.1046/j.1365-2311.1999.00224.x
Chen, X., and Palli, S. R. (2021). Transgenic overexpression of P450 genes confers deltamethrin resistance in the fall armyworm, Spodoptera frugiperda. Spodoptera frugiperda J. Pest Sci. 95, 1197–1205. doi:10.1007/s10340-021-01452-6
Dumas, P., Legeai, F., Lemaitre, C., Scaon, E., Orsucci, M., Labadie, K., et al. (2015). Spodoptera frugiperda (Lepidoptera: Noctuidae) host-plant variants: Two host strains or two distinct species? Genetica 143, 305–316. doi:10.1007/s10709-015-9829-2
Elzaki, M. E. A., Zhang, W., Feng, A., Qiou, X., Zhao, W., and Han, Z. (2016). Constitutive overexpression of cytochrome P450 associated with imidacloprid resistance in Laodelphax striatellus (Fallén). Pest Manag. Sci. 72 (5), 1051–1058. doi:10.1002/ps.4155
Feyereisen, R., Dermauw, W., Wybouw, N., Rombauts, S., Menten, B., Vontas, J., et al. (2012). A link between host plant adaptation and pesticide resistance in the polyphagous spider mite Tetranychus urticae. Tetranychus urticae P. Natl. Acad. Sci. USA. 110 (2), E113–E122. doi:10.1073/pnas.1213214110
Gao, C., Yao, R., Zhang, Z., Wu, M., Zhang, S., and Su, J. (2013). Susceptibility baseline and chlorantraniliprole resistance monitoring in Chilo suppressalis (Lepidoptera: Pyralidae). J. Econ. Entomol. 106, 2190–2194. doi:10.1603/ec13058
Giraudo, M., Hilliou, F., Fricaux, T., Audant, P., Feyereisen, R., and Le Goff, G. (2015). Cytochrome P450s from the fall armyworm (Spodoptera frugiperda): Responses to plant allelochemicals and pesticides. Insect Mol. Biol. 24 (1), 115–128. doi:10.1111/imb.12140
Gong, W., Yan, H. H., Gao, L., Guo, Y. Y., and Xue, C. B. (2014). Chlorantraniliprole resistance in the diamondback moth (Lepidoptera: Plutellidae). J. Econ. Entomol. 107, 806–814. doi:10.1603/ec13456
Gong, Y., Li, T., Zhang, L., Gao, X., and Liu, N. (2013). Permethrin induction of multiple cytochrome P450 genes in insecticide resistant mosquitoes, Culex quinquefasciatus. Int. J. Biol. Sci. 9 (9), 863–871. doi:10.7150/ijbs.6744
Gui, F., Lan, T., Zhao, Y., Guo, W., Dong, Y., Fang, D., et al. (2022). Genomic and transcriptomic analysis unveils population evolution and development of pesticide resistance in fall armyworm Spodoptera frugiperda. Protein Cell 13 (7), 513–531. doi:10.1007/s13238-020-00795-7
Gutierrez, M. R., Mota, S. D., Blanco, C. A., Whalon, M. E., Teran, S. H., Rodriguez, J. C., et al. (2019). Field-evolved resistance of the fall armyworm (Lepidoptera: Noctuidae) to synthetic insecticides in Puerto Rico and Mexico. J. Econ. Entomol. 112, 792–802. doi:10.1093/jee/toy372
Habig, W. H., and Jakoby, W. B. (1981). Assays for differentiation of glutathione S-transferases. Method. Enzymol. 77, 398–405. doi:10.1016/s0076-6879(81)77053-8
Lao, S. H., Huang, X. H., Huang, H. J., Liu, C. W., Zhang, C. X., and Bao, Y. Y. (2015). Genomic and transcriptomic insights into the cytochrome P450 monooxygenase gene repertoire in the rice pest Brown planthopper, Nilaparvata lugens. Nil. Lugens. BMC Genomics 106, 301–309. doi:10.1016/j.ygeno.2015.07.010
Li, F. C., Ni, M., Zhang, H., Wang, B. B., Xu, K. Z., Tian, J. H., et al. (2015). Expression profile analysis of silkworm P450 family genes after phoxim induction. Pestic. Biochem. Physiol. 122, 103–109. doi:10.1016/j.pestbp.2014.12.013
Li, Y. H., Wang, Z. Y., and Romeis, J. (2021). Managing the invasive fall armyworm through biotech crops: A Chinese perspective. Trends. Biotechnol. 39, 105–107. doi:10.1016/j.tibtech.2020.07.001
Liu, J., Hao, Z., Yang, S., Lin, Y., Zhong, H., and Jin, T. (2022). Insecticide resistance and its underlying synergism in field populations of Spodoptera frugiperda (J. E. Smith) from Hainan Island, China. Phytoparasitica 50, 933–945. doi:10.1007/s12600-022-01004-3
Liu, X. N., Liang, P., Gao, W. X., and Shi, X. Y. (2006). Induction of the cytochrome P450 activity by plant allelochemicals in the cotton bollworm, Helicoverpa armigera (Hübner). Pestic. Biochem. Physiol. 84, 127–134. doi:10.1016/j.pestbp.2005.06.002
Livak, K. J., and Schmittgen, T. D. (2001). Analysis of relative gene expression data using real-time quantitative PCR and the 2-ΔΔCT Method. Methods 25, 402–408. doi:10.1006/meth.2001.1262
Lu, Y. H., Wang, G. R., Zhong, L. Q., Zhang, F. C., Bai, Q., Zheng, S. R., et al. (2017). Resistance monitoring of Chilo suppressalis (Walker) (Lepidoptera: Crambidae) to chlorantraniliprole in eight field populations from east and central China. Crop Prot. 100, 196–202. doi:10.1016/j.cropro.2017.07.006
Mao, T. T., Li, F. C., Fang, Y. L., Wang, H., Chen, J., Li, M. X., et al. (2019). Effects of chlorantraniliprole exposure on detoxification enzyme activities and detoxification-related gene expression in the fat body of the silkworm, Bombyx mori. Ecotox. Environ. Safe. 176, 58–63. doi:10.1016/j.ecoenv.2019.03.074
McCaffery, A. R., Walker, A. J., and Topper, C. P. (1991). Insecticide resistance in the bollworm, Helicoverpa armigera from Indonesia. Pest Manag. Sci. 32, 85–90. doi:10.1002/ps.2780320109
Mohan, M., and Gujar, G. T. (2003). Local variation in susceptibility of the diamondback moth, Plutella xylostella (Linnaeus) to insecticides and role of detoxification enzymes. Crop Prot. 22, 495–504. doi:10.1016/S0261-2194(02)00201-6
Montezano, D. G., Specht, A., Sosa, D. R., Roque, V. F., Sousa, J. C., Paula, D., et al. (2018). Host plants of Spodoptera frugiperda (Lepidoptera: Noctuidae) in the americas. Afr. Entomol. 26, 286–300. doi:10.4001/003.026.0286
Pedersen, K. E., Pedersen, N. N., Meyling, N. V., Fredensborg, B. L., and Cedergreen, N. (2019). Differences in life stage sensitivity of the beetle Tenebrio molitor towards a pyrethroid insecticide explained by stage-specific variations in uptake, elimination, and activity of detoxifying enzymes. Pestic. Biochem. Physiol. 162, 113–121. doi:10.1016/j.pestbp.2019.09.009
Qin, C., Wang, C. H., Wang, Y. Y., Sun, S. Q., Wang, H. H., and Xue, C. B. (2018). Resistance to diamide insecticides in Plutella xylostella (Lepidoptera: Plutellidae): Comparison between lab-selected strains and field-collected populations. J. Econ. Entomol. 111, 853–859. doi:10.1093/jee/toy043
Riaz, M. A., Strode, C., Ranson, H., David, J. P., Poupardin, R., Reynaud, S., et al. (2009). Impact of glyphosate and benzo[a]pyrene on the tolerance of mosquito larvae to chemical insecticides. Role of detoxification genes in response to xenobiotics. Aquat. Toxicol. 93 (1), 61–69. doi:10.1016/j.aquatox.2009.03.005
Rose, R. L., Barbhaiya, L., Roe, R. M., Rock, G. C., and Hodgson, E. (1995). Cytochrome P450-associated insecticide resistance and the development of biochemical diagnostic assays in Heliothis virescens. Pestic. Biochem. Physiol. 51, 178–191. doi:10.1006/pest.1995.1018
Shu, B. S., Yu, H. K., Dai, J. H., Xie, Z. G., Qian, W. Q., and Lin, J. T. (2020). Stability evaluation of reference genes for real-time quantitative PCR normalization in S. frugiperda (Lepidoptera; Noctuidae). J. Integr. Agr. 19, 2471–2482. doi:10.1016/S2095-3119(20)63298-1
Sun, L., Yang, D., Rui, C., Cui, L., and Yuan, H. (2012). The effect of chlorantraniliprole to the mRNA expression of ryanodine receptor gene in. Diamondback Moth. J. Pest Sci. 14, 110–116. doi:10.1016/j.pestbp.2018.03.012
Sun, Y., Xu, L., Chen, Q., Qin, W. J., Huang, S. J., Qin, H. G., et al. (2018). Chlorantraniliprole resistance and its biochemical and new molecular target mechanisms in laboratory and field strains of Chilo suppressalis (Walker). Pest Manage. Sci. 123, 1416–1423. doi:10.1002/ps.4824
Tan, Q. M., Chen, W. W., Li, H. H., Liao, S. C., Yi, G. Q., Mei, Y., et al. (2022). Adipokinetic hormone signaling regulates cytochrome P450mediated chlorantraniliprole sensitivity in Spodoptera frugiperda (Lepidoptera: Noctuidae). Pest Manage. Sci. 78 (6), 2618–2628. doi:10.1002/ps.6896
Wang, H. H., Lv, S. L., Zhao, R., Liang, P., Zhang, S., and Gao, X. W. (2021). Establishment of the baseline for the relative sensitivity of Spodoptera frugiperda larvae to commonly used insecticides. J. Insect Physiol. 64 (12), 1427–1432. doi:10.16380/j.kcxb.2021.12.08
Wang, H. H., Zhao, R., Gao, J., Zhang, L., Zhang, S., Liang, P., et al. (2022). Genetic architecture and insecticide resistance in Chinese populations of Spodoptera frugiperda. J. Pest Sci. 2022. doi:10.1007/s10340-022-01569-2
Wang, X. G., Huang, Q., Hao, Q., Ran, S., Wu, Y. Q., Cui, P., et al. (2018). Resistance and enhanced cytochrome P450 monooxygenase activity in field populations of Spodoptera litura from Sichuan, China. Crop Prot. 106, 110–116. doi:10.1016/j.cropro.2017.12.020
Wei, Y. B., Yan, R., Zhou, Q. L., Qiao, L. Y., Zhu, G. N., Chen, M. L., et al. (2019). Monitoring and mechanisms of chlorantraniliprole resistance in Chilo suppressalis (Lepidoptera: Crambidae) in China. J. Econ. Entomol. 112, 1348–1353. doi:10.1093/jee/toz001
Xiao, T., and Lu, K. (2022). Functional characterization of CYP6AE subfamily P450s associated with pyrethroid detoxification in Spodoptera litura. Int. J. Biol. Macromol. 219, 452–462. doi:10.1016/j.ijbiomac.2022.08.014
Xu, L., Zhao, J., YangSun, Y., Xu, D. J., Xu, G. C., Xu, X. L., et al. (2019). Constitutive overexpression of cytochrome P450 monooxygenase genes contribute to chlorantraniliprole resistance in Chilo suppressalis (Walker). Pest Manage. Sci. 75, 718–725. doi:10.1002/ps.5171
Yu, H., Yang, X., Dai, J., Li, Y., Veeran, S., Lin, J., et al. (2022). Effects of azadirachtin on detoxification-related gene expression in the fat bodies of the fall armyworm, Spodoptera frugiperda. Spodoptera frugiperda Environ. Sci. Pollut. Res. Int. 30, 42587–42595. doi:10.1007/s11356-022-19661-6
Zhang, B., Su, X., Lu, L., Zhen, C., Zhu, B., Li, Y., et al. (2020a). Effects of three insecticides at the sublethal dose on the expression of cytochrome P450 genes in Spodoptera frugiperda (Lepidoptera: Noctuidae). Acta. Entomol. sini. 63 (5), 565–573. doi:10.16380/j.kcxb.2020.05.005
Zhang, B. Z., Su, X., Zhen, G. A., Lu, L. Y., Li, Y. S., Ge, X., et al. (2021). Silencing of cytochrome P450 in Spodoptera frugiperda (Lepidoptera: Noctuidae) by RNA interference enhances susceptibility to chlorantraniliprole. J. Insect. Sci. 2021. doi:10.1093/jisesa/ieaa047
Zhang, D. D., Xiao, Y. T., Xu, P. J., Yang, X. M., Wu, Q. L., Wu, K. M., et al. (2020b). Insecticide resistance monitoring for the invasive populations of Fall Armyworm, Spodoptera frugiperda in China. J. Integr. Agr. 19, 783–791. doi:10.1016/S2095-3119(20)63392-5
Zhang, M. Y., Zhang, P., Su, X., Guo, T. X., Zhou, J. L., Zhang, B. Z., et al. (2022). MicroRNA-190-5p confers chlorantraniliprole resistance by regulating CYP6K2 in Spodoptera frugiperda (Smith). Pestic. Biochem. Physiol. 184, 105133–133. doi:10.1016/j.pestbp.2022.105133
Keywords: Spodoptera frugiperda, chlorantraniliprole, cytochrome P450, qRT-PCR, resistance mechanism
Citation: Chen H-L, Hasnain A, Cheng Q-H, Xia L-J, Cai Y-H, Hu R, Gong C-W, Liu X-M, Pu J, Zhang L and Wang X-G (2023) Resistance monitoring and mechanism in the fall armyworm Spodoptera frugiperda (Lepidoptera: Noctuidae) for chlorantraniliprole from Sichuan Province, China. Front. Physiol. 14:1180655. doi: 10.3389/fphys.2023.1180655
Received: 06 March 2023; Accepted: 14 April 2023;
Published: 05 May 2023.
Edited by:
Qingli Shang, Jilin University, ChinaReviewed by:
Kangsheng Ma, Huazhong Agricultural University, ChinaTao Tang, Hunan Academy of Agricultural Sciences, China
Jianhong Li, Huazhong Agricultural University, China
Copyright © 2023 Chen, Hasnain, Cheng, Xia, Cai, Hu, Gong, Liu, Pu, Zhang and Wang. This is an open-access article distributed under the terms of the Creative Commons Attribution License (CC BY). The use, distribution or reproduction in other forums is permitted, provided the original author(s) and the copyright owner(s) are credited and that the original publication in this journal is cited, in accordance with accepted academic practice. No use, distribution or reproduction is permitted which does not comply with these terms.
*Correspondence: Lei Zhang, zhanglei86@cau.edu.cn; Xue-Gui Wang, wangxuegui@sicau.edu.cn
†These authors have contributed equally to this work and share first authorship