- 1College of Sports and Health, Shandong Sport University, Jinan, China
- 2School of Science, Shandong Jianzhu University, Jinan, China
- 3Shandong Institute of Sports Science, Jinan, China
Objective: This study aims to evaluate the test–retest reliability of kinematics and kinetics during single and dual-task stair walking in the elderly.
Methods: Fifteen healthy elderly adults were recruited. Kinematic and kinetic parameters were measured using an infrared motion analysis system (Vicon, Oxford Metrics Ltd., Oxford, United Kingdom) and force platforms (Switzerland, Kistler 9287BA and 9281CA). Participants were tested under single-task and dual-task (serial 3 subtractions or carrying a cup of water) conditions. Each participant completed two sessions on two separate days with a 1-week interval. Intraclass correlation coefficients (ICC), Pearson correlation coefficient (r), and Bland–Altman plot were used to assess the reliability of stair walking.
Results: When ascending stairs, the ICC of kinematics and kinetics ranged from fair to excellent (ICC = 0.500–0.979) in the single and dual tasks, except for step length (ICC = 0.394) in the single task. The r value of kinematics and kinetics ranged from 0.704 to 0.999. When descending stairs, the ICC of kinematics and kinetics ranged from good to excellent (ICC = 0.661–0.963), except for min hip moment (ICC = 0.133) and min ankle moment (ICC = 0.057) in the manual task. The r value of kinematics and kinetics ranged from 0.773 to 0.960 in the single and dual tasks. In the Bland–Altman plots, all the zero values and most of the dots fell in the 95% confidence interval, and the mean difference was found to be close to zero for all the parameters during stair walking.
Conclusion: These results obtained from this study show the good test-retest reliability of step cadence, step speed, and step width during single- and dual-task stair walking in the elderly, and the poor reliability of step length during ascending stairs. All the kinetic parameters, including min hip moment, max knee moment, and min ankle moment, had good test-retest reliability during single- and dual-task stair walking, but min hip moment and min ankle moment had poor reliability during manual-task descending stair. These results may help researchers in the assessment of biomechanics of dual-task stair walking in the elderly and to interpret the effect of interventions in this population.
1 Introduction
Approximately 30% of elderly people aged 65 years or older fall each year (Chippendale and Raveis, 2017). Falling during stair walking is a common cause of musculoskeletal injuries, hip fractures (Kim et al., 2022), craniocerebral injury, and even mortality (Clark and Arnold, 2021; Kim and Kim, 2022), leading to a substantial economic burden for family and society (Moreland et al., 2020). Stair walking is a difficult and challenging task for elderly people due to the deterioration of their physical function (Ito et al., 2020). More than half of all reported fall injuries occur when walking on stairs or slopes (Sheehan and Gottschall, 2012) (Blazewick et al., 2018).
The test–retest reliability of kinematic and kinetic parameters during single-task stair walking or level walking has been demonstrated in literature (Mentiplay and Clark, 2018); (Jarvis et al., 2021); (Leitner et al., 2011). As a previous study demonstrated, the test-retest reliability of all gait models was mostly good to excellent during level walking (Mentiplay and Clark, 2018). And the test-retest reliability of walking speed is excellent (ICC = 0.93–0.94) when using the Gait Box during level walking (Jarvis et al., 2021). Under a single task, stair walking had moderate to good reliability, with intraclass correlation coefficients (ICC) ranging from 0.537 to 0.836 and coefficients of variation (CV) ranging from 2.51% to 6.51% in vertical ground reaction forces (Leitner et al., 2011). In the single-task walking condition, all the components of the measure suggest good to excellent reliability (Brusola et al., 2021).
Dual-task interference could further impair gait performance and increase the risk of fall during stair walking in older people (Vallabhajosula et al., 2015). The dual-task (DT) paradigm leads to the competition of attention resources available and will influence the posture control of the elderly in stair negotiation (Simoni et al., 2013; Bianca et al., 2021). This paradigm refers to the performance of a cognitive task (CT) and manual task (MT) simultaneously, such as talking, calculating, and texting while moving an object to another place or carrying a tray with cups of water during stair walking (Paul et al., 2009; Asai et al., 2014; Belur et al., 2020). Dual tasks could significantly decrease gait performance and lower limb joint movement (Wang et al., 2022), reduce the gait speed, and increase the step width and body sway during stair walking (Madehkhaksar and Egges, 2016; Zhang et al., 2018).
However, the reliability of stair walking under a dual-task condition in the elderly is currently unknown. This study aimed to assess the test–retest reliability of kinematic and kinetic parameters in stair negotiation under single and dual-task conditions. Results may serve as a basis for clinical assessment of gait and motor control during stair walking in older people. We hypothesize that the 1) the reliability of kinematic and kinetic parameters of stair walking is high under single-task conditions 2) and poor under dual-task conditions.
2 Materials and methods
2.1 Participants
Fifteen healthy elderly adults (7 women and 8 men, 66.8 ± 3.6 years old, 161 ± 4.3 cm, 61.6 ± 4.2 kg) were recruited from a local community by distributing flyers in Jinan city, China. Some studies have reported that the estimation of the minimum sample size can be calculated based on the reliability of the ICC and the number of measurements of the subject (Donner and Eliasziw, 1987). A previous study found that the test-retest reliability was good to excellent for most models for lower limb kinematics and kinetics (ICC≥0.8) (Mentiplay and Clark, 2018). We set ICC = 0.8, α = 0.05, and power = 0.8, and when the number of measurements of the subject is two, according to the model provided by Donner A, the minimum sample size should be fifteen (Donner and Eliasziw, 1987). All the participants signed an informed consent form. This study was approved by the medical ethics committee of Shandong Sport University (No. 2017103). Participants met the following criteria for inclusion: able to ascend and descend stairs independently; able to understand verbal and written information; medically stable with no other diseases that significantly influenced gait performance (Flansbjer et al., 2005); and Body Mass Index of 18.5–25 kg/m2. Exclusion criteria included lower extremity contractures or pain (Dobkin et al., 2011); musculoskeletal or cardiovascular pathologies (Hashish et al., 2017); and Mini Mental State Examination score (MMSE) of <24 points (Lopez et al., 2005).
2.2 Data collection
Data were collected in the Biomechanical Laboratory of the Shandong Sport University. Each participant completed the stair walking test in two sessions on two separate days with a one-week interval. The tests were performed by the same experimenter at the same time of day in the same laboratory. A simulated staircase (17 cm riser, 29 cm tread) with six steps was constructed for data collection, which met the national residential standards (Figure 1). Two force platforms (Switzerland, Kistler 9287BA and 9281CA, 90 cm × 60 cm×10 cm) were used to collect kinetic data at 1,000 Hz and embedded in the third and fourth steps of the simulated staircase (Komnik et al., 2018). An infrared motion analysis system with 12 cameras (Vicon, Oxford Metrics Ltd., Oxford, United Kingdom) was used to collect kinematic data at 100 Hz (Komnik et al., 2018; Bates et al., 2021). Kinematic and kinetic data acquisition was synchronized using Vicon Nexus software. Prior to data collection, the participants were asked to wear unified black tight-fitting clothes and shoes and anthropometric data were collected (Law et al., 2021). The dominant leg was determined as the preferred leg for kicking a football. Forty-one reflective markers (14 mm) were placed on each participant’s anatomical landmarks based on the Visual 3D model, including four markers on the head, two on the trunk, 12 on the upper extremities, five on the pelvis, and 18 on the lower extremities. All the participants were instructed to ascend and descend the staircase with the dominant foot for the first step in a step-by-step manner at a comfortable pace under single-task, cognitive task, and manual-task conditions in random order. In the single-task condition, the participants descend and ascend stairs without cognitive or manual task interference. For the cognitive-task condition, the participants descend and ascend the stairs while performing subtraction of serial threes from a random three-digit number. For the manual-task condition, the participants descend and ascend the stairs while carrying a glass of water (0.63 kg) with the dominant hand. No instruction to prioritize the tasks were given to eliminate interference. Five trials were collected for each condition with a 1-min break between consecutive trials.
2.3 Data processing
When ascending the stairs, a stride cycle was defined as the first foot contact on the second step and ended at the same foot contact on the fourth step. When descending the stairs, the selected stride cycle started with the foot contact (of the same foot) on the fourth step and ended with the foot contact (of the same foot) on the second step (Protopapadaki et al., 2007). The first double-limb support phase was defined as when the right foot touched down until the left foot took off (Gorelick et al., 2009; Buckley et al., 2018). The single-limb support phase was defined as when the left foot took off until the left foot touched down. The second-double limb support phase was defined as when the left foot touched down until the right foot took off. The swing phase was defined as when the right foot took off until the right foot touched down (Singhal et al., 2014; Quintero et al., 2015). Vicon Nexus data were used to command, denoise the infrared reflective markers, and delete the markers in static and dynamic acquisition data. We completed the data by interpolation and intercepted the action frame number according to the gait cycle definition, renamed the intercepted data, and exported it to the C3d file. The exported static and dynamic c3d files were imported to Visual 3D for in-depth data processing and index extraction. A static model was built using the files and connected with the dynamic data. After selecting the data, the kinematic and kinetic signals were low-pass filtered by Pipeline with a cutoff frequency of 6 Hz (Singhal et al., 2014).
2.4 Parameters
2.4.1 Kinematic parameters
Step cadence was expressed as the number of steps in a minute (steps/min). Step speed was obtained by dividing the stride cycle duration into the displacement of the center of mass (m/s) (Buckley et al., 2018). Step length was expressed as the distance between the heel of the right foot during stair walking from the landing of the right foot to the landing again(m). Step width was defined as the medial–lateral distance between the midpoints of each foot(m) (Baudendistel et al., 2020).
2.4.2 Kinetic parameters
The net moment of the hip–knee–ankle joint was obtained based on the inverse dynamics calculation formula. Specific settings were used in V3d software to select the mechanical parameters to be calculated, the lower limb joint, and the reference link to complete the calculation of the net moment of the lower limb joint. In this study, the flexion and extension moments of the joint in the sagittal plane around the x-axis were selected for analysis. Joint movement was expressed as the peak flexion and extension moment of the hip, knee, and ankle joint in the sagittal plane (N·m/kg).
2.5 Statistical analysis
The kinematic and kinetic data were analyzed by SPSS (version 25.0) and presented as mean and standard deviation. Test—retest relative reliability was assessed using the intraclass correlation coefficient (ICC) test and interpreted as follows: poor (0 < ICC <0.39), fair (0.40 ≤ ICC <0.59), good (0.60 ≤ ICC < 0.74), and excellent (0.75 ≤ ICC < 1.0) (Cicchetti and Sparrow, 1981). Pearson correlation analysis was used to assess the linear correlation of the data from the two tests. The relationship was assessed as: small (0–0.3), moderate (0.3–0.5), significant (0.5–0.8), and highly relevant (0.8–1.0). The Bland–Altman method was used to assess the distribution of the mean and the difference of the two test data sets by using a scatter plot of the differences (Aandstad and Simon, 2013). Statistical significance was set at p < 0.05 (Clemons and Harrison, 2008).
3 Results
3.1 Test-retest reliability of kinematic and kinetic parameters during ascending stairs
Table 1 shows that the interclass correlation coefficient values ranged from 0.909 to 0.963 in step cadence, from 0.866 to 0.942 in step speed, from 0.394 to 0.553 in step length, and from 0.511 to 0.773 in step width under the three task conditions. The relationship values ranged from 0.840 to 0.929 in step cadence, from 0.768 to 0.891 in step speed, from 0.203 to 0.246 in step length, and from 0.344 to 0.630 in step width under the three conditions.
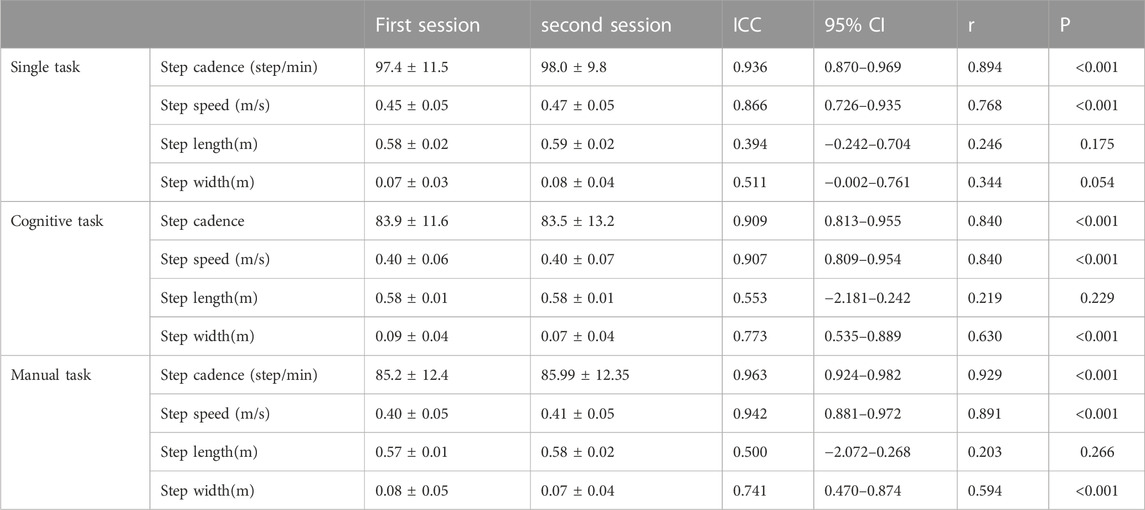
TABLE 1. The test-retest reliability of kinematic parameters under three conditions when ascending stairs.
Table 2 shows that the interclass correlation coefficient values ranged from 0.858 to 0.922 in min hip moment, from 0.881 to 0.954 in maximum knee moment, and from 0.857 to 0.979 in minimum ankle moment under the three task conditions. The relationship values ranged from 0.751 to 0.856 in minimum hip moment, from 0.787 to 0.914 in maximum knee moment, and from 0.764 to 0.960 in minimum ankle moment under the three conditions.
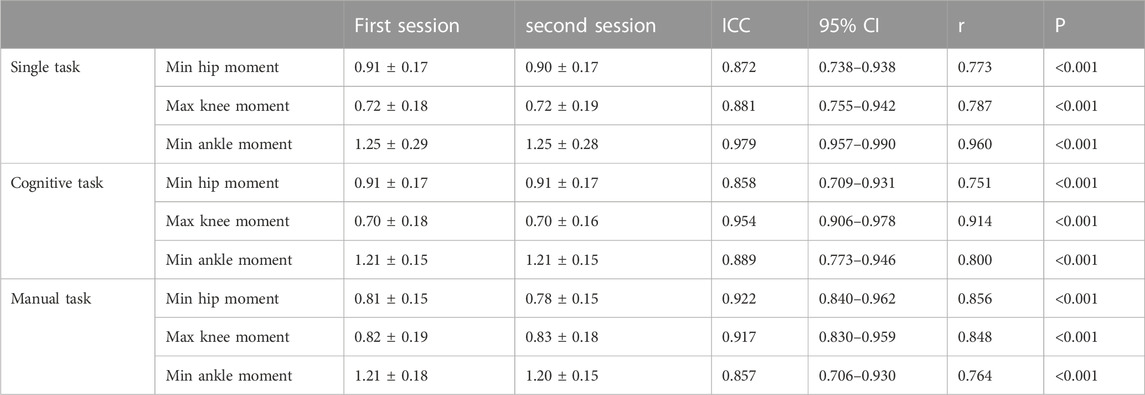
TABLE 2. The test-retest reliability of kinetic parameters under three conditions when ascending stairs. (N.m/kg).
Figure 2 shows the Bland—Altman plots of the kinematic and kinetic parameters when ascending stairs. The systematic basis around the zero line for kinematic and kinetic parameters was revealed. All the zero values fell in the 95% confidence interval. The numbers of dots inside the range of 95% CI interpreted the reliability. A higher number of dots indicated better reliability. In this study, the numbers of dots outside the range of 95% CI were one dot (3.1%) in step width to two dots (6.3%) in step cadence in the single task, three dots (9.4%) in step cadence in the cognitive task, and one dot (3.1%) in step cadence in the manual task. The numbers of dots outside the range of 95% CI were one dot (3.1%) in step speed to two dots (6.3%) in step length in the single task, from one dot (3.1%) in step speed to two dots (6.3%) in step length in the cognitive task, and one dot (3.1%) in step speed and step length in the manual task. The numbers of dots outside the range of 95% CI were two dots (6.3%) in minimum hip moment to three dots (9.4%) in maximum knee moment in the single task, two dots (6.3%) in minimum hip moment in the cognitive task, and one dot (3.1%) in minimum hip moment to two dots (6.3%) in maximum knee moment in the manual task. The numbers of dots outside the range of 95% CI were one dot (3.1%) in minimum ankle moment in the single task, two dots (6.3%) in minimum ankle moment in the cognitive task, and one dot (3.1%) in minimum ankle moment in the manual task.
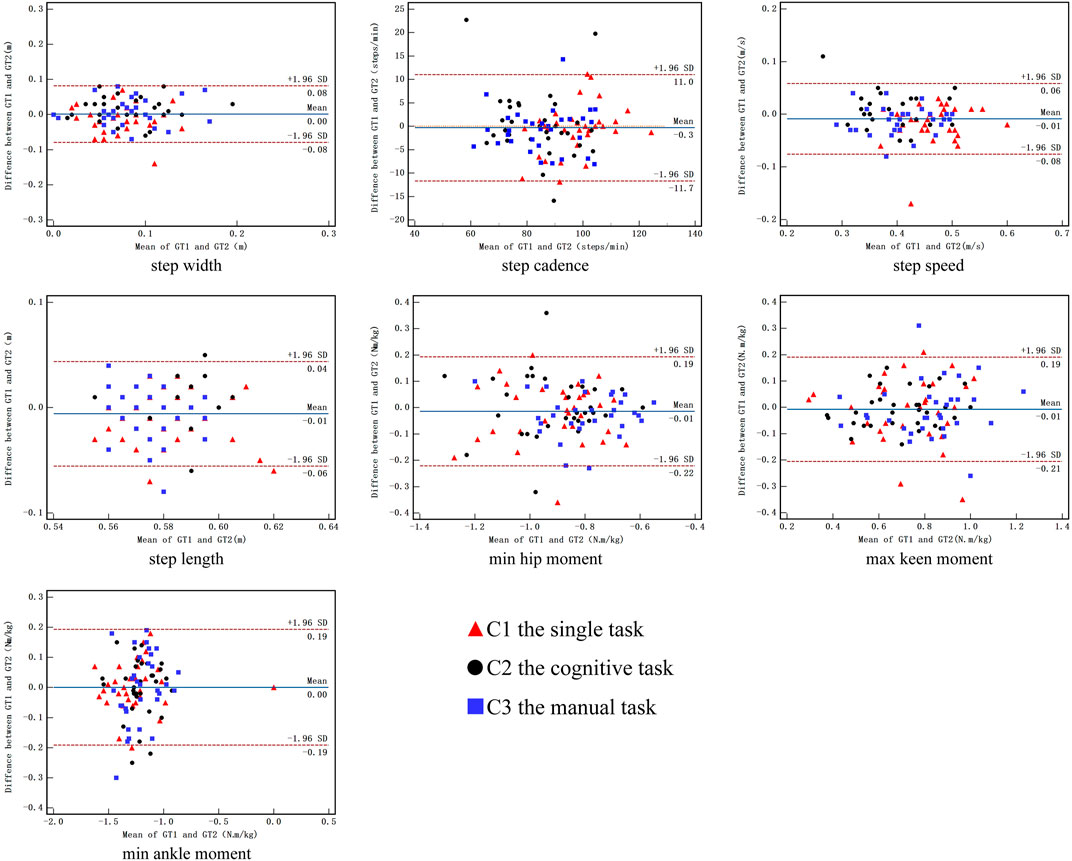
FIGURE 2. Bland-Altman plot of the difference between session 1 and session 2 against mean value during ascending stair for kinematic and kinetic parameters. C1, the single task; C2 the cognitive task; C3 the manual task.
3.2 Test—retest reliability of kinematic and kinetic parameters during descending stairs
Table 3 shows that the interclass correlation coefficient values ranged from 0.925 to 0.956 in step cadence, from 0.779 to 0.932 in step speed, from 0.305 to 0.745 in step length, and from 0.661 to 0.795 in step width under the three task conditions. The relationship values ranged from 0.866 to 0.916 in step cadence, from 0.639 to 0.873 in step speed, from 0.185 to 0.597 in step length, and from 0.500 to 0.660 in step width under the three conditions.
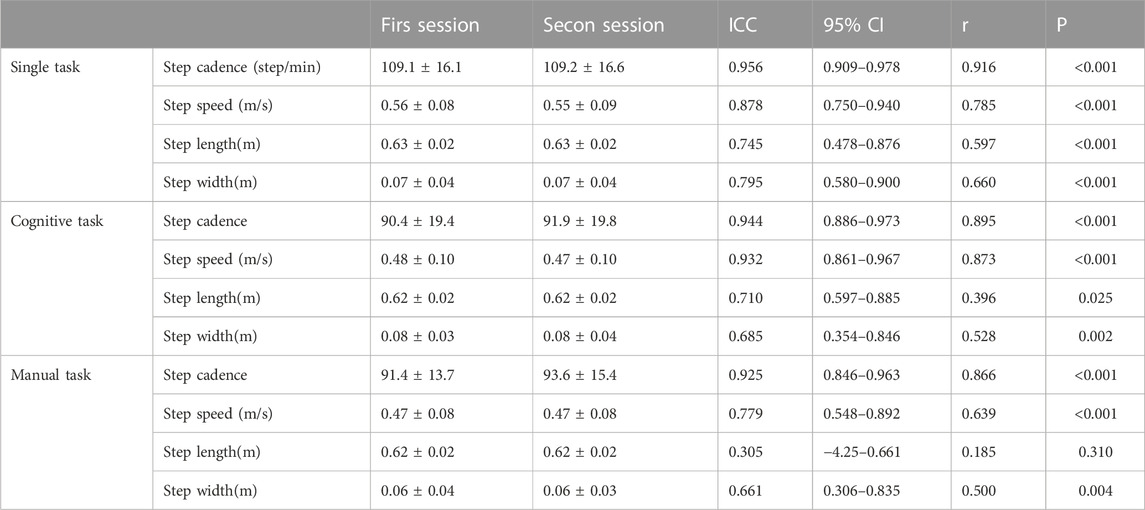
TABLE 3. The test-retest reliability of kinematic parameters under three conditions when descending stairs.
Table 4 shows that the interclass correlation coefficient values ranged from 0.133 to 0.963 in minimum hip moment, from 0.893 to 0.935 in maximum knee moment, and from 0.057 to 0.850 in minimum ankle moment under the three task conditions. The relationship values ranged from 0.075 to 0.930 in minimum hip moment, from 0.816 to 0.887 in maximum knee moment, and from 0.030 to 0.752 in minimum ankle moment under the three conditions.
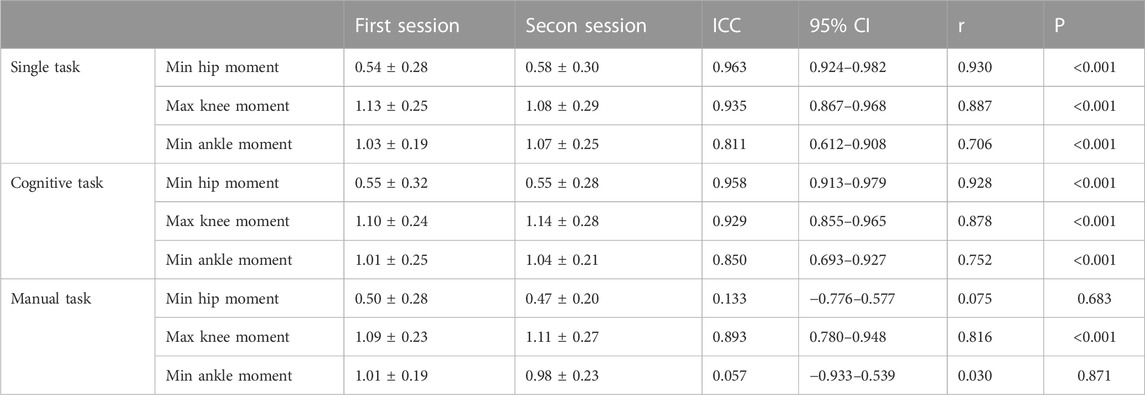
TABLE 4. The test-retest reliability of kinetic parameters under three conditions when descending stairs. (N m/kg).
Figure 3 shows the Bland—Altman plots of the kinematic and kinetic parameters during descending stairs. The numbers of dots outside the range of 95% CI were one dot (3.1%) in step width and step cadence in the single task, two dots (6.3%) in step width to four dots (12.5%) in step cadence in the cognitive task, and three dots (9.4%) in step width to one dot (3.1%) in step cadence in the manual task. The numbers of dots outside the range of 95% CI were one dot (3.1%) in step speed in the single task, two dots (6.3%) in step length in the cognitive task, and two dots (6.3%) in step speed to one dot (3.1%) in step length in the manual task. The numbers of dots outside the range of 95% CI were one dot (3.1%) in maximum knee moment in the single task, two dots (6.3%) in maximum knee moment in the cognitive task, and one dot (3.1%) in maximum knee moment to three dots (9.4%) in minimum hip moment in the manual task. The numbers of dots outside the range of 95% CI were one dot (3.1%) in minimum ankle moment in the single task and cognitive task and two dots (6.3%) in minimum ankle moment in the manual task.
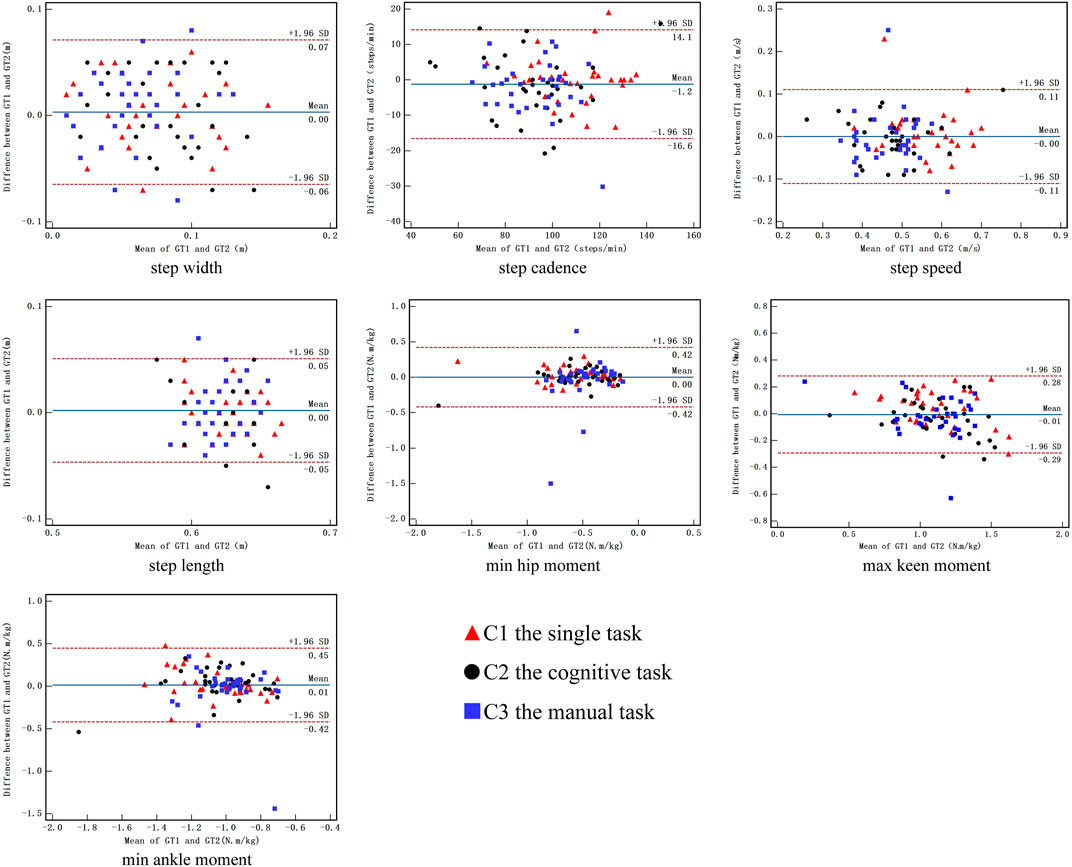
FIGURE 3. Bland-Altman plot of the difference between session 1 and session 2 against mean value during descending stair for kinematic and kinetic parameters. C1, the single task; C2 the cognitive task; C3 the manual task.
4 Discussion
This study revealed the test—retest reliability of kinematic and kinetic parameters in the elderly during stair walking under single task, cognitive task, and manual task conditions (Flansbjer et al., 2005; Gorelick et al., 2009). Most of the test–retest reliability values for these parameters were good to excellent.
ICC is a popular statistical method used to assess test–retest reliability (Chan and Pin, 2019) and is based on the calculation of the F-value from repeated-measures ANOVA. In this study, most of the test–retest reliability values of the kinematic and kinetic parameters were high in the single task, which supported the first hypothesis. The ICC value (0.511–0.979) demonstrated fair to excellent test–retest reliability in the single task, except for step length (Flansbjer et al., 2005). The finding was consistent with the study of Santos et al. (Santos et al., 2022), who reported that the agreement between manual stopwatches and photoelectric cells was excellent (ICCs between 0.92 and 0.97) when the distance was 5 m. Bland—Altman plot analysis is an important method used to detect systematic errors (Bland and Altman, 1999). The axis of ordinate was determined based on the differences between two measurements, and the axis of abscissa was determined based on the mean of two measurements. In some clinical studies, Bland—Altman analysis was used to evaluate the agreement between two testing sessions (Soulard et al., 2021). Reliability was assessed using the 95% limits of agreement and mean difference. As shown in Figures 2, 3, during single task, the zero values were included in the 95% CI, few dots were outside the range of 95% CI, and the mean difference was close to zero, indicating fair reproducibility (Dolatabadi et al., 2016). The good test–retest reliability of the kinematic and kinetic parameters during single-task stair walking might be attributed to several factors. First, the same testing control could help reduce measurement errors, including the same time interval between consecutive tests, the same testing commands, the same gait and postural control during stair walking, and the laboratory environment (Flansbjer et al., 2005). Second, three pre-test practices were provided to each participant before data collection to help them understand the procedure and minimize errors in learning (Sun et al., 2015). Finally, the test and retest sessions of reliability were scheduled a week apart, which could prevent learning and fatigue from affecting the reproducibility of the measurements.
In dual-task stair walking, the ICC and r values of the kinematic and kinetic parameters demonstrated fair to excellent test–retest reliability. The Bland–Altman plot also showed that the zero values were included in the 95% CI, the mean difference was close to zero, and about 14 dots (87.5%) were inside the range of 95% CI during cognitive and manual stair walking. Our results showed that the test–retest reliability of the kinematic and kinetic parameters during dual-task stair walking was fair to excellent, which did not support the second hypothesis. However, previous studies were in line with our results. A recent study by Cho et al. showed that some level walking gait parameters (e.g., speed, stride length, cadence, etc.) while talking had good reliability (ICC = 0.69–0.90) under dual-task conditions (Cho et al., 2015). Tsang et al., (2019) also found that the test–retest reliability of the dual-task walking distance tests were excellent (ICC = 0.891–0.984). When confronted by two attention-demanding activities, humans prioritize one task over the other based on counterbalancing capabilities and cognitive availability (Yogev-Seligmann et al., 2012). The elderly might prefer to perform posture control of gait stability rather than deal with the ‘‘secondary’’ cognitive task during walking to minimize the loss of balance and maximize the avoidance of hazards (Shumway-Cook et al., 1997). When facing more complexed situations, such as stair walking, individuals tend to allocate attention resources for posture control to ensure safety (Wang et al., 2021). Therefore, the test–retest reliability of posture control during stair walking is high.
However, the ICC value ranged from 0.394 to 0.553, and the r value ranged from 0.203 to 0.246 of step length when ascending stairs under single- and dual-task conditions, indicating the poor test–retest reliability of step length. The ICC values were 0.133 in minimum hip moment and 0.057 in minimum ankle moment; the r values were 0.075 in minimum hip moment and 0.030 in minimum ankle moment in the manual task when descending stairs, which showed the poor test–retest reliability of both parameters. Our finding was inconsistent with the results of this study (Ryan et al., 2006); at all speeds, inter device reliability was excellent for the activPAL physical activity monitor ((ICC (2,1)≥0.99)) for both step number and cadence. The discrepancy might be related to the differences in gait complexion between stair and level walking. More challenging and complex tasks might require more attentional resources (Ruffieux et al., 2015). Stair walking, as the most hazardous aspect of gait, might be more challenging and complex and requires greater attentional resources (Mian et al., 2007). According to capacity–sharing theory, cognitive resources are limited in capacity; when two tasks sharing common neural circuitry are performed at the same time, both of them are processed, but the processing ability between the tasks will decrease (Patel et al., 2014). When performing stair walking and dual tasks simultaneously (Wang et al., 2021), the poor test–retest reliability may have occurred due to the competition of limited attention resources, resulting in the decline of task performance in dual-task situation during stair walking (Ettwig and Bronkhorst, 2015). This study might provide reference for the selection of kinematic and kinetic parameters during dual-task stair walking.
This study has three limitations. First, the sample size was relatively small, and the test–retest reliability of stair walking should be used cautiously for clinical assessment. Second, all the participants were healthy elderly adults, and further studies should focus on the reliability of stair walking in frail elderly adults with a high fall risk. Finally, we only recruited people with a BMI of 18.5–25 Kg/m2, so the result should be cautiously used for the elderly population.
5 Conclusion
The results obtained from this study show the good test-retest reliability of step cadence, step speed, and step width during single- and dual-task stair walking in the elderly, and the poor reliability of step length when ascending stairs. All the kinetic parameters, including min hip moment, max knee moment, and min ankle moment, had good test-retest reliability during single- and dual-task stair walking, but min hip moment and min ankle moment had poor reliability during manual-task descending stairs. These results may help researchers in the assessment of biomechanics of dual-task stair walking in the elderly and to interpret the effect of interventions in this population.
Data availability statement
The original contributions presented in the study are included in the article/supplementary material, further inquiries can be directed to the corresponding author.
Ethics statement
The studies involving human participants were reviewed and approved by the Shandong Provincial Natural Science Foundation (No. ZR2020QC091). The patients/participants provided their written informed consent to participate in this study. Written informed consent was obtained from the individual(s) for the publication of any potentially identifiable images or data included in this article.
Author contributions
YL and WS are responsible for drafting this protocol and participating in the conception, study design, assessments, data interpretation, and writing and submission of the manuscript. JW and CZ are responsible for the study design and revision of the manuscript. QS and CZ are responsible for the study design. JW and NY are responsible for the data management and monitoring of this study. All authors have read the manuscript and approved the publication of this protocol.
Funding
This work was supported by the Shandong Provincial Natural Science Foundation (No. ZR2020QC091).
Acknowledgments
The authors are thankful to all the participants and their power of endurance to complete the study.
Conflict of interest
The authors declare that the research was conducted in the absence of any commercial or financial relationships that could be construed as a potential conflict of interest.
Publisher’s note
All claims expressed in this article are solely those of the authors and do not necessarily represent those of their affiliated organizations, or those of the publisher, the editors and the reviewers. Any product that may be evaluated in this article, or claim that may be made by its manufacturer, is not guaranteed or endorsed by the publisher.
References
Aandstad, A., and Simon, E. V. (2013). Reliability and validity of the soccer specific INTER field test. J. Sports Sci. 31 (13), 1383–1392. doi:10.1080/02640414.2013.781667-
Asai, T., Misu, S., Doi, T., Yamada, M., and Ando, H. (2014). Effects of dual-tasking on control of trunk movement during gait: Respective effect of manual- and cognitive-task. Gait Posture 39 (1), 54–59. doi:10.1016/j.gaitpost.2013.05.025
Bates, A. V., McGregor, A. H., and Alexander, C. M. (2021). Comparing sagittal plane kinematics and kinetics of gait and stair climbing between hypermobile and non-hypermobile people; a cross-sectional study. BMC Musculoskelet. Disord. 22 (1), 712. doi:10.1186/s12891-021-04549-2
Baudendistel, S. T., Grindstaff, T. L., Rosen, A. B., and Yentes, J. M. (2020). Bimanual load carriage alters sway patterns and step width. Appl. Ergon. 84, 103030. doi:10.1016/j.apergo.2019.103030
Belur, P., Hsiao, D., Myers, P. S., Earhart, G. M., and Rawson, K. S. (2020). Dual-task costs of texting while walking forward and backward are greater for older adults than younger adults. Hum. Mov. Sci. 71, 102619. doi:10.1016/j.humov.2020.102619
Bianca, M., Xue, W. T., Thierry, P., Philippe, D., Laurent, B., and Marie-Lyne, N. (2021). Can a knee brace prevent acl reinjury: A systematic review. Int. J. Environ. Res. Public Health 18 (14), 7611. doi:10.3390/ijerph18147611
Bland, J. M., and Altman, D. G. (1999). Measuring agreement in method comparison studies. Stat. Methods Med. Res. 8 (2), 135–160. doi:10.1177/096228029900800204-
Blazewick, D. H., Chounthirath, T., Hodges, N. L., Collins, C. L., and Smith, G. A. (2018). Stair-related injuries treated in United States emergency departments. Am. J. Emerg. Med. 36 (4), 608–614. doi:10.1016/j.ajem.2017.09.034
Brusola, G. A., Mitchell, K., Ellison, J., and Gleeson, P. (2021). Reliability and validity of the modified Walking and Remembering Test in persons with multiple sclerosis. Int. J. Rehabil. Res. 44 (4), 323–329. doi:10.1097/mrr.0000000000000493
Buckley, E., Mazzà, C., and McNeill, A. (2018). A systematic review of the gait characteristics associated with Cerebellar Ataxia. Gait Posture 60, 154–163. doi:10.1016/j.gaitpost.2017.11.024
Chan, W. L. S., and Pin, T. W. (2019). Reliability, validity and minimal detectable change of 2-minute walk test, 6-minute walk test and 10-meter walk test in frail older adults with dementia. Exp. Gerontol. 115, 9–18. doi:10.1016/j.exger.2018.11.001
Chippendale, T., and Raveis, V. (2017). Knowledge, behavioral practices, and experiences of outdoor fallers: Implications for prevention programs. Arch. Gerontol. Geriatr. 72, 19–24. doi:10.1016/j.archger.2017.04.008
Cho, K. H., Lee, H. J., and Lee, W. H. (2015). Test-retest reliability of the GAITRite walkway system for the spatio-temporal gait parameters while dual-tasking in post-stroke patients. Disabil. Rehabil. 37 (6), 512–516. doi:10.3109/09638288.2014.932445
Cicchetti, D. V., and Sparrow, S. A. (1981). Developing criteria for establishing interrater reliability of specific items: Applications to assessment of adaptive behavior. Am. J. Ment. Defic. 86 (2), 127–137.
Clark, B., and Arnold, W. (2021). Strategies to Prevent Serious Fall Injuries: A Commentary on Bhasin et al. A Randomized Trial of a Multifactorial Strategy to Prevent Serious Fall Injuries. N Engl J Med. 2020;383(2):129-140. Adv. geriatric Med. Res. 3 (1), e210002–e210140. doi:10.20900/agmr20210002-
Clemons, J., and Harrison, M. (2008). Validity and reliability of a new stair sprinting test of explosive power. J. Strength Cond. Res. 22 (5), 1578–1583. doi:10.1519/JSC.0b013e31817ae91d
Dobkin, B. H., Xu, X., Batalin, M., Thomas, S., and Kaiser, W. (2011). Reliability and validity of bilateral ankle accelerometer algorithms for activity recognition and walking speed after stroke. Stroke 42 (8), 2246–2250. doi:10.1161/strokeaha.110.611095
Dolatabadi, E., Taati, B., and Mihailidis, A. (2016). Concurrent validity of the Microsoft Kinect for Windows v2 for measuring spatiotemporal gait parameters. Med. Eng. Phys. 38 (9), 952–958. doi:10.1016/j.medengphy.2016.06.015
Donner, A., and Eliasziw, M. (1987). Sample size requirements for reliability studies. Stat. Med. 6 (4), 441–448. doi:10.1002/sim.4780060404
Ettwig, J. F., and Bronkhorst, A. W. (2015). Attentional switches and dual-task interference. PLoS One 10 (3), e0118216. doi:10.1371/journal.pone.0118216
Flansbjer, U. B., Holmbäck, A. M., Downham, D., Patten, C., and Lexell, J. (2005). Reliability of gait performance tests in men and women with hemiparesis after stroke. J. Rehabil. Med. 37 (2), 75–82. doi:10.1080/16501970410017215
Gorelick, M. L., Bizzini, M., Maffiuletti, N. A., Munzinger, J. P., and Munzinger, U. (2009). Test-retest reliability of the IDEEA system in the quantification of step parameters during walking and stair climbing. Clin. Physiol. Funct. Imaging 29 (4), 271–276. doi:10.1111/j.1475-097X.2009.00864.x
Hashish, R., Toney-Bolger, M. E., Sharpe, S. S., Lester, B. D., and Mulliken, A. (2017). Texting during stair negotiation and implications for fall risk. Gait Posture 58, 409–414. doi:10.1016/j.gaitpost.2017.09.004
Ito, Y., Aoki, T., Sato, T., Oishi, K., and Ishii, K. (2020). Comparison of quadriceps setting strength and knee extension strength tests to evaluate lower limb muscle strength based on health-related physical fitness values in elderly people. BMJ Open Sport Exerc Med. 6 (1), e000753. doi:10.1136/bmjsem-2020-000753
Jarvis, L. M., Peterson, M. J., and Caves, K. M. (2021). Development, validity, and reliability of a novel walking speed measurement device: The GaitBox. Gait Posture 84, 52–57. doi:10.1016/j.gaitpost.2020.11.009
Kim, K., Lui, L., and Cummings, S. (2022). Recent fall and high imminent risk of fracture in older men and women. Age ageing 51 (6), afac141. doi:10.1093/ageing/afac141(
Kim, M., and Kim, Y. (2022). Comparison of factors influencing fall recurrence in the young-old and old-old: A cross-sectional nationwide study in South Korea. BMC Geriatr. 22 (1), 520. doi:10.1186/s12877-022-03172-7
Komnik, I., David, S., Funken, J., Haberer, C., Potthast, W., and Weiss, S. (2018). Compromised knee internal rotation in total knee arthroplasty patients during stair climbing. PLoS One 13 (10), e0205492. doi:10.1371/journal.pone.0205492
Law, N. H., Li, J. X., Law, N. Y., Varin, D., and Lamontagne, M. (2021). Effects of body mass and sex on kinematics and kinetics of the lower extremity during stair ascent and descent in older adults. Sports Med. Health Sci. 3 (3), 165–170. doi:10.1016/j.smhs.2021.06.001
Leitner, M., Schmid, S., Hilfiker, R., and Radlinger, L. (2011). Test-retest reliability of vertical ground reaction forces during stair climbing in the elderly population. Gait Posture 34 (3), 421–425. doi:10.1016/j.gaitpost.2011.06.014
Lopez, M. N., Charter, R. A., Mostafavi, B., Nibut, L. P., and Smith, W. E. (2005). Psychometric properties of the folstein mini-mental state examination. Assessment 12 (2), 137–144. doi:10.1177/1073191105275412
Madehkhaksar, F., and Egges, A. (2016). Effect of dual task type on gait and dynamic stability during stair negotiation at different inclinations. Gait Posture 43, 114–119. doi:10.1016/j.gaitpost.2015.09.009
Mentiplay, B. F., and Clark, R. A. (2018). Modified conventional gait model versus cluster tracking: Test-retest reliability, agreement and impact of inverse kinematics with joint constraints on kinematic and kinetic data. Gait Posture 64, 75–83. doi:10.1016/j.gaitpost.2018.05.033
Mian, O. S., Thom, J. M., Narici, M. V., and Baltzopoulos, V. (2007). Kinematics of stair descent in young and older adults and the impact of exercise training. Gait Posture 25 (1), 9–17. doi:10.1016/j.gaitpost.2005.12.014
Moreland, B., Kakara, R., and Henry, A. (2020). Trends in nonfatal falls and fall-related injuries among adults aged ≥65 Years - United States, 2012-2018. MMWR. Morb. Mortal. Wkly. Rep. 69 (27), 875–881. doi:10.15585/mmwr.mm6927a5
Patel, P., Lamar, M., and Bhatt, T. (2014). Effect of type of cognitive task and walking speed on cognitive-motor interference during dual-task walking. Neuroscience 260, 140–148. doi:10.1016/j.neuroscience.2013.12.016
Paul, L., Ellis, B. M., Leese, G. P., McFadyen, A. K., and McMurray, B. (2009). The effect of a cognitive or motor task on gait parameters of diabetic patients, with and without neuropathy. Diabet. Med. 26 (3), 234–239. doi:10.1111/j.1464-5491.2008.02655.x
Protopapadaki, A., Drechsler, W. I., Cramp, M. C., Coutts, F. J., and Scott, O. M. (2007). Hip, knee, ankle kinematics and kinetics during stair ascent and descent in healthy young individuals. Clin. Biomech. (Bristol, Avon) 22 (2), 203–210. doi:10.1016/j.clinbiomech.2006.09.010
Quintero, D., Martin, A. E., and Gregg, R. D. (2015). Unifying the gait cycle in the control of a powered prosthetic leg. IEEE Int. Conf. Rehabil. Robot. 2015, 289–294. doi:10.1109/icorr.2015.7281214
Ruffieux, J., Keller, M., Lauber, B., and Taube, W. (2015). Changes in standing and walking performance under dual-task conditions across the lifespan. Sports Med. 45 (12), 1739–1758. doi:10.1007/s40279-015-0369-9
Ryan, C. G., Grant, P. M., Tigbe, W. W., and Granat, M. H. (2006). The validity and reliability of a novel activity monitor as a measure of walking. Br. J. Sports Med. 40 (9), 779–784. doi:10.1136/bjsm.2006.027276
Santos, F., Ozguler, A., Lenain, M., Zins, M., Artaud, F., and Elbaz, A. (2022). Comparison of manual and automated measures of walking speed: Distance and pace matter. Exp. Gerontol. 170, 111987. doi:10.1016/j.exger.2022.111987
Sheehan, R., and Gottschall, J. (2012). At similar angles, slope walking has a greater fall risk than stair walking. Appl. Ergon. 43 (3), 473–478. doi:10.1016/j.apergo.2011.07.004
Shumway-Cook, A., Woollacott, M., Kerns, K. A., and Baldwin, M. (1997). The effects of two types of cognitive tasks on postural stability in older adults with and without a history of falls. J. Gerontol. A Biol. Sci. Med. Sci. 52 (4), M232–M240. doi:10.1093/gerona/52a.4.m232
Simoni, D., Rubbieri, G., Baccini, M., Rinaldi, L., Becheri, D., Forconi, T., et al. (2013). Different motor tasks impact differently on cognitive performance of older persons during dual task tests. Clin. Biomech. (Bristol, Avon) 28 (6), 692–696. doi:10.1016/j.clinbiomech.2013.05.011
Singhal, K., Kim, J., Casebolt, J., Lee, S., Han, K. H., and Kwon, Y. H. (2014). Kinetic comparison of older men and women during walk-to-stair descent transition. Gait Posture 40 (4), 600–604. doi:10.1016/j.gaitpost.2014.07.004
Soulard, J., Vaillant, J., Balaguier, R., and Vuillerme, N. (2021). Spatio-temporal gait parameters obtained from foot-worn inertial sensors are reliable in healthy adults in single- and dual-task conditions. Sci. Rep. 11 (1), 10229. doi:10.1038/s41598-021-88794-4
Sun, W., Song, Q., Yu, B., Zhang, C., and Mao, D. (2015). Test-retest reliability of a new device for assessing ankle joint threshold to detect passive movement in healthy adults. J. Sports Sci. 33 (16), 1667–1674. doi:10.1080/02640414.2014.1003589
Tsang, C. S. L., Chong, D. Y. K., and Pang, M. Y. C. (2019). Cognitive-motor interference in walking after stroke: Test-retest reliability and validity of dual-task walking assessments. Clin. Rehabil. 33 (6), 1066–1078. doi:10.1177/0269215519828146
Vallabhajosula, S., Tan, C. W., Mukherjee, M., Davidson, A. J., and Stergiou, N. (2015). Biomechanical analyses of stair-climbing while dual-tasking. J. Biomech. 48 (6), 921–929. doi:10.1016/j.jbiomech.2015.02.024
Wang, C., Wang, G., Lu, A., and Zhao, Y. (2021). Effects of attentional control on gait and inter-joint coordination during dual-task walking. Front. Psychol. 12, 665175. doi:10.3389/fpsyg.2021.665175
Wang, J., Mao, M., Song, Q., Zhang, C., Chen, Y., and Sun, W. (2022). Effects of dual tasks on kinematic and kinetic performances during stair descent: Respective effects of manual and cognitive tasks. J. Sports Med. Phys. Fit. 62 (4), 492–499. doi:10.23736/s0022-4707.21.12204-2
Yogev-Seligmann, G., Hausdorff, J. M., and Giladi, N. (2012). Do we always prioritize balance when walking? Towards an integrated model of task prioritization. Mov. Disord. 27 (6), 765–770. doi:10.1002/mds.24963
Keywords: dual-task, stair walking, reliability, kinematics, kinetics
Citation: Li Y, Yu N, Zhang C, Song Q, Wang J and Sun W (2023) Test–retest reliability of kinematic and kinetic parameters during dual-task stair walking in the elderly. Front. Physiol. 14:1177159. doi: 10.3389/fphys.2023.1177159
Received: 01 March 2023; Accepted: 13 April 2023;
Published: 09 May 2023.
Edited by:
Simon Steib, Heidelberg University, GermanyReviewed by:
Zixiang Gao, Eötvös Loránd University, HungaryPedro Bezerra, Polytechnic Institute of Viana do Castelo, Portugal
Copyright © 2023 Li, Yu, Zhang, Song, Wang and Sun. This is an open-access article distributed under the terms of the Creative Commons Attribution License (CC BY). The use, distribution or reproduction in other forums is permitted, provided the original author(s) and the copyright owner(s) are credited and that the original publication in this journal is cited, in accordance with accepted academic practice. No use, distribution or reproduction is permitted which does not comply with these terms.
*Correspondence: Wei Sun, c3Vud2VpODQxMDI0QDE2My5jb20=