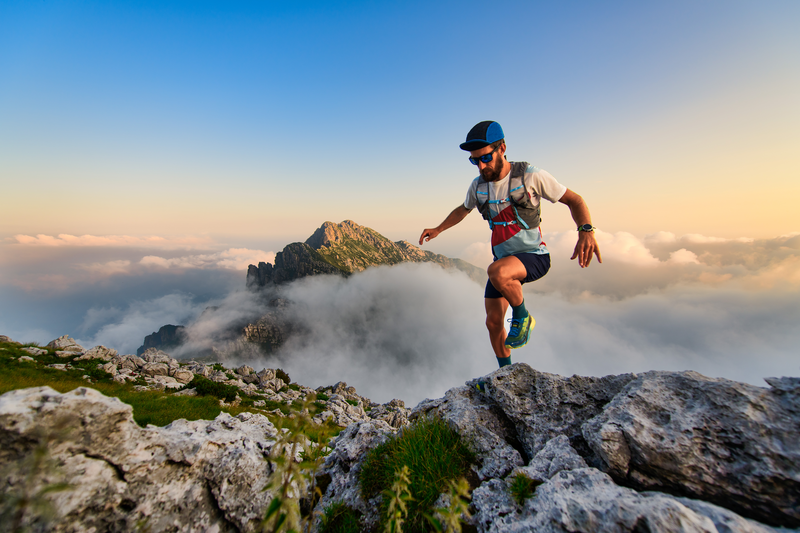
94% of researchers rate our articles as excellent or good
Learn more about the work of our research integrity team to safeguard the quality of each article we publish.
Find out more
OPINION article
Front. Physiol. , 31 March 2023
Sec. Avian Physiology
Volume 14 - 2023 | https://doi.org/10.3389/fphys.2023.1173988
This article is part of the Research Topic The Association between Avian Physiology and Meat Quality View all 12 articles
Poultry exposed to prolonged periods of thermal stress exhibit negative effects on breast meat quality through altered muscle structure, increased fat deposition, and altered protein levels. Birds are homotherms and maintain their body temperature in a narrow range (Yahav, 2000; 2015). Newly hatched poults and chicks are unable to maintain a consistent internal body temperature especially when challenged with external hot or cold temperatures (Dunnington and Siegel, 1984; Modrey and Nichelmann, 1992; Shinder et al., 2007). With more temperature extremes anticipated due to climate change, it is expected that the immediate posthatch period will result in newly hatched birds being more thermally challenged. The pectoralis major (p. major) muscle (breast muscle) has been shown to be sensitive to temperature extremes during the immediate posthatch timeframe with permanent effects on the morphological structure of the muscle including fat content and its overall development and growth (Velleman et al., 2014; Piestun et al., 2017; Patael et al., 2019; Halevy, 2020). Furthermore, chronic heat stress in chicks has been shown to increase collagen deposition (Halevy, 2020; Patael et al., 2019) and decrease circulatory supply in the p. major muscle (Hadad et al., 2014; Joiner et al., 2014). Proximity to the circulatory supply is required for activity of the adult myoblast (satellite cell) population of cells (Christov et al., 2007; Rhoads et al., 2009).
Satellite cells are responsible for posthatch muscle growth, repair and regeneration of the muscle, and are associated with the quality of poultry breast meat. Satellite cells were first reported by Mauro (1961) being localized between the basal lamina and sarcolemma of a muscle fiber. Muscle fiber formation is complete prior to hatch (Smith, 1963). After hatch, further muscle fiber growth occurs through the process of hypertrophy. Hypertrophy is dependent on satellite cell proliferation, differentiation, and fusion with existing muscle fibers donating their nuclei to increase protein synthesis potential (Moss and Leblond, 1971; Cardasis and Cooper, 1975). The first week posthatch is a period of maximal mitotic activity (Mozdziak et al., 1994; Halevy et al., 2000). During this period, satellite cells are sensitive to both cold and hot temperatures in terms of their proliferation (Xu et al., 2021), differentiation (Xu et al., 2021), cellular fate (Xu et al., 2022a), and ultimately will be a primary determinant of in vivo muscle fiber formation which will impact meat quality. For example, the proliferation (Halevy et al., 2001; Clark et al., 2016; Harding et al., 2016; Xu et al., 2021; Xu and Velleman, 2023) and differentiation (Halevy et al., 2001; Clark et al., 2016; Harding et al., 2016; Xu et al., 2021; Xu and Velleman, 2023) of satellite cells is affected by thermal stress both cold and hot. Cold temperatures inhibit both the proliferation and differentiation of satellite cells which will result in smaller myofibers and limit muscle mass accretion especially when satellite cells have high mitotic activity during the first wk posthatch and are responsive to temperature. Clark et al. (2016) in turkeys showed a linear increase in proliferation with temperature increasing from 33°C to 43°C in a stepwise manner. Similar results in chickens were reported by Harding et al. (2016). Thermal stress especially hot temperatures can increase the conversion of satellite cells to an adipogenic fate (Xu et al., 2022a) which will be associated with altered breast meat quality through increased fat content. Thus, the cellular biology of breast muscle satellite cells is central to both the morphological structure of muscle through their regulation of muscle mass accretion and meat quality. Furthermore, the repair and regeneration of myofibers back to their original state is under the control of satellite cells. If satellite cell activation back into the cell cycle from a quiescent state is suppressed or the proliferation and/or differentiation is impaired, the repair of existing muscle fibers to their original state will be negatively impacted. Interestingly, as shown by Xu et al. (2021) in turkeys that satellite cell proliferation and differentiation has increased with selection for growth whereas the opposite has occurred in broilers (Xu and Velleman, 2023). Thus, meat-type turkeys have a greater potential to repair and regenerate myofiber damage.
Satellite cells are not a homogeneous population of cells. Schultz and Lipton (1982) were the first to report satellite cell heterogeneity with proliferation being age dependent. Satellite cell heterogeneity can take many different forms with satellite cells from different muscle fiber types expressing the genes specific to the fiber type it originated from (Feldman and Stockdale, 1991; Lagord et al., 1998). Heterogeneity of satellite cells exists in a single fiber-type muscle like the turkey and chicken p. major muscle that contains homogenous Type IIb fibers. McFarland et al. (1995) and Yun et al. (1997) used single cell cloning to determine that satellite cells isolated from the p. major muscle of one turkey exhibit different rates of proliferation and differentiation and different growth factor responsiveness.
Commercial meat type poultry, chickens and turkeys, have been selected, in part, for rapid growth and heavy weight of the breast muscle. How selection for growth has affected the growth properties of satellite cells and their responsiveness to temperature is important to the morphological structure of the p. major muscle and breast meat quality. Faster growing poultry in general have a higher metabolic rate and produce more heat (Chwalibog et al., 2007; Buzala et al., 2015). To further complicate thermal regulation, growth-selected poultry tend to have reduced circulatory supply in the p. major muscle and thus reduced dissipation of heat (Yahav, 2000; Havenstein et al., 2003; Yahav et al., 2005). In general, faster growing heavy weight meat-type broilers have reduced thermal tolerance compared to slower growing lines (Yahav, 2000).
How selection for increased muscle mass accretion of the p. major muscle has impacted the biological activity of the satellite cells and response to thermal stress will affect muscle growth and development including the response to myofiber damage. Xu et al. (2021) reported results from a study comparing p. major satellite cell proliferation, differentiation, and response to hot and cold temperature in a historical turkey line representing commercial turkeys of the late 1960s (Nestor et al., 1969) to a modern-day commercial meat-type turkey line. It was found that both hot and cold thermal stress in vitro affected the proliferation and differentiation of the satellite cells isolated from both the historical and the current commercial turkey p. major muscle. Both proliferation and differentiation were increased by heat stress and reduced with cold. The cells during proliferation were more sensitive to temperature than during differentiation with temperature during proliferation having more of an effect on myotube formation. Myotube formation is the precursor to myofiber development and thus altering the proliferation process could have long-term effects on the morphological structure of the breast muscle in turkeys. Furthermore, the growth-selected faster growing modern commercial turkey satellite cells were significantly more sensitive to hot temperatures during both proliferation and differentiation. In vivo, this would result in the formation of larger diameter muscle fibers, giant fibers, reducing available connective tissue spacing between muscle fibers and bundles. In an anerobic muscle like the p. major muscle reducing the amount of available connective tissue spacing limits the area needed for capillary supply to support satellite cell activity and remove the by-products of anaerobic respiration. As shown by Wilson et al. (1990) and Velleman et al. (2003) as muscle begins to lose spacing between muscle fiber bundles and individual myofibers, degradation of the muscle fiber structure commences. Once the fibers are damaged, the fibers must be regenerated to their original state through the activation of satellite cells. If myofiber structure is not appropriately regenerated, the fiber structure of the muscle will be replaced with connective tissue and fat through fibrosis. Since the modern commercial turkey has increased proliferation and forms larger fibers with hot temperatures, it is likely to be more prone to myofiber degenerative myopathies.
Interestingly, p. major muscle necrotic/fibrotic myopathies are primarily observed in heavy weight fast growing chickens and not in meat type turkeys. This raises the issue of differences between satellite cell biological activity to regenerate muscle in chickens and turkeys, and if satellite cells between chickens and turkeys have a similar response to thermal stress. In a recent study, Xu et al. (2023) compared the current commercial meat-type chicken p. major muscle satellite cells to cell lines isolated in the 1990s. The modern commercial chicken satellite cells had decreased proliferation and differentiation and were less responsive to both hot and cold thermal stress compared to the 1990s p. major muscle satellite cells. This is completely opposite to that of the modern commercial meat-type turkey which has increased proliferation and differentiation compared to older slower-growing turkeys (Xu et al., 2021). Turkey derived p. major muscle satellite cells from current birds are also responsive to both hot and cold thermal stress during proliferation and differentiation through both the mechanistic target of rapamycin (mTOR) (Xu et al., 2022b) and Frizzled 7 (Fzd7)-mediated wingless-type mouse mammary tumor virus integration site family/planar cell polarity (Wnt/PCP) pathway (Xu et al., 2022a). The mTOR pathway stimulates protein synthesis and enhances myofiber hypertrophy (Bodine et al., 2001; Wang and Proud, 2006), whereas the Wnt/PCP is a regulator of satellite cell migration (Fortier et al., 2008; Wang et al., 2018). The modern-day commercial meat-type chicken satellite cells are less sensitive to temperature and during proliferation are more responsive to mTOR and Fzd7 expression. Taken together, the results of Xu et al. (2023) are suggestive of the modern-day broiler satellite cells having reduced regeneration potential of damaged muscle due to decreased proliferation and differentiation and temperature sensitivity of the satellite cells.
Reduced regeneration potential of broiler p. major satellite cells would result in current commercial broilers being more susceptible to the negative effects of necrotic/fibrotic myopathies like Wooden breast which are not observed in turkeys. In support of the negative impact of reduced regeneration potential of broiler satellite cells, Wooden breast affected muscle is composed of a high percentage of smaller diameter myofibers with disorganized contractile sarcomeres (Clark and Velleman, 2017; Velleman et al., 2018). Based on the reduced biological activity of modern-day broiler satellite cells, the ability to regenerate damaged myofibers is reduced leading to the necrosis and subsequent fibrosis. Furthermore, it is evident that selection for accretion of breast muscle mass in both turkeys and chickens has changed the satellite cell populations from those in slower growing or historic lines with changes in proliferation and differentiation (Xu et al., 2021; Xu et al., 2023) and in the case of turkeys documented changes in key cell surface receptors affecting satellite cell function and cellular fate (Xu et al., 2023).
In summary, both thermal stress and the biological activity of satellite cells pose multidimensional threats to the growth, development, and subsequent meat quality of the poultry breast muscle. Satellite cells have their peak mitotic activity, the first week posthatch, and during this time are responsive to extrinsic stimuli including temperature which can alter cellular fate, and proliferation and differentiation. In addition, satellite cells are not a homogenous population of cells and have been altered by selection for growth. In the case of broiler chickens, satellite cell proliferation, differentiation, and the potential to regenerate myofiber damage has declined likely being associated with the onset of fibrotic/necrotic myopathies (Xu and Velleman, 2023). Also, the satellite cell response to temperature extremes has changed in both turkeys and chickens. Selection strategies used by the poultry industry as it continues to move forward should include assessments of satellite cell biological activity and responsiveness to both hot and cold temperature extremes.
The author confirms being the sole contributor of this work and has approved it for publication.
The authors declare that the research was conducted in the absence of any commercial or financial relationships that could be construed as a potential conflict of interest.
All claims expressed in this article are solely those of the authors and do not necessarily represent those of their affiliated organizations, or those of the publisher, the editors and the reviewers. Any product that may be evaluated in this article, or claim that may be made by its manufacturer, is not guaranteed or endorsed by the publisher.
Bodine, S. C., Stitt, T. N., Gonzalez, M., Kline, W. O., Stover, G. L., Bauerlein, R., et al. (2001). Akt/mTOR pathway is a crucial regulator of skeletal muscle hypertrophy and can prevent muscle atrophy in vivo. Nat. Cell Biol. 3, 1014–1019. doi:10.1038/ncb1101-1014
Buzala, M., Janicki, B., and Czarnecki, R. (2015). Consequences of different growth rates in broiler breeder and layer hens on embryogenesis, metabolism and metabolic rate: A review. Poult. Sci. 94, 728–733. doi:10.3382/ps/pev015
Cardasis, C. A., and Cooper, G. W. (1975). An analysis of nuclear numbers in individual muscle fibers during differentiation and growth: A satellite cell-muscle fiber growth unit. J. Exp. Zool. 191, 347–358. doi:10.1002/jez.1401910305
Christov, C., Chrétien, F., Abou-Khalil, R., Bassez, G., Vallet, G., Authier, F-J., et al. (2007). Muscle satellite cells and endothelial cells: Close neighbors and privileged partners. Mol. Biol. Cell 18, 1397–1409. doi:10.1091/mbc.e06-08-0693
Chwalibog, A., Tauson, A.-H., Ali, A., Matthiesen, C., Thorhauge, K., and Thorbek, G. (2007). Gas exchange, heat production and oxidation of fat in chicken embryos from a fast or slow growing line. Comp. Biochem. Physiol. Part A Mol. Integr. Physiol. 146, 305–309. doi:10.1016/j.cbpa.2006.10.035
Clark, D. L., Coy, C. S., Strasburg, G. M., Reed, K. M., and Velleman, S. G. (2016). Temperature effect on proliferation and differentiation of satellite cells from turkeys with different growth rates. Poult. Sci. 95, 934–947. doi:10.3382/ps/pev437
Clark, D. L., and Velleman, S. G. (2017). Spatial influence on breast muscle morphological structure, myofiber size, and gene expression associated with the wooden breast myopathy in broilers. Poult. Sci. 95, 2930–2945. doi:10.3382/ps/pew243
Dunnington, E. A., and Siegel, P. B. (1984). Thermoregulation in newly hatched chicks. Poult. Sci. 63, 1303–1313. doi:10.3382/ps.0631303
Feldman, J. L., and Stockdale, F. E. (1991). Skeletal muscle satellite cell diversity: Satellite cells form fibers of different types in cell culture. Dev. Biol. 143, 320–334. doi:10.1016/0012-1606(91)90083-f
Fortier, M., Comunale, F., Kucharczak, J., Blangy, A., Charrasse, S., and Gauthier-Rouvière, C. (2008). RhoE controls myoblast alignment prior fusion through RhoA and ROCK. Cell Death Differ. 15, 1221–1231. doi:10.1038/cdd.2008.34
Hadad, Y., Cahaner, A., and Halevy, O. (2014). Featherless and feathered broilers under control versus hot conditions. 2. Breast muscle development and growth in pre- and posthatch periods. Poult. Sci. 93, 1076–1088. doi:10.3382/ps.2013-03592
Halevy, O., Geyra, A., Barak, M., Uni, Z., and Sklan, D. (2000). Early posthatch starvation decreases satellite cell proliferation and skeletal muscle growth in chicks. J. Nutr. 130, 858–864. doi:10.1093/jn/130.4.858
Halevy, O., Krispin, A., Leshem, Y., McMurty, J. P., and Yahav, S. (2001). Early-age heat exposure affects skeletal muscle satellite cell proliferation and differentiation in chicks. Am. J. Physiol. Regul. Integr. Comp. Physiol. 281, R302–R309. doi:10.1152/ajpregu.2001.281.1.R302
Halevy, O. (2020). Timing is everything-the high sensitivity of avian satellite cells to thermal conditions during embryonic and posthatch periods. Front. Physiol. 11, 235. doi:10.3389/fphys.2020.00235
Harding, R. L., Halevy, O., Yahav, S., and Velleman, S. G. (2016). The effect of temperature on proliferation and differentiation of chicken skeletal muscle satellite cells isolated from different muscle types. Physiol. Rep. 4, e12770. doi:10.14814/phy2.12770
Havenstein, G., Ferket, P., and Qureshi, M. (2003). Carcass composition and yield of 1957 versus 2001 broilers when fed representative 1957 and 2001 broiler diets. Poult. Sci. 82, 1509–1518. doi:10.1093/ps/82.10.1509
Joiner, K. S., Hamlin, G. A., Lien, R. J., and Bilgili, S. F. (2014). Evaluation of capillary and myofiber density in the pectoralis major muscles of rapidly growing, high-yield broiler chickens during increased heat stress. Avian Dis. 58, 377–382. doi:10.1637/10733-112513-Reg.1
Lagord, C., Soulet, L., Bonavaud, S., Bassaglia, Y., Rey, C., Barlovatz-Meimon, G., et al. (1998). Differential myogenicity of satellite cells isolated from extensor digitorum longus (EDL) and soleus rat muscles revealed in vitro. Cell Tissue Res. 291, 455–468. doi:10.1007/s004410051015
Mauro, A. (1961). Satellite cell of skeletal muscle fibers. J. Biophys. Biochem. Cy. 9, 493–495. doi:10.1083/jcb.9.2.493
McFarland, D. C., Pesall, J. E., Gilkerson, K. K., and Ferrin, N. H. (1995). The response to growth factors of cultured satellite cells derived from turkeys having different growth rates. Cytobios 82, 229–238.
Modrey, P., and Nichelmann, M. (1992). Development of autonomic and behavioural thermoregulation in turkeys (Meleagris gallopavo). J. Therm. Biol. 17, 287–292. doi:10.1016/0306-4565(92)90035-e
Moss, F. P., and LeBlond, C. P. (1971). Satellite cells as the source of nuclei in muscles of growing rats. Anat. Rec. 170, 421–435. doi:10.1002/ar.1091700405
Mozdziak, P. E., Schultz, E., and Cassens, R. G. (1994). Satellite cell mitotic activity in posthatch Turkey skeletal muscle growth. Poult. Sci. 73, 547–555. doi:10.3382/ps.0730547
Nestor, K., McCartney, M., and Bachev, N. (1969). Relative contributions of genetics and environment to Turkey improvement. Poult. Sci. 48, 1944–1949. doi:10.3382/ps.0481944
Patael, T., Piestun, Y., Soffer, A., Mordechay, S., Yahav, S., Velleman, S. G., et al. (2019). Early posthatch thermal stress causes long-term adverse effects on pectoralis muscle development in broilers. Poult. Sci. 98, 3268–3277. doi:10.3382/ps/pez123
Piestun, Y., Patael, T., Yahav, S., Velleman, S. G., and Halevy, O. (2017). Early posthatch thermal stress affects breast muscle development and satellite cell growth and characteristics in broilers. Poult. Sci. 96, 2877–2888. doi:10.3382/ps/pex065
Rhoads, R. P., Johnson, R. M., Rathbone, C. R., Liu, X., Temm-Grove, C., Sheehan, S. M., et al. (2009). Satellite cell-mediated angiogenesis in vitro coincides with a functional hypoxia-inducible factor pathway. Am. J. Physiol. Cell Physiol. 296, C1321–C1328. doi:10.1152/ajpcell.00391.2008
Schultz, E., and Lipton, B. H. (1982). Skeletal muscle satellite cells: Changes in proliferation potential as a function of age. Mech. Ageing Dev. 20, 377–383. doi:10.1016/0047-6374(82)90105-1
Shinder, D., Rusal, M., Tanny, J., Druyan, S., and Yahav, S. (2007). Thermoregulatory responses of chicks (Gallus domesticus) to low ambient temperatures at an early age. Poult. Sci. 86, 2200–2209. doi:10.1093/ps/86.10.2200
Smith, J. H. (1963). Relation of body size to muscle cell size and number in the chicken. Poult. Sci. 42, 283–290. doi:10.3382/ps.0420283
Velleman, S. G., Anderson, J. W., Coy, C. S., and Nestor, K. E. (2003). Effect of selection for growth rate on muscle damage during Turkey breast muscle development. Poult. Sci. 82, 1069–1074. doi:10.1093/ps/82.7.1069
Velleman, S. G., Clark, D. L., and Tonniges, J. R. (2018). The effect of the wooden breast myopathy on sarcomere structure and organization. Avian Dis. 62, 28–35. doi:10.1637/11766-110217-Reg.1
Velleman, S. G., Coy, C. S., and Emmerson, D. A. (2014). Effect of the timing of posthatch feed restrictions on the deposition of fat during broiler breast muscle development. Poult. Sci. 93, 2622–2627. doi:10.3382/ps.2014-04206
Wang, W., Chen, M., Gao, Y., Song, X., Zheng, H., Zhang, K., et al. (2018). P2Y6 regulates cytoskeleton reorganization and cell migration of C2C12 myoblasts via ROCK pathway. J. Cell. Biochem. 119, 1889–1898. doi:10.1002/jcb.26350
Wang, X., and Proud, C. G. (2006). The mTOR pathway in the control of protein synthesis. Physiology 21, 362–369. doi:10.1152/physiol.00024.2006
Wilson, B. W., Nieberg, P. S., Buhr, R. J., Kelly, B. J., and Shultz, F. T. (1990). Turkey muscle growth and focal myopathy. Poult. Sci. 69, 1553–1562. doi:10.3382/ps.0691553
Xu, J., Strasburg, G. M., Reed, K. M., Bello, N. M., and Velleman, S. G. (2023). Differential effects of temperature and mTOR and wnt-planar cell polarity pathways on syndecan-4 and CD44 expression in growth-selected Turkey satellite cell populations. PLoS One 18, e0281350. doi:10.1371/journal.pone.0281350
Xu, J., Strasburg, G. M., Reed, K. M., and Velleman, S. G. (2021). Response of Turkey pectoralis major muscle satellite cells to hot and cold thermal stress: Effect of growth selection on satellite cell proliferation and differentiation. Comp. Biochem. Physiol. Pt. A. Mol. Integr. Physiol. 252, 110823. doi:10.1016/j.cbpa.2020.110823
Xu, J., Strasburg, G. M., Reed, K. M., and Velleman, S. G. (2022a). Temperature and growth selection effects on proliferation, differentiation, and adipogenic potential of Turkey myogenic satellite cells through frizzled-7-mediated Wnt planar cell polarity pathway. Front. Physiol. 13, 892887. doi:10.3389/fphys.2022.892887
Xu, J., Strasburg, G. M., Reed, K. M., and Velleman, S. G. (2022b). Thermal stress affects proliferation and differentiation of Turkey satellite cells through the mTOR/S6K pathway in a growth-dependent manner. PLoS One 17, e0262576. doi:10.1371/journal.pone.0262576
Xu, J., and Velleman, S. G. (2023). Effects of thermal stress and mechanistic target of rapamycin and wingless-type mouse mammary tumor virus integration site family pathways on the proliferation and differentiation of satellite cells derived from the breast muscle of different chicken lines. Poult. Sci. 102, 102608. doi:10.1016/j.psj.2023.102608
Yahav, S. (2000). Domestic fowl-strategies to confront environmental conditions. Poult. Avian Biol. Rev. 11, 81–95.
Yahav, S. (2015). “Regulation of body temperature,” in Sturkie’s avian Physiology (Cambridge, MA, USA: Elsevier), 869–905.
Yahav, S., Shinder, D., Tanny, J., and Cohen, S. (2005). Sensible heat loss: The broiler's paradox. Worlds Poult. Sci. J. 61, 419–434. doi:10.1079/wps200453
Keywords: chicken, growth selection, muscle, satellite cell, temperature, turkey
Citation: Velleman SG (2023) Satellite cell-mediated breast muscle growth and repair: The impact of thermal stress. Front. Physiol. 14:1173988. doi: 10.3389/fphys.2023.1173988
Received: 25 February 2023; Accepted: 24 March 2023;
Published: 31 March 2023.
Edited by:
Krystyna Pierzchala-Koziec, University of Agriculture in Krakow, PolandReviewed by:
Xing Fu, Louisiana State University Agricultural Center, United StatesCopyright © 2023 Velleman. This is an open-access article distributed under the terms of the Creative Commons Attribution License (CC BY). The use, distribution or reproduction in other forums is permitted, provided the original author(s) and the copyright owner(s) are credited and that the original publication in this journal is cited, in accordance with accepted academic practice. No use, distribution or reproduction is permitted which does not comply with these terms.
*Correspondence: Sandra G. Velleman, dmVsbGVtYW4uMUBvc3UuZWR1
Disclaimer: All claims expressed in this article are solely those of the authors and do not necessarily represent those of their affiliated organizations, or those of the publisher, the editors and the reviewers. Any product that may be evaluated in this article or claim that may be made by its manufacturer is not guaranteed or endorsed by the publisher.
Research integrity at Frontiers
Learn more about the work of our research integrity team to safeguard the quality of each article we publish.