- 1Wuxi Fisheries College, Nanjing Agricultural University, Wuxi, China
- 2Key Laboratory of Freshwater Fisheries and Germplasm Resources Utilization, Ministry of Agriculture and Rural Affairs, Freshwater Fisheries Research Center, Chinese Academy of Fishery Sciences, Wuxi, China
- 3National Demonstration Center for Experimental Fisheries Science Education, Shanghai Ocean University, Shanghai, China
- 4Jiangsu Haorun Biological Industry Group Co., Ltd, Taizhou, China
The change in temperature will change the composition of intestinal microorganisms of juvenile Eriocheir sinensis, and the composition of intestinal microorganisms will affect the growth and development of juvenile crabs. In order to explore the relationship between intestinal microorganisms and growth of E. sinensis at different temperatures, the status of growth and intestinal microflora of juvenile E. sinensis reared at different water temperatures (15 °C, 23 °C, and 30 °C) were compared in this study. The results showed that the respective survival rate of juvenile E. sinensis in the three water temperature groups was 100%, 87.5%, and 64.44%. Moreover, the molting rate increased with an increase in water temperature, which was at 0%, 10%, and 71.11% for the three respective temperature groups. The average weight gain rate showed an overall increasing trend with the increase of water temperature. Moreover, the final fatness of the crabs in the 30 °C water temperature group was significantly lower than that in the 15 °C and 23 °C groups (p < 0.05); there was no significant difference in the liver-to-body ratio among the three groups. The results of the alpha diversity analysis of the 16S rRNA data revealed that there was no significant difference in the intestinal microbial abundance among the three water temperature groups; however, the intestinal microbial diversity in the 23 °C water temperature group was significantly lower than that in the 15 °C and 30 °C groups. At the phylum level, the dominant flora of the three groups was Firmicutes, Proteobacteria, and Bacteroidota. At the genus level, the abundance of Parabacteroides and Aeromonas in the intestine of the crabs in the 30 °C water temperature group was significantly higher than that in the 15 °C and 23 °C groups (p < 0.05). The function prediction showed that the main functional diversity of intestinal microflora of juvenile E. sinensis in the three water temperature groups was similar and mainly involved in metabolic-related functions, but there were still differences in the effects of water temperature on functional pathways such as metabolism, immunity, and growth among each group, either promoting or inhibiting. In conclusion, different water temperatures can affect the composition and function of intestinal flora of E. sinensis, and 23 °C–30 °C is the optimal water temperature for the growth of juvenile E. sinensis.
1 Introduction
Crustaceans are variable temperature animals with weak thermoregulation ability and a body temperature that directly depends on the surrounding ambient temperature. Therefore, water temperature is one of the most important environmental factors in the survival of aquatic crustaceans as it directly affects metabolism, growth, and molting (Gong et al., 2015; Wittmann et al., 2018; Shields, 2019). A study showed that a change in temperature directly affected the routine metabolic rate of Litopenaeus vannamei, and the rate increased significantly with the increase in temperature, both for fed and starved shrimp species (Walker et al., 2011). Another study demonstrated that temperature affected the growth, feeding rate, and feed conversion rate (FCR) of juvenile L. vannamei. The growth and feeding rate of L. vannamei increased directly with the increase in temperature. In addition, the data showed that the optimal temperature (the fastest growing temperature) was specific to size and decreased with the increase of the shrimp size (Wyban et al., 1995). In a certain temperature range, the development and growth rate of shrimp and crab larvae accelerate with the increase in temperature. With the increase in temperature, the larger the body length and weight of L. vannamei, the higher is its growth rate (Al-Masqari et al., 2022). The growth rate of Macrobrachium nipponense in a temperature ranged 22 °C–32 °C was significantly higher than that at 16 °C and 20 °C (Wang et al., 2006). The embryonic development process of Procambarus clarkii accelerated, and the larval development time shortened with the increase in temperature (Jin et al., 2019). This phenomenon has also been reported in the studies of Panulirus ornatus (Sachlikidis et al., 2010), Eogammarus sinensis (Xue et al., 2021), and Penaeus japonicus (Coman et al., 2002).
Neuparth et al. found that temperature was the most significant factor affecting the life cycle of Gammarus locusta. Under ambient temperature, high temperature shortened the generation time, increased the individual growth rate, accelerated sexual maturity, and increased the population density of G. locusta (Neuparth et al., 2002). Moreover, there are many reports on the effect of temperature in the growth and behavior of E. sinensis. When the water temperature is lower than 5 °C, E. sinensis enters a dormant state; at 6 °C–19 °C, it will crawl with low frequency and eat at a small amount. When the water temperature is 20 °C–26 °C, the food intake is the most exuberant (Dong et al., 2010). The results showed that the growth rate of juvenile E. sinensis (0.06–0.15 g) at 28 °C and 30 °C was significantly faster than that at 18 °C and 22 °C. The first molting period was significantly shortened with the increase in temperature. The optimum water temperature for juvenile growth and molting of E. sinensis is 28 °C–30 °C (Yuan et al., 2017). First-year juvenile E. sinensis have weak tolerance to high temperature. When the water temperature exceeds 30 °C, they will gradually die in a short period of time (Peng et al., 2019).
Intestinal microorganisms refer to the large number of microorganisms in the intestines of humans and animals. These microorganisms promote the growth and development of the host to help in food digestion, detoxify harmful molecules, provide essential nutrients, combat invasive pathogens, and regulate immunity (Engel and Moran, 2013). The development of the intestinal tract is also strongly affected by the existence and composition of intestinal microflora (Sommer and Bäckhed, 2013; Robertson et al., 2019). Genetic factors, lifestyle, and natural environment such as diet and ambient temperature (Gould et al., 2018; Osadchiy et al., 2019) affect the composition of microorganisms (Zmora et al., 2019). Changes in temperature can lead to changes in the function of intestinal flora, thus affecting the digestion and absorption of food (Sun et al., 2022). In a certain temperature range, the increase in temperature helps in colonizing some beneficial bacteria in the intestinal tract and promotes the digestion and absorption of the host. However, when the temperature is very high, it will lead to an increase in the content of pathogenic bacteria in the intestinal tract and damage to the intestinal tract, thus affecting the growth and development of the host and even leading to the death of the host. Some studies have shown that a moderate increase in water temperature promoted the proliferation of beneficial bacteria such as Bifidobacterium and Lactobacillus in the gut of L. vannamei to inhibit the colonization of pathogens, improving the digestion and absorption of food by the host (Yin et al., 2004). However, the rise in Vibrio, Candidatus Bacilloplasma, and Photobacterium spurred the invasion of pathogenic bacteria when the water temperature reached 30 °C, which hampered normal growth and development of the shrimp and ultimately resulted in death (Al-Masqari et al., 2022). Due to the diversity of living environments, feeding habits, and intestinal structure of aquatic animals, the dominant groups of microorganisms in their intestines vary. Firmicutes, Actinomycetes, Fusobacteria, and Proteobacteria dominate the intestinal tract of shrimp, while the proportion of Bacteroides is relatively low (Rungrassamee et al., 2014; Zeng et al., 2017). Compared with higher animals, fewer counts of Bacteroidota are observed in the intestinal tract of aquatic animals, while Fusobacteria and Proteobacteria predominate (Kostic et al., 2013).
There are also some reports on the relationship between temperature, gut microbiota, and host growth and development. Temperature variation structures the composition and function of gut microbiomes in animals (Sepulveda and Moeller, 2020). The destruction of gut microbiota by heat stress may ultimately affect the growth and reproductive characteristics of the host (Dubos and Schaedler, 1960; Gould et al., 2018; Moeller et al., 2019). Changes in ambient temperature could more easily alter the diversity and composition of gut microbiota of ectotherms, potentially affecting their development and health (Fontaine et al., 2018; Huyben et al., 2018; McMunn et al., 2022). Research has found that gut microbial diversity is reduced in salamanders and lizards when they experienced increases in environmental temperatures, which reduced digestive performance in the former and decreased survival in the latter (Bestion et al., 2017; Fontaine et al., 2018; Zhu et al., 2021).
There have been several reports on intestinal microorganisms of E. sinensis; however, these were mainly based on the classification and identification of dominant intestinal microflora. E. sinensis has high phenotypic plasticity, and the composition of intestinal microbial community is significantly affected by the habitat environment (Su et al., 2020; Chen et al., 2021a). Chen et al. (2015) studied the microflora in the intestines of E. sinensis cultured in Tai Lake and found that Proteobacteria, Bacteroidetes, and Tenericutes were the dominant groups. Guan et al. (2021) found that the dominant flora in the intestinal microflora of E. sinensis in an alkaline region in Northwest China was Firmicutes. In addition, there is relatively little research on the effect of temperature on the relationship between gut microbiota and growth of E. sinensis. Studies have shown that under acute heat stress, the immune function and intestinal microbial community of E. sinensis were affected. At 32 °C, the abundance of the beneficial bacteria Candidatus, Hepatoplasma, and Marinifilum decreased significantly, while the abundance of the pathogenic bacteria Rhodococcus and Morganella increased significantly, thereby affecting the normal growth and development of E. sinensis (Li et al., 2023).
With the global warming and the increase of various extreme weather events in recent years, there are more and more studies on the effects of temperature on crustaceans. However, only few studies explored the environmental factors (e.g., temperature and salinity) that may affect the intestinal microorganisms of juvenile E. sinensis. The growth and development of juvenile E. sinensis is closely related to temperature, and the change in temperature will lead to changes in the composition of intestinal flora of juvenile E. sinensis, which has a great impact on the growth and survival of the host. Currently, no study has fully studied whether the growth difference is caused by the change in intestinal microflora due to the change in temperature. In this study, three different temperature groups (15 °C, 23 °C, and 30 °C) were used to compare and analyze the growth and abundance of intestinal microflora of juvenile E. sinensis. The purpose of this study was to explore the effect of temperature on intestinal microorganisms, and evaluate the relationship between intestinal flora and growth. The results of this study can provide a reference basis for optimizing the breeding conditions of juvenile E. sinensis, promoting the healthy and rapid growth of juvenile crabs, and preventing and diagnosing crab diseases.
2 Materials and methods
2.1 Feeding and management of experimental crabs
Juvenile E. sinensis (10.4976 ± 0.38 g) were obtained from Yangcheng Lake Shrimp and the Crab Green Culture Base of Freshwater Fisheries Research Center of Chinese Academy of Fisheries Sciences. All crabs were allowed to adapt to laboratory conditions for 1 week inside a transparent glass tank (100 cm × 45 cm × 50 cm). During this period, the water quality parameters were maintained at water depth 15 cm, temperature 15°C ± 1 °C, pH 8.0 ± 0.5, dissolved oxygen ≥5.0 mg/L, ammonia ≤0.1 mg/L, and nitrite ≤0.05 mg/L (Pan et al., 2020). Juvenile E. sinensis was fed at 14:00 daily, and the remaining feed and feces were retrieved at 8:00 the next day. One-third of the water in the tank was changed regularly to keep the water quality stable.
2.2 Experimental design and sample collection
Three temperature gradients of 15 °C (low), 23 °C (mid), and 30 °C (high) were set. The temperature was adjusted using the temperature control electric heating rod and monitored in real time using a thermometer. A total of 120 juvenile E. sinensis were randomly divided into three groups: the low-temperature group (n = 35), middle-temperature group (n = 40), and high-temperature group (n = 45). Each temperature group of juvenile crabs was then randomly divided into three glass tanks for experiments. The crabs were individually fed based on 2% of the total mass at a normal time daily for 30 days, and the residual bait and feces were cleaned up every day.
2.2.1 Growth index determination
Before the start of the feeding experiment, the crabs fasted for 24 h, and their body mass, body length, body width, and body height were measured. Ten crabs were randomly sampled; their hepatopancreas and body mass were measured to calculate the initial liver-body ratio. After fasting for 24 h at the end of the feeding experiment, the body mass, body length, body width, and body height were measured, and the weight gain rate was calculated. Ten crabs were randomly selected from each group, and the hepatopancreas and body mass were measured to calculate the final liver-body ratio. During the culture experiment, the number of dead crabs was recorded to calculate the survival rate. The molting date and times for each experimental crab were recorded, and the growth index was counted.
2.2.2 Sample collection
The crabs fasted for 24 h before sampling. Twelve crabs were selected for each water temperature group, and the intestines were collected and mixed into six replicates for sequencing and analysis of intestinal microbial communities. In a sterile environment, the intestines were removed with tweezers and placed in a 1.5-mL centrifuge tube. The samples were flash frozen with liquid nitrogen and stored in a refrigerator at −80 °C. The animals used in the experiment were approved by the Freshwater Fisheries Research Center, and all experimental procedures were performed in accordance with the Animal Protection Guide.
2.3 Extraction and PCR amplification of total DNA of intestinal flora
The genomic DNA of the sample was extracted using the CTAB method. The purity and concentration of the extracted DNA were detected by agarose gel electrophoresis. Subsequently, sufficient amount of DNA was taken in the centrifuge tube and diluted to 1 ng/μL with sterile water. Using the diluted genomic DNA as the template, the specific primers with barcode, Phusion ®High-Fidelity PCR Master Mix with GC Buffer of New England Biolabs, and high efficiency and high-fidelity enzyme were selected according to the amplification region to ensure the amplification efficiency and accuracy. The PCR products were detected by 2% agarose gel electrophoresis, and the target bands were recovered using the gel recovery kit (QIAGEN). The library was constructed using the NEBNext® Ultra IIDNA Library Prep Kit, and the constructed library was quantified using Qubit and Q-PCR. The library was sequenced using the NovaSeq 6000 System.
2.4 Sequencing data processing and analysis of intestinal microorganisms
According to the barcode sequence and PCR amplification sequence, the sample data were separated from the offline data, and the barcode and primer sequences were truncated. The sample reads were then spliced using FLASH (V1.2.11, http://ccb.jhu.edu/software/FLASH/) (Magoč and Salzberg, 2011) to obtain the raw tags. Quality control of the raw tags was assessed using fastp, and high-quality clean tags were obtained. The USEARCH software application is used to compare the clean tags with the database to detect and remove chimerism (Haas et al., 2011) to obtain the final effective tags. The DADA2 module of QIIME2 software was used to reduce noise and filter out the sequences with abundance <5, obtaining the final amplified subsequence variation (ASV) and feature table. The classify-sklearn module of QIIME2 software was used to compare the ASVs with the database to obtain the corresponding species information. Observed_otus, Shannon, Simpson, chao1, goods_coverage, dominance, and pielou_e indices were calculated using QIIME2 software. Moreover, QIIME2 software was used to calculate the UniFrac distance, and R software was used to perform PCA, PCoA, and NMDS dimensionality reduction maps. Subsequently, adonis and anosim functions in QIIME2 software were used to analyze the significant differences in community structure between groups. LEfSe or R software (R Foundation for Statistical Computing, Vienna, Austria) was used to analyze the significant species differences between groups. SPSS version 23.0 (IBM Corp., Armonk, NY, United States) was used to analyze the statistical differences.
3 Results
3.1 Growth performance of juvenile E. sinensis at different water temperatures
The survival rate of the experimental crabs in the 30-day feeding experiment gradually decreases with the increase in water temperature. Specifically, the survival rate of experimental crabs at 15 °C water temperature was 100% and dropped to 64.44% at 30 °C. The molting rate was proportional to the water temperature. The experimental crab in the 15 °C water temperature group did not molt; however, 10% and 71.11% of the crabs molted in the 23 °C and 30 °C water temperature groups, respectively. With the increase in water temperature, the average weight gain rate showed an overall increasing trend and the differences between groups were significant. The final fatness of crabs in the 30 °C water temperature group was significantly lower than that in the 15 °C and 23 °C (p < 0.05) water temperature groups, and there was no significant difference in the liver-body ratio among the three groups (p < 0.05). However, compared with the initial liver-body ratio, the final liver-body ratio showed a downward trend. The molting and growth characteristics of juvenile E. sinensis are shown in (Table 1).
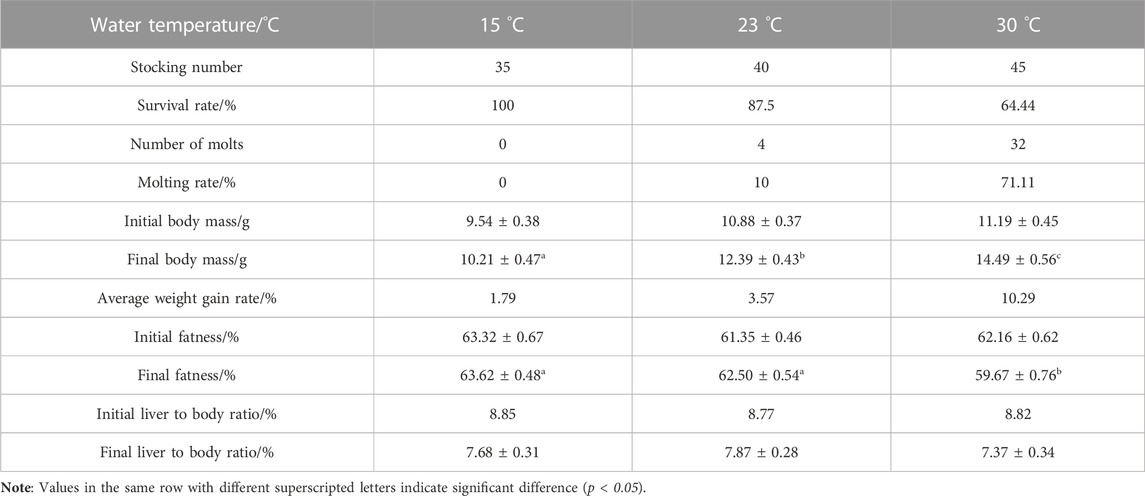
TABLE 1. Molting and growth characteristics of juvenile E. sinensis cultured under different water temperatures.
3.2 Intestinal microbiological analysis
3.2.1 Results of ASV cluster analysis
A total of 3263 ASVs were obtained from 18 samples. Moreover, 1901, 1555, and 1642 representative ASVs were obtained in 15 °C, 23 °C and 30 °C water temperature groups, respectively. The results showed that with the increase in temperature, the number of ASVs initially decreased and gradually increased. The total number of ASVs in the three groups was 542, while the number of endemic ASVs was 732, 597, and 641 for the respective groups (Figure 1).
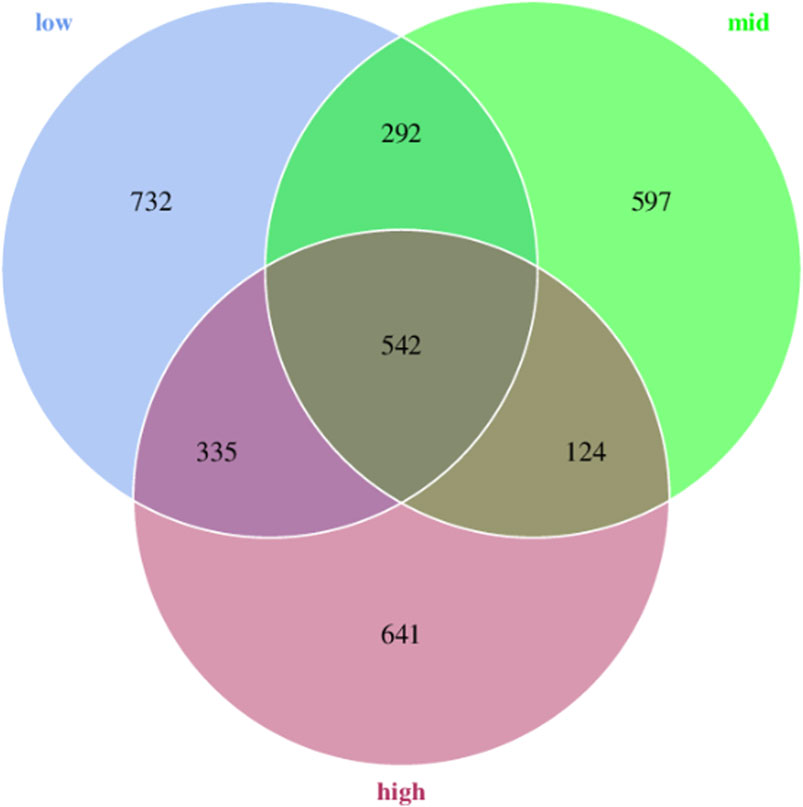
FIGURE 1. Venn diagram analysis of ASVs in the intestinal microorganisms of juvenile E. sinensis at different water temperatures.
3.2.2 Results of diversity analysis
After feeding for 30 days at different water temperatures, the PCoA of intestinal microbial composition of juvenile E. sinensis showed that the contribution of principal components to sample differences was 40.66% and 18.37% (Figure 2). Alpha diversity analysis showed that Simpson and Pielou_e indexes in the 23 °C water temperature group were significantly lower than those in the 15 °C and 30 °C groups (p < 0.05), while Simpson and Pielou_e indexes initially decreased and gradually increased, indicating that species diversity and evenness increased at first and then decreased with the increase in water temperature. There was no significant difference in the Chao and Shannon index among the three groups, however, all showed an increasing trend and decreased subsequently (Table 2).
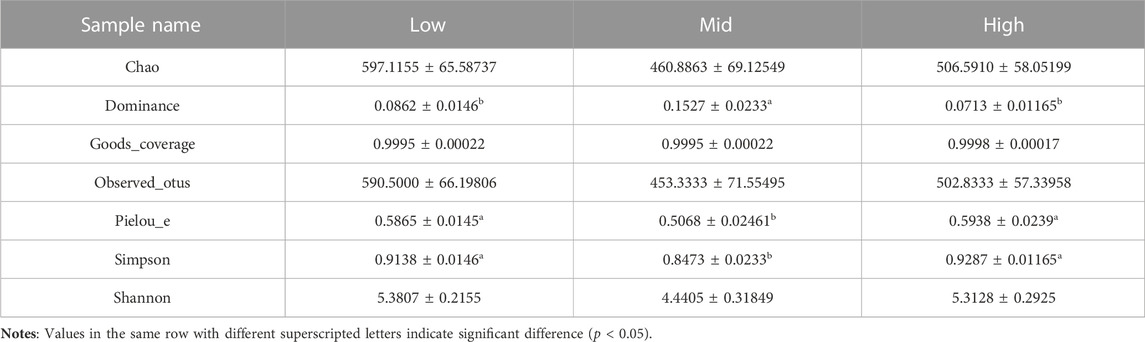
TABLE 2. Alpha diversity of intestinal bacterial community of juvenile E. sinensis at different water temperatures.
3.2.3 Composition of intestinal microflora of juvenile E. sinensis under different water temperatures
The top 10 microorganisms at the phylum level in the intestinal abundance of juvenile E. sinensis reared at different water temperatures include Firmicutes, Proteobacteria, Bacteroidota, Fusobacteriota, Patescibacteria, Actinobacteriota, Campylobacterota, Gemmatimonadota, Verrucomicrobiota, and Acidobacteriota. The dominant flora of the three groups was Firmicutes, Proteobacteria, and Bacteroidota, which accounted for 90.75% of the intestinal flora of juvenile E. sinensis (Figure 3). There was no significant difference in the composition and abundance of intestinal microflora among different water temperature groups; however, the abundance of Firmicutes decreased with the increase in water temperature, while the abundance of Proteobacteria changed in direct proportion to the water temperature. The abundance of Bacteroidota decreased at first and gradually increased with the increase in water temperature.
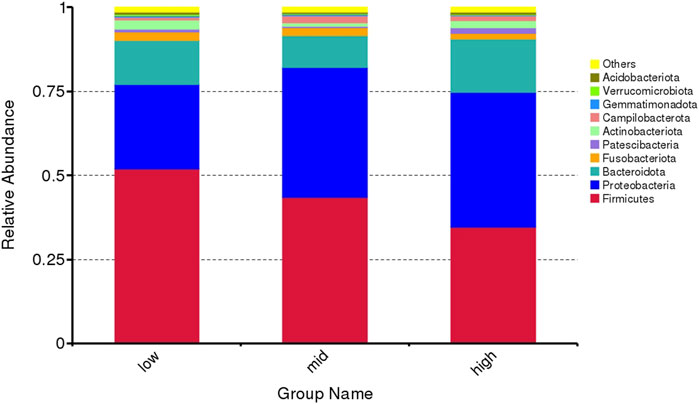
FIGURE 3. Microflora abundance at the phylum level of intestinal microorganisms in juvenile E. sinensis at different water temperatures.
The top 10 abundant genera are Candidatus Bacilloplasma, Vibrio, Parabacteroides, Shewanella, Aeromonas, Dysgonomonas, Lactovum, Fusobacterium, Rosmarinus, and Saccharimonadales (Figure 4). Among them, the abundance of Parabacteroides and Aeromonas in crab intestine in the 30 °C water temperature group was significantly higher than that in the 15 °C and 23 °C groups (Figure 5, p < 0.05).
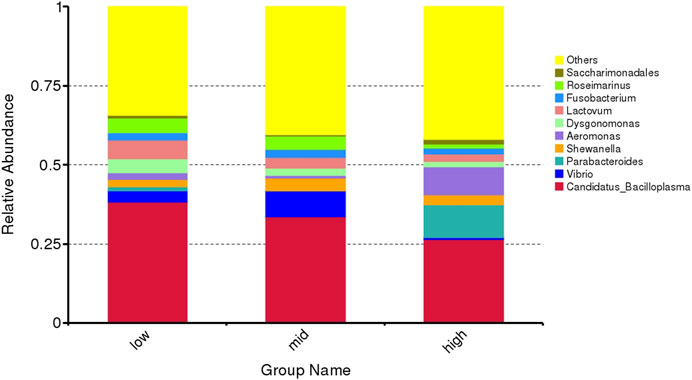
FIGURE 4. Microflora abundance at the genus level of intestinal microorganisms in juvenile E. sinensis at different water temperatures.
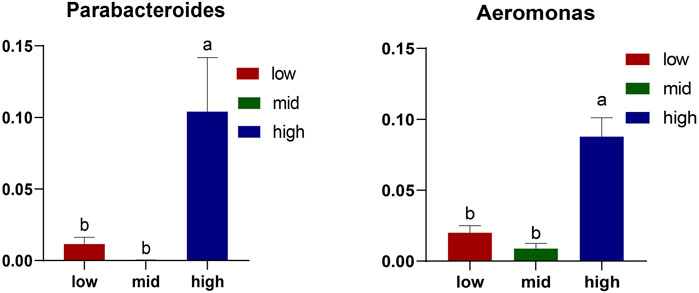
FIGURE 5. Differential microorganisms at the genus level. The results are expressed as mean ± SEM (n = 6); different letters indicate significate differences among different groups (p < 0.05).
3.2.4 Analysis of the difference of the intestinal microbial community
Using LEfSe algorithm analysis, the evolutionary branch diagram of microorganisms in the intestine was obtained (Figure 6). The results showed that the 30 °C water temperature group (high) was the most differentially abundant, followed by 15 °C (low), with the 23 °C (mid) water temperature group having the least abundant.
Through the analysis of microorganisms with an LDA value <4 in each group (Figure 7), the 30 °C water temperature group (high) is the group with the most number of microbial species (eight species), which include Proteobacteria, Tannerellaceae, Parabacteroides, Blattella germanica, Aeromonadales, Aeromonadaceae, Aeromonas, and Pseudaeromonas sharmana. This is followed by the 15 °C water temperature group (low), with six species namely Prolixibacteraceae, Rosmarinus, Lachnospiraceae, Lachnospiraceae, Tyzzerella, and Clostridia. In the 23 °C water temperature group (mid), there were four species, namely, Gammaproteobacteria, Vibrionaceae, Vibrio, and Vibrionales.
3.2.5 Prediction of the function of intestinal microflora
Based on the KEGG database, the potential function of intestinal flora of juvenile E. sinensis in different water temperature groups was predicted by Tax4Fun. The results showed that there was no significant difference in the prediction of main functions among the three water temperature groups. In terms of “Metabolism,” intestinal microorganisms of juvenile E. sinensis play an important role in “Carbohydrate metabolism” and “Amino acid metabolism.” The main roles in “Genetic Information Processing” are “Translation” and “Replication and repair;” “Environmental Information Processing” mainly involves “Membrane transport” and “Signal transduction.” However, a minimal role involving “Signaling molecules and interaction” was detected (Figure 8). At KEGG level 3, the difference of gene function was shown by a heatmap (Figure 9). Function prediction revealed that the intestinal microflora in the 15 °C water temperature group was higher in “Exosome,” “Glycolysis/Gluconeogenesis,” and “Mitochondrial biogenesis” pathways. Moreover, the “Alanine, aspartate, and glutamate metabolism” pathway was more enriched in the 23 °C water temperature group. Meanwhile, the intestinal microflora in the 30 °C water temperature group was highly enriched in “Pyruvate metabolism,” “Glyoxylate and dicarboxylate metabolism,” and “Citrate cycle (TCA cycle)” pathways. A t-test analysis was performed on the functional differences of KEGG level 3 among the three water temperature groups (Figures 10–12). The results indicated that 23 pathways mainly including “Ribosome_biogenesis”, “Secretion_system”, and “Glycolysis/Gluconeogenesis” showed a maximum difference between the 15 °C and the 23 °C water temperature groups (p < 0.05). In total, 50 pathways mainly including “Transporters,” “DNA_repair_and_recombination_proteins,” and “ABC_transporters” showed a maximum difference between the 15 °C and the 30 °C water temperature groups (p < 0.05). Furthermore, 20 pathways mainly including “Transfer_RNA_biogenesis,” “Pyruvate_metabolism,” and “Propanoate_metabolism” showed a maximum difference between the 23 °C and 30 °C water temperature groups (p < 0.05).
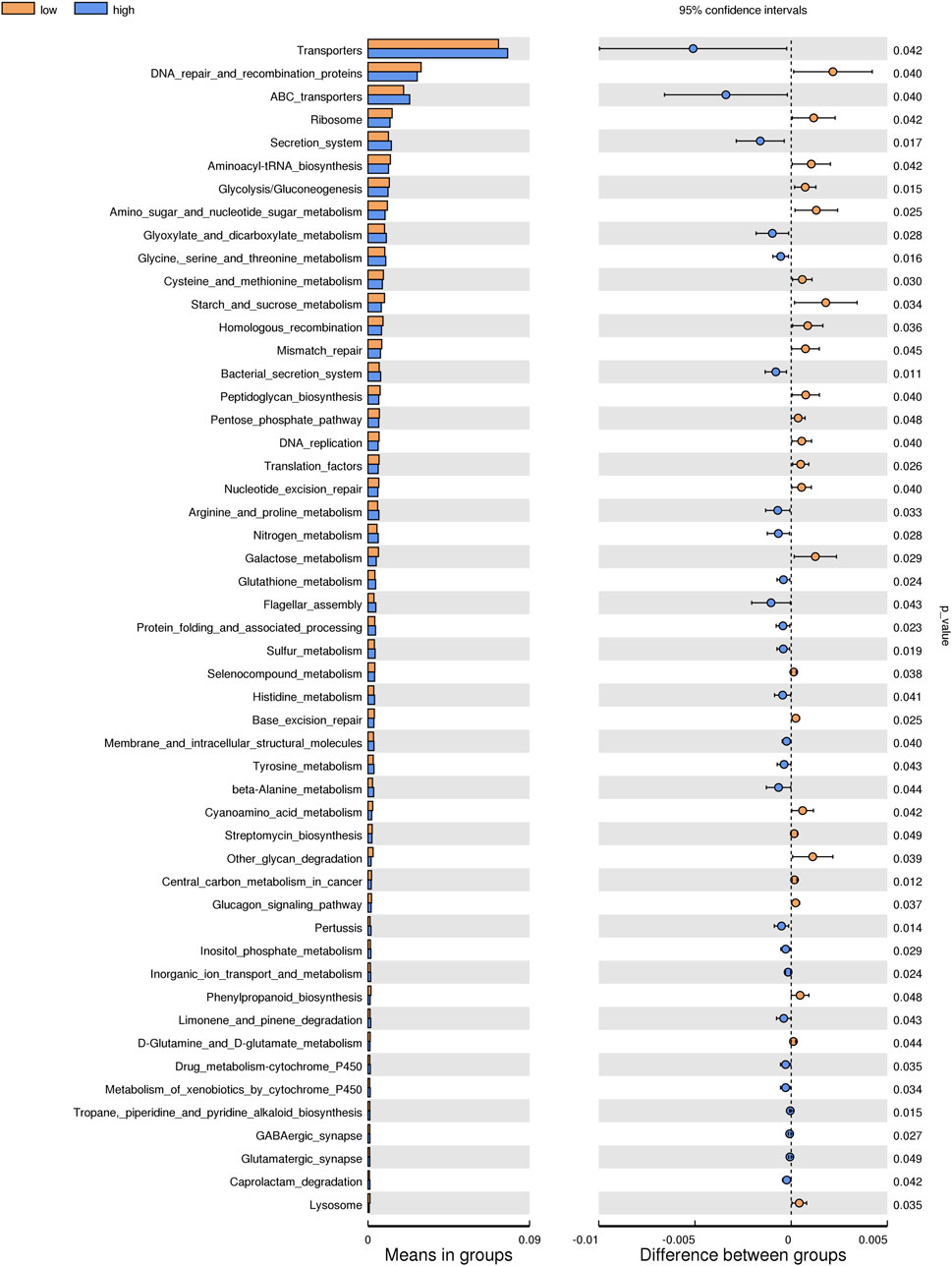
FIGURE 11. KEGG level analysis of predicted functional differences between low group and high group.
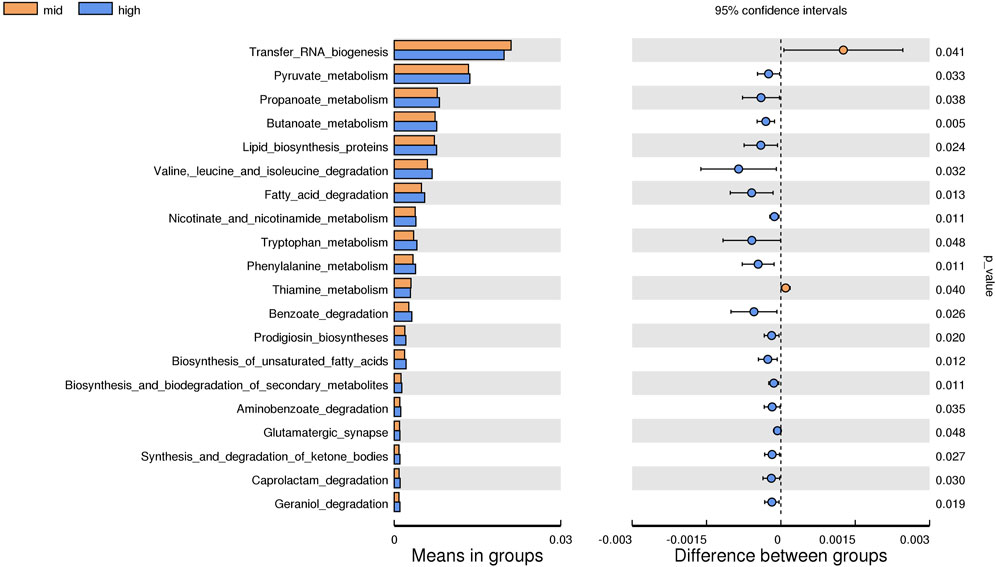
FIGURE 12. KEGG level analysis of predicted functional differences between mid group and high group.
4 Discussion
Temperature is one of the most important external factors affecting the growth and development of crustaceans, as well as the culture of juvenile E. sinensis. In this study, the effects of three different water temperatures on the growth and intestinal microorganisms of juvenile E. sinensis were studied. The results showed that the molting and weight gain rate of experimental crabs increased significantly with the increase in water temperature, which was consistent with the results of Yuan et al. (2017), and reflected the promoting effect of temperature on the growth and development of crustaceans. However, with the increase in temperature, the survival rate of experimental crabs decreased substantially. A higher water temperature can change the concentration of ammonia nitrogen and total nitrogen, which can spoil the aquaculture water body, leading to low survival and mortality among E. sinensis (Peng et al., 2019). E. sinensis may become weaker and be at a higher risk of mortality if the water temperature exceeds the physiological bearing limit of E. sinensis. Studies have shown that in a certain temperature range, the higher the temperature, the more exuberant the metabolism and gonadal development of E. sinensis will be, leading to precocious puberty, thus inhibiting its growth (Li et al., 2011). With the increase in temperature, the fatness and liver-body ratio of experimental crabs showed a downward trend, which confirmed that higher temperature can lead to the occurrence of precocious puberty, thus affecting the size of adult crabs. Since this experiment was performed under laboratory conditions, the water temperature is constant, which better reflects the effect of temperature on the growth of the juvenile E. sinensis. Based on our findings, the optimum water temperature for the growth of juvenile E. sinensis is approximately between 23 °C and 30 °C.
Intestinal microorganisms form a colony in the intestinal tract and play an important role in the growth and development, physiological balance, intestinal development, metabolism, and immune regulation of the host (Kwong and Moran, 2016; Zhang et al., 2019; Yukgehnaish et al., 2020). Temperature has an important effect on the composition and metabolites of intestinal microorganisms. It was found that both low- and high-temperature heat stress could change the abundance of host intestinal microflora (Liu et al., 2020). Ramos-Romero et al. (2020) found that low temperature increased the abundance of the Clostridium subgroup and Enterobacter, and decreased the abundance of Lactobacillus in rats. Kohl and Yahn (2016) found that a warm environment significantly increased the relative abundance of Planctomycetes and Mycobacterium in tadpole intestinal microflora. In this study, the results of alpha diversity analysis showed that the Chao index values of the 23 °C and 30 °C water temperature groups were lower than that of the 15 °C group, which indicated that the abundance of intestinal microorganisms decreased with the increase in temperature. This suggests that lower temperature was more beneficial to the survival and reproduction of intestinal microorganisms, which was consistent with the results of Zhang et al. (2022). However, the Simpson and Pielou_e indexes of the 23 °C group were significantly lower than those of 15 °C and 30 °C water temperature groups. The diversity and evenness of intestinal microorganisms in juvenile E. sinensis decreased at first and then increased with the increase in water temperature, but the numerical difference was small. We speculated that compared with the ambient temperature of 15 °C, the intestinal microbial diversity and evenness of the 23 °C group decreased slightly due to temperature stress, while the 30 °C group was close to the optimum survival temperature of juvenile crabs. Therefore, the microbial diversity and evenness of the 30 °C group are higher than those of the 23 °C group, and the specific reasons need to be further studied. Concurrently, the growth of the juvenile E. sinensis was not optimal at 23 °C, indicating that a water temperature of 23 °C was not ideal for the growth of the juvenile E. sinensis. Optimal proportion of microflora but not higher abundance and diversity can be indicative of better growth for juvenile E. sinensis (Chen et al., 2021b), making intestinal microorganisms reach a stable state in the host, thus promoting its growth and development.
The abundance and proportion of Firmicutes and Bacteroidota flora, two dominant intestinal microflora, play an important role in the health and metabolism of the host (Singh et al., 2017; Lin et al., 2019). In mammals, energy accumulation is related to the distribution of intestinal flora. When intestinal Firmicutes is more abundant than Bacteroidota, the host can absorb and utilize the heat in food more effectively (Mazier et al., 2021). In this study, Firmicutes are the most abundant in the three temperature groups, followed by Proteobacteria and Bacteroidota. However, with the increase in temperature, the abundance of Firmicutes decreased, while Proteobacteria increased gradually. The abundance of Proteobacteria in the intestines of the crabs in the three respective water temperature groups was 25.1%, 38.56%, and 40.09%. Studies have shown that Proteobacteria is a dominant microbiota of the intestinal tract of the crustaceans (Zhang et al., 2014; Sun et al., 2018). As a group of Gram-negative bacteria, it plays an important role in nutritional circulation and mineralization of organic compounds (Kirchman, 2002; Liu et al., 2021). Concomitantly, Proteobacteria, including several pathogens, is the largest phylum of bacteria (Han et al., 2019), and thus, its continuous accumulation can represent the health of the host or the imbalance structure of intestinal microorganisms (Shin et al., 2015). Sun et al. (2018) speculated that the increase in relative abundance of Proteobacteria may be the reason for the poor growth performance of Portunus trituberculatus. We believe that in the early stage, with the increase in water temperature, the content of beneficial bacteria in the intestines of juvenile E. sinensis increases, promoting their digestion and absorption of food, and accelerating their growth. Concurrently, with the increase in stress time, a relatively higher temperature can lead to the rapid reproduction of pathogens, destroying the balance of intestinal microorganisms of the juvenile E. sinensis.
Candidatus_Bacilloplasma is the most abundant genus in the intestine of juvenile E. sinensis. Its abundance in the 15 °C and 23 °C water temperature groups is higher than that in the 30 °C water temperature group. Some studies have shown that the significant increase in Candidatus_Bacilloplasma and Phascolarctobacterium and the decrease in Paracoccus and Lactococcus may lead to white feces syndrome (WFS) of the Pacific white shrimp (Kostanjsek et al., 2007; Hou et al., 2018). Moreover, the abundance of Vibrio in the 15 °C and 23 °C groups was significantly higher than that in the 30 °C water temperature group. Some studies have shown that the presence of Vibrio may lead to intestinal diseases (Almagro-Moreno et al., 2015; Baker-Austin et al., 2018). The intestinal diseases caused by these pathogenic bacteria have a considerable impact on the growth and development of crabs, which is consistent with the growth of crabs in the 15 °C and 23 °C groups. The abundance of Parabacteroides and Aeromonas in crab intestine in the 30 °C water temperature group was significantly higher than that in the 15 °C and 23 °C groups (p < 0.05). Parabacteroides is a group of gram-negative anaerobes, which usually colonized the gastrointestinal tract of many species. Currently, the genus consists of 15 species. Parabacteroides distasonis is a typical strain of Parabacteroides, which plays an important role in alleviating host obesity, metabolic dysfunction (Wang et al., 2019), and enteritis (Kverka et al., 2011). This can promote the growth of crabs in the 30 °C water temperature group. Aeromonas is a facultative anaerobe with an optimal growth temperature of 30 °C. It can cause several intestinal diseases in humans and animals. Studies have shown that injecting Aeromonas into Ictalurus punctatus may lead to septicemia and can seriously affect the health of the host (Zhang et al., 2016; Ran et al., 2018). We speculate that this is an important reason for the higher mortality of crabs in the 30 °C water temperature group.
The results of predicting the gene function of intestinal microorganisms in juvenile E. sinensis showed that the functional diversity of intestinal microflora in the three water temperature groups was similar and mainly concentrated in metabolism, including carbohydrate and amino acid metabolism, which was consistent with other crustacean studies (Sun and Xu, 2021; Yan et al., 2021). Past studies have shown that Firmicutes, Proteobacteria, Actinobacteria, and Bacteroides play important roles in carbohydrate fermentation, polysaccharide catabolism, and amino acid and protein utilization (Flint et al., 2012; Flint et al., 2015). This is consistent with the results that the dominant flora of the three water temperature groups is Firmicutes, Proteobacteria, and Bacteroidota. In this study, the pyruvate metabolism, glyoxylate and dicarboxylate metabolism, and citrate cycle were more abundant in the 30 °C water temperature group, which might provide more energy for growth and other physiological activities (Zhang et al., 2020). Although the main functional diversity of intestinal flora in the three water temperature groups was similar, there were still differences in the effects of water temperature on functional pathways such as metabolism, immunity, and growth among each group, either promoting or inhibiting. Thus, our data indicated that different water temperatures can affect the composition and function of intestinal microorganisms. In addition, information regarding the function of intestinal microbiota in crustacean is currently limited and further investigation needs to be carried out in future study.
5 Conclusion
In summary, the results of this study show that temperature has a substantial effect on the growth and development of juvenile E. sinensis. Crabs raised at 15 °C grow slowly and can further grow with the increase in temperature. However, when the temperature reaches 30 °C, a substantial number of crabs may face mortality. Concomitantly, different temperatures alter the intestinal flora composition and function of juvenile E. sinensis, and high temperature can lead to the proliferation of pathogenic bacteria. Therefore, water temperature between 23 °C and 30 °C is most beneficial to the growth of juvenile E. sinensis.
Data availability statement
The original contributions presented in the study are included in the article/supplementary material; further inquiries can be directed to the corresponding author.
Ethics statement
The animals used in the experiment were approved by the Freshwater Fisheries Research Center, and all experimental procedures were performed in accordance with the Animal Protection Guide.
Author contributions
ML: methodology, formal analysis, and writing—original draft. YT: ideas, formulation of overarching research goals and aims, and project administration. WF: writing—review and editing. WeL and XC: investigation and validation. JL: resources, investigation, and visualization. All authors contributed to the article and approved the submitted version.
Funding
This work was supported by the Jiangsu Revitalization of Seed Industry (JBGS(2021)031), the Key Project for Jiangsu Agricultural New Variety Innovation (PZCZ201749), the Central Public-interest Scientific Institution Basal Research Fund, CAFS (2020TD36), and the Key Research and Development Program of Jiangsu Province (BE2022360).
Acknowledgments
The authors thank the students and staff of the Aquatic Genetic Laboratory, FFRC, for their assistance in this study.
Conflict of interest
The author WL is employed by Jiangsu Haorun Biological Industry Group Co., Ltd.
The remaining authors declare that the research was conducted in the absence of any commercial or financial relationships that could be construed as a potential conflict of interest.
Publisher’s note
All claims expressed in this article are solely those of the authors and do not necessarily represent those of their affiliated organizations, or those of the publisher, the editors, and the reviewers. Any product that may be evaluated in this article, or claim that may be made by its manufacturer, is not guaranteed or endorsed by the publisher.
References
Almagro-Moreno, S., Pruss, K., and Taylor, R. K. (2015). Intestinal colonization dynamics of Vibrio cholerae. PLoS Pathog. 11, e1004787. doi:10.1371/journal.ppat.1004787
Al-Masqari, Z. A., Guo, H., Wang, R., Yan, H., Dong, P., Wang, G., et al. (2022). Effects of high temperature on water quality, growth performance, enzyme activity and the gut bacterial community of shrimp (Litopenaeus vannamei). Aquac. Res. 53, 3283–3296. doi:10.1111/are.15836
Baker-Austin, C., Oliver, J. D., Alam, M., Ali, A., Waldor, M. K., Qadri, F., et al. (2018). Vibrio spp. infections. Nat. Rev. Dis. Prim. 4, 8–19. doi:10.1038/s41572-018-0005-8
Bestion, E., Jacob, S., Zinger, L., Di, G. L., Richard, M., White, J., et al. (2017). Climate warming reduces gut microbiota diversity in a vertebrate ectotherm. Nat. Ecol. Evol. 1, 0161. doi:10.1038/s41559-017-0161
Chen, X., Chen, H., Liu, Q., Ni, K., Ding, R., Wang, J., et al. (2021a). High plasticity of the gut microbiome and muscle metabolome of Chinese mitten crab (Eriocheir sinensis) in diverse environments. J. Microbiol. Biotechnol. 31, 240–249. doi:10.4014/jmb.2011.11018
Chen, X., Di, P., Wang, H., Li, B., Pan, Y., Yan, S., et al. (2015). Bacterial community associated with the intestinal tract of Chinese mitten crab (Eriocheir sinensis) farmed in Lake Tai, China. PloS one 10, e0123990. doi:10.1371/journal.pone.0123990
Chen, X., Lu, D., Li, Z., Yue, W., Wang, J., Jiang, X., et al. (2021b). Plant and animal-type feedstuff shape the gut microbiota and metabolic processes of the Chinese mitten crab Eriocheir sinensis. Front. Veterinary Sci. 8, 589624. doi:10.3389/fvets.2021.589624
Coman, G. J., Crocos, P. J., Preston, N. P., and Fielder, D. (2002). The effects of temperature on the growth, survival and biomass of different families of juvenile Penaeus japonicus Bate. Aquaculture 214, 185–199. doi:10.1016/S0044-8486(02)00361-7
Dong, Y., Jiang, H., Yu, Y., Zhou, S., and Sun, B. (2010). Water-temperature characteristics of rice-field-crab" Panshan mode. Agric. Sci. Technology-Hunan 11, 152–155. doi:10.3969/j.issn.1009-4229-B.2010.04.042
Dubos, R. J., and Schaedler, R. W. (1960). The effect of the intestinal flora on the growth rate of mice, and on their susceptibility to experimental infections. J. Exp. Med. 111, 407–417. doi:10.1084/jem.111.3.407
Engel, P., and Moran, N. A. (2013). The gut microbiota of insects - diversity in structure and function. FEMS Microbiol. Rev. 37, 699–735. doi:10.1111/1574-6976.12025
Flint, H. J., Duncan, S. H., Scott, K. P., and Louis, P. (2015). Links between diet, gut microbiota composition and gut metabolism. Proc. Nutr. Soc. 74, 13–22. doi:10.1017/S0029665114001463
Flint, H. J., Scott, K. P., Louis, P., and Duncan, S. H. (2012). The role of the gut microbiota in nutrition and health. Nat. Rev. Gastroenterology hepatology 9, 577–589. doi:10.1038/nrgastro.2012.156
Fontaine, S. S., Novarro, A. J., and Kohl, K. D. (2018). Environmental temperature alters the digestive performance and gut microbiota of a terrestrial amphibian. J. Exp. Biol. 221, jeb187559. doi:10.1242/jeb.187559
Gong, J., Yu, K., Shu, L., Ye, H., Li, S., and Zeng, C. (2015). Evaluating the effects of temperature, salinity, starvation and autotomy on molting success, molting interval and expression of ecdysone receptor in early juvenile mud crabs, Scylla paramamosain. J. Exp. Mar. Biol. Ecol. 464, 11–17. doi:10.1016/j.jembe.2014.12.008
Gould, A. L., Zhang, V., Lamberti, L., Jones, E. W., Obadia, B., Korasidis, N., et al. (2018). Microbiome interactions shape host fitness. Proc. Natl. Acad. Sci. 115, E11951–E11960. doi:10.1073/pnas.1809349115
Guan, W., Li, K., Zhao, S., and Li, K. (2021). A high abundance of Firmicutes in the intestine of Chinese mitten crabs (Eriocheir sinensis) cultured in an alkaline region. Amb. Express 11, 141–149. doi:10.1186/s13568-021-01301-w
Haas, B. J., Gevers, D., Earl, A. M., Feldgarden, M., Ward, D. V., Giannoukos, G., et al. (2011). Chimeric 16S rRNA sequence formation and detection in Sanger and 454-pyrosequenced PCR amplicons. Genome Res. 21, 494–504. doi:10.1101/gr.112730.110
Han, F., Wang, X., Guo, J., Qi, C., Xu, C., Luo, Y., et al. (2019). Effects of glycinin and β-conglycinin on growth performance and intestinal health in juvenile Chinese mitten crabs (Eriocheir sinensis). Fish shellfish Immunol. 84, 269–279. doi:10.1016/j.fsi.2018.10.013
Hou, D., Huang, Z., Zeng, S., Liu, J., Wei, D., Deng, X., et al. (2018). Intestinal bacterial signatures of white feces syndrome in shrimp. Appl. Microbiol. Biotechnol. 102, 3701–3709. doi:10.1007/s00253-018-8855-2
Huyben, D., Sun, L., Moccia, R., Kiessling, A., Dicksved, J., and Lundh, T. (2018). Dietary live yeast and increased water temperature influence the gut microbiota of rainbow trout. J. Appl. Microbiol. 124, 1377–1392. doi:10.1111/jam.13738
Jin, S., Jacquin, L., Huang, F., Xiong, M., Li, R., Lek, S., et al. (2019). Optimizing reproductive performance and embryonic development of red swamp crayfish Procambarus clarkii by manipulating water temperature. Aquaculture 510, 32–42. doi:10.1016/j.aquaculture.2019.04.066
Kirchman, D. L. (2002). The ecology of Cytophaga–Flavobacteria in aquatic environments. FEMS Microbiol. Ecol. 93, 91–100. doi:10.1111/j.1574-6941.2002.tb00910.x
Kohl, K. D., and Yahn, J. (2016). Effects of environmental temperature on the gut microbial communities of tadpoles. Environ. Microbiol. 18, 1561–1565. doi:10.1111/1462-2920.13255
Kostanjsek, R., Strus, J., and Avgustin, G. (2007). Candidatus Bacilloplasma," a novel lineage of Mollicutes associated with the hindgut wall of the terrestrial isopod Porcellio scaber (Crustacea: Isopoda). Appl. Environ. Microbiol. 73, 5566–5573. doi:10.1128/AEM.02468-06
Kostic, A. D., Howitt, M. R., and Garrett, W. S. (2013). Exploring host-microbiota interactions in animal models and humans. Genes and Dev. 27, 701–718. doi:10.1101/gad.212522.112
Kverka, M., Zakostelska, Z., Klimesova, K., Sokol, D., Hudcovic, T., Hrncir, T., et al. (2011). Oral administration of Parabacteroides distasonis antigens attenuates experimental murine colitis through modulation of immunity and microbiota composition. Clin. Exp. Immunol. 163, 250–259. doi:10.1111/j.1365-2249.2010.04286.x
Kwong, W. K., and Moran, N. A. (2016). Gut microbial communities of social bees. Nat. Rev. Microbiol. 14, 374–384. doi:10.1038/nrmicro.2016.43
Li, X., Li, Z., Liu, J., De Silva, S. S., and Liu, T. (2011). Advances in precocity research of the Chinese mitten crab Eriocheir sinensis. Aquac. Int. 19, 251–259. doi:10.1007/978-3-642-23629-7_31
Li, Z., Zhao, Z., Luo, L., Wang, S., Zhang, R., Guo, K., et al. (2023). Immune and intestinal microbiota responses to heat stress in Chinese mitten crab (Eriocheir sinensis). Aquaculture 563, 738965. doi:10.1016/J.AQUACULTURE.2022.738965
Lin, S., Wang, Z., Lam, K. L., Zeng, S., Tan, B. K., and Hu, J. (2019). Role of intestinal microecology in the regulation of energy metabolism by dietary polyphenols and their metabolites. Food and Nutr. Res. 63, 63. doi:10.29219/fnr.v63.1518
Liu, B., Song, C., Gao, Q., Zhou, Q., Sun, C., Zhang, H., et al. (2021). Maternal and environmental microbes dominate offspring microbial colonization in the giant freshwater prawn Macrobrachium rosenbergii. Sci. Total Environ. 790, 148062. doi:10.1016/j.scitotenv.2021.148062
Liu, X., Sha, Y., Dingkao, R., Zhang, W., Lv, W., Wei, H., et al. (2020). Interactions between rumen microbes, VFAs, and host genes regulate nutrient absorption and epithelial barrier function during cold season nutritional stress in Tibetan sheep. Front. Microbiol. 11, 593062. doi:10.3389/fmicb.2020.593062
Magoč, T., and Salzberg, S. L. (2011). Flash: Fast length adjustment of short reads to improve genome assemblies. Bioinformatics 27, 2957–2963. doi:10.1093/bioinformatics/btr507
Mazier, W., Corf, K. L., Martinez, C., Tudela, H., Kissi, D., Kropp, C., et al. (2021). A new strain of Christensenella minuta as a potential biotherapy for obesity and associated metabolic diseases. Cells 10, 823. doi:10.3390/cells10040823
McMunn, M. S., Hudson, A. I., Zemenick, A. T., Egerer, M., Bennett, L., Philpott, S. M., et al. (2022). Thermal sensitivity and seasonal change in the gut microbiome of a desert ant, Cephalotes rohweri. Cephalotes Rohweri. FEMS Microbiol. Ecol. 98, fiac062. doi:10.1093/FEMSEC/FIAC062
Moeller, A. H., Gomes-Neto, J. C., Mantz, S., Kittana, H., Segura, M. R. R., Schmaltz, R. J., et al. (2019). Experimental evidence for adaptation to species-specific gut microbiota in house mice. MSphere 4, 003877–e419. doi:10.1128/mSphere.00387-19
Neuparth, T., Costa, F. O., and Costa, M. H. (2002). Effects of temperature and salinity on life history of the marine amphipod Gammarus locusta. Implications for ecotoxicological testing. Ecotoxicology 11, 61–73. doi:10.1023/A:1013797130740
Osadchiy, V., Martin, C. R., and Mayer, E. A. (2019). The gut–brain axis and the microbiome: Mechanisms and clinical implications. Clin. Gastroenterology Hepatology 17, 322–332. doi:10.1016/j.cgh.2018.10.002
Pan, J., Wu, R., Wu, X., Long, X., Wang, X., and Cheng, Y. (2020). Impacts of different feeding modes on the gonadal development, total edible yield, and nutritional composition of male Chinese mitten crab (Eriocheir sinensis). Aquac. Fish. 5, 300–307. doi:10.1016/j.aaf.2020.01.004
Peng, J., Zhao, Y. L., Xu, Z. G., Liu, B. Q., Duan, C. C., and Tang, Y. C. (2019). Effect of temperature stress on the survival of juvenile Chinese mitten crab (Eriocheir sinensis). Iran. J. Fish. Sci. 18, 763–774. http://jifro.ir/article-1-2527-en.html.
Ramos-Romero, S., Santocildes, G., Piñol-Piñol, D., Rosés, C., Pagés, T., Hereu, M., et al. (2020). Implication of gut microbiota in the physiology of rats intermittently exposed to cold and hypobaric hypoxia. PloS one 15, e0240686. doi:10.1371/journal.pone.0240686
Ran, C., Qin, C., Xie, M., Zhang, J., Li, J., Xie, Y., et al. (2018). Aeromonas veronii and aerolysin are important for the pathogenesis of motile aeromonad septicemia in cyprinid fish. Environ. Microbiol. 20, 3442–3456. doi:10.1111/1462-2920.14390
Robertson, R. C., Manges, A. R., Finlay, B. B., and Prendergast, A. J. (2019). The human microbiome and child growth–first 1000 days and beyond. Trends Microbiol. 27, 131–147. doi:10.1016/j.tim.2018.09.008
Rungrassamee, W., Klanchui, A., Maibunkaew, S., Chaiyapechara, S., Jiravanichpaisal, P., and Karoonuthaisiri, N. (2014). Characterization of intestinal bacteria in wild and domesticated adult black tiger shrimp (Penaeus monodon). PloS one 9, e91853. doi:10.1371/journal.pone.0091853
Sachlikidis, N. G., Jones, C. M., and Seymour, J. E. (2010). The effect of temperature on the incubation of eggs of the tropical rock lobster Panulirus ornatus. Aquaculture 305, 79–83. doi:10.1016/j.aquaculture.2010.04.015
Sepulveda, J., and Moeller, A. H. (2020). The effects of temperature on animal gut microbiomes. Front. Microbiol. 11, 384. doi:10.3389/fmicb.2020.00384
Shields, J. D. (2019). Climate change enhances disease processes in crustaceans: Case studies in lobsters, crabs, and shrimps. J. Crustacean Biol. 39, 673–683. doi:10.1093/jcbiol/ruz072
Shin, N. R., Whon, T. W., and Bae, J. W. (2015). Proteobacteria: Microbial signature of dysbiosis in gut microbiota. Trends Biotechnol. 33, 496–503. doi:10.1016/j.tibtech.2015.06.011
Singh, R. K., Chang, H. W., Yan, D. I., Lee, K. M., Ucmak, D., Wong, K., et al. (2017). Influence of diet on the gut microbiome and implications for human health. J. Transl. Med. J. Transl. Med. 15, 73–17. doi:10.1186/s12967-017-1175-y
Sommer, F., and Bäckhed, F. (2013). The gut microbiota-masters of host development and physiology. Nat. Rev. Microbiol. 11, 227–238. doi:10.1038/nrmicro2974
Su, S., Munganga, B. P., Du, F., Yu, J., Li, J., Yu, F., et al. (2020). Relationship between the fatty acid profiles and gut bacterial communities of the Chinese mitten crab (Eriocheir sinensis) from ecologically different habitats. Front. Microbiol. 11, 565267. doi:10.3389/fmicb.2020.565267
Sun, F., and Xu, Z. (2021). Significant differences in intestinal microbial communities in aquatic animals from an aquaculture area. J. Mar. Sci. Eng. 9, 104. doi:10.3390/jmse9020104
Sun, P., Jin, M., Ding, L., Lu, Y., Ma, H., Yuan, Y., et al. (2018). Dietary lipid levels could improve growth and intestinal microbiota of juvenile swimming crab, Portunus trituberculatus. Portunus Trituberculatus. Aquac. 490, 208–216. doi:10.1016/j.aquaculture.2018.02.018
Sun, X., Yuan, Q., Du, B., Jin, X., Huang, X., Li, Q., et al. (2022). Relationship between changes in intestinal microorganisms and effect of high temperature on the growth and development of Bombyx mori larvae. Int. J. Mol. Sci. 23, 10289. doi:10.3390/ijms231810289
Walker, S. J., Neill, W. H., Lawrence, A. L., and Gatlin, D. M. (2011). Effects of temperature and starvation on ecophysiological performance of the Pacific white shrimp (Litopenaeus vannamei). Aquaculture 319, 439–445. doi:10.1016/j.aquaculture.2011.07.015
Wang, K., Liao, M., Zhou, N., Bao, L., Ma, K., Zheng, Z., et al. (2019). Parabacteroides distasonis alleviates obesity and metabolic dysfunctions via production of succinate and secondary bile acids. Cell Rep. 26, 222–235.e5. doi:10.1016/j.celrep.2018.12.028
Wang, W., Wang, A., Liu, Y., Xiu, J., Liu, Z., and Sun, R. (2006). Effects of temperature on growth, adenosine phosphates, ATPase and cellular defense response of juvenile shrimp Macrobrachium nipponense. Aquaculture 256, 624–630. doi:10.1016/j.aquaculture.2006.02.009
Wittmann, A. C., Benrabaa, S. A. M., López-Cerón, D. A., Chang, E. S., and Mykles, D. L. (2018). Effects of temperature on survival, moulting, and expression of neuropeptide and mTOR signalling genes in juvenile Dungeness crab (Metacarcinus magister). J. Exp. Biol. 221, jeb187492. doi:10.1242/jeb.187492
Wyban, J., Walsh, W. A., and Godin, D. M. (1995). Temperature effects on growth, feeding rate and feed conversion of the Pacific white shrimp (Penaeus vannamei). Aquaculture 138, 267–279. doi:10.1016/0044-8486(95)00032-1
Xue, S., Mao, Y., Li, J., and Zhao, F. (2021). Effects of rising temperature on growth and energy budget of juvenile Eogammarus possjeticus (Amphipoda: Anisogammaridae). Acta Oceanol. Sin. 40, 82–89. doi:10.1007/s13131-021-1863-6
Yan, M., Li, W., Chen, X., He, Y., Zhang, X., and Gong, H. (2021). A preliminary study of the association between colonization of microorganism on microplastics and intestinal microbiota in shrimp under natural conditions. J. Hazard. Mater. 408, 124882. doi:10.1016/j.jhazmat.2020.124882
Yin, J., Shen, W., and Li, P. (2004). Study on the influence of water temperature on the intestinal microfloraof Penaeusvannamei. Mar. Sci. 28, 33–36. doi:10.3969/j.issn.1000-3096.2004.05.007
Yuan, Q., Wang, Q., Zhang, T., Li, Z., and Liu, J. (2017). Effects of water temperature on growth, feeding and molting of juvenile Chinese mitten crab Eriocheir sinensis. Aquaculture 468, 169–174. doi:10.1016/j.aquaculture.2016.10.007
Yukgehnaish, K., Kumar, P., Sivachandran, P., Marimuthu, K., Arshad, A., Paray, B. A., et al. (2020). Gut microbiota metagenomics in aquaculture: Factors influencing gut microbiome and its physiological role in fish. Rev. Aquac. 12, raq12416–1927. doi:10.1111/raq.12416
Zeng, S., Huang, Z., Hou, D., Liu, J., Weng, S., and He, J. (2017). Composition, diversity and function of intestinal microbiota in Pacific white shrimp (Litopenaeus vannamei) at different culture stages. PeerJ 5, e3986. doi:10.7717/peerj.3986
Zhang, D., Xu, D., Shoemaker, C., and Yao, Y. G. (2016). Integrative analyses of leprosy susceptibility genes indicate a common autoimmune profile. Aquac. Rep. 3, 18–27. doi:10.1016/j.jdermsci.2016.01.001
Zhang, K., Jiang, P., Wang, J., Chen, X., and Wang, C. (2022). Effects of different temperatures on growth and gut microbiota of Chinese mitten crab(Eriocheir sinensis). J. Shanghai Ocean Univ. 31, 384–393. doi:10.12024/jsou.20210303325
Zhang, M., Sun, Y., Chen, K., Yu, N., Zhou, Z., Chen, L., et al. (2014). Characterization of the intestinal microbiota in Pacific white shrimp, Litopenaeus vannamei, fed diets with different lipid sources. Aquaculture 434, 449–455. doi:10.1016/j.aquaculture.2014.09.008
Zhang, R., Wang, X. W., Zhu, J. Y., Liu, L. L., Liu, Y. C., and Zhu, H. (2019). Dietary sanguinarine affected immune response, digestive enzyme activity and intestinal microbiota of Koi carp (cryprinus carpiod). Aquaculture 502, 72–79. doi:10.1016/j.aquaculture.2018.12.010
Zhang, Y., Liu, Y., Li, J., Xing, T., Jiang, Y., Zhang, L., et al. (2020). Dietary resistant starch modifies the composition and function of caecal microbiota of broilers. J. Sci. Food Agric. 100, 1274–1284. doi:10.1002/jsfa.10139
Zhu, L., Zhu, W., Zhao, T., Chen, H., Zhao, C., Xu, L., et al. (2021). Environmental temperatures affect the gastrointestinal microbes of the Chinese giant salamander. Front. Microbiol. 12, 543767. doi:10.3389/FMICB.2021.543767
Keywords: temperature, E. sinensis, survival, growth performance, intestinal bacterial composition
Citation: Liang M, Feng W, Chen X, Tang Y, Li J and Li W (2023) Effects of different temperatures on growth and intestinal microbial composition of juvenile Eriocheir sinensis. Front. Physiol. 14:1163055. doi: 10.3389/fphys.2023.1163055
Received: 10 February 2023; Accepted: 22 June 2023;
Published: 13 July 2023.
Edited by:
Hongyu Ma, Shantou University, ChinaCopyright © 2023 Liang, Feng, Chen, Tang, Li and Li. This is an open-access article distributed under the terms of the Creative Commons Attribution License (CC BY). The use, distribution or reproduction in other forums is permitted, provided the original author(s) and the copyright owner(s) are credited and that the original publication in this journal is cited, in accordance with accepted academic practice. No use, distribution or reproduction is permitted which does not comply with these terms.
*Correspondence: Yongkai Tang, dGFuZ3lrQGZmcmMuY24=; Wenjing Li, d2VuamluZ2hhb3l1bjIwMDhAMTYzLmNvbQ==