- 1The Key Laboratory of Pufferfish Breeding and Culture in Liaoning Province, Dalian Ocean University, Dalian, China
- 2College of Fisheries an Life, Dalian Ocean University, Dalian, Liaoning, China
- 3Hebei Key Laboratory of Ocean Dynamics Resources and Environments, Hebei Normal University of Science and Technology, Qinhuangdao, China
- 4Department of Nutrition and Health, China Agricultural University, Beijing, China
There will be generated some adverse conditions in the process of acquculture farming with the continuous improvement of the intensive degree of modern aquaculture, such as crowding stress, hypoxia, and malnutrition, which will easily lead to oxidative stress. Se is an effective antioxidant, participating and playing an important role in the antioxidant defense system of fish. This paper reviews the physiological functions of selenoproteins in resisting oxidative stress in aquatic animals, the mechanisms of different forms of Se in anti-oxidative stress in aquatic animals and the harmful effects of lower and higher levels of Se in aquaculture. To summarize the application and research progress of Se in oxidative stress in aquatic animals and provide scientific references for its application in anti-oxidative stress in aquaculture.
1 Introduction
Selenium (Se) is an essential micronutrient that maintains growth, health and development in human and animals (Hatfield et al., 2012). Se exists in nature is available as inorganic form and organic form. In addition, there is also a new type of synthetic nano form. The biological functions of Se include promoting the growth and development of animals, improving the antioxidant capacity of the body, avoiding oxidative stress, enhancing immunity, improving the health of the body, and are widely used in the growth and development of aquaculture animals (Wischhusen et al., 2020; Khan et al., 2017; Jingyuan et al., 2020). However, Se cannot be directly digested and absorbed by organisms. Se appears functions in the form of selenoprotein and exerts multiple and complex effects (Wrobel et al., 2016).
There will be generated some adverse conditions in the process of aquaculture farming with the continuous improvement of the intensive degree of modern aquaculture, such as crowding stress, hypoxia, and malnutrition. Meanwhile, that will promote the production of excessive reactive oxygen species (ROS), change the balance of oxidation and reduction in cells and will lead to oxidative stress (Rider et al., 2009; Abdel Tawwab et al., 2019). Once oxidative stress occurs, it may lead to the dynamic imbalance of free radical stability, which ultimately the fish poor growth and decline the meat quality (Chadio et al., 2015). The damage of oxidative stress in production is increasingly prominent, so it is urgent to solve the problem of oxidative stress in aquaculture animals.
In order to prevent oxidative damage caused by adverse conditions, aquaculture animals must have an effective antioxidant system, such as endogenous free radical scavenging enzymes and exogenous antioxidants (Ighodaro and Akinloye, 2018). Se is just a extra-effective antioxidant and plays an important role in the antioxidant defense mechanism of fish. It protects the cell membrane from oxidative damage through regulate glutathione peroxidase (GPX) activity (Hamilton, 2004). Se can decompose and clear peroxides, thus preventing the accumulation of reactive oxygen species and free radicals such as hydrogen peroxide (H2O2) in the body tissues (Zuo et al., 2019), enhancing resistance to inflammation, maintaining immune function and stabilizing the internal environment (Wande et al., 2020). However, dietary Se deficiency will reduce the expression of selenoproteins, increase the peroxidation reaction of lipids, proteins, DNA and then pose a threat to the health of the body (Rao et al., 2020). Excessive Se will also produce toxic side effects on the body, leading to liver damage, physiological function decline, immune capacity reduction, and even death (Chadio et al., 2015).
At present, the research on Se antioxidant stress has involved inorganic Se (such as sodium selenite), organic Se (such as yeast Se, selenomethionines) and nano Se. In the study of the effects of sodium selenite and lactobacillus on the growth and immune response of rainbow trout, the results showed that the use of sodium selenite and lactobacillus as feed additives could improve the growth and metabolism. Also, the effect of lactobacillus on the natural immune response of rainbow trout was also enhanced under the effect of selenite (Kousha et al., 2020). Another study found that, the mortality rate and malondialdehyde (MDA) level of grass carp fed a high-fat diet supplemented with 0.3 and 0.6 mg/kg nano Se was significantly reduced after hypoxia stress, indicating that nano Se can alleviate lipid peroxidation, thus alleviating the oxidative damage of grass carp under hypoxia stress (Yu et al., 2020). Liu et al. (2017) showed that 0.4 mg/kg yeast Se significantly increased the activity of catalase (CAT) and GPX in the liver of blunt snout bream and significantly decreased the activity of glutathione reductase (GR) and the content of MDA, showing strong antioxidant capacity.
2 The role of selenoproteins in the antioxidant system of aquatic animals
In general, Se sources in animals will first be converted into selenides for play on organisms and then will be converted into proteins containing selenocysteine (Sec), that is, selenoprotein which then will be absorbed and used by organisms (Yoboue et al., 2018). During the process of fish growth, any pressure condition will directly or indirectly cause oxidative stress, leading to the level of intracellular ROS related to oxidative stress increasing, causing destructive effects on lipids, proteins, and DNA (Martínez Álvarez et al., 2005; Zenteno Savín et al., 2006). The lack of balance between the production of ROS and the antioxidant defense system of animals will lead to DNA hydroxylation, protein denaturation, lipid peroxidation, cell apoptosis, ultimately lead to cell damage (Yang et al., 2016). As an effective antioxidant, Se plays an important role in the antioxidant defense system of aquaculture animals. It can maintain balance in the body and antioxidant system by generating and removing ROS (Steinbrenner and Sies, 2009).
It has been found that the thioredoxin reductase system and glutathione peroxidase system play an important role in cellular oxidative stress defense (Lee et al., 2012; Benfeitas et al., 2014). The main function of GPX in mammals is to eliminate excessive free radicals in cells, including superoxide anions (O2-), H2O2, and hydroxyl radicals (OH−). Then through reduce peroxides to corresponding alcohols, prevent lipid peroxidation, and regulate the balance of redox in cells (Yoboue et al., 2018; Rocca et al., 2018). The Thioredoxin system is one of the main redox buffer systems in the body, which plays a key role in the transduction of redox signals (Canter et al., 2021; Shchedrina et al., 2010). In addition, the endoplasmic reticulum (ER) is one of the important places where redox reactions occur in cells, which can produce H2O2 and ROS (Varlamova, 2018). SELENOF, Recombinant Deiodinase, Iodothyronine, Type II (DIO2), SELENOS, SELENON, SELENOK, SELENOM, and SELENOT are considered to be seven endoplasmic reticulum resident proteins with oxidoreductase activity and can participate in a series of processes, such as the regulation of endoplasmic reticulum redox state, endoplasmic reticulum stress and intracellular Ca2+ homeostasis (Malandrakis et al., 2014; Pitts and Hoffmann, 2017; Drew et al., 2008; Addinsall et al., 2018; Rathore et al., 2021; Xu et al., 2022) Specific selenoprotein involved in redox reaction are shown in Table 1.
3 Application of different forms of Se on antioxidant system of aquatic animals
In many studies, Se has been found that supplement oxidative stress caused by hypoxia, crowding, heavy metal pollution, intake oxidized oil and other pressure conditions (Schieber and Chandel, 2014; Chen et al., 2013). Oxidative stress is considered to be a major problem in aquaculture because it is reported that this phenomenon will occur naturally in most aquatic environments, especially in high-density intensive fish farming which will induce metabolic changes and produce a large number of reactive oxygen species (Zenteno Savín et al., 2006). In order to avoid the imbalance of ROS production, aquatic animals can reduce the formation of ROS or reduce the high reactivity of such compounds through antioxidant components which are produced by cells (endogenous sources) or obtained through diet (exogenous sources). A Lack of balance between ROS formation and the ability of organisms to process reactive oxygen will lead to oxidative stress (Wang et al., 2013; Ji et al., 2019). At present, many aquatic animals have been studied on the antioxidant system, and there is evidence that they can prevent or repair oxidative damage (Biller and Takahashi, 2018) (Table 2).
4 Harmful effects of Se deficiency and excess on antioxidant function in aquatic animals
After these environmental pressures, Se may have protective effects on the pathology related to oxidative stress of aquatic animals. It is particularly important for intensive aquaculture animals, because environmental pressures may cause significant losses to fish growers. The demand for Se will increase after suffering environmental pressures, and supplementation is a necessary condition to meet the needs of fish growth (Ashouri et al., 2015). Se can act as a dietary supplement to effectively improve the oxidation status of aquaculture animals. Although, dietary Se application in aquatic animal feed is considered to have a narrow intake range for high concentration may be toxic (Nasir et al., 2015) and lack may have adverse effects on fish health, leading to tissue damage and physiological function weakening (Khan and Bano, 2016). Therefore, their safety in aquaculture animals is controversial.
4.1 Harmful effects of selenium excess
Se is an essential micronutrient required for fish, which plays an important role in the antioxidant system and also affects the lipid metabolism, sugar metabolism and amino acid metabolism of aquatic animals (Yoboue et al., 2018; Fontagné Dicharry et al., 2015; Abdel Tawwab et al., 2007). Although Se supplementation in aquatic feed can alleviate the oxidation state of cultured animals, but excessive Se content will also have some toxic effects (Jobling, 2012). When the Se content in the aquatic feed is slightly higher than the demand, it will have other adverse effects including oxidative stress, cytotoxicity and genotoxicity (Gobi et al., 2018). If the fish is exposed to excessive Se, the degree of oxidative stress in the body can be significantly increased, then cause oxidative stress. Glutathione (GSH) is largely used to maintain the homeostasis of ROS in the body, can cause an imbalance in the antioxidant system (Atencio et al., 2009). In Atlantic salmon, higher Se levels (at least 15 mg/kg in diet) can lead to oxidative stress and change the lipid metabolism of organic and inorganic Se (Berntssen et al., 2017). In addition, in Acipenser sturgeon (Acipenser transmontanus), excessive Se in the diet leads to vacuolar degeneration of cells and necrosis of the liver (Tashjian et al., 2006). In a word, the mechanism of the negative effect of high Se intake on the antioxidant system of aquatic animals is still unclear.
4.2 Harmful effects of Se deficiency
The necessity and demand for dietary Se have been estimated in various fish (Khan et al., 2017). Although Se poisoning may cause multiple damages to fish, the effect of dietary Se deficiency in fish cannot be ignored. It has been reported that Se deficiency is closely related to the occurrence of many diseases in aquatic animals and the impairment of physiological functions under various conditions (Zheng et al., 2018; Wang et al., 2018). Se deficiency can inhibit organs growth and reduce immune functions, thus leading to many inflammatory diseases (Zheng et al., 2018; Hofstee et al., 2017). Se has been proved to be a protective trace element in the liver of many animals. Se deficiency can lead to immune deficiency, inhibit fish growth and induce oxidative stress in the liver (Zheng et al., 2018; Byron and Santolo, 2018). Oxidative stress can stimulate liver inflammation, thereby aggravating liver injury (Mukhopadhyay et al., 2018). In a research on the mechanism of carp liver inflammation, dietary Se deficiency can lead to Se deficiency in blood and liver, leading to overexpression of heat shock protein HSP60 in the liver, aggravating and forming inflammatory damage to the liver (Gao et al., 2019). Se deficiency also impairs the structural integrity of the head kidney, spleen and skin of juvenile grass carp, leads to oxidative damage and aggravating cell apoptosis due to downregulate the activities of antioxidant enzymes (SOD, CAT, GPX, GSTR, and GR) and the mRNA levels related to the signal transduction part (Zheng et al., 2018).
5 Effects of Se-rich fish on humans
Food is the main source of Se intake by the human. As like fish, Se is mainly involved in regulating human various physiological activities in the form of selenoproteins. Se deficiency or Se excess can have adverse effects on the human (Rayman, 2008). Fish is a kind of food with the highest natural Se content and tender flesh, rich in mineral elements, proteins and vitamins, which is the preferred ingredient for people to supplement trace elements through diet. Fish reared in a Se-rich water environment and fed with Se-rich feed can be processed and prepared into bioactive peptide foods such as fish collagen peptides. Eating Se-rich bioactive peptide foods can improve the absorption rate of Se in the body and Se supplement is more efficient (Xia et al., 2022).
Se can reduce the incidence rate of heart disease in humans, but ROS formed during oxidative stress can initiate lipid peroxidation, damage normal cell function, and lead to myocardial ischemia reperfusion (I/R) injury. Se enzyme and the whole thioredoxin system are directly reduce the content of lipid peroxide, protect vascular endothelial cells from oxidative damage, and inhibit Low Density Lipoprotein (LDL) oxidation (Furman et al., 2004; Venardos and Kaye, 2007). SELENOM may be able to alleviate disorders of lipid metabolism and high-fat diet (HFD)-mediated mitochondrial damage in NAFLD. SELENOM regulates Parkin-mediated mitophagy via the AMPKα1–MFN2 signaling pathway, blockade of the AMPKα1–MFN2 pathway and inhibit mitophagy. Moreover, SELENOM deletion inhibits the expression of FAO-related genes (Ppara, Cpt1α, Cdh15, Acox1 and Acadm), and increases the expression of lipogenic genes (Gpam, Plin1, Scd1, Lipe, Fasn, Acly, and Pparg) (Cai et al., 2022). It has been shown that during wound healing some selenoproteins (GPX, SELENOS and SELENOP) bind together with produce effects in the inflammatory phase, such as antioxidant, inhibition of inflammatory cytokines, scavenging of peroxynitrite and enhancing immune function by increasing the survival of phagocytes during phagocytosis (Lei et al., 2009). Se is play a key role in reducing and preventing cancer caused by several carcinogens and inducing apoptosis of cancer cells (Tinggi, 2008). Se level is negatively correlated with human cancer risk. Genomic studies and animal models have indicated that Se intake influences the expression of selenoprotein genes and pathways key to colorectal carcinogenesis such as the antioxidant response, immune and inflammatory pathways (including NF-kB and Nrf2 signaling), the Wnt signaling pathway, protein synthesis pathway (Ye et al., 2021). Se also reduces the incidence of autoimmune thyroiditis and other related conditions, and the reduction of thyroid autoimmune effects is related to the role of GPX and thioredoxin reductase (TrxR) as antioxidant defense systems that scavenge ROS and excess H2O2 produced by thyroid cells during thyroid hormone synthesis, which can lead to thyroid cell necrosis and increased macrophage invasion in severe nutritional Se deficiency (Duntas, 2006).
6 Antioxidant mechanism of different Se forms in fish
In the intensive aquaculture system, cultured fish mainly obtain the selenium from the feed. There are three main forms of selenium additives: one is inorganic Se, which enters animals through passive diffusion in the form of Se4+, and is quickly reduced to selenides (H2Se) in the intestine; the second is organic Se, which is actively absorbed by fish in the form of amino acids, combines with plasma protein to form SeMet; the third is Nano-Se can activate Nrf2 signal transduction pathway to express genes related to antioxidant enzymes. No matter inorganic Se, organic Se or Nano-Se, it will be converted into selenoproteins to play a role after being absorbed by fish. Selenoproteins related to fish antioxidant mechanism include GPXs, TXNRDs, SELENOP, SELENOS, and other selenoproteins, which regulate lipid metabolism, inflammatory damage, ROS and H2O2 formation oxidative stress through antioxidant enzymes (SOD, GPX, and CAT) in fish antioxidant system. In addition, the regulation of selenium deficiency in the human antioxidant system will cause diseases of the heart system, immune system and thyroid function damage (Yu et al., 2020; Wischhusen et al., 2019) (Figure 1).
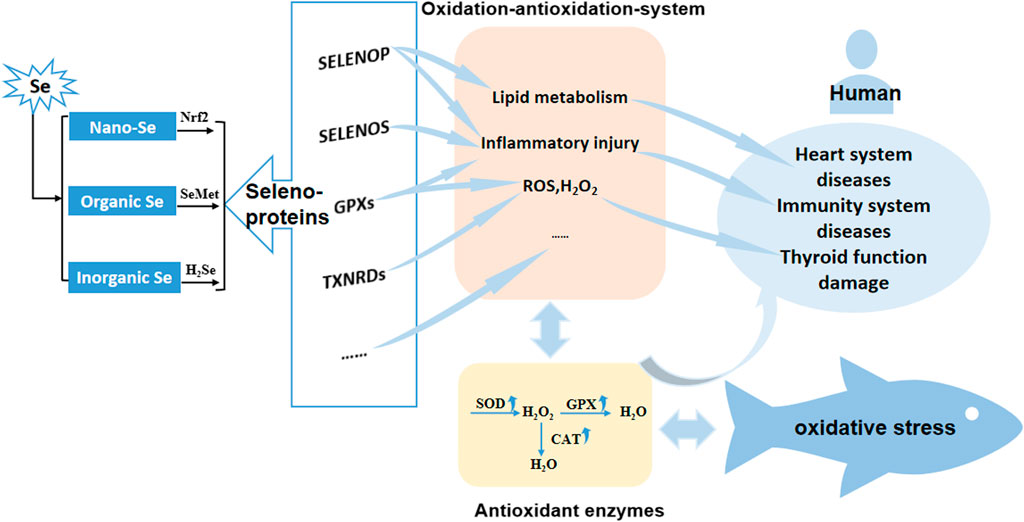
FIGURE 1. Antioxidant mechanism of different Se forms in fish. Nano-Se, Nano-Selenium; Organic Se, Organic Selenium; Inorganic Se, Inorganic Selenium; GPXs, glutathione peroxidase family; TXNRDs, thioredoxin reductase family.
7 Conclusion
In summary, Se is an essential mineral element for antioxidant stress of aquatic animals and plays an important role in the antioxidant system of aquaculture. We can further study the most effective form of Se in the antioxidant system of aquatic animals, selectively prevent the oxidative stress of aquaculture animals, clarify the mechanism of selenoproteins in the antioxidant system of aquatic animals, and provide direction for the study of Se toxicity. Due to the various functions of selenoproteins, the mechanism of specific selenoprotein function and expression can be considered to prevent the oxidation state of aquatic animals. In the future, we can also compare the effects of Se on other organisms and study the mechanism of Se on the antioxidant system of aquatic animals. This will provide important information for the extensive application of antioxidant systems in aquaculture. This will provide important information for the extensive application of antioxidant systems in aquaculture.
Author contributions
Conceptualization, Z-ML and L-SW; methodology, J-QH; software, Z-ML validation, Z-ML, X-MJ, and L-SW; formal analysis, Z-ML; investigation, Z-ML; resources, J-QH; data curation, Z-ML; writing—original draft preparation, Z-ML; writing—review and editing, Z-ML, JQH, and L-SW; visualization, X-MJ; supervision, L-SW; project administration, L-SW; funding acquisition, L-SW. All authors have read and agreed to the published version of the manuscript.
Funding
This research was funded by Innovation Team Project of Dalian Ocean University, China (B202102) and the Central Guidance on Local Science and Technology Development Fund of Hebei Province (226Z7103G), China.
Conflict of interest
The authors declare that the research was conducted in the absence of any commercial or financial relationships that could be construed as a potential conflict of interest.
Publisher’s note
All claims expressed in this article are solely those of the authors and do not necessarily represent those of their affiliated organizations, or those of the publisher, the editors and the reviewers. Any product that may be evaluated in this article, or claim that may be made by its manufacturer, is not guaranteed or endorsed by the publisher.
References
Abdel Tawwab, M., Monier, M. N., Hoseinifar, S. H., and Faggio, C. (2019). Fish response to hypoxia stress: Growth, physiological, and immunological biomarkers. Fish Physiology Biochem. 45, 997–1013. doi:10.1007/s10695-019-00614-9
Abdel Tawwab, M., Mousa, M. A. A., and Abbass, F. E. (2007). Growth performance and physiological response of African catfish, Clarias gariepinus (B.) fed organic selenium prior to the exposure to environmental copper toxicity. Aquaculture 272, 335–345. doi:10.1016/j.aquaculture.2007.09.004
Addinsall, A. B., Wright, C. R., Andrikopoulos, S., van der Poel, C., and Stupka, N. (2018). Emerging roles of endoplasmic reticulum-resident selenoproteins in the regulation of cellular stress responses and the implications for metabolic disease. Biochem. J. 475, 1037–1057. doi:10.1042/BCJ20170920
Ashouri, S., Keyvanshokooh, S., Salati, A. P., Johari, S. A., and Pasha Zanoosi, H. (2015). Effects of different levels of dietary selenium nanoparticles on growth performance, muscle composition, blood biochemical profiles and antioxidant status of common carp (Cyprinus carpio). Aquaculture 446, 25–29. doi:10.1016/j.aquaculture.2015.04.021
Atencio, L., Moreno, I., Jos, Á., Prieto, A. I., Moyano, R., Blanco, A., et al. (2009). Effects of dietary selenium on the oxidative stress and pathological changes in tilapia (Oreochromis niloticus) exposed to a microcystin-producing cyanobacterial water bloom. Toxicon 53, 269–282. doi:10.1016/j.toxicon.2008.11.011
Benfeitas, R., Selvaggio, G., Antunes, F., Coelho, P., and Salvador, A. (2014). P9 - is the Peroxiredoxin 2/Thioredoxin/Thioredoxin Reductase system in human erythrocytes designed for redox signaling? Free Radic. Biol. Med. 75, S24. doi:10.1016/j.freeradbiomed.2014.10.741
Berntssen, M. H. G., Sundal, T. K., Olsvik, P. A., Amlund, H., Rasinger, J. D., Sele, V., et al. (2017). Sensitivity and toxic mode of action of dietary organic and inorganic selenium in Atlantic salmon (Salmo salar). Aquat. Toxicol. 192, 116–126. doi:10.1016/j.aquatox.2017.09.014
Biller, J., and Takahashi, L. (2018). Oxidative stress and fish immune system: Phagocytosis and leukocyte respiratory burst activity. An. Acad. Bras. Ciências 90, 3403–3414. doi:10.1590/0001-3765201820170730
Bityutskyy, V. S., Tsekhmistrenko, S. I., Tsekhmistrenko, O. S., Oleshko, O. A., and Heiko, L. М. (2020). Influence of selenium on redox processes, selenoprotein metabolism and antioxidant status of aquaculture facilities. Taurian Sci. Her. 114, 231–240. doi:10.32851/2226-0099.2020.114.28
Byron, E. R., and Santolo, G. M. (2018). Fish whole-body selenium: Interspecies translation experiment. Environ. Monit. Assess. 191, 17. doi:10.1007/s10661-018-7148-7
Cai, J., Huang, J., Yang, J., Chen, X., Zhang, H., Zhu, Y., et al. (2022). The protective effect of selenoprotein M on non-alcoholic fatty liver disease: The role of the ampkα1–MFN2 pathway and Parkin mitophagy. Cell. Mol. Life Sci. 79, 354. doi:10.1007/s00018-022-04385-0
Canter, J. A., Ernst, S. E., Peters, K. M., Carlson, B. A., Thielman, N. R. J., Grysczyk, L., et al. (2021). Selenium and the 15kDa selenoprotein impact colorectal tumorigenesis by modulating intestinal barrier integrity. Int. J. Mol. Sci. 22, 10651. doi:10.3390/ijms221910651
Castellano, S., Lobanov, A. V., Chapple, C., Novoselov, S. V., Albrecht, M., Hua, D., et al. (2005). Diversity and functional plasticity of eukaryotic selenoproteins: Identification and characterization of the SelJ family. Proc. Natl. Acad. Sci. 102, 16188–16193. doi:10.1073/pnas.0505146102
Chadio, S. E., Pappas, A. C., Papanastasatos, A., Pantelia, D., Dardamani, A., Fegeros, K., et al. (2015). Effects of high selenium and fat supplementation on growth performance and thyroid hormones concentration of broilers. J. Trace Elem. Med. Biol. 29, 202–207. doi:10.1016/j.jtemb.2014.09.010
Chen, Y. J., Liu, Y. J., Tian, L. X., Niu, J., Liang, G. Y., Yang, H. J., et al. (2013). Effect of dietary vitamin E and selenium supplementation on growth, body composition, and antioxidant defense mechanism in juvenile largemouth bass (Micropterus salmoides) fed oxidized fish oil. Fish Physiology Biochem. 39, 593–604. doi:10.1007/s10695-012-9722-1
Chernorudskiy, A., Varone, E., Colombo, S., Fumagalli, S., Cagnotto, A., Cattaneo, A., et al. (2020). Selenoprotein N is an endoplasmic reticulum calcium sensor that links luminal calcium levels to a redox activity. Proc. Natl. Acad. Sci. 117, 21288–21298. doi:10.1073/pnas.2003847117
Drew, R., Rodnick, K., Settles, M., Wacyk, J., Churchill, E., Powell, M., et al. (2008). Effect of starvation on transcriptomes of brain and liver in adult female zebrafish (Danio rerio). Physiol. Genomics 35, 283–295. doi:10.1152/physiolgenomics.90213.2008
Duntas, L. H. (2006). The role of selenium in thyroid autoimmunity and cancer. Thyroid 16, 455–460. doi:10.1089/thy.2006.16.455
Fontagné Dicharry, S., Godin, S., Liu, H., Antony Jesu Prabhu, P., Bouyssière, B., Bueno, M., et al. (2015). Influence of the forms and levels of dietary selenium on antioxidant status and oxidative stress-related parameters in rainbow trout (Oncorhynchus mykiss) fry. Br. J. Nutr. 113, 1876–1887. doi:10.1017/S0007114515001300
Fontagné Dicharry, S., Véron, V., Larroquet, L., Godin, S., Wischhusen, P., Aguirre, P., et al. (2020). Effect of selenium sources in plant-based diets on antioxidant status and oxidative stress-related parameters in rainbow trout juveniles under chronic stress exposure. Aquaculture 529, 735684. doi:10.1016/j.aquaculture.2020.735684
Furman, C., Rundlöf, A. K., Larigauderie, G., Jaye, M., Bricca, G., Copin, C., et al. (2004). Thioredoxin reductase 1 is upregulated in atherosclerotic plaques: Specific induction of the promoter in human macrophages by oxidized low-density lipoproteins. Free Radic. Biol. Med. 37, 71–85. doi:10.1016/j.freeradbiomed.2004.04.016
Gao, X. J., Tang, B., Liang, H. H., Yi, L., and Wei, Z. G. (2019). Selenium deficiency induced an inflammatory response by the HSP60 - TLR2-MAPKs signalling pathway in the liver of carp. Fish Shellfish Immunol. 87, 688–694. doi:10.1016/j.fsi.2019.02.017
Giuliani Durigon, E., Kunz, D., Peixoto, N., Uczay, J., and Lazzari, R. (2018). Diet selenium improves the antioxidant defense system of juveniles Nile tilapia (Oreochromis niloticus L.). Braz. J. Biol. 79, 527–532. doi:10.1590/1519-6984.187760
Gobi, N., Vaseeharan, B., Rekha, R., Vijayakumar, S., and Faggio, C. (2018). Bioaccumulation, cytotoxicity and oxidative stress of the acute exposure selenium in Oreochromis mossambicus. Ecotoxicol. Environ. Saf. 162, 147–159. doi:10.1016/j.ecoenv.2018.06.070
Guderley, H., Lapointe, D., Bédard, M., and Dutil, J. D. (2003). Metabolic priorities during starvation: Enzyme sparing in liver and white muscle of atlantic cod, Gadus morhua L. Comp. Biochem. Physiology Part A Mol. Integr. Physiology 135, 347–356. doi:10.1016/S1095-6433(03)00089-8
Hamilton, S. J. (2004). Review of selenium toxicity in the aquatic food chain. Sci. Total Environ. 326, 1–31. doi:10.1016/j.scitotenv.2004.01.019
Hatfield, D., Berry, M., and Gladyshev, V. (2012). Selenium: Its molecular biology and role in human health. Germany: Springer Science & Business Media. doi:10.1007/978-1-4614-1025-6
He, P., Zhang, X., Wu, W., Liu, G., Liu, S., Wang, C., et al. (2022). Molecular characterization and tissue distribution of nine selenoprotein genes in grass carp Ctenopharyngodon idella and their mRNA expressions in response to high-fat diet and high-fat diet supplemented with selenium. Comp. Biochem. Physiology Part B Biochem. Mol. Biol. 259, 110706. doi:10.1016/j.cbpb.2021.110706
Hofstee, P., Bartho, L., McKeating, D., Holland, O., Vanderlelie, J., Cuffe, J., et al. (2017). Maternal selenium deficiency in mice alters placental function, reduces foetal glucose concentrations and impairs foetal growth. Placenta 57, 329. doi:10.1016/j.placenta.2017.07.328
Ighodaro, O. M., and Akinloye, O. A. (2018). First line defence antioxidants-superoxide dismutase (SOD), catalase (CAT) and glutathione peroxidase (GPX): Their fundamental role in the entire antioxidant defence grid. Alexandria J. Med. 54, 287–293. doi:10.1016/j.ajme.2017.09.001
Ji, Y., Qiu, J., Xie, T., McCarron, P., and Aifeng, L. (2019). Accumulation and transformation of azaspiracids in scallops (Chlamys farreri) and mussels (Mytilus galloprovincialis) fed with Azadinium poporum, and response of antioxidant enzymes. Toxicon 158, S42–S43. doi:10.1016/j.toxicon.2018.10.149
Jingyuan, H., Yan, L., Wenjing, P., Wenqiang, J., Bo, L., Linghong, M., et al. (2020). Dietary selenium enhances the growth and anti-oxidant capacity of juvenile blunt snout bream (Megalobrama amblycephala). Fish Shellfish Immunol. 101, 115–125. doi:10.1016/j.fsi.2020.03.041
Jobling, M. (2012). National research council (NRC): Nutrient requirements of fish and shrimp. Aquac. Int. 20, 601–602. doi:10.1007/s10499-011-9480-6
Khan, K. U., Zuberi, A., Fernandes, J. B. K., Ullah, I., and Sarwar, H. (2017). An overview of the ongoing insights in selenium research and its role in fish nutrition and fish health. Fish Physiology Biochem. 43, 1689–1705. doi:10.1007/s10695-017-0402-z
Khan, N., and Bano, A. (2016). Modulation of phytoremediation and plant growth by the treatment with PGPR, Ag nanoparticle and untreated municipal wastewater. Int. J. Phytoremediation 18, 1258–1269. doi:10.1080/15226514.2016.1203287
Kousha, M., Yeganeh, S., and Amirkolaie, A. K. (2020). Synergistic effect of sodium selenite and pediococcus acidilactici on growth, intestinal bacterial counts, selenium bioavailability, hepatic enzymes and non-specific immune response in rainbow trout (Oncorhynchus mykiss). Aquac. Nutr. 26, 74–87. doi:10.1111/anu.12968
Lee, S., Kim, S. M., and Lee, R. T. (2012). Thioredoxin and thioredoxin target proteins: From molecular mechanisms to functional significance. Antioxidants Redox Signal. 18, 1165–1207. doi:10.1089/ars.2011.4322
Lei, C., Niu, X., Wei, J., Zhu, J., and Zhu, Y. (2009). Interaction of glutathione peroxidase-1 and selenium in endemic dilated cardiomyopathy. Clin. Chim. Acta 399, 102–108. doi:10.1016/j.cca.2008.09.025
Liu, G. X., Jiang, G. Z., Lu, K. L., Li, X. F., Zhou, M., Zhang, D. D., et al. (2017). Effects of dietary selenium on the growth, selenium status, antioxidant activities, muscle composition and meat quality of blunt snout bream, Megalobrama amblycephala. Aquac. Nutr. 23, 777–787. doi:10.1111/anu.12444
Malandrakis, E., Exadactylos, A., Olga, D., Golomazou, E., Klaoudatos, S., and Panagiota, P. (2014). Molecular cloning of four glutathione peroxidase (GPX) homologs and expression analysis during stress exposure of the marine teleost Sparus aurata. Comp. Biochem. Physiology Part B Biochem. Mol. Biol. 168, 53–61. doi:10.1016/j.cbpb.2013.11.005
Martínez Álvarez, R. M., Morales, A. E., and Sanz, A. (2005). Antioxidant defenses in fish: Biotic and abiotic factors. Rev. Fish Biol. Fish. 15, 75–88. doi:10.1007/s11160-005-7846-4
Mechlaoui, M., Dominguez, D., Robaina, L., Geraert, P. A., Kaushik, S., Saleh, R., et al. (2019). Effects of different dietary selenium sources on growth performance, liver and muscle composition, antioxidant status, stress response and expression of related genes in gilthead seabream (Sparus aurata). Aquaculture 507, 251–259. doi:10.1016/j.aquaculture.2019.04.037
Mukhopadhyay, P., Eid, N., Abdelmegeed, M. A., and Sen, A. (2018). Interplay of oxidative stress, inflammation, and autophagy: Their role in tissue injury of the heart, liver, and kidney. Oxidative Med. Cell. Longev. 2018, 2090813. doi:10.1155/2018/2090813
Nasir, A., Kausar, A., and Younus, A. (2015). A review on preparation, properties and applications of polymeric nanoparticle-based materials. Polymer-Plastics Technol. Eng. 54, 325–341. doi:10.1080/03602559.2014.958780
Penglase, S., Hamre, K., and Ellingsen, S. (2014). Selenium prevents downregulation of antioxidant selenoprotein genes by methylmercury. Free Radic. Biol. Med. 75, 95–104. doi:10.1016/j.freeradbiomed.2014.07.019
Pitts, M., and Hoffmann, P. (2017). Endoplasmic reticulum-resident selenoproteins as regulators of calcium signaling and homeostasis. Cell. Calcium 70, 76–86. doi:10.1016/j.ceca.2017.05.001
Qiang, J., Duan, X. J., Zhu, C. K., He, J., Bao, J. W., Tao, Y. F., et al. (2020). Selenium-cultured potamogeton maackianus in the diet can alleviate oxidative stress and immune suppression in Chinese Mitten Crab (Eriocheir sinensis) under copper exposure. Front. Physiology 11, 713. doi:10.3389/fphys.2020.00713
Rao, Y., Chen, J., Guo, Y., Ji, T., and Xie, P. (2020). Rivaroxaban ameliorates angiotensin II-induced cardiac remodeling by attenuating TXNIP/Trx2 interaction in KKAy mice. Thrombosis Res. 193, 45–52. doi:10.1016/j.thromres.2020.05.030
Rathore, S. S., Murthy, H. S., Girisha, S. K., Nithin, M. S., Nasren, S., Mamun, M. A. A., et al. (2021). Supplementation of nano-selenium in fish diet: Impact on selenium assimilation and immune-regulated selenoproteome expression in monosex Nile tilapia (Oreochromis niloticus). Comp. Biochem. Physiology Part C Toxicol. Pharmacol. 240, 108907. doi:10.1016/j.cbpc.2020.108907
Rayman, M. P. (2008). Food-chain selenium and human health: Emphasis on intake. Br. J. Nutr. 100, 254–268. doi:10.1017/S0007114508939830
Rider, S. A., Davies, S. J., Jha, A. N., Fisher, A. A., Knight, J., and Sweetman, J. W. (2009). Supra-nutritional dietary intake of selenite and selenium yeast in normal and stressed rainbow trout (Oncorhynchus mykiss): Implications on selenium status and health responses. Aquaculture 295, 282–291. doi:10.1016/j.aquaculture.2009.07.003
Rocca, C., Boukhzar, L., Granieri, M. C., Alsharif, I., Mazza, R., Lefranc, B., et al. (2018). A selenoprotein T-derived peptide protects the heart against ischaemia/reperfusion injury through inhibition of apoptosis and oxidative stress. Acta Physiol. 223, e13067. doi:10.1111/apha.13067
Saffari, S., Keyvanshokooh, S., Zakeri, M., Johari, S. A., and Pasha Zanoosi, H. (2017). Effects of different dietary selenium sources (sodium selenite, selenomethionine and nanoselenium) on growth performance, muscle composition, blood enzymes and antioxidant status of common carp (Cyprinus carpio). Aquac. Nutr. 23, 611–617. doi:10.1111/anu.12428
Saito, Y. (2021). Selenium transport mechanism via selenoprotein P—Its physiological role and related diseases. Front. Nutr. 8, 685517. doi:10.3389/fnut.2021.685517
Schieber, M., and Chandel, N. S. (2014). ROS function in redox signaling and oxidative stress. Curr. Biol. 24, R453–R462. doi:10.1016/j.cub.2014.03.034
Shchedrina, V. A., Zhang, Y., Labunskyy, V. M., Hatfield, D. L., and Gladyshev, V. N. (2010). Structure-function relations, physiological roles, and evolution of mammalian ER-resident selenoproteins. Antioxidants Redox Signal. 12, 839–849. doi:10.1089/ars.2009.2865
Solovyev, N. D. (2015). Importance of selenium and selenoprotein for brain function: From antioxidant protection to neuronal signalling. J. Inorg. Biochem. 153, 1–12. doi:10.1016/j.jinorgbio.2015.09.003
Steinbrenner, H., and Sies, H. (2009). Protection against reactive oxygen species by selenoproteins. Biochimica Biophysica Acta (BBA) - General Subj. 1790, 1478–1485. doi:10.1016/j.bbagen.2009.02.014
Tashjian, D. H., Teh, S. J., Sogomonyan, A., and Hung, S. S. O. (2006). Bioaccumulation and chronic toxicity of dietary l-selenomethionine in juvenile white sturgeon (Acipenser transmontanus). Aquat. Toxicol. 79, 401–409. doi:10.1016/j.aquatox.2006.07.008
Thompson, J. L., See, V. H. L., Thomas, P. M., and Schuller, K. A. (2010). Cloning and characterization of two glutathione peroxidase cDNAs from southern bluefin tuna (Thunnus maccoyii). Comp. Biochem. Physiology Part B Biochem. Mol. Biol. 156, 287–297. doi:10.1016/j.cbpb.2010.04.007
Tinggi, U. (2008). Selenium: Its role as antioxidant in human health. Environ. Health Prev. Med. 13, 102–108. doi:10.1007/s12199-007-0019-4
Varlamova, E. G. (2018). Participation of selenoproteins localized in the ER in the processes occurring in this organelle and in the regulation of carcinogenesis-associated processes. J. Trace Elem. Med. Biol. 48, 172–180. doi:10.1016/j.jtemb.2018.04.005
Venardos, K. M., Kaye, D. M., and Headrick, J. (2007). Myocardial ischemia-reperfusion injury, antioxidant enzyme systems, and selenium: A review. Curr. Med. Chem. 14, 1539–1549. doi:10.2174/092986707780831078
Wande, Y., jie, L., aikai, Z., yaguo, Z., linlin, Z., yue, G., et al. (2020). Berberine alleviates pulmonary hypertension through Trx1 and β-catenin signaling pathways in pulmonary artery smooth muscle cells. Exp. Cell. Res. 390, 111910. doi:10.1016/j.yexcr.2020.111910
Wang, C., Yue, X., Lu, X., and Liu, B. (2013). The role of catalase in the immune response to oxidative stress and pathogen challenge in the clam Meretrix meretrix. Fish Shellfish Immunol. 34, 91–99. doi:10.1016/j.fsi.2012.10.013
Wang, J., Liu, Z., He, X., Lian, S., Liang, J., Yu, D., et al. (2018b). Selenium deficiency induces duodenal villi cell apoptosis via an oxidative stress-induced mitochondrial apoptosis pathway and an inflammatory signaling-induced death receptor pathway. Metallomics 10, 1390–1400. doi:10.1039/C8MT00142A
Wang, L., Harris, S. M., Espinoza, H. M., McClain, V., and Gallagher, E. P. (2012). Characterization of phospholipid hydroperoxide glutathione metabolizing peroxidase (gpx4) isoforms in Coho salmon olfactory and liver tissues and their modulation by cadmium. Aquat. Toxicol. 114-115, 134–141. doi:10.1016/j.aquatox.2012.02.025
Wang, L., Xiao, J. X., Hua, Y., Xiang, X. W., Zhou, Y. F., Ye, L., et al. (2019). Effects of dietary selenium polysaccharide on growth performance, oxidative stress and tissue selenium accumulation of juvenile black sea bream, Acanthopagrus schlegelii. Acanthopagrus Schlegelii. Aquac. 503, 389–395. doi:10.1016/j.aquaculture.2019.01.033
Wang, L., Zhang, X., Wu, L., Liu, Q., Zhang, D., and Yin, J. (2018a). Expression of selenoprotein genes in muscle is crucial for the growth of rainbow trout (Oncorhynchus mykiss) fed diets supplemented with selenium yeast. Aquaculture 492, 82–90. doi:10.1016/j.aquaculture.2018.03.054
Wischhusen, P., Larroquet, L., Durand, T., Oger, C., Galano, J. M., Amandine, R., et al. (2020). Oxidative stress and antioxidant response in rainbow trout fry exposed to acute hypoxia is affected by selenium nutrition of parents and during first exogenous feeding. Free Radic. Biol. Med. 155, 99–113. doi:10.1016/j.freeradbiomed.2020.05.006
Wischhusen, P., Parailloux, M., Geraert, P. A., Briens, M., Bueno, M., Mounicou, S., et al. (2019). Effect of dietary selenium in rainbow trout (Oncorhynchus mykiss) broodstock on antioxidant status, its parental transfer and oxidative status in the progeny. Aquaculture 507, 126–138. doi:10.1016/j.aquaculture.2019.04.006
Wrobel, J. K., Power, R., and Toborek, M. (2016). Biological activity of selenium: Revisited. IUBMB Life 68, 97–105. doi:10.1002/iub.1466
Xia, Z., Miao, J., Chen, B., Guo, J., Ou, Y., Liang, X., et al. (2022). Purification, identification, and antioxidative mechanism of three novel selenium-enriched oyster antioxidant peptides. Food Res. Int. 157, 111359. doi:10.1016/j.foodres.2022.111359
Xu, X. J., Zhang, D. G., Zhao, T., Xu, Y. H., and Luo, Z. (2022). Dietary selenium sources differentially regulate selenium concentration, mRNA and protein expression of representative selenoproteins in various tissues of yellow catfish Pelteobagrus fulvidraco. Br. J. Nutr. 127, 490–502. doi:10.1017/S000711452100194X
Yang, Z., Liu, C., Liu, C., Teng, X., and Li, S. (2016). Selenium deficiency mainly influences antioxidant selenoproteins expression in broiler immune organs. Biol. Trace Elem. Res. 172, 209–221. doi:10.1007/s12011-015-0578-y
Ye, R., Huang, J., Wang, Z., Chen, Y., and Dong, Y. (2021). Trace element selenium effectively alleviates intestinal diseases. Int. J. Mol. Sci. 22, 11708. doi:10.3390/ijms222111708
Yoboue, E. D., Sitia, R., and Simmen, T. (2018). Redox crosstalk at endoplasmic reticulum (ER) membrane contact sites (MCS) uses toxic waste to deliver messages. Cell. Death Dis. 9, 331. doi:10.1038/s41419-017-0033-4
Yu, H., Zhang, C., Zhang, X., Wang, C., Li, P., Liu, G., et al. (2020). Dietary nano-selenium enhances antioxidant capacity and hypoxia tolerance of grass carp Ctenopharyngodon idella fed with high-fat diet. Aquac. Nutr. 26, 545–557. doi:10.1111/anu.13016
Yu, Q., Xia, C., Han, F., Xu, C., Rombenso, A., Qin, J., et al. (2022). Effect of different dietary selenium sources on growth performance, antioxidant capacity, gut microbiota, and molecular responses in Pacific white shrimp litopenaeus vannamei. Aquac. Nutr. 2022, 1–16. doi:10.1155/2022/5738008
Zenteno Savín, T., Saldierna, R., and Ahuejote Sandoval, M. (2006). Superoxide radical production in response to environmental hypoxia in cultured shrimp. Comp. Biochem. Physiology Part C Toxicol. Pharmacol. 142, 301–308. doi:10.1016/j.cbpc.2005.11.001
Zhang, D. G., Zhao, T., Xu, X. J., Lv, W. H., and Luo, Z. (2021). Dietary marginal and excess selenium increased triglycerides deposition, induced endoplasmic reticulum stress and differentially influenced selenoproteins expression in the anterior and middle intestines of Yellow Catfish Pelteobagrus fulvidraco. Antioxidants 10, 535. doi:10.3390/antiox10040535
Zhang, D. G., Zhao, T., Xu, X. J., Xu, Y. H., Wei, X. L., Jiang, M., et al. (2022). Selenoprotein F (SELENOF)-mediated AKT1-FOXO3a-PYGL axis contributes to selenium supranutrition-induced glycogenolysis and lipogenesis. Biochimica Biophysica Acta (BBA) - Gene Regul. Mech. 1865, 194814. doi:10.1016/j.bbagrm.2022.194814
Zheng, L., Feng, L., Jiang, W. D., Wu, P., Tang, L., Kuang, S. Y., et al. (2018). Selenium deficiency impaired immune function of the immune organs in young grass carp (Ctenopharyngodon idella). Fish Shellfish Immunol. 77, 53–70. doi:10.1016/j.fsi.2018.03.024
Keywords: selenium, aquatic animals, antioxidant, oxidative stress, selenoprotein
Citation: Li Z-M, Wang X-L, Jin X-M, Huang J-Q and Wang L-S (2023) The effect of selenium on antioxidant system in aquaculture animals. Front. Physiol. 14:1153511. doi: 10.3389/fphys.2023.1153511
Received: 29 January 2023; Accepted: 13 February 2023;
Published: 26 April 2023.
Edited by:
Zhen Zhang, Feed Research Institute (CAAS), ChinaReviewed by:
Ziwei Zhang, Northeast Agricultural University, ChinaBin Feng, Sichuan Agricultural University, China
Copyright © 2023 Li, Wang, Jin, Huang and Wang. This is an open-access article distributed under the terms of the Creative Commons Attribution License (CC BY). The use, distribution or reproduction in other forums is permitted, provided the original author(s) and the copyright owner(s) are credited and that the original publication in this journal is cited, in accordance with accepted academic practice. No use, distribution or reproduction is permitted which does not comply with these terms.
*Correspondence: Lian-Shun Wang, wanglianshun@dlou.edu.cn