- 1Department of Respiratory Medicine, Faculty of Medicine, Hokkaido University, Sapporo, Japan
- 2Department of Respiratory Medicine, Graduate School of Medicine, Kyoto University, Kyoto, Japan
- 3Department of Medicine, Division of Pulmonary Medicine, Keio University School of Medicine, Tokyo, Japan
- 4Department of Respiratory Medicine, Nara Medical University, Kashihara, Japan
- 5Department of Respiratory Medicine, Tsukuba Medical Center Hospital, Tsukuba, Japan
- 6Data Science Center, Promotion Unit, Institute of Health Science Innovation for Medical Care, Hokkaido University Hospital, Sapporo, Japan
- 7Hokkaido Medical Research Institute for Respiratory Diseases, Sapporo, Japan
Background: The mechanism of high transfer coefficients of the lungs for carbon monoxide (Kco) in non-smokers with asthma is explained by the redistribution of blood flow to the area with preserved ventilation, to match the ventilation perfusion.
Objectives: To examine whether ventilation heterogeneity, assessed by pulmonary function tests, is associated with computed tomography (CT)-based vascular indices and Kco in patients with asthma.
Methods: Participants were enrolled from the Hokkaido-based Investigative Cohort Analysis for Refractory Asthma (Hi-CARAT) study that included a prospective asthmatic cohort. Pulmonary function tests including Kco, using single breath methods; total lung capacity (TLC), using multiple breath methods; and CT, were performed on the same day. The ratio of the lung volume assessed using single breath methods (alveolar volume; VA) to that using multiple breath methods (TLC) was calculated as an index of ventilation heterogeneity. The volume of the pulmonary small vessels <5 mm2 in the whole lung (BV5 volume), and number of BV5 at a theoretical surface area of the lungs from the plural surface (BV5 number) were evaluated using chest CT images.
Results: The low VA/TLC group (the lowest quartile) had significantly lower BV5 number, BV5 volume, higher BV5 volume/BV5 number, and higher Kco compared to the high VA/TLC group (the highest quartile) in 117 non-smokers, but not in 67 smokers. Multivariable analysis showed that low VA/TLC was associated with low BV5 number, after adjusting for age, sex, weight, lung volume on CT, and CT emphysema index in non-smokers (not in smokers).
Conclusion: Ventilation heterogeneity may be associated with low BV5 number and high Kco in non-smokers (not in smokers). Future studies need to determine the dynamic regional system in ventilation, perfusion, and diffusion in asthma.
1 Introduction
Single breath methods are used to determine the rate constant of the alveolar uptake of carbon monoxide (CO) for 10 s at barometric pressure, that is, transfer coefficient of the lung for CO (Kco) and alveolar volume (VA) (Krogh, 1915; Hughes and Pride, 2012). Kco more sensitively reflects the uptake efficiency of alveolar-capillary units than diffusing capacity of the lung for carbon monoxide (DLco), because DLco is affected by the two components of Kco and VA (Shimizu et al., 2018). Though high Kco is known to be a feature of non-smokers with asthma, the mechanism of increasing Kco is not fully understood. Low forced expiratory flow at 50% of the forced vital capacity (FVC) (FEF50) is associated with increased capillary blood volume when adjusted for VA and high Kco in asthma (Stewart, 1988), which led to a speculation that increased blood volume due to negative intrathoracic pressure causes high Kco. Meanwhile, ventilation heterogeneity has been shown to be strongly correlated with airway hyperresponsiveness (Downie et al., 2007) and asthma symptoms (Farah et al., 2012). To regulate pulmonary perfusion, ventilation heterogeneity should be compensated by the redistribution of blood volume, i.e., decreased blood volume to poorly-ventilated area and increased blood volume to well-ventilated area, for an efficient gas-exchange (Prisk et al., 2020), which is suggested as the theory for high Kco in non-smokers with asthma (Hughes and Pride, 2012).
To infer the nature and degree of the ventilation heterogeneity, the physiological assessment such as plethysmography (Cournand et al., 1941; King et al., 2005), has been utilized, together with radiological assessment, including magnetic resonance imaging (MRI) (Svenningsen et al., 2018), single photon emission computed tomography (Svenningsen et al., 2018), and computed tomography (CT) (Shima et al., 2022). The ratio of VA assessed by single breath methods (Krogh, 1915) to total lung capacity (TLC) (Roberts et al., 1990) assessed by multiple breath methods (VA/TLC) has clinical convenience for examining ventilation heterogeneity. VA/TLC decreases along with ventilation heterogeneity because VA is more strongly affected by ventilation heterogeneity due to less CO/helium (He) mixture in single breath, compared with TLC measured in multiple breath methods, where CO/He dilutes more broadly (Hughes and Pride, 2012).
The morphological assessment of pulmonary small vessels on CT has progressed from two dimensional (Matsuoka et al., 2010) to three dimensional (Estépar et al., 2013; Ash et al., 2018; Washko et al., 2019), and was confirmed to correlate with cross-sectional vessel dimensions on histology (Rahaghi et al., 2019). The ratio of the aggregate of small vessel cross-sectional area <5 mm2 (CSA<5) to total lung area (%CSA<5) is higher in patients with pulmonary arterial hypertension than that in healthy controls (Shimizu et al., 2017), suggesting that an elevated pulmonary arterial pressure likely increases the number of detectable small vessels, resulting in an increased number of CSA<5 and higher %CSA<5 value. Contrary to this phenomenon, ventilation heterogeneity may decrease the detectable numbers of CSA<5 reflecting the reduction of blood flow/volume in less ventilated areas.
Collectively, we hypothesized that an efficient blood volume regulation, i.e., a reduced blood volume in less ventilated area and an increased blood volume in well ventilated area, contributes to high Kco in patients with asthma. The aim of this study was to examine whether VA/TLC is associated with the number of small vessels at a cross-sectional area <5 mm2 of theoretical lung surface areas (BV5 number) and the aggregate of blood vessel volumes <5 mm2 in the lungs (BV5 volume), in a multi-center prospective asthmatic cohort.
2 Methods
2.1 Participants
All patients with asthma included in this study had participated in the Hokkaido-based Investigative Cohort Analysis for Refractory Asthma (Hi-CARAT) study, that included individuals with varying levels of asthma severity. From February 2010 to September 2012, patients with asthma were recruited at Hokkaido University Hospital and 29 affiliated hospitals and clinics. The Hi-CARAT study was approved by the Ethics Committee of the Hokkaido University School of Medicine (approval number, 02-001) and registered in the University Hospital Medical Information Network Clinical Trials Registry (UMIN-CTR) system (https://upload.umin.ac.jp/cgi-open-bin/ctr/ctr_view.cgi?recptno; R000003917; ID no. 000003254) (Kimura et al., 2017; Konno et al., 2018). The respiratory physicians made the diagnosis of asthma based on the Global Initiative for Asthma (GINA) criteria (Reddel et al., 2022); the recurrent episodes of respiratory symptoms associated with demonstrable reversible airflow limitation. The definition of severe asthma was based on the American Thoracic Society (ATS) criteria of refractory asthma in 2000 (Proceedings of the ATS workshop, 2000), with a slight modification of the inhaled corticosteroid doses due to its availability in Japan (Konno et al., 2018).
We classified the participants into two groups based on the number of cigarette packs they smoked [non-smokers (<10 pack-years) (N = 117) and smokers (≥10 pack-years) (N = 67)]. Pulmonary function tests followed by CT scan were performed on the same day. Given the severity of asthma in patients, the use of short-acting bronchodilators for at least 12 h, but no other respiratory medications, before obtaining all measurements, was prohibited.
2.2 Quantitative chest CT
Participants underwent a multidetector row spiral CT scan with a 64-detector array (Aquilion Multi, TSX-101A/6A; Toshiba Medical Systems, Tochigi, Japan) at the Hokkaido University Hospital (Shimizu et al., 2022). The scan was performed with no contrast during the full inspiration phase. The acquisition parameters were 120 kVp, 300 mA, 64 detectors, 0.5 mm collimation, slice thickness of 0.5 mm, 0.5 s/rotation, helical pitch of 41, and smooth and sharp reconstruction kernels (FC03 and FC52). Vessel and parenchymal analyses were conducted using FC03, while airway analysis was done using FC52.
2.3 Assessment of vascular-related, airway, and parenchymal indices
2.3.1 Vascular-related indices including BV5 volume and BV5 number
Quantitative analysis of Digital Imaging and Communications in Medicine (DICOM) data were performed using A-VIEW software (Coreline soft Inc., Seoul, South Korea). In brief, the pulmonary vessels were extracted using a threshold of −750 Hounsfield units (HU) (Jerman et al., 2015; Cho et al., 2018). Beyond Frangi algorithm has been adapted to the software to improve the accuracy of evaluating vasculature (Park et al., 2022). The assessment based on Beyond Frangi algorism was validated by s 3D synthetic image with a grid of 230 × 320×100 voxels with isotropic spacing of 0.5 mm created similar to the shape of (cerebral) angiograms: tubular structures with various radii, bents, bifurcations and aneurysms, together with the fifteen 3D-digitally subtracted angiograms. Compared to other algorism by Frangi et al. (1998); Sato et al. (2000); Li et al. (2003), and λ2 (Wiemker et al., 2013) in the novel algorism by Park et al. (2022) showed the relatively higher area under the precision-recall curve, median of the normalized lter’s response inside the reference segmentation and signal-to-noise ratio. The summed number of small vessels at a cross-sectional area <5 mm2 of theoretical lung surface area s at 6 mm from the pleural surfaces in all the lobe (BV5 number) was assessed (Jerman et al., 2015; Cho et al., 2018), while the aggregate blood vessel volume <5 mm2 of the total lungs was defined as BV5 volume (17, 25.26). The ratio of BV5 volume to VA (BV5 volume/VA) was used to assess the relative blood volume to well-ventilated area, which might reflect the state of the capillary bed and the efficacy of diffusion, especially Kco. Given the presumable increase in blood volume in well-ventilated area, we examined whether the relative ratio of blood volume to detectable blood number (BV5 volume/BV5 number) was increased in heterogeneously ventilated lungs.
2.3.2 Percent wall area (%WA), airway fractal dimension (AFD), and percent low attenuation area (%LAA)
Quantitative assessment of airway dimensions and emphysematous regions was performed using Synapse Vincent (Fujifilm Medical, Tokyo, Japan) (Shimizu et al., 2022). The average of airway luminal area and wall area in the central 1/3 of the branches were measured automatically. Luminal area (LA), wall area percent [the ratio of the wall area to the summed area, between the wall and lumen (%WA) at the right apical (RB1) and lateral basal (RB8) segmental airways], and averaged LAs were normalized by the body surface area (BSA). To quantify a whole airway structure, the AFD was calculated based on the box-counting method, as reported (Bodduluri et al., 2018; Kimura et al., 2022). We adopted a percentage of low attenuation area (%LAA), which is the ratio of the LAA < −950 HU to the lung volume on CT, as an index of emphysema.
2.4 Pulmonary function tests
Chestac (Chest MI Inc., Tokyo, Japan) was used for the evaluation of spirometry, DLco, and lung volume. A quality-control protocol was based on the criteria adopted by the Lung Health Study and Japanese Respiratory Society (JRS) guidelines, to improve the accuracy of the examination (Konno et al., 2018). Maintenance and calibration were conducted, in line with the JRS guidelines (Guidelines for pulmonary function tests, 2004).
2.4.1 Spirometry
All measurements of forced expiratory volume in one second (FEV1) or FVC were performed using a rolling seal spirometer. Acceptable spirometry measurements required the examinations to be performed in triplicate and greater than two reproducible measurements were mandatory from up to eight forced expirations, in accordance with JRS guidelines. Of those measurements, the best FEV1 and FVC were recorded. Furthermore, the acceptability of the spirometric data, using flow volume curves, were assessed by an independent investigator, without any other information on the participants.
Predicted values of spirometric measurements were in accordance with the JRS guidelines on pulmonary function tests. The best FEV1 and FVC were recorded, in accordance with the JRS guideline. The pre-bronchodilator values of spirometry are used for the analysis, which are the simultaneous measures with the single breath methods.
2.4.2 DLco, Kco, and VA
Patients were required to refrain from smoking on the day of the test for at least 2 h before measurements. DLco was assessed using the single breath method just after pre-bronchodilator spirometry, in a seated position. After rapid inspiration of a gas mixture that comprised of approximately 0.3% carbon monoxide, 10% helium, 21% oxygen, and 68.7% nitrogen, from the residual volume (RV) level, the patients held their breath for approximately 10 s. Alveolar samples were collected and the washout volumes of 750 mL and 500 mL were discarded in patients with vital capacity ≥2.0 and <2.0 L, respectively. Kco, and VA were measured for the analyses. Regarding the correction for hemoglobin concentration, we adopted an equation by ATS and European Respiratory Society guidelines (Reddel et al., 2022). The prediction equation of Burrows was adopted for DLco and Kco (Burrows et al., 1961).
We considered VA/TLC as the index of ventilation heterogeneity. Low VA/TLC may indicate severe ventilation heterogeneity.
2.4.3 Lung volumes
Lung volumes [TLC, functional residual capacity (FRC), and RV] were assessed using the multiple breaths in helium closed circuit methods. Lung volumes were expressed as percentages of the predicted values according to Nishida prediction equations (Nishida et al., 1961).
2.5 Statistical analysis
We divided non-smokers and smokers with asthma based on the VA/TLC into three groups: low (lowest quartile), moderate (second and third quartiles), and high VA/TLC (highest quartile). For the comparisons of demographics and pulmonary function test values between non-smokers and smokers, Student’s t-test and Wilcoxon signed ran test were used for normally-distributed; log Eosinophil, log IgE, pre%FEV1, FEV1/FVC, and VA/TLC, and non-normally distributed variables; age, height, weight, BMI, duration of asthma, pack-year of tobacco, DLco, %DLco, Kco, %Kco, RVTLC, and %TLC, respectively. Comparing demographics and pulmonary function tests among the three groups (low, moderate, and high VA/TLC), ANOVA, followed by Tukey’s multiple comparison test; Dunn’s test for continuous variables, and chi-square test for categorical variables, were used. As for CT indices, Dunnett’s test was used, taking high VA/TLC group as controls. The correlation of VA/TLC and morphological (CT-based) indices such as BV5 number, BV5 volume, BV5 volume/VA, BV5 volume/BV5 number, %LAA, %WA, and AFD, was examined using Pearson and Spearman correlation coefficients. Multiple regression analysis was performed to evaluate the relationships of BV5 number, BV5 volume with VA/TLC including sex (as a categorical variable), age, weight, lung volume on CT (Wu et al., 2022), %LAA, and %WA. All analyses were performed using JMP 16.0.0 (SAS Institute, Cary, North Carolina, United States).
3 Results
3.1 Characteristics of the participants
Among 213 patients enrolled in the Hi-CARAT study, 24 patients did not have the analyzable CT data for BV5 number, BV5 volume, %WA, AFD, and %LAA, and five patients underwent CT scan that was obtained using different scanners from the other patients, then 184 patients with asthma of 117 non-smokers (male n = 25/female n = 92) and 67 smokers (male n = 20/female n = 47) were included (Figure 1, Table 1). Weight and height were higher in smokers with asthma compared with non-smokers with asthma. %FEV1, FEV1/FVC, Kco, and %Kco were lower in smokers with asthma compared with non-smokers with asthma, while DLco, VA/TLC, RV/TLC, and %TLC were not different between the two groups.
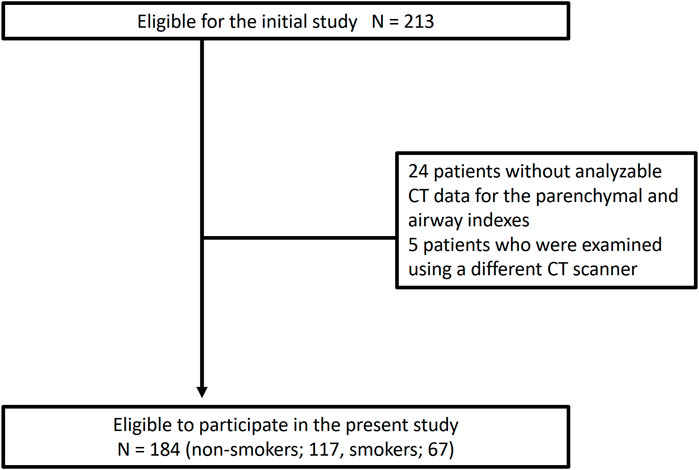
FIGURE 1. Flow chart of participants Of 213 patients in the initial study, 24 patients did not have analyzable CT data for pulmonary vessels, airway, and parenchymal indexes, five patients were examined using a different CT scanner. Finally, 184 patients were included for further analysis.
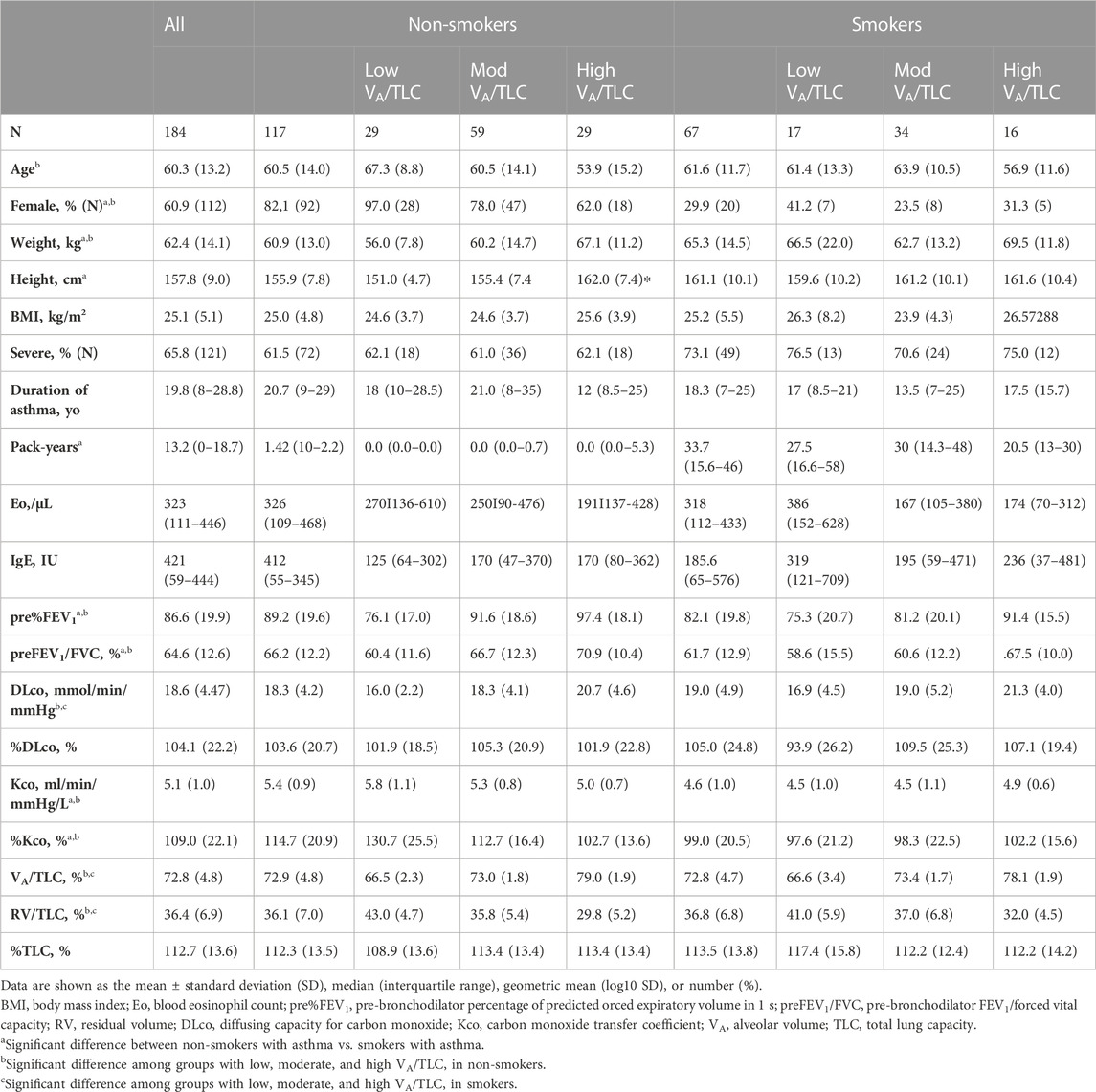
TABLE 1. Characteristics of the participants according to the VA/TLC categories in non-smokers and smokers.
As for the comparison of the groups based on VA/TLC, patients in low VA/TLC group were older and female dominant. In non-smokers with asthma, %FEV1, FEV1/FVC, DLco, BV5 number, and BV5 volume, were significantly lower in low VA/TLC group than in high VA/TLC group (Table 1; Figure 2). Kco and %Kco, RV/TLC, BV5 volume/VA were significantly higher in low VA/TLC group than those in high VA/TLC group. As for smokers with asthma, there were no significant differences in BV5 number, BV5 volume, %Kco, and BV5 volume/VA, but DLco was lower, RV/TLC was higher in low VA/TLC group than those in high VA/TLC group. Figure 3 shows representative three-dimensional imaging and axial CT images in two female participants. One with low VA/TLC (60.9%) had 1943 counts of BV5 number, and 109.8 mL of BV5 volume (A), while another with high VA/TLC (83.3%) had 2812 counts of BV5 number, and 156 mL of BV5 volume (B).
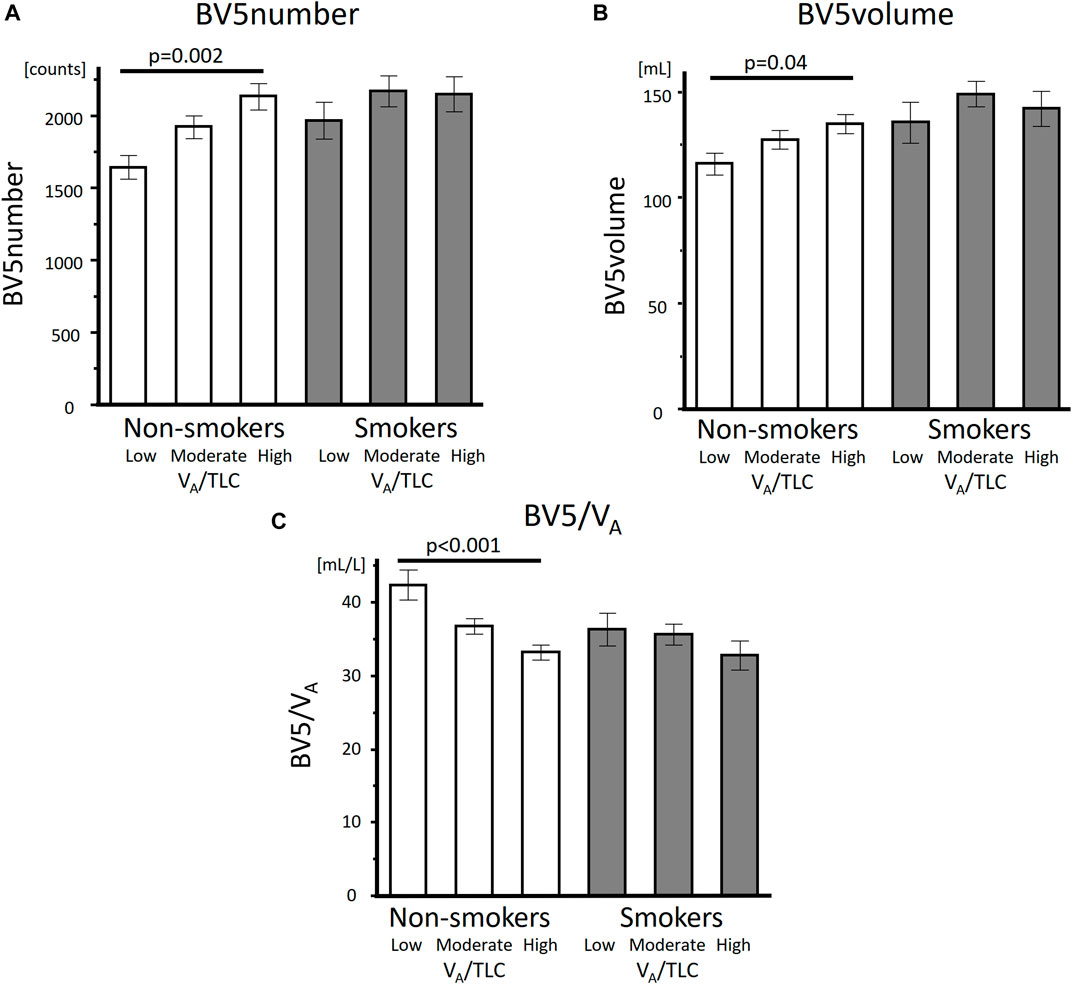
FIGURE 2. Comparisons of BV5 number, BV5 volume, and BV5 volume/VA among the low, moderate, and high VA/TLC groups in non-smokers with asthma and smokers with asthma BV5 number (A), BV5 volume (B) were lower and BV5 volume/VA (C) was higher in low VA/TLC group than those in the high VA/TLC group in non-smokers with asthma, but not in smokers with asthma.
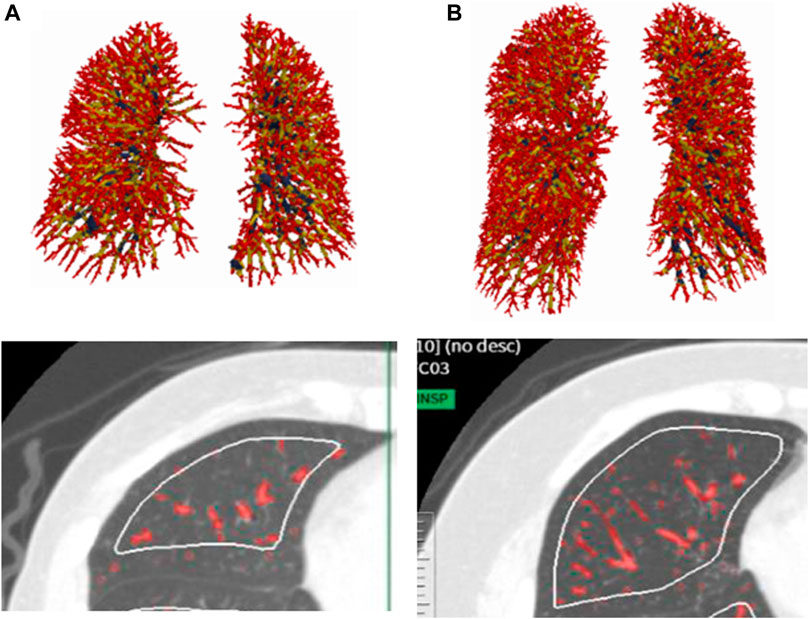
FIGURE 3. Representative three-dimensional imaging and axial CT images in two female patients. One with low VA/TLC (60.9%) had 1943 counts of BV5 number, and 109.8 mL of BV5 volume (A), while another with high VA/TLC (83.%) had 2812 counts of BV5 number, and 156 mL of BV5 volume (B).
3.2 Univariable analysis of morphological CT-based indices and VA/TLC
3.2.1 Non-smokers with asthma
%LAA was .88 (.11–.81); median (interquartile range). %WA and AFD were 59.2 (7.5), 1.93 (.06); average (standard deviation), respectively. %LAA is Low VA/TLC significantly correlated with high %WA, but there was no significant correlation between VA/TLC and %LAA (Table 2).
3.2.2 Smokers with asthma
%LAA was 2.75 (.16–2.39); median (interquartile range). %WA and AFD were 58.8 (6.0), 1.94 (.06); average (standard deviation), respectively. Low VA/TLC significantly correlated with airway disease (high %WA) and parenchymal disease (%LAA) (Table 2).
3.3 Relationships of VA/TLC with BV5 number and BV5 volume, and with BV5 volume/BV5 number
3.3.1 Non-smokers with asthma
VA/TLC was positively associated with BV5 number and negatively with BV5 volume/BV5 number (Figure 4 and Figure 5) Multivariable analysis showed that low VA/TLC correlated with low BV5 number, but not low BV5 volume, independent of age, sex, weight, lung volume on CT, %LAA (Table 3 and Table 4).
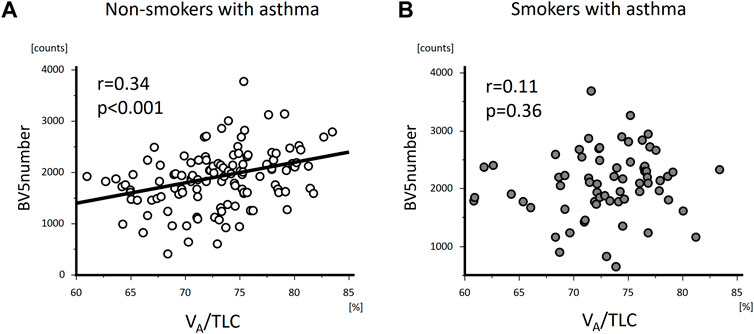
FIGURE 4. The relationships of VA/TLC with BV5 number in non-smokers and smokers with asthma. VA/TLC was positively associated with BV5 number in non-smokers with asthma (A), but not in smokers with asthma (B).
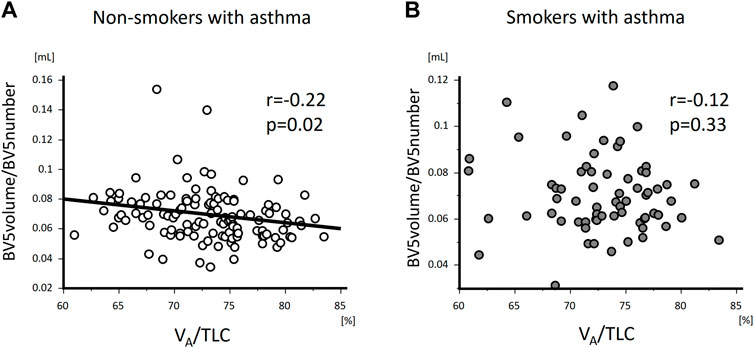
FIGURE 5. The relationships of VA/TLC with BV5 volume/BV5 number in non-smokers and smokers with asthma VA/TLC was negatively associated with BV5 volume/BV5 number in non-smokers with asthma (A), but not in smokers with asthma (B).
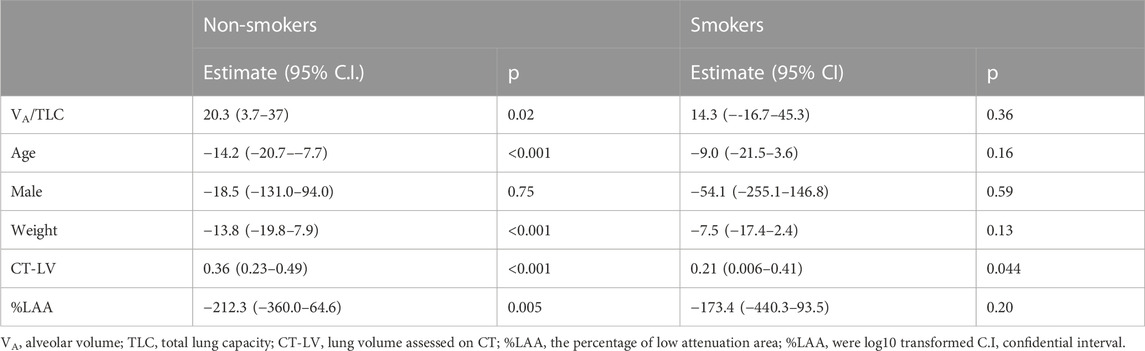
TABLE 3. Relationships of VA/TLC with BV5 number in non-smokers and smoker with asthma (Multivariable anaiysis).
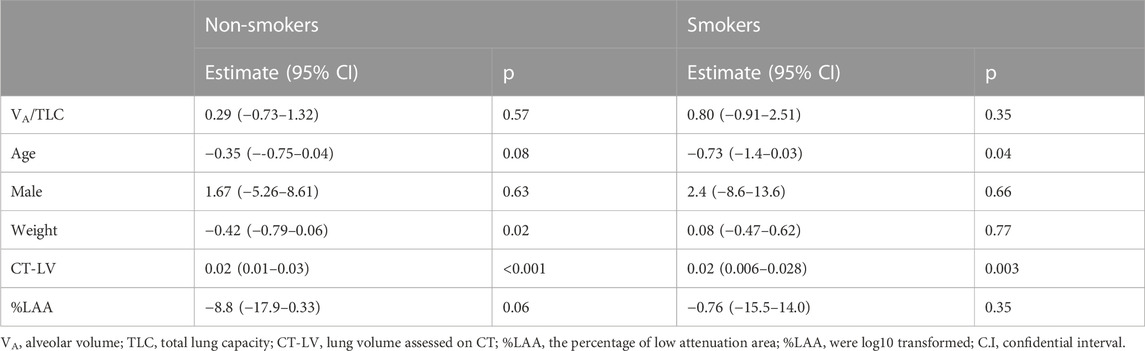
TABLE 4. Relationships of VA/TLC with BV5 volume in non-smokers and smokers with asthma (Multivariable analysis).
3.3.2 Smokers with asthma
VA/TLC did not correlate with BV5 number or BV5 volume/BV5 number (Figure 4 and Figure 5), as was the case with the multivariable analysis on the relationship with BV5 number or BV5 volume (Table 3 and Table 4).
4 Discussion
This morphological study demonstrated that low VA/TLC was associated with a reduction in pulmonary small vessel number (BV5 number), volume (BV5 volume), an increase in BV5 volume/BV5 number, and Kco in non-smokers with asthma. These may suggest that the number of detectable small blood vessels decreases and the volume of detectable small vessels increases as ventilation heterogeneity progresses, which is in harmony with the physiological theory for the redistribution of blood volume to match the ventilation-perfusion, followed by high Kco in intact alveolar-capillary units in non-smokers with asthma. Whereas low VA/TLC did not show any association with BV5 number, BV5 volume, or Kco in smokers with asthma.
Ventilation heterogeneity represented by VA/TLC was related to decreased BV5 number after adjusting for age, sex, lung volume on CT (Wu et al., 2022), and emphysema index in non-smokers with asthma. Moreover, the ratio of BV5 volume to BV5 number increased as VA/TLC decreased, which might suggest an increased blood volume in the well-ventilated area. Thus, those alterations of CT-based vasculature may support the redistribution of blood volume for ventilation-perfusion matching in non-smokers with asthma. Stewart showed that a high ratio of pulmonary capillary blood volume to lung volume assessed by the single breath methods was related to a high Kco in asthma with mild airflow limitation (Stewart, 1988), which is concordant to a high BV5 volume/VA and %Kco in the low VA/TLC group, in the current study. Increased blood flow to the apex of the lungs assessed by an increased Xe uptake in the upright position was reported as an associated factor for high Kco in non-smokers with asthma (Collard et al., 1994). A regional (Sato et al., 2015) and dynamic simultaneous assessment of pulmonary circulation and ventilation with high resolution under the patient being in an upright position, would totally unveil the linkage of ventilation, perfusion, and diffusion in heterogeneously ventilated lungs of non-smokers with asthma.
Of note, low VA/TLC correlated with neither high Kco, low BV5 number, nor low BV5 volume in smokers with asthma. It is presumed that emphysematous lungs fail to compensate the diffusion via the redistribution of blood volume (Hughes and Pride, 2012). Though the patients with asthma in the current study had limited evidence of emphysema on CT, smoking may cause the dysfunction of the alveolar capillary units, which might be linked to a decreased vascular endothelial growth factor in the bronchoalveolar lavage fluid (Nagai et al., 2005). A dynamic CT study showed more prominent heterogeneity of the mean transient time and the pulmonary blood flow was identified in smokers with subtle centrilobular emphysema, compared with non-smokers or smokers with no emphysema on CT (Alford et al., 2010), which might imply that the vascular dysfunction led to a failure of V/Q match; this should be fully explored in a future study.
BV5 volume and BV5 number have differential roles in assessing a distribution of blood volume on CT. BV5 number is the number of vessels <5 mm2 in the theoretical lung surface area at 6 mm depths from the pleural surface, while BV5 volume is equivalent to the total volume of vessels <5 mm2 in the entire lungs. The maldistribution of ventilation causes an increase in the blood volume in well-ventilated areas and a decrease in poorly-ventilated areas, simultaneously, leading to the relatively preserved BV5 volume. It is presumed that a low number of small vessels may reflect the reduction in the blood volume at the level of the alveolar capillary unit more sensitively, compared with that of BV5 volume. The novel modality, which enables the combined assessment of ventilation and perfusion (Wang et al., 2021) beyond the resolution of CT, would further broaden the insight into the micro hemodynamics in the lungs.
Low VA/TLC correlated with low %FEV1 and FEV1/FVC both in non-smokers and smokers with asthma. However, the differential associations of CT-based morphological indices with VA/TLC were suggested between non-smokers and smokers with asthma in this study. %WA at the segmental generation and AFD, but not %LAA, were related to VA/TLC in non-smokers with asthma, while %WA, %LAA, but not AFD, were related to VA/TLC in smokers with asthma. AFD reflects the morphological complexity of the whole bronchial tree on CT, a decrease of which is caused by airway narrowing due to the remodeling of the airway or secretion/mucus in the airway (Bodduluri et al., 2018). All proximal airway diseases may affect ventilation heterogeneity in non-smokers with asthma, which is concordant with previous reports (Foy et al., 2020).
To understand that the relationship of ventilation heterogeneity with gas-exchange is of clinical use in the management of respiratory diseases (Neder et al., 2019; Stanojevic et al., 2022). The value of DLco, which is composed both of VA and Kco, does not specify the state of ventilation or gas-exchange. This study confirms the significance of combined interpretation of VA and Kco, suggesting that reduced VA leads to increased Kco in the lungs with no distinct alveolar-capillary damage, vice versa, a reduced Kco combined with reduced VA implies the existence of alveolar-capillary damages.
There are several limitations in this study. First, this was not a large-scale study. However, the participants cut across the disease severity categories in both non-smokers and smokers with asthma. Therefore, the findings may be generalized. Second, BV5 volume is the total of the blood volume <5 mm2 in the lungs, while the BV5 number was not the total count of BV5 in the whole lungs, but that of the vasculature <5 mm2 on CT at the theoretical surface area 6 mm from the pleural surface in all the lobes. Nevertheless, for differences in indices, both BV5 number and BV5 volume may relatively reflect the features of the whole lung. Third, the patients were in the supine position during the CT scan, while they were in the upright position during the pulmonary function tests; therefore, the differential postures may affect the results. A novel modality, such as radiological examination, where the patients are in the upright position would ultimately solve this issue of radiological structure-function study. Fourth, this study assessed the relationship of ventilation heterogeneity assessed by pulmonary function tests with vasculature on CT in the whole lung. Thus, the regional micro-network on ventilation-perfusion match should be explored to broaden our insight into ventilation and the corresponding blood redistribution, which might lead to one of the novel interventions for hypo-oxygenation. Fifth, according to the quantitative analysis of pulmonary vessels, the cut-off value of −750HU to extract vasculature on volumetric CT should be validated by future studies.
In conclusion, CT-based morphological vascular assessment showed a smaller number of detectable small vasculature and increased blood volume for the given VA (BV5 volume/VA) or vessel on CT (BV5 volume/BV5 number) and high diffusion in heterogeneously-ventilated lungs of non-smokers with asthma. Advanced methodology is required for the comprehensive knowledge of the intact regulation among respiratory organs and disruption of that system.
Data availability statement
The original contributions presented in the study are included in the article/Supplementary materials, further inquiries can be directed to the corresponding author.
Ethics statement
The studies involving human participants were reviewed and approved by Hokkaido University Hospital. The patients/participants provided their written informed consent to participate in this study.
Author contributions
KS: study conception and design, CT analysis, statistical analysis, acquisition and interpretation of data, and drafting of the manuscript; HK, MS, and HM: acquisition and interpretation of data; NaT, SC, SS, KT, and HI: design of the study, editing of the interpretation of data; AO: CT analysis, YI: statistical advice, NW, MT-S, MM-S, YA, and NoT: interpretation of data; MN and SK: acquisition and interpretation of data and finalizing of the manuscript.
Funding
The Hokkaido-based Investigative Cohort Analysis for Refractory Asthma (Hi-CARAT) Study is supported by the Ministry of Education, Culture, Sports, Science and Technology of Japan (24249049 to MN, 26461151 to SK) and a research grant from the Japan Allergy Foundation, AstraZeneca, and Kyorin.
Acknowledgments
The authors thank Ichizo Tsujino for the advices on the assessment of pulmonary small vessels, the Hokkaido-based Investigative Cohort Analysis for Refractory Asthma investigators for recruitment of patients as well as Hideka Ashikaga, Ayako Kondo, and Yuko Takagi of the Central Office of the Hokkaido-based Investigative Cohort Analysis for Refractory Asthma (Sapporo, Japan) and the staff of Exam Co., Ltd. (Sapporo, Japan) for data management, Tsukasa Sasaki in the Division of Radiology, Department of Diagnosis and Treatment Support, the staff in the Division of Pulmonary Function, Department of Laboratory Medicine, Hokkaido University Hospital for technical support.
Conflict of interest
MS received grants from GlaxoSmithKline, Novartis, and AstraZeneca outside the current work. NT, and SS, report a grant from Fujifilm Medical outside the current work.
The remaining authors declare that the research was conducted in the absence of any commercial or financial relationships that could be construed as a potential conflict of interest.
Publisher’s note
All claims expressed in this article are solely those of the authors and do not necessarily represent those of their affiliated organizations, or those of the publisher, the editors and the reviewers. Any product that may be evaluated in this article, or claim that may be made by its manufacturer, is not guaranteed or endorsed by the publisher.
References
Alford, S. K., van Beek, E. J., McLennan, G., and Hoffman, E. A. (2010). Heterogeneity of pulmonary perfusion as a mechanistic image-based phenotype in emphysema susceptible smokers. Proc. Natl. Acad. Sci. U. S. A. 107, 7485–7490. doi:10.1073/pnas.0913880107
Ash, S. Y., Rahaghi, F. N., Come, C. E., Ross, J. C., Colon, A. G., Cardet-Guisasola, J. C., et al. (2018). Pruning of the pulmonary vasculature in asthma. The severe asthma research program (SARP) cohort. Am. J. Respir. Crit. Care Med. 198, 39–50. doi:10.1164/rccm.201712-2426OC
Bodduluri, S., Puliyakote, A. S. K., Gerard, S. E., Reinhardt, J. M., Hoffman, E. A., Newell, J. D., et al. Investigators, COPDGene (2018). Airway fractal dimension predicts respiratory morbidity and mortality in COPD. J. Clin. Invest 128, 5676–5382. doi:10.1172/JCI125987
Burrows, B., Kasik, J. E., Niden, A. H., and Barclay, W. R. (1961). Clinical usefulness of the single-breath pulmonucy diffusing capacity test. Am. Rev. Respir. Dis. 84, 789–806. doi:10.1164/arrd.1961.84.6.789
Cho, Y. H., Lee, S. M., Seo, J. B., Kim, N., Bae, J. P., Lee, J. S., et al. (2018). Quantitative assessment of pulmonary vascular alterations in chronic obstructive lung disease: Associations with pulmonary function test and survival in the KOLD cohort. Eur. J. Radiol. 108, 276–282. doi:10.1016/j.ejrad.2018.09.013
Collard, P., Njinou, B., Nejadnik, B., Keyeux, A., and Frans, A. (1994). Single breath diffusing capacity for carbon monoxide in stable asthma. Chest 105, 1426–1429. doi:10.1378/chest.105.5.1426
Cournand, A., Baldwin, E. D., Darling, R. C., and Richards, D. W. (1941). Studies on intrapulmonary mixture of gases: IV. The significance of the pulmonary emptying rate and a simplified open circuit measurement of residual air. J. Clin. Invest 20, 681–689. doi:10.1172/JCI101261
Downie, S., Salome, C., Verbanck, S., Thompson, B., and King, G. G. (2007). Ventilation heterogeneity is a major determinant of airway hyperresponsiveness in asthma, independent of airway inflammation. Thorax 62, 684–689. doi:10.1136/thx.2006.069682
Estépar, R. S., Kinney, G. L., Black-Shinn, J. L., Bowler, R. P., Kindlmann, G. L., Ross, J. C., et al. COPDGene, Study (2013). Computed tomographic measures of pulmonary vascular morphology in smokers and their clinical implications. Am. J. Respir. Crit. Care Med. 188, 231–239. doi:10.1164/rccm.201301-0162OC
Farah, C. S., King, G. G., Brown, N. J., Downie, S. R., Kermode, J. A., Hardaker, K. M., et al. (2012). The role of the small airways in the clinical expression of asthma in adults. J. Allergy Clin. Immunol. 129, 381–387, 387.e1. doi:10.1016/j.jaci.2011.11.017
Foy, B., Kay, D., Siddiqui, S., Brightling, C., Paiva, M., and Verbanck, S. (2020). Increased ventilation heterogeneity in asthma can be attributed to proximal bronchioles. Eur. Respir. J. 55, 1901345. doi:10.1183/13993003.01345-2019
Frangi, A. F., Niessen, W. J., Vincken, K. L., and Viergever, M. A. (1998). Multiscale vessel enhancement filtering, [Medical image computing and computer-assisted intervention - miccai’98 ], W. M. Wells, A. Colchester, and S Delp, eds, 1496, 130–137. Springer-Verlag Berlin, Berlin.
Guidelines for pulmonary function tests (2004). Guidelines for pulmonary function tests: Spirometry, flow-volume curve, diffusion capacity of the lung. In Japanese. Tokyo, Japan: The Japanese Respiratory Society. Available at: https://www.ncbi.n lm.nih.gov/pubmed/15565748 (Accessed March 20, 2021).
Hughes, J. M., and Pride, N. B. (2012). Examination of the carbon monoxide diffusing capacity (DL(CO)) in relation to its KCO and VA components. Am. J. Respir. Crit. Care Med. 186, 132–139. doi:10.1164/rccm.201112-2160CI
Jerman, T., Pernuš, F., Likar, B., and Špiclin, Ž. (2015). Beyond Frangi: An improved multiscale vesselness filter medical imaging 2015: Image processing. SPIE, 623–633. doi:10.1117/12.2081147
Kimura, H., Konno, S., Nakamaru, Y., Makita, H., Taniguchi, N., Shimizu, K., et al. (2017). Sinus computed tomographic findings in adult smokers and nonsmokers with asthma. Analysis of clinical indices and biomarkers. Ann. Am. Thorac. Soc. 14, 332–341. doi:10.1513/AnnalsATS.201606-463OC
Kimura, H., Shimizu, K., Tanabe, N., Makita, H., Taniguchi, N., Kimura, H., et al. (2022). Further evidence for association of YKL-40 with severe asthma airway remodeling. Ann. Allergy Asthma Immunol. 128, 682–688.e5. doi:10.1016/j.anai.2022.03.016
King, G. G., Downie, S. R., Verbanck, S., Thorpe, C. W., Berend, N., Salome, C. M., et al. (2005). Effects of methacholine on small airway function measured by forced oscillation technique and multiple breath nitrogen washout in normal subjects. Respir. Physiol. Neurobiol. 148, 165–177. doi:10.1016/j.resp.2005.05.001
King, G. G., Eberl, S., Salome, C. M., Young, I. H., and Woolcock, A. J. (1998). Differences in airway closure between normal and asthmatic subjects measured with single-photon emission computed tomography and technegas. Am. J. Respir. Crit. Care Med. 158, 1900–1906. doi:10.1164/ajrccm.158.6.9608027
Konno, S., Taniguchi, N., Makita, H., Nakamaru, Y., Shimizu, K., Shijubo, N., et al. (2018). Distinct phenotypes of smokers with fixed airflow limitation identified by cluster analysis of severe asthmafied by cluster analysis of severe asthma. Ann. Am. Thorac. Soc. 15, 33–41. doi:10.1513/AnnalsATS.201701-065OC
Krogh, M. (1915). The diffusion of gases through the lungs of man. J. Physiol. 49, 271–300. doi:10.1113/jphysiol.1915.sp001710
Li, Q., Sone, S., and Doi, K. (2003). Selective enhancement filters for nodules, vessels, and airway walls in two- and three-dimensional CT scans. Med. Phys. 30, 2040–2051. doi:10.1118/1.1581411
Matsuoka, S., Washko, G. R., Yamashiro, T., Estepar, R. S., Diaz, A., Silverman, E. K., et al. National Emphysema Treatment Trial Research Group (2010). Pulmonary hypertension and computed tomography measurement of small pulmonary vessels in severe emphysema. Am. J. Respir. Crit. Care Med. 181, 218–225. doi:10.1164/rccm.200908-1189OC
Nagai, K., Betsuyaku, T., Ito, Y., Nasuhara, Y., and Nishimura, M. (2005). Decrease of vascular endothelial growth factor in macrophages from long-term smokers. Eur. Respir. J. 25, 626–633. doi:10.1183/09031936.05.00110504
Neder, J. A., Berton, D. C., Muller, P. T., and O’Donnell, D. E. (2019). Incorporating lung diffusing capacity for carbon monoxide in clinical decision making in chest medicine. Clin. Chest Med. 40, 285–305. doi:10.1016/j.ccm.2019.02.005
Nishida, O., Sewake, N., Kambe, M., Okamoto, T., and Takano, M. (1961). Pulmonary function in healthy subjects and its prediction. 4. Subdivisions of lung volume in adults. (In Japanese) Rinsho Byori 24, 837–884.
Park, S. W., Lim, M. N., Kim, W. J., and Bak, S. H. (2022). Quantitative assessment the longitudinal changes of pulmonary vascular counts in chronic obstructive pulmonary disease. Respir. Res. 23, 29. doi:10.1186/s12931-022-01953-7
Prisk, G. K., Petersen, G. M., Geier Etsá, R. C., and Sa, R. C. (2020). Ventilatory heterogeneity in the normal human lung is unchanged by controlled breathing. J. Appl. Physiol. 129, 1152–1160. doi:10.1152/japplphysiol.00278.2020
Proceedings of the ATS workshop on refractory asthma: Current understanding, recommendations, and unanswered questions. Am. Thorac. Soc. Am J Respir Crit Care Med (2000)162:2341–2351. doi:10.1164/ajrccm.162.6.ats9-00
Rahaghi, F. N., Argemí, G., Nardelli, P., Domínguez-Fandos, D., Arguis, P., Peinado, V. I., et al. (2019). Pulmonary vascular density: Comparison of findings on computed tomography imaging with histology. Eur. Respir. J. 54, 1900370. doi:10.1183/13993003.00370-2019
Reddel, H. K., Bacharier, L. B., Bateman, E. D., Brightling, C. E., Brusselle, G. G., Buhl, R., et al. (2022). Global initiative for asthma strategy 2021: Executive summary and rationale for key changes. Am. J. Respir. Crit. Care Med. 205, 17–35. doi:10.1164/rccm.202109-2205PP
Roberts, C. M., MacRae, K. D., and Seed, W. A. (1990). Multi-breath and single breath helium dilution lung volumes as a test of airway obstruction. Eur. Respir. J. 3, 515–520. doi:10.1183/09031936.93.03050515
Sato, S., Bartolák-Suki, E., Harikrishnan Parameswaran, H., Hamakawa, H., and Suki, B. (2015). Scale dependence of structure-function relationship in the emphysematous mouse lung. Front. Physiol. 12, 6–146. doi:10.3389/fphys.2015.00146
Sato, Y., Westin, C. F., Bhalerao, A., Nakajima, S., Shiraga, N., Tamura, S., et al. (2000). Tissue classification based on 3d local intensity structures for volume rendering, IEEE T. Vis. Comput. Gr. 6, 160–180. doi:10.1109/2945.856997
Shima, H., Tanabe, N., Oguma, A., Shimizu, K., Kaji, S., Terada, K., et al. (2022). Subtyping emphysematous COPD by respiratory volume change distributions on CT. Thorax 29, 218288. Online ahead of print. doi:10.1136/thoraxjnl-2021-218288
Shimizu, K., Konno, S., Makita, H., Kimura, H., Kimura, H., Suzuki, M., et al. (2018). Transfer coefficients better reflect emphysematous changes than carbon monoxide diffusing capacity in obstructive lung diseases. J. Appl. Physiol. 125, 183–189. doi:10.1152/japplphysiol.01062.2017
Shimizu, K., Tanabe, N., Oguma, A., Kimura, H., Suzuki, M., Yokota, I., et al. (2022). Parenchymal destruction in asthma: Fixed airflow obstruction and lung function trajectory. J. Allergy Clin. Immunol. 149, 934–942.e8. doi:10.1016/j.jaci.2021.07.042
Shimizu, K., Tsujino, I., Sato, T., Sugimoto, A., Nakaya, T., Watanabe, T., et al. (2017). Performance of computed tomography-derived pulmonary vasculature metrics in the diagnosis and haemodynamic assessment of pulmonary arterial hypertension. Eur. J. Radiol. 96, 31–38. doi:10.1016/j.ejrad.2017.09.010
Stanojevic, S., Kaminsky, D. A., Miller, M. R., Thompson, B., Aliverti, A., Barjaktarevic, I., et al. (2022). ERS/ATS technical standard on interpretive strategies for routine lung function tests. Eur. Respir. J. 60, 2101499. doi:10.1183/13993003.01499-2021
Stewart, R. I. (1988). Carbon monoxide diffusing capacity in asthmatic patients with mild airflow limitation. Chest 94, 332–336. doi:10.1378/chest.94.2.332
Svenningsen, S., Eddy, R. L., Lim, H. F., Cox, P. G., Nair, P., and Parraga, G. (2018). Sputum eosinophilia and magnetic resonance imaging ventilation heterogeneity in severe asthma. Am. J. Respir. Crit. Care Med. 197, 876–884. doi:10.1164/rccm.201709-1948OC
Wang, Z., Rankine, L., Bier, E. A., Mummy, D., Lu, J., Church, A., et al. (2021). Using hyperpolarized 129Xe gas-exchange MRI to model the regional airspace, membrane, and capillary contributions to diffusing capacity. J. Appl. Physiol. 130, 1398–1409. doi:10.1152/japplphysiol.00702.2020
Washko, G. R., Nardelli, P., Ash, S. Y., Vegas Sanchez-Ferrero, G., Rahaghi, F. N., Come, C. E., et al. (2019). Arterial Vascular pruning, right ventricular size, and clinical outcomes in chronic obstructive pulmonary disease. A longitudinal observational study. Am. J. Respir. Crit. Care Med. 200, 454–461. doi:10.1164/rccm.201811-2063OC
Wiemker, R., Klinder, T., Bergtholdt, M., Meetz, K., Carlsen, I. C., and Buelow, T. (2013). A radial structure tensor and its use for shape-encoding medical visualization of tubular and nodular structures. IEEE Trans. Vis. Comput. Graph. 19, 353–366. doi:10.1109/TVCG.2012.136
Keywords: asthma, computed tomography, pulmonary small vessels, ventilation heterogeneity, Kco
Citation: Shimizu K, Kimura H, Tanabe N, Chubachi S, Sato S, Suzuki M, Tanimura K, Iijima H, Oguma A, Ito YM, Wakazono N, Takimoto-Sato M, Matsumoto-Sasaki M, Abe Y, Takei N, Makita H, Nishimura M, Konno S and Hi-CARAT investigators (2023) Relationships of computed tomography-based small vessel indices of the lungs with ventilation heterogeneity and high transfer coefficients in non-smokers with asthma. Front. Physiol. 14:1137603. doi: 10.3389/fphys.2023.1137603
Received: 04 January 2023; Accepted: 17 February 2023;
Published: 01 March 2023.
Edited by:
Lars Knudsen, Hannover Medical School, GermanyReviewed by:
Sanghun Choi, Kyungpook National University, Republic of KoreaHaribalan Kumar, The University of Auckland, New Zealand
Copyright © 2023 Shimizu, Kimura, Tanabe, Chubachi, Sato, Suzuki, Tanimura, Iijima, Oguma, Ito, Wakazono, Takimoto-Sato, Matsumoto-Sasaki, Abe, Takei, Makita, Nishimura, Konno and Hi-CARAT investigators. This is an open-access article distributed under the terms of the Creative Commons Attribution License (CC BY). The use, distribution or reproduction in other forums is permitted, provided the original author(s) and the copyright owner(s) are credited and that the original publication in this journal is cited, in accordance with accepted academic practice. No use, distribution or reproduction is permitted which does not comply with these terms.
*Correspondence: Kaoruko Shimizu, okaoru@med.hokudai.ac.jp