- 1Department of Implantology, School and Hospital of Stomatology, Cheeloo College of Medicine, Shandong Key Laboratory of Oral Tissue Regeneration & Shandong Engineering Laboratory for Dental Materials and Oral Tissue Regeneration, Shandong Provincial Clinical Research Center for Oral, Shandong University , Jinan, China
- 2Taian Municipal Hospital, Tai’an, China
- 3School of Stomatology, Shandong First Medical University, Shandong Academy of Medical Sciences, Jinan, China
Soft tissue seal around implant prostheses is considered the primary barrier against adverse external stimuli and is a critical factor in maintaining dental implants’ stability. Soft tissue seal is formed mainly by the adhesion of epithelial tissue and fibrous connective tissue to the transmembrane portion of the implant. Type 2 diabetes mellitus (T2DM) is one of the risk factors for peri-implant inflammation, and peri-implant disease may be triggered by dysfunction of the soft tissue barrier around dental implants. This is increasingly considered a promising target for disease treatment and management. However, many studies have demonstrated that pathogenic bacterial infestation, gingival immune inflammation, overactive matrix metalloproteinases (MMPs), impaired wound healing processes and excessive oxidative stress may trigger poor peri-implant soft tissue sealing, which may be more severe in the T2DM state. This article reviews the structure of peri-implant soft tissue seal, peri-implant disease and treatment, and moderating mechanisms of impaired soft tissue seal around implants due to T2DM to inform the development of treatment strategies for dental implants in patients with dental defects.
1 Introduction
Diabetes mellitus is a group of metabolic diseases characterized by hyperglycemia. Chronic high blood glucose levels are associated with physical damage and failure of various organs and tissues and are one of the leading causes of death worldwide (Awad et al., 2021). Data released by the International Diabetes Federation in 2021 show that the world population with diabetes is approximately 463 million (20–79 years old) (Schacter and Leslie, 2021). Diabetes seriously affects patients’ quality of life and life expectancy and has become a severe public health problem (Zheng et al., 2018a; Hu and Jia, 2018). The vast majority of diabetes cases can be divided into two major classes, type 1 diabetes mellitus (T1DM) and type 2 diabetes mellitus (T2DM) (American Diabetes Association, 2013). T1DM is an autoimmune chronic disease, and its cause is an absolute deficiency of insulin due to the autoimmune destruction of the pancreatic beta cells (Rodrigues Oliveira et al., 2023). In distinction, T2DM is an endocrine metabolic disorder, accounting for approximately 90%–95% of all diabetic patients (American Diabetes Association, 2019), due to resistance to insulin action and inadequate compensatory insulin secretory response (American Diabetes Association, 2013).
Implant therapy is gradually becoming the restorative option for patients with missing or edentulous teeth for its advantages, such as comfort and aesthetics. The longevity and long-term functional stability of implant dentures depend on the integration of the implant with the bone and the soft tissue barrier that protects the implant and the alveolar bone from external stimuli. The soft tissue is firmly attached to the implant, promoting its aesthetic and functional stability, and is an essential factor in the long-term outcome of the implants (Zhao et al., 2014). In recent years, peri-implant soft tissue seal has received increasing attention. However, relevant clinical and basic research is scarce, and this unique implant-mucosa interface’s role and formation process are still unclear.
Nevertheless, complications inevitably occur during implant restoration and later denture function, including surgical, mechanical, and biological complications (Romanos et al., 2019). Biological complications of implant prostheses refer to inflammatory damage to the soft and bone tissues surrounding the implant, mainly including peri-implant mucositis and peri-implantitis (Kwon et al., 2020). Peri-implantitis is an inflammation of the peri-implant mucosa and progressive peri-implant bone loss initiated by pathogenic bacteria and is the leading cause of implant detachment (Buser et al., 2017). The oral mucosa protects the periodontal tissues against bacteria and other harmful substances. Studies have revealed that peri-implant soft tissue inflammation can induce pathological changes at the implant-surrounding tissue interface, causing resorption of the peri-implant bone (Chackartchi et al., 2019). In short, a suitable implant denture must perform the occlusal function and form an excellent soft tissue barrier to achieve the aesthetic requirements and produce a protective effect.
The literature on diabetes and obesity reports that up to 50% of dental implants may be affected by peri-implantitis, that diabetes rises the risk of peri-implantitis, and that poor glycemic control significantly elevates the rate of implant failure (Moy et al., 2005; Ferreira et al., 2006; Daubert et al., 2015; Monje et al., 2017). Patients with glucose levels greater than 8.0% HbA1c at the time of implant placement have a detrimental effect on bone metabolism and implant integration rates, with a 2-fold delay in implant integration rates (Aguilar-Salvatierra et al., 2016). Although implants are initially stable in diabetic and obese patients, implant-bone integration is reduced, making the implant more susceptible to peri-implantitis (Chrcanovic et al., 2014; Coelho et al., 2018). In addition to this, the diabetic state may increase the pathogenic flora and exacerbate the local inflammatory response, increasing the susceptibility and severity of peri-implant mucosal tissue and bone, and inflammation extending from the soft tissue margins may exacerbate the alteration of the implant-bone interface, further accelerating the bone loss associated with peri-implantitis (Nibali et al., 2022). And especially, differing from T1DM, which did not adversely affect the incidence of inflammation (Sannino et al., 2020), marginal bone loss and implant survival in dental implants, patients with T2DM had increased clinical and radiological peri-implant indices such as plaque index (PI), bleeding on probing (BOP), probing depth (PD) and marginal bone loss (MBL) after implant surgery compared to the non-diabetic group (Alrabiah et al., 2018). As a result, T2DM patients may be at higher risk of implant loss associated with peri-implantitis.
A detailed dissection of the physiological structure and microstructure of the implant-gingival interface is presented here, presenting evidence for the influence of pathogenic bacteria, gingival inflammation, and epithelial migration on soft tissue seal, and the effect of diabetes on peri-implant soft tissue sealing is analyzed in detail. It is essential to note that some molecular mechanisms are explicitly mentioned in this review, implying that this could be a potential target for ameliorating soft tissue seals.
2 The importance of the soft tissue seal in dental implant therapy and peri-implant disease
Since Professor Branmark introduced the concept of osseointegration 50 years ago (Brånemark et al., 1969), dental implants have evolved into a successful and predictable treatment modality for replacing missing teeth (Buser et al., 2017). The key to achieving good clinical results with restorative implant treatment is establishing tight osseointegration, such as direct structural and functional contact between the surface of the load-bearing implant and the ordered arrangement of bone tissue. Dental implants preserve the adjacent tooth structure and bone and can improve chewing function and quality of life in edentulous and partially edentulous patients with restorations (Jivraj and Chee, 2006; Battle-Siatita et al., 2009; Jofre et al., 2013; Hartlev et al., 2014). As a result, oral implant restoration has become an increasingly popular option for restoring missing teeth in patients with missing teeth or edentulous jaws (Tarnow, 2014; Buser et al., 2017).
A unique feature of implants is a mucosa-penetrating component, whereby the abutment forms a transition zone between the implant and the crown, penetrating the soft tissue. In addition to osseointegration, the soft tissue interface surrounding the implant plays a critical role in the long-term success of implant-supported restoration (Chackartchi et al., 2019). These soft tissues separate the peri-implant bone from the oral environment to avoid disruptions in the balance that could lead to the penetration of pathogenic bacteria causing peri-implant disease or bone loss.
The peri-implant disease usually presents in two forms: peri-implant mucositis and peri-implantitis. Peri-implant mucositis is an inflammatory reaction of the peri-implant mucosa without marginal bone loss around the implant; without proper care, it can progress to a more severe condition called peri-implantitis (Matarazzo et al., 2018). Moreover, peri-implantitis is a chronic inflammatory disease caused by dental plaque, a destructive host response caused by local, systemic and environmental factors, including soft tissue inflammation and progressive loss of supporting bone, which can promote osteoclast-mediated bone resorption and inhibit osteoblast-mediated bone formation, resulting in progressive loss of peri-implant bone and is the primary cause of implant loss (Buser et al., 2017). Peri-implant tissues exhibit a more pronounced inflammatory response than teeth with the same amount of plaque, and reversing this inflammation takes longer in the peri-implant tissues (Salvi et al., 2012; Ivanovski and Lee, 2018). Pathogenic bacterial infestation is the initiating factor of peri-implantitis and the leading cause of biological complications in implant dentures (Dreyer et al., 2018; Daubert and Weinstein, 2019).
Implant dentures have been developed as a tool for the dental rehabilitation of patients with diabetic edentulism. Good osseointegration and bio-containment of the soft tissues around the implant are essential for long-term success and stability. The soft tissue-implant integration is the primary defense of the implant against adverse external stimuli and is a fundamental biological basis for oral implants (Chackartchi et al., 2019).
3 The structure of the soft tissue seal around dental implants
3.1 The soft tissue seal in the gingiva–abutment interface
When implants are placed, the healing process occurs, such as connective tissue repair, while epithelial cells move horizontally to cover the wound on the connective tissue (Atsuta et al., 2005a; Sculean et al., 2014; Tomasi et al., 2014). Since the implant blocks the growth and horizontal migration of epithelial and connective tissue, they begin to spread along the implant surface at depth and turn back at a certain depth, thus forming a mucosal tissue similar to that of natural teeth, i.e., the pores formed by the implant are gradually sealed by epithelial and connective tissue (Fujii et al., 1998; Gulati et al., 2020).
Histologically, the peri-implant mucosa consists of well-keratinized oral epithelium, sulcular epithelium and a thin barrier epithelium facing the abutment, which corresponds to the around the tooth, called the peri-implant Junctional Epithelium (JE) (Schroeder et al., 1981; Gould et al., 1984; Buser and Brägger, 1989). The height of the peri-implant JE is approximately 2 mm, and the connective tissue below the JE is about 1.5 mm. Thus, the average biological width usually reaches 3.8 mm (Berglundh and Lindhe, 1996). When the biological width of any part of the peri-implant mucosa decreases, marginal bone resorption is generally observed, so the biological width is adjusted to compensate for these changes. The desmosome junctions in JE are fewer than the oral epithelium, so the intercellular gaps are wide and contain many immune cells such as neutrophils (Shimono et al., 2003). The JE is the first line of defense of the titanium or zirconium oxide peri-implant tissue against pathogen invasion. The epithelial tissue attaches to the surface of the implant system via the inner basement membrane and hemidesmosomes (HDs) (Ivanovski and Lee, 2018; Ren et al., 2019). The HDs are mainly located on the root side of the JE, so the implant’s JE is weakly closed (Bartold et al., 2000; Atsuta et al., 2013; Atsuta et al., 2016).
Among the connective tissue surrounding the implant, it is noteworthy that, due to the absence of the osseous layer, there are no vertical fibers inserted vertically into the implant surface, and the gingiva is directly “adapted” to the implant surface (Fujii et al., 1998), indicating that the sealing capacity of this structure is more fragile than that of a natural tooth and more prone to periodontal rupture and subsequent bacterial invasion (Moon et al., 1999). Compared to natural teeth, fewer fibroblasts and mesenchymal cells are usually intermediate to collagen fibers (Al Rezk et al., 2018). The connective tissue is adhered by gingival fibroblasts to the surface of the implant system through adhesive plaque attachment, tight junctions and extracellular matrix connections, and collagen fibers parallel to the implant surface (Bartold et al., 2000; Amberg et al., 2018).
3.2 The molecular structure of the soft tissue seal
3.2.1 Epithelial tissue
The gingival epithelial cells in the JE rely mainly on the Internal Basal Lamina (IBL) and HDs structures to attach to the surface of the implant system (Bartold et al., 2000). The gingival epithelium secures the internal basal lamina and is divided into a dense and hyaline layer.
The main components of the dense layer are Laminin 332 (Laminin 5) and Collagen XVIII (BP180, BPAG2). Laminin 332 is an important molecule closely related to epithelial cell adhesion and migration and is involved in the attachment of peri-implant epithelium to the implant surface (Ikeda et al., 2000; LeBleu et al., 2007). Laminin is a family of macromolecular glycoproteins composed of disulfide-linked heterotrimeric chains (α, β, γ) that form a cross-like shape (Atsuta et al., 2005a; Aumailley et al., 2005; Atsuta et al., 2005b; Aumailley, 2013; Yamada and Sekiguchi, 2015; Abdallah et al., 2017). It acts as a ligand for Integrins, linked to integrin α6β4 via the α3 chain to form HDs and adhere to the extracellular matrix. The peptide coating containing Laminin 332 directly induces the sealing of keratinocytes around dental implants (Koidou et al., 2018). BP180 is a transmembrane protein adjacent to integrins and plays a vital role in maintaining the link between intracellular and extracellular structural elements involved in adhesion.
Lamina Lucinda is part of the basal lamina and consists of five major components: partial integrin α6β4, partial BP180, plectin, CD151, and BP230 (BPAG1e) (Ghohestani et al., 2001). Integrin α6β4 is a non-covalent heterodimer that is a transmembrane component of HDs (Davis et al., 2001; Holmes and Rout, 2011). The extracellular region α6 of integrin binds to BP180, CD151 and laminin 332 (Rezniczek et al., 1998; Kazarov et al., 2002). Integrin β4 mediates intracellular interactions between plakin family members plectin and BP230 and the keratin cytoskeleton attached to the transmembrane protein BP180. HDs are highly specialized integrin-mediated epithelial attachment structures that allow cells to adhere firmly to the implant surface and establish connections between the epithelial keratin cytoskeleton and the hyaline layer below (Fontao et al., 2001; Koster et al., 2004).
In the intracellular part of the HDs, part of the plectin and BP230 form the dense external block, which, together with the keratin filament-linked subunits and keratin filaments, are called the thick internal block. HDs become the transmembrane connection between the tooth or implant and the gingiva. JE forms a protective barrier for the mechanical stability of the tooth or dental implant and a physical barrier against biofilm invasion (Walko et al., 2015; Atsuta et al., 2016). However, they are distributed almost exclusively in the lower part of the JE at the implant interface, so the peri-implant epithelial seal is significantly weaker than the periodontal tissue. Gingival epithelial cells upregulate the expression of adherent plaques and HDs at early time points, thus forming an infection-free seal surrounding dental implants (Atsuta et al., 2005a; Pendegrass et al., 2015).
3.2.2 Connective tissue
Between the apical edge of the JE and the bone tissue, there is a 200 µm wide zone of connective tissue divided into a central and a lateral zone. And the 40 µm wide central site immediately adjacent to the implant surface contains a lower volume of collagen and a higher density of elongated fibroblasts but no blood vessels (Buser et al., 1992). The connective tissue under the JE contains collagen I, III, IV, and VII. Collagen I is the main component of the apical connective tissue of the peri-implant mucosa, and collagen V is more abundant in the peri-implant tissue (Chavrier and Couble, 1999). The collagen fibers of the connective tissue are parallel to the long axis of the implant, thus creating only a physical adaptation without any biological integration/insertion and having less adhesion (Chavrier et al., 1994). In addition, the limited number of gingival fibroblasts around the implant may prolong the healing, regeneration and maturation cycle of the connective tissue, allowing bacterial invasion (Al Rezk et al., 2018). As the main cellular component of the connective tissue layer surrounding the implant, gingival fibroblasts are responsible for the secretion and remodeling of the extracellular matrix (ECM), especially collagen fibers. After surgery, gingival fibroblasts begin proliferating, repopulating, and producing collagen-rich ECM in the submucosal region attached to the implant surface (Romanos et al., 1995). Collagen fibril formation continues for approximately 4–6 weeks, followed by forming a mature connective tissue seal between 6 and 12 weeks (Fujii et al., 1998; Al Rezk et al., 2018). In conclusion, the low number of gingival fibroblasts results in poor integration of the transmucosal connective tissue layer on the implanted dental abutment due to the lack of fibrous connections to the abutment surface.
Gingival fibroblasts adhere to the implant surface mainly through adhesive plaque attachment, tight junctions and ECM attachment. The adhesion patch complex consists of proteins communicating with the ECM, the transmembrane protein integrins, and the intracellular cytoskeleton (Burridge et al., 1988). The seven signs, represented by talin, paxillin, and vinculin, regulate gene expression to stabilize the adhesion complex through a feedback system and transmit different signals from the ECM to the cytosol (Hynes, 2002; Owen et al., 2005). To obtain enhanced connective tissue integration, upregulation of fibroblast activity and enhanced expression of integrins α5, β1, and β3 are required to ensure the early establishment of direct fibrous connections.
The firm attachment of soft tissues to the implant (as shown in Figure 1), which ultimately allows complete closure of the subgingival bone tissue and protection from the oral environment, promotes implant stability and is the most critical factor influencing the long-term maintenance of dental implants (An et al., 2012). Studies are scarce to quantify the strength of cell-implant adhesion and evaluate the role of attachment proteins. In conclusion, soft tissue closure as a biological barrier is vital in remodeling soft and hard tissues around implants and the long-term maintenance of clinical implants.
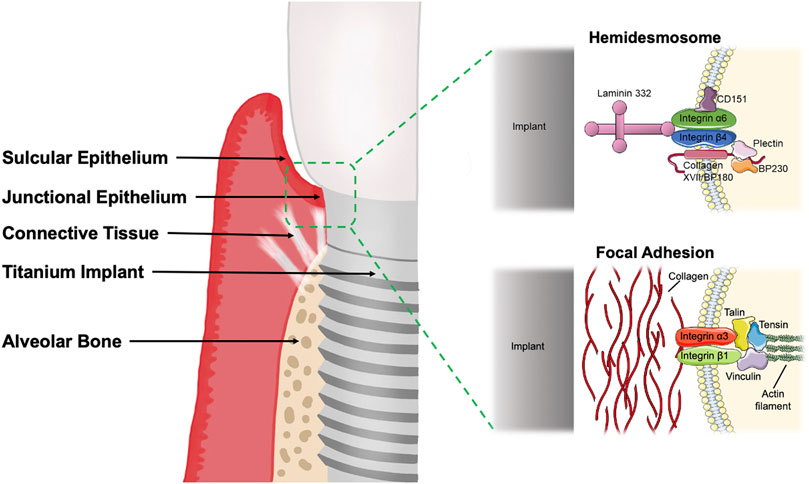
FIGURE 1. The soft tissue seal around the implant consists of gingival junctional epithelium and connective tissue. The peri-implant junctional epithelium adheres to the implant mainly through hemidesmosome structures, while the gingival fibroblasts exert adhesion mainly through focal adhesions. The figure was partly generated using Servier Medical Art, provided by Servier, licensed under a Creative Commons Attribution 3.0 unported license. BP180, Bullous pemphigoid antigen 180; BP230, Bullous pemphigoid antigen 230.
4 The mechanisms of diabetes leading to impaired soft tissue seal
4.1 Subgingival microbiome and bacterial susceptibility affected by diabetes
Studies have found an increase in Actinomyces naeslundi and Streptococcus oralis around implants in healthy patients compared to natural teeth (Gürlek et al., 2017). There are still inconsistent and even contradictory conclusions about whether diabetes alters the bacterial composition of the oral cavity (Chapple et al., 2013; Graves et al., 2020). In type 2 diabetic periodontitis, the detection rate of orange complex species in subgingival plaque was significantly higher compared to a healthy periodontal state (Sabancı et al., 2022). A 16s rRNA assay of the subgingival flora of patients with periodontitis showed a significant augment in the relative abundance of Aggregatibacter, Neisseria, Fusobacterium, and Actinomycetes in type 2 diabetic patients (Casarin et al., 2013). Another report indicated that the levels of Porphyromonas gingivalis (P. gingivalis) or Porphyromonas forsythia (P. forsythia) showed an increase (da Cruz et al., 2008). Furthermore, a recent metagenomic shotgun sequencing analysis comparing the subgingival flora of non-diabetic and diabetic patients found that subgingival flora tended to be more abundant in pathogenic species in the T2DM state, regardless of whether the periodontal tissue was healthy, suggesting that T2DM patients face a higher risk of developing periodontitis (Shi et al., 2020).
Similarly, diabetes may also affect peri-implant inflammation by altering the subgingival flora. One study divided patients suffering from peri-implantitis into a systemic health group and a T2DM group (Sabancı et al., 2022). There are no significant differences in microbial species were found in the shallow peri-implant pockets of the two groups (Sabancı et al., 2022). However, Campylobacter rectus (C. rectus), P. gingivalis, Actinobacillus actinomycetemcomitans (A. actinomycetemcomitans) and Tannerella forsythia (T. forsythia) may be reduced in deep pockets around implants by T2DM (Sabancı et al., 2022). An interesting study to identify the microbiota in peri-implantitis pockets by matrix-assisted laser desorption/ionization time-of-flight mass spectrometry showed Neisseria flavescens, Streptococcus constellatus, Slackia exigua, Streptococcus intermedius, Fusobacterium nucleatum, and Gemella morbillorum were the central resident folk detected in the peri-implantitis pockets (Yeh et al., 2019). The above findings suggest that the sequencing identification of bacteria alone may not determine whether they are pathogenic in patients with type 2 diabetes. Non-etheless, the diabetic state produces changes in some microbial species in the peri-implant pocket, and these changes may affect microbial homeostasis, but the exact effects are inconclusive.
Since the discovery of bacterial binding to ECM proteins (e.g., collagen, fibronectin, and laminin) more than 40 years ago (Kuusela, 1978), our understanding of pathogen-host cell interactions has gradually enhanced. The adhesion of bacteria to host tissues is the first decisive step in the infection process (Keller et al., 2015). In Gram-negative bacteria, adhesion to host cells can be achieved by binding to ECM proteins as a tool for pathogen-host contact (Vaca et al., 2020). Even pathogens secrete bacterial proteases to degrade ECM proteins and thus disrupt the barrier (Singh et al., 2012). For example, Treponema denticola produces chymotrypsin-like proteases targeted to degrade Laminin, collagen IV, and fibrinogen, allowing bacteria to invade the basement membrane after ECM degradation (Bamford et al., 2007; Singh et al., 2012). In the oral cavity, JE can express defensins, chemokines and cytokines (Groeger and Meyle, 2019). When bacteria colonize JE, the adhesion of keratinocytes is negatively affected, and laminin and collagen present in JE become binding sites for pathogens (Marre et al., 2020). In conclusion, the difference between hyperglycemia and normoglycemia is mainly in the deep pockets around the implants (Sabancı et al., 2022), and diabetic patients are more susceptible to microbiome changes than normal healthy individuals (Sabancı et al., 2022).
4.2 Increased immuno-inflammatory response in diabetes
Diabetes increases the physical inflammatory response (Andriankaja et al., 2012; Pacios et al., 2012). Diabetic patients have increased tumor necrosis factor-α (TNF-α), elevated polymorphonuclear leukocyte infiltration, and more bone loss in diabetic rats than in normoglycemic rats (Kang et al., 2012). Injection of equal amounts of bacteria into the soft tissues of diabetic animals caused a more severe inflammatory response than in normal animals (Naguib et al., 2004). These results suggest that diabetic animals have a more dramatic host response to bacterial attacks.
Diabetes augments levels of inflammatory cytokines such as TNF-α, interleukin-1β (IL-1β), interleukin-17 (IL-17), interleukin-23 (IL-23), and interleukin-6 (IL-6) in human periodontal tissue (Bastos et al., 2012; Polak and Shapira, 2018). Increased resistance and permeability of the gingival epithelial cell layer after TNF-α and IL-1β treatment is associated with the development of gingival inflammation (Fujita et al., 2012; Miyagawa et al., 2016; Lagha and Grenier, 2019). Moreover, human gingival fibroblasts (HGF) treated with TNF-α and IL-1β induced increased HGF infiltration (Lv et al., 2020). Enhanced expression of inflammatory cytokines leads to rose vascular permeability and inflammatory cell recruitment (Domingueti et al., 2016) with upregulated receptor activator of nuclear factor kappa-B ligand (RANKL) or decreased osteoprotegerin (OPG) expression, stimulating increased bone resorption (Bastos et al., 2012). The more severe the hyperglycemia, the higher the levels of IL-1β and IL-6 in the oral saliva and the greater the probability of peri-implantitis (Al-Askar et al., 2018; Al-Sowygh et al., 2018; Vissink et al., 2018). And the restraint in anti-inflammatory factors may be one of the reasons for the exacerbation of periodontitis. Regulatory T-cells and M2-type macrophages responsible for anti-inflammation produce anti-inflammatory factors such as interleukin-4 (IL-4), interleukin-10 (IL-10), transforming growth factor-β (TGF-β) and anti-inflammatory lipid mediators, significantly reduced in diabetic complications (Acharya et al., 2017; Van Dyke, 2017).
Diabetes also affects the innate and adaptive immune responses of cells. Neutrophils make up the majority of cells in the gingival sulcus and are an essential component of the host response to tooth-associated biofilms (Hajishengallis, 2014). High glucose stimulates the production of more chemokines, which induce neutrophil recruitment in response to bacterial challenges (Cintra et al., 2014; Manosudprasit et al., 2017; Zheng et al., 2018b), and stimulates neutrophil initiation by increasing protein kinase C (PKC) activity (Karima et al., 2005). In addition, diabetes augments neutrophil activation and reactive oxygen species (ROS) production to increase damage to periodontal tissue (Guerra and Otton, 2011). Macrophages are associated with peri-implant disease. Diabetes promotes the production of IL-1β and TNF-α by macrophages, which may contribute to the enhancement of peri-implant infection (Salvi et al., 1998). Diabetes may increase the polarization of M1 macrophages to upregulate the susceptibility and severity of peri-implantitis (Sreedhar et al., 2017). In addition, higher levels of TNF-α, CC chemokine receptor 5 (CCR5) and CXC chemokine receptor 3 (CXCR3) at peri-implant sites in patients with chronic periodontitis and diabetes suggest a high potential for peri-implant bone loss (Venza et al., 2010). Dendritic cells are also associated with peri-implant disease, and their function may be regulated by diabetes, thereby promoting the disease process. Diabetes may control dendritic cells to alter alveolar bone loss by increasing the production of Th1 or Th17 lymphocytes or decreasing the formation of regulatory T-cells (Santos et al., 2010; Silva et al., 2012; Song et al., 2018).
A fundamental cause of the pathogenesis of peri-implantitis is how diabetes affects the soft tissue barrier of the implant, but it has not received sufficient attention. The sealing structure formed by the soft tissues is an effective barrier to protect the implant from external microorganisms, but the corresponding specific mechanisms remain to be thoroughly investigated.
4.3 High-level matrix metalloproteinases in diabetes
Matrix Metalloproteinases (MMPs) are a group of protein hydrolases that play an essential role in the turnover of ECM and maintain the balance between remodeling and degradation of ECM (Klein and Bischoff, 2011; Bastos et al., 2017). The substrate of MMP is mainly collagen, but it also includes many other ECM proteins, including fibronectin, laminin, etc. (Ruoslahti, 1996; Stamenkovic, 2003). Regarding the mechanism of neurological damage by cerebral ischemia, MMP-9 destroys neurons by degrading laminin (Lee et al., 2009). MMPs are associated with diabetes-related peri-implantitis. MMP-8 and MMP-9 levels in oral fluids can reflect the disease status of periodontal disease to some extent (Domenyuk et al., 2019). In particular, the level of MMP-8 in oral fluid is upregulated proportionally to the severity of periodontal/peri-implant disease (Sorsa et al., 2000; Kiili et al., 2002; Sorsa et al., 2010; Sexton et al., 2011; Sorsa et al., 2011). MMP-8 in saliva was similar in composition to its counterpart within the gingival sulcus (Gangbar et al., 1990), and a similar pattern of elevated MMP-8 was observed in the gingival sulcus of peri-implantitis to that observed at the site of periodontitis (Ma et al., 2000; Arakawa et al., 2012; Janska et al., 2016). A study reported that patients with both chronic periodontitis and diabetes had much higher levels of MMP-8 and MMP-9 in periodontal tissue compared to patients with chronic periodontitis but systemically healthy patients and healthy controls (Kumar et al., 2006), and there was a trend toward significantly increased MMP-8 in patients transitioning from the absence of both diseases (Hardy et al., 2012). In addition, the excessive release of various MMPs by fibroblasts affected by reactive oxygen species promoted the degradation of connective tissue and bone matrix (Guan et al., 2009; Kaur et al., 2013). The interaction of MMPs with advanced glycosylation end-products (AGEs)/receptors for advanced glycosylation end-products (RAGEs) further contributes to increased inflammation in diabetes. AGEs accumulate and destroy periodontal tissue continuously under hyperglycemic conditions and directly or indirectly mediate intracellular effects through RAGE receptors of epithelial cells, gingival fibroblasts and other cells (Plemmenos and Piperi, 2022). The combination of AGEs and RAGEs in specific cell lines can stimulate the production and activity of MMPs, making the inflammatory response more severe (Ryan et al., 1999; Nesto and Rutter, 2002). AGEs can also influence the production and structure of ECM proteins by affecting the cross-linking of collagen (Snedeker and Gautieri, 2014).
4.4 Wound healing delayed by diabetes
Both oral mucosal and skin wound healing requires four stages: Hemostasis, inflammation, proliferation, and maturation/stromal remodeling. Soft tissue healing after implant placement surgery takes 6–8 weeks, which is much longer than the epithelial healing time for dental surgical wounds (7–14 days) (Salvi et al., 2000; Hämmerle et al., 2014). Wound healing involves re-epithelialization, massive new connective tissue formation and new bone formation (Ko et al., 2021). The diabetic oral wound healing process is characterized by impaired keratinocyte proliferation and migration, altered levels of inflammation, and reduced neo-connective tissue and bone formation (Ko et al., 2021). The factors involved in delayed wound healing in diabetes include hypoxia, fibroblast and epidermal cell dysfunction, impaired angiogenesis, and neovascularization, elevated MMPs, damage from ROS and advanced glycosylation end-products (AGEs), neuropathy and decreased multilevel host immune resistance (Lan et al., 2008; Chrcanovic et al., 2014; Brizeno et al., 2016). Most of these conditions are exacerbated in diabetes mellitus with poor glycemic control and significantly increase the risk of poor wound healing in dental procedures. An in vitro study confirmed that hyperglycemia significantly inhibits the adhesion and proliferation of human gingival fibroblasts on titanium (Liu et al., 2021). Diabetic wounds have more neutrophils, enhanced TNF expression levels, and declined expression of growth factors in wound fluid, which may delay wound re-epithelialization (Siqueira et al., 2010; Romanos et al., 2019). In cutaneous wounds, diabetes reduced the switch from the M1 macrophage phenotype to the M2 macrophage phenotype (Al-Mulla et al., 2011). More pro-inflammatory macrophages implied increased expression of inflammatory cytokines (e.g., IL-1, IL-6, and IL-8) and MMP, which may lead to more prolonged inflammation (Wilkinson et al., 2019). Genes involved in apoptosis, mucosal migration and intercellular communication were reported to be upregulated 4-fold–200-fold when inflammation around the implant increased, which simultaneously slowed the rate of wound healing (Ganesan et al., 2022).
4.5 Excess reactive oxygen species in diabetes
Chronic exposure to high glucose induces cytokine expression and responses to cytokine stimuli in diabetic patients, including the production of ROS (Lim et al., 2017; Zheng et al., 2018b). ROS are a series of molecular oxygen derivatives with pleiotropic properties, classified into radical ROS (e.g., hydroxyl radicals, superoxide anion radicals O2−, peroxyl radicals, and alkoxyl radicals) and non-radical ROS (e.g., H2O2, organic hydroperoxides, single linear state molecular oxygen, ozone, hypochlorous acid, and hypobromous acid) (Forrester et al., 2018; Hawkins and Davies, 2019; Lushchak and Lushchak, 2021). Physiological levels of ROS regulate various life processes in cells and organs, including cell proliferation, differentiation, migration, and angiogenesis (Niki, 2016). However, when ROS levels exceed physiological concentrations, it will lead to cell growth arrest and death (Niki, 2016; Li et al., 2021). Due to high intracellular glucose levels, the mitochondrial electron transport chain is overactive, inducing the formation of reactive oxygen species and producing and releasing inflammatory factors. Reactive oxygen species can induce apoptosis and damage cellular and stromal DNA and structural components (Yang et al., 2015). Increased mitochondrial reactive oxygen species production exacerbates periodontitis in diabetic patients (Sun et al., 2017).
4.6 Elevated advanced glycation end products in diabetes
High glucose levels can lead to the formation of advanced glycosylation end products (AGEs). AGEs accumulate in most tissues of diabetic patients, including kidney, retina, gums, bone and periodontal tissue (Schmidt et al., 1996; Lalla and Papapanou, 2011; Napoli et al., 2017). AGEs bind to receptors for advanced glycosylation end products (RAGEs) and other receptors, activate nuclear factor κB (NF-κB), stimulate the production of reactive oxygen species, and induce the expression of inflammatory cytokines such as IL-6 and TNF-α (Singh et al., 2014; Nonaka et al., 2018). AGEs may inhibit the viability of human gingival fibroblasts and reduce the expression of type I and type III collagen (Ren et al., 2009). Interestingly, one study observed an increased concentration of AGEs in the fluid around the implant in the diabetic group and a significant correlation with the depth of the pocket around the implant (Al-Sowygh et al., 2018). In osteoblasts, excess AGEs promote apoptosis and inhibit osteogenic activity (Alikhani et al., 2007). Inhibition of RAGE reduced TNF-α production, which confirms the role of RAGE receptors in slowing the development of periodontitis (Lalla et al., 2003). A report on periodontitis and systemic disease found that combining AGEs and RAGEs leads to excessive inflammatory response and periodontal tissue destruction in patients with type 2 diabetes (Chapple et al., 2013). The effects of diabetes on dental implants’ soft tissue sealing are shown as follows (Figure 2).
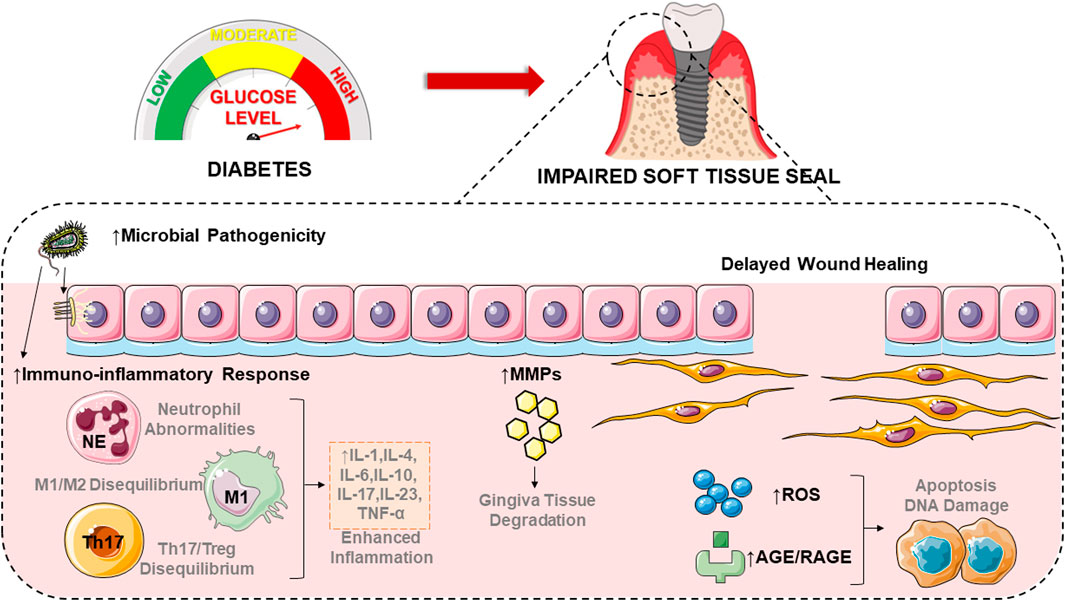
FIGURE 2. Diabetes affects the soft tissues around implants through a variety of mechanisms, including altering bacterial colony composition, promoting inflammatory responses, elevating matrix metalloproteinase concentrations, delaying the rate of wound healing, and increasing oxidative stress. The figure was partly generated using Servier Medical Art, provided by Servier, licensed under a Creative Commons Attribution 3.0 unported license. IL-1, interleukin-1; IL-4, interleukin-4; IL-6, interleukin-6; IL-10, interleukin-10; IL-17, interleukin-17; IL-23, interleukin-23; TNF-α, tumor necrosis factor-α; MMPs, matrix metalloproteinases; ROS, reactive oxygen species; AGE, advanced glycosylation end-product; RAGE, advanced glycosylation end product.
5 Clinical implications and future perspectives
The soft tissue around the transmural part of the dental implant is remodeled so that the bone tissue around the implant is separated from the oral cavity, creating a biological width that serves as a defense mechanism against bacteria, such as soft tissue seals. This closure prevents inflammatory diseases around the implant and ensures a healthy condition and stable osseointegration. The damaging effect of diabetes, one of the risk factors for peri-implantitis, on peri-implant soft tissue seal may be reflected in the altered composition of the subgingival microbiome, a more intense host inflammatory response, impaired wound healing processes, and an excess of reactive oxygen species (ROS) and advanced glycosylation end products (AGEs). More importantly, excessive inflammatory factors and matrix metalloproteinases (MMPs) production promote the ablation of the extracellular matrix (ECM), which to some extent hinders the adhesion of epithelial and fibrous tissues to the implant surface achieved based on ECM structures such as hemidesmosomes and focal adhesion. However, there are no targeted methods to resist the development of peri-implantitis in diabetic patients, especially by preserving the ECM structures. Therefore, remodeling the adhesion structures of the peri-implant soft tissues is seen as a more effective potential direction. Understanding the effects of diabetes on gingival epithelial cells and gingival fibroblasts and thoroughly exploring the mechanisms involved are essential for developing and improving therapeutic approaches, which are suitable for establishing and maintaining soft tissue seals for the prevention of peri-implantitis and the longevity of successfully osseointegrated implants in diabetic patients. Reduced strength and time delays in soft tissue integration can affect the long-term stability of implant dentures. Researchers have never stopped searching for ways to improve the success of dental implants in diabetic patients, especially by focusing their studies on resisting the development and progression of diabetic peri-implantitis, and focusing on the improvement of soft tissue sealing is necessary to improve outcomes.
Author contributions
ZZ, CJ, and XX contributed to the conception and design of the study. ZZ organized the database. CJ performed the statistical analysis. ZZ, CJ, and MW wrote the first draft of the manuscript. DW, DS, and DZ wrote sections of the manuscript. XX and DZ critically reviewed it for important intellectual content. All authors contributed to the manuscript revision, read, and approved the submitted version.
Funding
This research was funded by the National Natural Science Foundation of China, grant no. 82071148; the Natural Science Foundation of Shandong Province, grant no. ZR2021MH092; and the Natural Science Foundation of Jiangsu Province (Suzhou Research Institute of Shandong University), grant no. BK20210111.
Conflict of interest
The authors declare that the research was conducted in the absence of any commercial or financial relationships that could be construed as a potential conflict of interest.
Publisher’s note
All claims expressed in this article are solely those of the authors and do not necessarily represent those of their affiliated organizations, or those of the publisher, the editors and the reviewers. Any product that may be evaluated in this article, or claim that may be made by its manufacturer, is not guaranteed or endorsed by the publisher.
References
Abdallah M. N., Badran Z., Ciobanu O., Hamdan N., Tamimi F. (2017). Strategies for optimizing the soft tissue seal around osseointegrated implants. Adv. Healthc. Mater 6 (20), 1700549. doi:10.1002/adhm.201700549
Acharya A. B., Thakur S., Muddapur M. V., Kulkarni R. D. (2017). Cytokine ratios in chronic periodontitis and type 2 diabetes mellitus. Diabetes Metab. Syndr. 11 (4), 277–278. doi:10.1016/j.dsx.2016.12.007
Aguilar-Salvatierra A., Calvo-Guirado J. L., González-Jaranay M., Moreu G., Delgado-Ruiz R. A., Gómez-Moreno G. (2016). Peri-implant evaluation of immediately loaded implants placed in esthetic zone in patients with diabetes mellitus type 2: A two-year study. Clin. Oral Implants Res. 27 (2), 156–161. doi:10.1111/clr.12552
Al Rezk F., Trimpou G., Lauer H. C., Weigl P., Krockow N. (2018). Response of soft tissue to different abutment materials with different surface topographies: A review of the literature. Gen. Dent. 66 (1), 18–25.
Al-Askar M., Ajlan S., Alomar N., Al-Daghri N. M. (2018). Clinical and radiographic peri-implant parameters and whole salivary interleukin-1β and interleukin-6 levels among type-2 diabetic and nondiabetic patients with and without peri-implantitis. Med. Princ. Pract. 27 (2), 133–138. doi:10.1159/000488032
Al-Mulla F., Leibovich S. J., Francis I. M., Bitar M. S. (2011). Impaired tgf-Β signaling and a defect in resolution of inflammation contribute to delayed wound healing in a female rat model of type 2 diabetes. Mol. Biosyst. 7 (11), 3006–3020. doi:10.1039/c0mb00317d
Al-Sowygh Z. H., Ghani S. M. A., Sergis K., Vohra F., Akram Z. (2018). Peri-implant conditions and levels of advanced glycation end products among patients with different glycemic control. Clin. Implant Dent. Relat. Res. 20 (3), 345–351. doi:10.1111/cid.12584
Alikhani M., Alikhani Z., Boyd C., MacLellan C. M., Raptis M., Liu R., et al. (2007). Advanced glycation end products stimulate osteoblast apoptosis via the map kinase and cytosolic apoptotic pathways. Bone 40 (2), 345–353. doi:10.1016/j.bone.2006.09.011
Alrabiah M., Al-Aali K. A., Al-Sowygh Z. H., Binmahfooz A. M., Mokeem S. A., Abduljabbar T. (2018). Association of advanced glycation end products with peri-implant inflammation in prediabetes and type 2 diabetes mellitus patients. Clin. Implant Dent. Relat. Res. 20 (4), 535–540. doi:10.1111/cid.12607
Amberg R., Elad A., Rothamel D., Fienitz T., Szakacs G., Heilmann S., et al. (2018). Design of a migration assay for human gingival fibroblasts on biodegradable magnesium surfaces. Acta Biomater. 79, 158–167. doi:10.1016/j.actbio.2018.08.034
American Diabetes Association (2019). 2. Classification and diagnosis of diabetes: Standards of medical care in diabetes-2019. Diabetes Care 42 (1), S13–s28. doi:10.2337/dc19-S002
American Diabetes Association (2013). Diagnosis and classification of diabetes mellitus. Diabetes Care 36 (1), S67–S74. doi:10.2337/dc13-S067
An N., Rausch-fan X., Wieland M., Matejka M., Andrukhov O., Schedle A. (2012). Initial attachment, subsequent cell proliferation/viability and gene expression of epithelial cells related to attachment and wound healing in response to different titanium surfaces. Dent. Mater 28 (12), 1207–1214. doi:10.1016/j.dental.2012.08.007
Andriankaja O. M., Galicia J., Dong G., Xiao W., Alawi F., Graves D. T. (2012). Gene expression dynamics during diabetic periodontitis. J. Dent. Res. 91 (12), 1160–1165. doi:10.1177/0022034512465292
Arakawa H., Uehara J., Hara E. S., Sonoyama W., Kimura A., Kanyama M., et al. (2012). Matrix metalloproteinase-8 is the major potential collagenase in active peri-implantitis. J. Prosthodont Res. 56 (4), 249–255. doi:10.1016/j.jpor.2012.07.002
Atsuta I., Ayukawa Y., Furuhashi A., Yamaza T., Tsukiyama Y., Koyano K. (2013). Promotive effect of insulin-like growth factor-1 for epithelial sealing to titanium implants. J. Biomed. Mater Res. A 101 (10), 2896–2904. doi:10.1002/jbm.a.34608
Atsuta I., Ayukawa Y., Kondo R., Oshiro W., Matsuura Y., Furuhashi A., et al. (2016). Soft tissue sealing around dental implants based on histological interpretation. J. Prosthodont Res. 60 (1), 3–11. doi:10.1016/j.jpor.2015.07.001
Atsuta I., Yamaza T., Yoshinari M., Goto T., Kido M. A., Kagiya T., et al. (2005). Ultrastructural localization of laminin-5 (Gamma2 chain) in the rat peri-implant oral mucosa around a titanium-dental implant by immuno-electron microscopy. Biomaterials 26 (32), 6280–6287. doi:10.1016/j.biomaterials.2005.03.046
Atsuta I., Yamaza T., Yoshinari M., Mino S., Goto T., Kido M. A., et al. (2005). Changes in the distribution of laminin-5 during peri-implant epithelium formation after immediate titanium implantation in rats. Biomaterials 26 (14), 1751–1760. doi:10.1016/j.biomaterials.2004.05.033
Aumailley M., Bruckner-Tuderman L., Carter W. G., Deutzmann R., Edgar D., Ekblom P., et al. (2005). A simplified laminin nomenclature. Matrix Biol. 24 (5), 326–332. doi:10.1016/j.matbio.2005.05.006
Awad S. F., Al-Mawali A., Al-Lawati J. A., Morsi M., Critchley J. A., Abu-Raddad L. J. (2021). Forecasting the type 2 diabetes mellitus epidemic and the role of key risk factors in Oman up to 2050: Mathematical modeling analyses. J. Diabetes Investig. 12 (7), 1162–1174. doi:10.1111/jdi.13452
Bamford C. V., Fenno J. C., Jenkinson H. F., Dymock D. (2007). The chymotrypsin-like protease complex of Treponema denticola atcc 35405 mediates fibrinogen adherence and degradation. Infect. Immun. 75 (9), 4364–4372. doi:10.1128/iai.00258-07
Bartold P. M., Walsh L. J., Narayanan A. S. (2000). Molecular and cell Biology of the gingiva. Periodontol 24, 28–55. doi:10.1034/j.1600-0757.2000.2240103.x
Bastos A. S., Graves D. T., Loureiro A. P., Rossa Júnior C., Abdalla D. S., Faulin Tdo E., et al. (2012). Lipid peroxidation is associated with the severity of periodontal disease and local inflammatory markers in patients with type 2 diabetes. J. Clin. Endocrinol. Metab. 97 (8), E1353–E1362. doi:10.1210/jc.2011-3397
Bastos M. F., Tucci M. A., de Siqueira A., de Faveri M., Figueiredo L. C., Vallim P. C., et al. (2017). Diabetes may affect the expression of matrix metalloproteinases and their inhibitors more than smoking in chronic periodontitis. J. Periodontal Res. 52 (2), 292–299. doi:10.1111/jre.12394
Battle-Siatita S. O., Bartoloni J. A., Hancock R. H., Chong C. H. (2009). Retrospective analysis of dental implants among United States air force basic military trainees. Mil. Med. 174 (4), 437–440. doi:10.7205/milmed-d-02-5008
Berglundh T., Lindhe J. (1996). Dimension of the periimplant mucosa. Biological width revisited. J. Clin. Periodontol. 23 (10), 971–973. doi:10.1111/j.1600-051x.1996.tb00520.x
Brånemark P. I., Adell R., Breine U., Hansson B. O., Lindström J., Ohlsson A. (1969). Intra-osseous anchorage of dental prostheses. I. Experimental studies. Scand. J. Plast. Reconstr. Surg. 3 (2), 81–100. doi:10.3109/02844316909036699
Brizeno L. A., Assreuy A. M., Alves A. P., Sousa F. B., de B. S. P. G., de Sousa S. C., et al. (2016). Delayed healing of oral mucosa in a diabetic rat model: Implication of tnf-Α, il-1β and fgf-2. Life Sci. 155, 36–47. doi:10.1016/j.lfs.2016.04.033
Burridge K., Fath K., Kelly T., Nuckolls G., Turner C. (1988). Focal adhesions: Transmembrane junctions between the extracellular matrix and the cytoskeleton. Annu. Rev. Cell. Biol. 4, 487–525. doi:10.1146/annurev.cb.04.110188.002415
Buser D., Brägger U. (1989). Two-Part Iti-Hollow cylinder and hollow screw implant. Phillip J. 6 (5), 263–274.
Buser D., Sennerby L., De Bruyn H. (2017). Modern implant dentistry based on osseointegration: 50 years of progress, current trends and open questions. Periodontol 73 (1), 7–21. doi:10.1111/prd.12185
Buser D., Weber H. P., Donath K., Fiorellini J. P., Paquette D. W., Williams R. C. (1992). Soft tissue reactions to non-submerged unloaded titanium implants in beagle dogs. J. Periodontol. 63 (3), 225–235. doi:10.1902/jop.1992.63.3.225
Casarin R. C., Barbagallo A., Meulman T., Santos V. R., Sallum E. A., Nociti F. H., et al. (2013). Subgingival biodiversity in subjects with uncontrolled type-2 diabetes and chronic periodontitis. J. Periodontal Res. 48 (1), 30–36. doi:10.1111/j.1600-0765.2012.01498.x
Chackartchi T., Romanos G. E., Sculean A. (2019). Soft tissue-related complications and management around dental implants. Periodontol 81 (1), 124–138. doi:10.1111/prd.12287
Chapple I. L., Genco R. and Working group 2 of the joint EFP/AAP workshop (2013). Diabetes and periodontal diseases: Consensus report of the joint efp/aap workshop on periodontitis and systemic diseases. J. Periodontol. 84 (4), S106–S112. doi:10.1902/jop.2013.1340011
Chavrier C., Couble M. L., Hartmann D. J. (1994). Qualitative study of collagenous and noncollagenous glycoproteins of the human healthy keratinized mucosa surrounding implants. Clin. Oral Implants Res. 5 (3), 117–124. doi:10.1034/j.1600-0501.1994.050301.x
Chavrier C. A., Couble M. L. (1999). Ultrastructural immunohistochemical study of interstitial collagenous components of the healthy human keratinized mucosa surrounding implants. Int. J. Oral Maxillofac. Implants 14 (1), 108–112.
Chrcanovic B. R., Albrektsson T., Wennerberg A. (2014). Diabetes and oral implant failure: A systematic review. J. Dent. Res. 93 (9), 859–867. doi:10.1177/0022034514538820
Cintra L. T., Samuel R. O., Azuma M. M., Ribeiro C. P., Narciso L. G., de Lima V. M., et al. (2014). Apical periodontitis and periodontal disease increase serum il-17 levels in normoglycemic and diabetic rats. Clin. Oral Investig. 18 (9), 2123–2128. doi:10.1007/s00784-014-1192-7
Coelho P. G., Pippenger B., Tovar N., Koopmans S. J., Plana N. M., Graves D. T., et al. (2018). Effect of obesity or metabolic syndrome and diabetes on osseointegration of dental implants in a miniature swine model: A pilot study. J. Oral Maxillofac. Surg. 76 (8), 1677–1687. doi:10.1016/j.joms.2018.02.021
da Cruz G. A., de Toledo S., Sallum E. A., Sallum A. W., Ambrosano G. M., de Cássia Orlandi Sardi J., et al. (2008). Clinical and laboratory evaluations of non-surgical periodontal treatment in subjects with diabetes mellitus. J. Periodontol. 79 (7), 1150–1157. doi:10.1902/jop.2008.070503
Daubert D. M., Weinstein B. F. (2019). Biofilm as a risk factor in implant treatment. Periodontol 81 (1), 29–40. doi:10.1111/prd.12280
Daubert D. M., Weinstein B. F., Bordin S., Leroux B. G., Flemming T. F. (2015). Prevalence and predictive factors for peri-implant disease and implant failure: A cross-sectional analysis. J. Periodontol. 86 (3), 337–347. doi:10.1902/jop.2014.140438
Davis T. L., Rabinovitz I., Futscher B. W., Schnölzer M., Burger F., Liu Y., et al. (2001). Identification of a novel structural variant of the alpha 6 integrin. J. Biol. Chem. 276 (28), 26099–26106. doi:10.1074/jbc.M102811200
Domenyuk D., Samedov F., Dmitrienko S., Anfinogenova O., Glizhova T., Lysan D., et al. (2019). Matrix metalloproteinases and their tissue inhibitors in the pathogenesis of periodontal diseases in type 1 diabetes mellitus. Arch. EUROMEDICA 9 (3), 81–90. doi:10.35630/2199-885X/2019/9/3.25
Domingueti C. P., Dusse L. M., Carvalho M., de Sousa L. P., Gomes K. B., Fernandes A. P. (2016). Diabetes mellitus: The linkage between oxidative stress, inflammation, hypercoagulability and vascular complications. J. Diabetes Complicat. 30 (4), 738–745. doi:10.1016/j.jdiacomp.2015.12.018
Dreyer H., Grischke J., Tiede C., Eberhard J., Schweitzer A., Toikkanen S. E., et al. (2018). Epidemiology and risk factors of peri-implantitis: A systematic review. J. Periodontal Res. 53 (5), 657–681. doi:10.1111/jre.12562
Ferreira S. D., Silva G. L., Cortelli J. R., Costa J. E., Costa F. O. (2006). Prevalence and risk variables for peri-implant disease in Brazilian subjects. J. Clin. Periodontol. 33 (12), 929–935. doi:10.1111/j.1600-051X.2006.01001.x
Fontao L., Geerts D., Kuikman I., Koster J., Kramer D., Sonnenberg A. (2001). The interaction of plectin with actin: Evidence for cross-linking of actin filaments by dimerization of the actin-binding domain of plectin. J. Cell. Sci. 114 (11), 2065–2076. doi:10.1242/jcs.114.11.2065
Forrester S. J., Kikuchi D. S., Hernandes M. S., Xu Q., Griendling K. K. (2018). Reactive oxygen species in metabolic and inflammatory signaling. Circ. Res. 122 (6), 877–902. doi:10.1161/circresaha.117.311401
Fujii N., Kusakari H., Maeda T. (1998). A histological study on tissue responses to titanium implantation in rat maxilla: The process of epithelial regeneration and bone reaction. J. Periodontol. 69 (4), 485–495. doi:10.1902/jop.1998.69.4.485
Fujita T., Yumoto H., Shiba H., Ouhara K., Miyagawa T., Nagahara T., et al. (2012). Irsogladine maleate regulates epithelial barrier function in tumor necrosis factor-Α-stimulated human gingival epithelial cells. J. Periodontal Res. 47 (1), 55–61. doi:10.1111/j.1600-0765.2011.01404.x
Ganesan S. M., Dabdoub S. M., Nagaraja H. N., Mariotti A. J., Ludden C. W., Kumar P. S. (2022). Biome-microbiome interactions in peri-implantitis: A pilot investigation. J. Periodontol. 93 (6), 814–823. doi:10.1002/jper.21-0423
Gangbar S., Overall C. M., McCulloch C. A., Sodek J. (1990). Identification of polymorphonuclear leukocyte collagenase and gelatinase activities in mouthrinse samples: Correlation with periodontal disease activity in adult and juvenile periodontitis. J. Periodontal Res. 25 (5), 257–267. doi:10.1111/j.1600-0765.1990.tb00914.x
Ghohestani R. F., Li K., Rousselle P., Uitto J. (2001). Molecular organization of the cutaneous basement membrane zone. Clin. Dermatol 19 (5), 551–562. doi:10.1016/s0738-081x(00)00175-9
Gould T. R., Westbury L., Brunette D. M. (1984). Ultrastructural study of the attachment of human gingiva to titanium in vivo. J. Prosthet. Dent. 52 (3), 418–420. doi:10.1016/0022-3913(84)90459-1
Graves D. T., Ding Z., Yang Y. (2020). The impact of diabetes on periodontal diseases. Periodontol 82 (1), 214–224. doi:10.1111/prd.12318
Groeger S., Meyle J. (2019). Oral mucosal epithelial cells. Front. Immunol. 10, 208. doi:10.3389/fimmu.2019.00208
Guan S. M., Shu L., Fu S. M., Liu B., Xu X. L., Wu J. Z. (2009). Prevotella intermedia upregulates mmp-1 and mmp-8 expression in human periodontal ligament cells. FEMS Microbiol. Lett. 299 (2), 214–222. doi:10.1111/j.1574-6968.2009.01748.x
Guerra B. A., Otton R. (2011). Impact of the carotenoid astaxanthin on phagocytic capacity and ros/rns production of human neutrophils treated with free fatty acids and high glucose. Int. Immunopharmacol. 11 (12), 2220–2226. doi:10.1016/j.intimp.2011.10.004
Gulati K., Moon H. J., Kumar P. T. S., Han P., Ivanovski S. (2020). Anodized anisotropic titanium surfaces for enhanced guidance of gingival fibroblasts. Mater Sci. Eng. C Mater Biol. Appl. 112, 110860. doi:10.1016/j.msec.2020.110860
Gürlek Ö., Gümüş P., Nile C. J., Lappin D. F., Buduneli N. (2017). Biomarkers and bacteria around implants and natural teeth in the same individuals. J. Periodontol. 88 (8), 752–761. doi:10.1902/jop.2017.160751
Hajishengallis G. (2014). Aging and its impact on innate immunity and inflammation: Implications for periodontitis. J. Oral Biosci. 56 (1), 30–37. doi:10.1016/j.job.2013.09.001
Hämmerle C. H., Giannobile W. V. and Working Group 1 of the European Workshop on Periodontology (2014). Biology of soft tissue wound healing and regeneration-consensus report of group 1 of the 10th European workshop on periodontology. J. Clin. Periodontol. 41 (15), S1–S5. doi:10.1111/jcpe.12221
Hardy D. C., Ross J. H., Schuyler C. A., Leite R. S., Slate E. H., Huang Y. (2012). Matrix metalloproteinase-8 expression in periodontal tissues surgically removed from diabetic and non-diabetic patients with periodontal disease. J. Clin. Periodontol. 39 (3), 249–255. doi:10.1111/j.1600-051X.2011.01788.x
Hartlev J., Kohberg P., Ahlmann S., Andersen N. T., Schou S., Isidor F. (2014). Patient satisfaction and esthetic outcome after immediate placement and provisionalization of single-tooth implants involving a definitive individual abutment. Clin. Oral Implants Res. 25 (11), 1245–1250. doi:10.1111/clr.12260
Hawkins C. L., Davies M. J. (2019). Detection, identification, and quantification of oxidative protein modifications. J. Biol. Chem. 294 (51), 19683–19708. doi:10.1074/jbc.REV119.006217
Holmes R. S., Rout U. K. (2011). Comparative studies of vertebrate beta integrin genes and proteins: Ancient genes in vertebrate evolution. Biomolecules 1 (1), 3–31. doi:10.3390/biom1010003
Hu C., Jia W. (2018). Diabetes in China: Epidemiology and genetic risk factors and their clinical utility in personalized medication. Diabetes 67 (1), 3–11. doi:10.2337/dbi17-0013
Hynes R. O. (2002). Integrins: Bidirectional, allosteric signaling machines. Cell. 110 (6), 673–687. doi:10.1016/s0092-8674(02)00971-6
Ikeda H., Yamaza T., Yoshinari M., Ohsaki Y., Ayukawa Y., Kido M. A., et al. (2000). Ultrastructural and immunoelectron microscopic studies of the peri-implant epithelium-implant (Ti-6al-4v) interface of rat maxilla. J. Periodontol. 71 (6), 961–973. doi:10.1902/jop.2000.71.6.961
Ivanovski S., Lee R. (2018). Comparison of peri-implant and periodontal marginal soft tissues in health and disease. Periodontology 76 (1), 116–130. doi:10.1111/prd.12150
Janska E., Mohr B., Wahl G. (2016). Correlation between peri-implant sulcular fluid rate and expression of Collagenase2 (Mmp8). Clin. Oral Investig. 20 (2), 261–266. doi:10.1007/s00784-015-1501-9
Jivraj S., Chee W. (2006). Rationale for dental implants. Br. Dent. J. 200 (12), 661–665. doi:10.1038/sj.bdj.4813718
Jofre J., Castiglioni X., Lobos C. A. (2013). Influence of minimally invasive implant-retained overdenture on patients' quality of life: A randomized clinical trial. Clin. Oral Implants Res. 24 (10), 1173–1177. doi:10.1111/j.1600-0501.2012.02529.x
Kang J., de Brito Bezerra B., Pacios S., Andriankaja O., Li Y., Tsiagbe V., et al. (2012). Aggregatibacter actinomycetemcomitans infection enhances apoptosis in vivo through a caspase-3-dependent mechanism in experimental periodontitis. Infect. Immun. 80 (6), 2247–2256. doi:10.1128/iai.06371-11
Karima M., Kantarci A., Ohira T., Hasturk H., Jones V. L., Nam B. H., et al. (2005). Enhanced superoxide release and elevated protein kinase C activity in neutrophils from diabetic patients: Association with periodontitis. J. Leukoc. Biol. 78 (4), 862–870. doi:10.1189/jlb.1004583
Kaur S., White S., Bartold P. M. (2013). Periodontal disease and rheumatoid arthritis: A systematic review. J. Dent. Res. 92 (5), 399–408. doi:10.1177/0022034513483142
Kazarov A. R., Yang X., Stipp C. S., Sehgal B., Hemler M. E. (2002). An extracellular site on tetraspanin Cd151 determines alpha 3 and alpha 6 integrin-dependent cellular morphology. J. Cell. Biol. 158 (7), 1299–1309. doi:10.1083/jcb.200204056
Keller B., Mühlenkamp M., Deuschle E., Siegfried A., Mössner S., Schade J., et al. (2015). Yersinia enterocolitica exploits different pathways to accomplish adhesion and toxin injection into host cells. Cell. Microbiol. 17 (8), 1179–1204. doi:10.1111/cmi.12429
Kiili M., Cox S. W., Chen H. Y., Wahlgren J., Maisi P., Eley B. M., et al. (2002). Collagenase-2 (Mmp-8) and collagenase-3 (Mmp-13) in adult periodontitis: Molecular forms and levels in gingival crevicular fluid and immunolocalisation in gingival tissue. J. Clin. Periodontol. 29 (3), 224–232. doi:10.1034/j.1600-051x.2002.290308.x
Klein T., Bischoff R. (2011). Physiology and pathophysiology of matrix metalloproteases. Amino Acids 41 (2), 271–290. doi:10.1007/s00726-010-0689-x
Ko K. I., Sculean A., Graves D. T. (2021). Diabetic wound healing in soft and hard oral tissues. Transl. Res. 236, 72–86. doi:10.1016/j.trsl.2021.05.001
Koidou V. P., Argyris P. P., Skoe E. P., Mota Siqueira J., Chen X., Zhang L., et al. (2018). Peptide coatings enhance keratinocyte attachment towards improving the peri-implant mucosal seal. Biomater. Sci. 6 (7), 1936–1945. doi:10.1039/c8bm00300a
Koster J., van Wilpe S., Kuikman I., Litjens S. H., Sonnenberg A. (2004). Role of binding of plectin to the integrin Beta4 subunit in the assembly of hemidesmosomes. Mol. Biol. Cell. 15 (3), 1211–1223. doi:10.1091/mbc.e03-09-0697
Kumar M. S., Vamsi G., Sripriya R., Sehgal P. K. (2006). Expression of matrix metalloproteinases (Mmp-8 and -9) in chronic periodontitis patients with and without diabetes mellitus. J. Periodontol. 77 (11), 1803–1808. doi:10.1902/jop.2006.050293
Kuusela P. (1978). Fibronectin binds to Staphylococcus aureus. Nature 276 (5689), 718–720. doi:10.1038/276718a0
Kwon T., Wang C. W., Salem D. M., Levin L. (2020). Nonsurgical and surgical management of biologic complications around dental implants: Peri-implant mucositis and peri-implantitis. Quintessence Int. 51 (10), 810–820. doi:10.3290/j.qi.a44813
Lagha A. B., Grenier D. (2019). Tea polyphenols protect gingival keratinocytes against tnf-Α-induced tight junction barrier dysfunction and attenuate the inflammatory response of monocytes/macrophages. Cytokine 115, 64–75. doi:10.1016/j.cyto.2018.12.009
Lalla E., Lamster I. B., Hofmann M. A., Bucciarelli L., Jerud A. P., Tucker S., et al. (2003). Oral infection with a periodontal pathogen accelerates early atherosclerosis in apolipoprotein E-null mice. Arterioscler. Thromb. Vasc. Biol. 23 (8), 1405–1411. doi:10.1161/01.Atv.0000082462.26258.Fe
Lalla E., Papapanou P. N. (2011). Diabetes mellitus and periodontitis: A tale of two common interrelated diseases. Nat. Rev. Endocrinol. 7 (12), 738–748. doi:10.1038/nrendo.2011.106
Lan C. C., Liu I. H., Fang A. H., Wen C. H., Wu C. S. (2008). Hyperglycaemic conditions decrease cultured keratinocyte mobility: Implications for impaired wound healing in patients with diabetes. Br. J. Dermatol 159 (5), 1103–1115. doi:10.1111/j.1365-2133.2008.08789.x
LeBleu V. S., Macdonald B., Kalluri R. (2007). Structure and function of basement membranes. Exp. Biol. Med. (Maywood) 232 (9), 1121–1129. doi:10.3181/0703-mr-72
Lee H., Park J. W., Kim S. P., Lo E. H., Lee S. R. (2009). Doxycycline inhibits matrix metalloproteinase-9 and laminin degradation after transient global cerebral ischemia. Neurobiol. Dis. 34 (2), 189–198. doi:10.1016/j.nbd.2008.12.012
Li D., Ding Z., Du K., Ye X., Cheng S. (2021). Reactive oxygen species as a link between antioxidant pathways and autophagy. Oxid. Med. Cell. Longev. 2021, 5583215. doi:10.1155/2021/5583215
Lim J. C., Ko K. I., Mattos M., Fang M., Zhang C., Feinberg D., et al. (2017). Tnfα contributes to diabetes impaired angiogenesis in fracture healing. Bone 99, 26–38. doi:10.1016/j.bone.2017.02.014
Liu Y. Y., Lu R. J., Guo B., Gao L. L., Zhang N., Chang W. X. (2021). The effects of hyperglycemia on the biological behavior of human gingival fibroblasts on a titanium surface. Ann. Palliat. Med. 10 (9), 9685–9691. doi:10.21037/apm-21-2277
Lushchak V. I., Lushchak O. (2021). Interplay between reactive oxygen and nitrogen species in living organisms. Chem. Biol. Interact. 349, 109680. doi:10.1016/j.cbi.2021.109680
Lv J., Liu Y., Jia S., Zhang Y., Tian H., Li J., et al. (2020). Carbon monoxide-releasing molecule-3 suppresses tumor necrosis factor-Α- and interleukin-1β-induced expression of junctional molecules on human gingival fibroblasts via the heme oxygenase-1 pathway. Mediat. Inflamm. 2020, 6302391. doi:10.1155/2020/6302391
Ma J., Kitti U., Teronen O., Sorsa T., Husa V., Laine P., et al. (2000). Collagenases in different categories of peri-implant vertical bone loss. J. Dent. Res. 79 (11), 1870–1873. doi:10.1177/00220345000790110901
Manosudprasit A., Kantarci A., Hasturk H., Stephens D., Van Dyke T. E. (2017). Spontaneous pmn apoptosis in type 2 diabetes and the impact of periodontitis. J. Leukoc. Biol. 102 (6), 1431–1440. doi:10.1189/jlb.4A0416-209RR
Marre A. T. O., Domingues R., Lobo L. A. (2020). Adhesion of anaerobic periodontal pathogens to extracellular matrix proteins. Braz J. Microbiol. 51 (4), 1483–1491. doi:10.1007/s42770-020-00312-2
Matarazzo F., Sabóia-Gomes R., Alves B. E. S., de Oliveira R. P., Araújo M. G. (2018). Prevalence, extent and severity of peri-implant diseases. A cross-sectional study based on a university setting in Brazil. J. Periodontal Res. 53 (5), 910–915. doi:10.1111/jre.12582
Miyagawa T., Fujita T., Yumoto H., Yoshimoto T., Kajiya M., Ouhara K., et al. (2016). Azithromycin recovers reductions in barrier function in human gingival epithelial cells stimulated with tumor necrosis factor-Α. Arch. Oral Biol. 62, 64–69. doi:10.1016/j.archoralbio.2015.11.015
Monje A., Catena A., Borgnakke W. S. (2017). Association between diabetes mellitus/hyperglycaemia and peri-implant diseases: Systematic review and meta-analysis. J. Clin. Periodontol. 44 (6), 636–648. doi:10.1111/jcpe.12724
Moon I. S., Berglundh T., Abrahamsson I., Linder E., Lindhe J. (1999). The barrier between the keratinized mucosa and the dental implant. An experimental study in the dog. J. Clin. Periodontol. 26 (10), 658–663. doi:10.1034/j.1600-051x.1999.261005.x
Moy P. K., Medina D., Shetty V., Aghaloo T. L. (2005). Dental implant failure rates and associated risk factors. Int. J. Oral Maxillofac. Implants 20 (4), 569–577.
Naguib G., Al-Mashat H., Desta T., Graves D. T. (2004). Diabetes prolongs the inflammatory response to a bacterial stimulus through cytokine dysregulation. J. Invest. Dermatol 123 (1), 87–92. doi:10.1111/j.0022-202X.2004.22711.x
Napoli N., Chandran M., Pierroz D. D., Abrahamsen B., Schwartz A. V., Ferrari S. L., et al. (2017). Mechanisms of diabetes mellitus-induced bone fragility. Nat. Rev. Endocrinol. 13 (4), 208–219. doi:10.1038/nrendo.2016.153
Nesto R. W., Rutter M. K. (2002). Impact of the atherosclerotic process in patients with diabetes. Acta Diabetol. 39 (2), S22–S28. doi:10.1007/s005920200022
Nibali L., Gkranias N., Mainas G., Di Pino A. (2022). Periodontitis and implant complications in diabetes. Periodontol 90 (1), 88–105. doi:10.1111/prd.12451
Niki E. (2016). Oxidative stress and antioxidants: Distress or eustress? Arch. Biochem. Biophys. 595, 19–24. doi:10.1016/j.abb.2015.11.017
Nonaka K., Kajiura Y., Bando M., Sakamoto E., Inagaki Y., Lew J. H., et al. (2018). Advanced glycation end-products increase il-6 and icam-1 expression via rage, mapk and nf-?b pathways in human gingival fibroblasts. J. Periodontal Res. 53 (3), 334–344. doi:10.1111/jre.12518
Owen G. R., Meredith D. O., ap Gwynn I., Richards R. G. (2005). Focal adhesion quantification - a new assay of material biocompatibility? Review. Eur. Cell. Mater 9, 85–96. doi:10.22203/ecm.v009a10
Pacios S., Kang J., Galicia J., Gluck K., Patel H., Ovaydi-Mandel A., et al. (2012). Diabetes aggravates periodontitis by limiting repair through enhanced inflammation. Faseb J. 26 (4), 1423–1430. doi:10.1096/fj.11-196279
Pendegrass C. J., Lancashire H. T., Fontaine C., Chan G., Hosseini P., Blunn G. W. (2015). Intraosseous transcutaneous amputation prostheses versus dental implants: A comparison between keratinocyte and gingival epithelial cell adhesion in vitro. Eur. Cell. Mater 29, 237–249. doi:10.22203/ecm.v029a18
Plemmenos G., Piperi C. (2022). Pathogenic molecular mechanisms in periodontitis and peri-implantitis: Role of advanced glycation end products. Life (Basel) 12 (2), 218. doi:10.3390/life12020218
Polak D., Shapira L. (2018). An update on the evidence for pathogenic mechanisms that may link periodontitis and diabetes. J. Clin. Periodontol. 45 (2), 150–166. doi:10.1111/jcpe.12803
Ren L., Fu Y., Deng Y., Qi L., Jin L. (2009). Advanced glycation end products inhibit the expression of collagens type I and iii by human gingival fibroblasts. J. Periodontol. 80 (7), 1166–1173. doi:10.1902/jop.2009.080669
Ren X., van der Mei H. C., Ren Y., Busscher H. J. (2019). Keratinocytes protect soft-tissue integration of dental implant materials against bacterial challenges in a 3d-tissue infection model. Acta Biomater. 96, 237–246. doi:10.1016/j.actbio.2019.07.015
Rezniczek G. A., de Pereda J. M., Reipert S., Wiche G. (1998). Linking integrin alpha6beta4-based cell adhesion to the intermediate filament cytoskeleton: Direct interaction between the Beta4 subunit and plectin at multiple molecular sites. J. Cell. Biol. 141 (1), 209–225. doi:10.1083/jcb.141.1.209
Rodrigues Oliveira S. M., Rebocho A., Ahmadpour E., Nissapatorn V., de Lourdes Pereira M. (2023). Type 1 diabetes mellitus: A review on advances and challenges in creating insulin producing devices. Micromachines (Basel) 14 (1), 151. doi:10.3390/mi14010151
Romanos G. E., Delgado-Ruiz R., Sculean A. (2019). Concepts for prevention of complications in implant therapy. Periodontol 81 (1), 7–17. doi:10.1111/prd.12278
Romanos G. E., Schröter-Kermani C., Weingart D., Strub J. R. (1995). Health human periodontal versus peri-implant gingival tissues: An immunohistochemical differentiation of the extracellular matrix. Int. J. Oral Maxillofac. Implants 10 (6), 750–758.
Ruoslahti E. (1996). Integrin signaling and matrix assembly. Tumour Biol. 17 (2), 117–124. doi:10.1159/000217975
Ryan M. E., Ramamurthy N. S., Sorsa T., Golub L. M. (1999). Mmp-mediated events in diabetes. Ann. N. Y. Acad. Sci. 878, 311–334. doi:10.1111/j.1749-6632.1999.tb07692.x
Sabancı A., Eltas A., Celik B., Otlu B. (2022). The influence of diabetes mellitus on the peri-implant microflora: A cross-sectional study. J. Oral Biol. Craniofac Res. 12 (4), 405–409. doi:10.1016/j.jobcr.2022.05.007
Salvi G. E., Aglietta M., Eick S., Sculean A., Lang N. P., Ramseier C. A. (2012). Reversibility of experimental peri-implant mucositis compared with experimental gingivitis in humans. Clin. Oral Implants Res. 23 (2), 182–190. doi:10.1111/j.1600-0501.2011.02220.x
Salvi G. E., Beck J. D., Offenbacher S. (1998). Pge2, il-1 beta, and tnf-alpha responses in diabetics as modifiers of periodontal disease expression. Ann. Periodontol. 3 (1), 40–50. doi:10.1902/annals.1998.3.1.40
Salvi G. E., Bosshardt D. D., Lang N. P., Abrahamsson I., Berglundh T., Lindhe J., et al. (2000). Temporal sequence of hard and soft tissue healing around titanium dental implants. Periodontol 68 (1), 135–152. doi:10.1111/prd.12054
Sannino G., Montemezzi P., Pantaleo G., Agliardi E. (2020). Dental implants survival rate in controlled type I diabetic patients: A prospective longitudinal study with a 2-year follow-up. J. Biol. Regul. Homeost. Agents 34 (63), 37–45.
Santos V. R., Ribeiro F. V., Lima J. A., Napimoga M. H., Bastos M. F., Duarte P. M. (2010). Cytokine levels in sites of chronic periodontitis of poorly controlled and well-controlled type 2 diabetic subjects. J. Clin. Periodontol. 37 (12), 1049–1058. doi:10.1111/j.1600-051X.2010.01624.x
Schacter G. I., Leslie W. D. (2021). Diabetes and osteoporosis: Part I, epidemiology and pathophysiology. Endocrinol. Metab. Clin. North Am. 50 (2), 275–285. doi:10.1016/j.ecl.2021.03.005
Schmidt A. M., Weidman E., Lalla E., Yan S. D., Hori O., Cao R., et al. (1996). Advanced glycation endproducts (ages) induce oxidant stress in the gingiva: A potential mechanism underlying accelerated periodontal disease associated with diabetes. J. Periodontal Res. 31 (7), 508–515. doi:10.1111/j.1600-0765.1996.tb01417.x
Schroeder A., van der Zypen E., Stich H., Sutter F. (1981). The reactions of bone, connective tissue, and epithelium to endosteal implants with titanium-sprayed surfaces. J. Maxillofac. Surg. 9 (1), 15–25. doi:10.1016/s0301-0503(81)80007-0
Sculean A., Gruber R., Bosshardt D. D. (2014). Soft tissue wound healing around teeth and dental implants. J. Clin. Periodontol. 41 (15), S6–S22. doi:10.1111/jcpe.12206
Sexton W. M., Lin Y., Kryscio R. J., Dawson D. R., Ebersole J. L., Miller C. S. (2011). Salivary biomarkers of periodontal disease in response to treatment. J. Clin. Periodontol. 38 (5), 434–441. doi:10.1111/j.1600-051X.2011.01706.x
Shi B., Lux R., Klokkevold P., Chang M., Barnard E., Haake S., et al. (2020). The subgingival microbiome associated with periodontitis in type 2 diabetes mellitus. ISME J. 14 (2), 519–530. doi:10.1038/s41396-019-0544-3
Shimono M., Ishikawa T., Enokiya Y., Muramatsu T., Matsuzaka K., Inoue T., et al. (2003). Biological characteristics of the junctional epithelium. J. Electron Microsc. (Tokyo) 52 (6), 627–639. doi:10.1093/jmicro/52.6.627
Silva J. A., Ferrucci D. L., Peroni L. A., Abrahão P. G., Salamene A. F., Rossa-Junior C., et al. (2012). Sequential il-23 and il-17 and increased Mmp8 and Mmp14 expression characterize the progression of an experimental model of periodontal disease in type 1 diabetes. J. Cell. Physiol. 227 (6), 2441–2450. doi:10.1002/jcp.22979
Singh B., Fleury C., Jalalvand F., Riesbeck K. (2012). Human pathogens utilize host extracellular matrix proteins laminin and collagen for adhesion and invasion of the host. FEMS Microbiol. Rev. 36 (6), 1122–1180. doi:10.1111/j.1574-6976.2012.00340.x
Singh V. P., Bali A., Singh N., Jaggi A. S. (2014). Advanced glycation end products and diabetic complications. Korean J. Physiol. Pharmacol. 18 (1), 1–14. doi:10.4196/kjpp.2014.18.1.1
Siqueira M. F., Li J., Chehab L., Desta T., Chino T., Krothpali N., et al. (2010). Impaired wound healing in mouse models of diabetes is mediated by tnf-alpha dysregulation and associated with enhanced activation of forkhead box O1 (Foxo1). Diabetologia 53 (2), 378–388. doi:10.1007/s00125-009-1529-y
Snedeker J. G., Gautieri A. (2014). The role of collagen crosslinks in ageing and diabetes - the good, the bad, and the ugly. Muscles Ligaments Tendons J. 4 (3), 303–308. doi:10.32098/mltj.03.2014.07
Song L., Dong G., Guo L., Graves D. T. (2018). The function of dendritic cells in modulating the host response. Mol. Oral Microbiol. 33 (1), 13–21. doi:10.1111/omi.12195
Sorsa T., Gursoy U. K., Nwhator S., Hernandez M., Tervahartiala T., Leppilahti J., et al. (2000). Analysis of matrix metalloproteinases, especially mmp-8, in gingival creviclular fluid, mouthrinse and saliva for monitoring periodontal diseases. Periodontol 70 (1), 142–163. doi:10.1111/prd.12101
Sorsa T., Hernández M., Leppilahti J., Munjal S., Netuschil L., Mäntylä P. (2010). Detection of gingival crevicular fluid mmp-8 levels with different laboratory and chair-side methods. Oral Dis. 16 (1), 39–45. doi:10.1111/j.1601-0825.2009.01603.x
Sorsa T., Mäntylä P., Tervahartiala T., Pussinen P. J., Gamonal J., Hernandez M. (2011). Mmp activation in diagnostics of periodontitis and systemic inflammation. J. Clin. Periodontol. 38 (9), 817–819. doi:10.1111/j.1600-051X.2011.01753.x
Sreedhar R., Arumugam S., Thandavarayan R. A., Karuppagounder V., Koga Y., Nakamura T., et al. (2017). Role of 14-3-3η protein on cardiac fatty acid metabolism and macrophage polarization after high fat diet induced type 2 diabetes mellitus. Int. J. Biochem. Cell. Biol. 88, 92–99. doi:10.1016/j.biocel.2017.05.009
Stamenkovic I. (2003). Extracellular matrix remodelling: The role of matrix metalloproteinases. J. Pathol. 200 (4), 448–464. doi:10.1002/path.1400
Sun X., Mao Y., Dai P., Li X., Gu W., Wang H., et al. (2017). Mitochondrial dysfunction is involved in the aggravation of periodontitis by diabetes. J. Clin. Periodontol. 44 (5), 463–471. doi:10.1111/jcpe.12711
Tarnow D. P. (2014). Commentary: Replacing missing teeth with dental implants: A century of progress. J. Periodontol. 85 (11), 1475–1477. doi:10.1902/jop.2014.140327
Tomasi C., Tessarolo F., Caola I., Wennström J., Nollo G., Berglundh T. (2014). Morphogenesis of peri-implant mucosa revisited: An experimental study in humans. Clin. Oral Implants Res. 25 (9), 997–1003. doi:10.1111/clr.12223
Vaca D. J., Thibau A., Schütz M., Kraiczy P., Happonen L., Malmström J., et al. (2020). Interaction with the host: The role of fibronectin and extracellular matrix proteins in the adhesion of gram-negative bacteria. Med. Microbiol. Immunol. 209 (3), 277–299. doi:10.1007/s00430-019-00644-3
Van Dyke T. E. (2017). Pro-resolving mediators in the regulation of periodontal disease. Mol. Asp. Med. 58, 21–36. doi:10.1016/j.mam.2017.04.006
Venza I., Visalli M., Cucinotta M., De Grazia G., Teti D., Venza M. (2010). Proinflammatory gene expression at chronic periodontitis and peri-implantitis sites in patients with or without type 2 diabetes. J. Periodontol. 81 (1), 99–108. doi:10.1902/jop.2009.090358
Vissink A., Spijkervet F., Raghoebar G. M. (2018). The medically compromised patient: Are dental implants a feasible option? Oral Dis. 24 (1-2), 253–260. doi:10.1111/odi.12762
Walko G., Castañón M. J., Wiche G. (2015). Molecular architecture and function of the hemidesmosome. Cell. Tissue Res. 360 (2), 363–378. doi:10.1007/s00441-014-2061-z
Wilkinson H. N., Clowes C., Banyard K. L., Matteuci P., Mace K. A., Hardman M. J. (2019). Elevated local senescence in diabetic wound healing is linked to pathological repair via Cxcr2. J. Invest. Dermatol 139 (5), 1171–1181. doi:10.1016/j.jid.2019.01.005
Yamada M., Sekiguchi K. (2015). Molecular basis of laminin-integrin interactions. Curr. Top. Membr. 76, 197–229. doi:10.1016/bs.ctm.2015.07.002
Yang X., Gandhi C., Rahman M. M., Appleford M., Sun L. W., Wang X. (2015). Age-related effects of advanced glycation end products (ages) in bone matrix on osteoclastic resorption. Calcif. Tissue Int. 97 (6), 592–601. doi:10.1007/s00223-015-0042-1
Yeh H. C., Lu J. J., Chang S. C., Ge M. C. (2019). Identification of microbiota in peri-implantitis pockets by matrix-assisted laser desorption/ionization time-of-flight mass spectrometry. Sci. Rep. 9, 774. doi:10.1038/s41598-018-37450-5
Zhao B., van der Mei H. C., Subbiahdoss G., de Vries J., Rustema-Abbing M., Kuijer R., et al. (2014). Soft tissue integration versus early biofilm formation on different dental implant materials. Dent. Mater 30 (7), 716–727. doi:10.1016/j.dental.2014.04.001
Zheng J., Chen S., Albiero M. L., Vieira G. H. A., Wang J., Feng J. Q., et al. (2018). Diabetes activates periodontal ligament fibroblasts via nf-?b in vivo. J. Dent. Res. 97 (5), 580–588. doi:10.1177/0022034518755697
Keywords: diabetes, dental implants, soft tissue sealing, mucous integration, peri-implantitis, extracellular matrix
Citation: Zhang Z, Ji C, Wang D, Wang M, Song D, Xu X and Zhang D (2023) The burden of diabetes on the soft tissue seal surrounding the dental implants. Front. Physiol. 14:1136973. doi: 10.3389/fphys.2023.1136973
Received: 03 January 2023; Accepted: 08 February 2023;
Published: 16 February 2023.
Edited by:
Andrea Pilloni, Sapienza University of Rome, ItalyReviewed by:
Constanza Eugenia Martinez, Pontificia Universidad Católica de Chile, ChileYasunori Ayukawa, Kyushu University, Japan
Copyright © 2023 Zhang, Ji, Wang, Wang, Song, Xu and Zhang. This is an open-access article distributed under the terms of the Creative Commons Attribution License (CC BY). The use, distribution or reproduction in other forums is permitted, provided the original author(s) and the copyright owner(s) are credited and that the original publication in this journal is cited, in accordance with accepted academic practice. No use, distribution or reproduction is permitted which does not comply with these terms.
*Correspondence: Dongjiao Zhang, djzhang1109@163.com; Xin Xu, xinxu@sdu.edu.cn