- 1NUTRIM School of Nutrition and Translational Research in Metabolism, Maastricht University Medical Centre, Department of Nutrition and Movement Sciences, Maastricht, Netherlands
- 2INSERM UMR1093, Cognition Action et Plasticité Sensorimotrice, Faculty of Sport Sciences, University of Bourgogne, Dijon, France
Purpose: This study assessed the body composition, cardiorespiratory fitness, fiber type and mitochondrial function, and training characteristics of a 71-year-old runner who broke the world record marathon of the men’s 70–74 age category and held several other world records. The values were compared to those of the previous world-record holder.
Methods: Body fat percentage was assessed using air-displacement plethysmography.
Results: Body fat percentage was 13.5%,
Conclusion: The 71-year-old world-record holder marathon showed a relatively similar
1 Introduction
Participation in distance running events such as marathons has risen in recent years, with this rise being primarily driven by the increased participation of master (>35 years) athletes (Willy and Paquette, 2019). Distance running performance is largely determined by three physiological factors, including the maximal oxygen uptake (
The study of master athletes, and elite or world-class master athletes in particular, can provide essential insights into the ability of humans to maintain a high
On the 8th of May 2022, the marathon world record for the over 70 years category was broken by 4s (2:54:19 vs. 2:54:23 (h:min:s)). Robinson et al. (Robinson et al., 2019) published a case study that documented the physiological profile of the previous 70-year-old record holder who set the record in December 2018. Here, we report the physiological profile of the new world record holder, and compare it to that of the previous world record holder to gain insight into the physiological requirements for world-class performance in aging athletes. We hypothesize that the new world record holder would show a better running economy than his predecessor as the new world record holder completed a high training volume that in turn may improve running economy (Barnes and Kilding, 2014). We also hypothesized that the new record holder would have a similar or lower
2 Methods
2.1 Subject
A 71-year-old Dutch world-class Caucasian long-distance athlete with a height and weight of 181.0 cm and 64.8 kg participated in this study. His competition distances were half-marathons to ultramarathons, with a focus on marathons. He started running at the age of 36 years after being advised to pick up exercise by a physician to lower his blood cholesterol levels. His best running times are 16:42 min:sec at 5 km at the age of 54, 34:35 min:sec at 10 km at the age of 49, and 2:41:01 h:min:sec at the marathon at 54 years old. The athlete currently holds the world record for the 6-h run in the 55–59, 60–64, and 65–69 age categories. He recently got the world record marathon in 2:54:19 h:min:sec in the 70–74 age category (Supplemental Figure S1)
The athletes’ self-reported average weekly training distance in the year leading up to his world-record marathon was 139 km week−1, completed in seven sessions per week (Table 1). In this year (as well as the other years) he regularly performed running sessions of up to 2 h 30 min. He performed no interval sessions on the track, but instead performed two fartlek sessions per week. In these sessions, the athlete would do very variable duration intervals, but typically ranging from 1 min to 15 min. All intervals were performed at an estimated 90% of his maximum capacity, and he tried to keep short (e.g., <1 min) rest periods between the intervals. The other training sessions were low-intensity runs guided by the ability to talk easily while running. Often, these sessions were performed as part of his job as a trainer in which he joined the start-to-run groups. This resulted in two and sometimes even three training sessions being completed per day. In addition, the athlete typically performed two core stability (e.g., front and side planks, body weight squat) sessions per week during his warm-up and reported including numerous easy hill runs in most of his training sessions as a form of strength training. He trained about 50% on concrete/asphalt and 50% on country roads or forest tracks. The athlete tracked all his training sessions with a wristwatch that included a GPS and optical heart rate monitor, and this data was used to compute the weekly and yearly training volumes and average running speed for several years (Table 1), as well as the training intensity distribution (Supplementary File S1).
The athlete volunteered for the study and was informed about its nature and aims and the associated risks and discomfort before giving his oral and written consent to participate in the investigation. Specific written informed consent was obtained from the individual for the publication of any potentially identifiable images or data included in this article. The protocol was in conformity with the Declaration of Helsinki and was approved by the Research Ethics Committee of Maastricht University (nr. FHML-REC2022100). All experiments except for the muscle biopsy were performed on the same day with anthropometrics being measured first, followed by running economy, and finally,
2.2 Anthropometrics
The height and weight of the athlete were taken using a wall-mounted stadiometer (Seca© 216 stadiometer Seca, Hamburg) with an accuracy of 0.1 cm and a calibrated scale included in the Pod Bod technology to the nearest 0.1 kg, respectively. Body fat percentage was assessed before the cardiorespiratory measurements using air-displacement plethysmography (Bod Pod, Life measurement) using a Siri-3 compartment model and predicted lung volume, as described previously (Plasqui et al., 2012; Van Hooren et al., 2022).
2.3 Laboratory running economy and (v) assessment
The running protocol for measuring the running economy,
Running economy and
The rate of oxygen consumption (
The gas exchange and respiratory compensation points were determined using a combination of methods as detailed by Keir and colleagues (Keir et al., 2022). The gas exchange threshold reflects the highest metabolic rate not associated with metabolic acidosis or disruption of physiological homeostasis, while the respiratory compensation point reflects the highest metabolic rate at which physiological homeostasis can be maintained despite a slight but stable metabolic acidosis (Keir et al., 2022).
Because we observed some hyperventilation during the first minute of the
2.4 Muscle fiber content and mitochondrial function
Two years before the experiments in the current study, the athlete had a muscle biopsy taken from the vastus lateralis as part of other studies registered at clinicaltrials.gov with identifier NCT03666013. Here, we report the athlete’s muscle fiber composition and mitochondrial capacity as previously determined in these studies (Grevendonk et al., 2021; McCrum et al., 2021). Briefly, muscle fiber content was determined using histochemical analysis as described previously (McCrum et al., 2021). Mitochondrial capacity was assessed ex vivo using high-resolution respirometry assessed with an Oxygraph (Grevendonk et al., 2021). This approach measures the rate at which mitochondria consume oxygen after different substrate/inhibitor combinations at saturating concentrations are added to the permeabilized muscle fibers in a hyper-oxygenated (∼400 μmol L−1 O2) respiration chamber. This approach is therefore not limited by oxygen or substrate delivery to the fiber as could be the case in vivo. The coupled (state 3) mitochondrial respiration was measured as this provides information on the maximum capacity of the mitochondria to consume oxygen/generate ATP. To this purpose, the following two combinations of substrates and inhibitors were added to the permeabilized muscle fibers: a) 4.0 mmol L−1 malate plus 50 μmol L−1 octanoylcarnitine and 2 mmol L−1 ADP, and b) 4 mmol L−1 malate plus 10 mmol L−1 glutamate and 2 mmol L-1 ADP. Finally, carbonylcyanide-4-(trifluoromethoxy)-phenylhydrazone was added to assess the maximal capacity of the electron transport chain (state 3 uncoupled respiration). Results of these the coupled and uncoupled mitochondrial respiration are presented as the oxygen consumption per mg of wet mass per second. Furthermore, mitochondrial protein complex content was also estimated using OXPHOS protein expression determined from Western blot analysis as described previously (Grevendonk et al., 2021).
3 Results
Body fat percentage was 13.5%, corresponding to an estimated fat mass of 8.8 kg and fat-free mass of 56.0 kg.
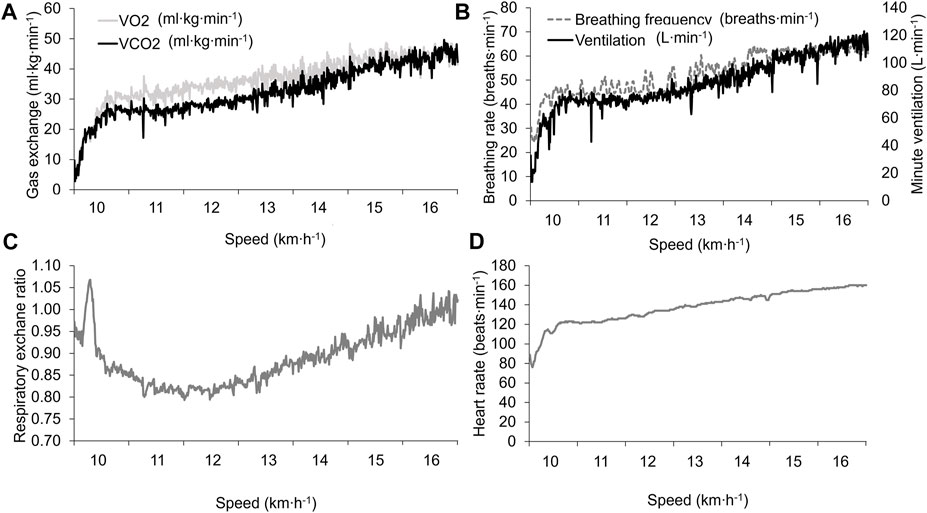
FIGURE 1. Physiological outcomes during the laboratory vV̇O2max assessment. (A) V̇O2 and V̇CO2 relative to body mass; (B) respiratory rate and minute ventilation; (C) respiratory exchange ratio; and (D) heart rate.
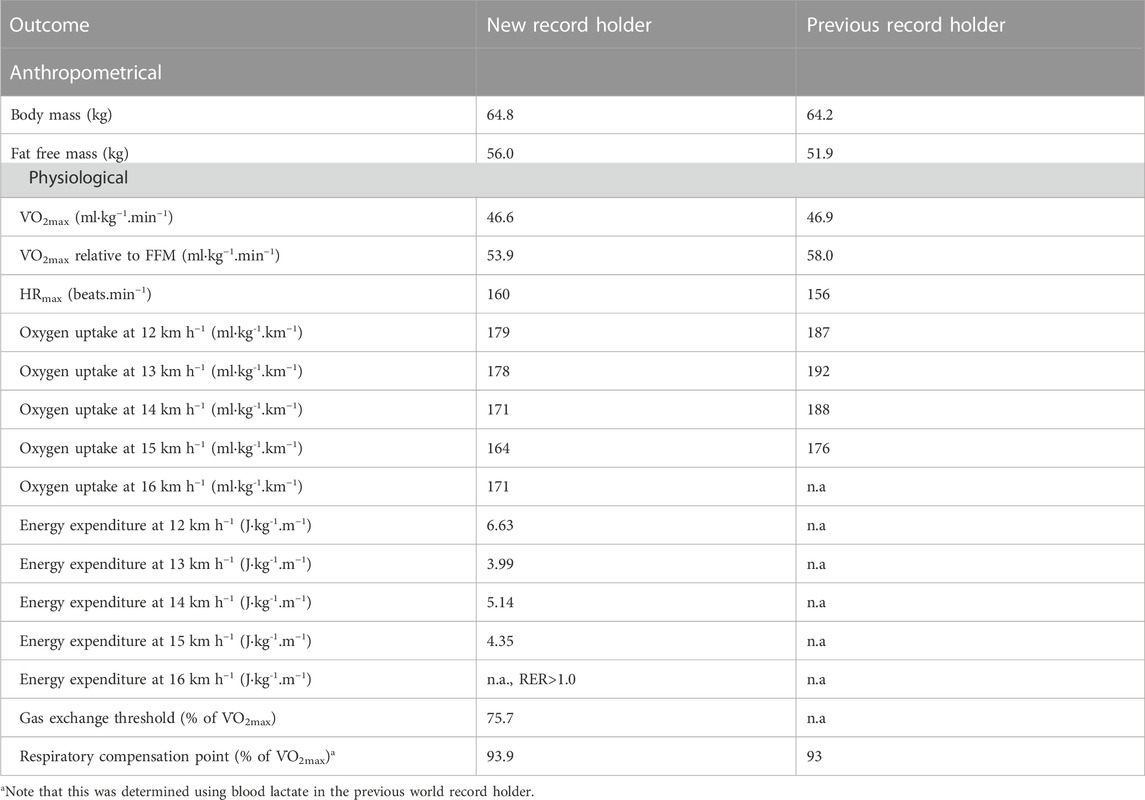
TABLE 2. Anthropometrical and physiological variables of the current and previous world record holder.
Oxygen uptake during the steady state for different speeds is depicted in Figure 2 and reported in Table 2. The oxygen uptake of the previous world record holder is also graphed. The predicted oxygen uptake at the world record pace was 41.3 ml kg−1∙min−1, corresponding to 88.5% of the
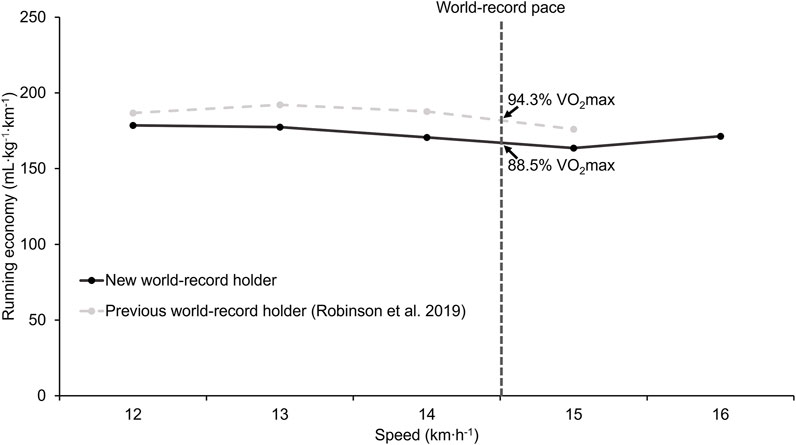
FIGURE 2. Oxygen uptake at different running speeds for the new and previous world record holders. Data from the previous world-record holder were obtained from Robinson et al. (Robinson et al., 2019) using WebPlotDigitizer. The vertical dashed line represents the world-record running speed of 14.52 km h−1.
4 Discussion
This study reports the physiological profile of a 71-year-old distance runner who recently (May 2022) broke the world-record marathon for the age category older than 70. The athlete had a very high cardiorespiratory fitness, as indicated by a
Our primary aim was to investigate which physiological variables might have allowed the present master athlete to improve the world record compared to the previous world-record holder. Because his
The better economy of the new world-record holder, as opposed to the previous record holder, might be explained by aspects such as fiber typology and unmeasured aspects such as mitochondrial efficiency, tendon stiffness, and running technique. Specifically, type I fibers have a higher metabolic efficiency than type II fibers at slow contraction velocities (He et al., 2000), and a high percentage of type I fibers has also been correlated to a better running economy in studies investigating relatively slow running speeds of 12 km h−1 (Bosco et al., 1987). Because the current athlete exhibited >90% type I fibers in the vastus lateralis, this fiber distribution may have contributed to his excellent running economy. Indeed, this percentage of type I fibers is substantially higher than the average type I fiber percentage for older adults (e.g., 68% for master cyclists (Pollock et al., 2018) or 41%–45% for sedentary older men (Houmard et al., 1998; McCrum et al., 2021)). Note however that some studies also observed poorer running economy with higher type I fiber percentages at relatively slow running speeds (e.g., 10–11 km h−1) (Hunter et al., 2015), thus questioning the overall relevance of fiber type to running economy. As type I fibers may only be more efficient than type II fibers at slow contraction velocities (He et al., 2000), it could be speculated that the current athlete had relatively stiff tendons, which resulted in slow fiber contraction velocities and therefore allowed him to benefit from the higher efficiency of a large percentage of type I fibers. In contrast, individuals with more compliant tendons could show less benefit from a large percentage of type I fibers, especially at higher running speeds. Although this hypothesis requires more research, Achilles tendon stiffness has been shown to be an important factor contributing to running economy (Fletcher et al., 2010). Mechanistically, a stiff Achilles tendon may improve the force-length-velocity potential of muscle fibers, thereby reducing the volume of muscle mass that needs to be recruited to produce force, which in turn reduces energy cost (Bohm et al., 2019). The high Achilles tendon stiffness could in turn be an adaptation to the numerous hills included in most training runs, as uphill running requires a larger force production of the calf muscles, and thus would be expected to lead to larger increases in tendon stiffness. It can be speculated that the lower V̇O2max relative to fat free mass of the new world-record holder, as opposed to the previous record holder might also have contributed to the better running economy because previous studies have observed trade-offs between V̇O2max and running economy (Fletcher et al., 2009; Shaw et al., 2015; Lannerstrom et al., 2021). The mechanistic explanation for this trade-off is that a higher oxidative phosphorylation associated with a higher V̇O2max leads to an increased production of reactive oxygen species (ROS). The larger ROS production can be counteracted by a higher mitochondrial uncoupling (i.e. shunting of protons across the inner mitochondrial membrane, thus decreasing membrane potential and ROS, hereby producing heat instead of ATP), but this process is also associated with a higher oxygen consumption (Hunter et al., 2019). Other findings also indirectly support such a mechanistic trade-off (Larsen et al., 2011; Schiffer et al., 2016). In other words, the lower V̇O2max of the new world record holder may have reduced the need for ROS removal and associated higher oxygen consumption by mitochondria during submaximal running, thus also contributing to a better running economy. However, the gross efficiency during cycling of the new world record holder has also been investigated in previous research and was only slightly better than the average gross efficiency in active older athletes (20.8% (Grevendonk et al., 2021)). This collectively suggests that non-metabolic factors such as tendon stiffness and running technique may primarily contribute to the superior running economy.
The physiological adaptations that may contribute to the superior performance of the new world record holder may be a consequence of an almost double training volume of the current athlete compared to the previous record holder (139 vs 72 km wk−1, respectively). Indeed, previous studies found associations between training volume and the percentage of type I fibers (Pollock et al., 2018), and larger training volumes have also been associated with smaller age-related reductions in V̇O2max (dos Anjos Souza et al., 2023; Burtscher et al., 2022). An important factor contributing to the athlete’s ability to complete such a volume is his resilience to injuries, as he trained every day in 2021 and 2022 leading up to the marathon. The athlete attributed this to performing most of his runs at an easy pace, as guided by the ability to talk easily. Indeed, the average running speed throughout the years (Table 1) and heart rate during three training weeks prior to the world record (Supplementary Figure S4) are lower than his first ventilatory threshold, providing some further support for the generally low intensity of his runs. For example, the athlete spend 97% of his training time in zone 1 (i.e., below the gas exchange threshold; Supplementary Figure S4). Notably, the athlete did rarely perform track interval sessions in an attempt to minimize injury risk. Further, the inclusion of both level and up- and downhill running may have resulted in a better load distribution across tissues, hereby further decreasing injury risk. These three characteristics have previously also been reported in a 75-year-old world-class middle-distance runner (Van Hooren et al., 2022), and suggest that exceptional performance with aging might require high training volumes, but primarily at an easy pace and with variation in inclination to minimize injury risk, thereby allowing athletes to train for years in a row continuously. This continued training might be particularly essential for aging athletes considering the potentially more rapid decrease in physiological parameters with rest in older individuals (Toraman, 2005) and subsequent blunted response to training as compared to younger individuals observed in some (LaRoche et al., 2008) (but not all (Hakkinen et al., 2000)) studies. Such effects might not allow aging athletes to return to high-performance levels after an injury. Mechanistically, type I fibers are less susceptible to muscle damage (Schiaffino and Reggiani, 2011), and the high percentage of type I fibers may have allowed the athlete to complete a high training volume, and hereby reach exception performance at this age. Indeed, whereas most older athletes reduce their training volume with increasing age (Aguiar et al., 2020) to compensate for the reduced recovery ability with aging (Brisswalter and Nosaka, 2013), the current athlete increased in training volume in the last few years (Table 1). This likely slowed down the decrease in performance generally observed with aging (Supplementary Figure S1). His decline in marathon performance between 51 and 71 years old (from 2:41 to 2:54) corresponds to an 8% reduction in running speed in 20 years (4% per decade). This age-related performance decline is lower than the 5%–7% per decade generally observed for well-trained marathoners (Lepers et al., 2020; Lepers et al., 2021). Note that the athlete used short (e.g., 10 min) powernaps between two sessions on the same day, but did not apply periodization, did not follow a special diet, and did not perform any heavy resistance training. Implementing such aspects may further improve the world record in the future.
A limitation of this study is that the athlete had rarely run on a treadmill before, and the running economy might therefore be poorer than overground running economy. However, we provided 8 min of familiarization in line with previous guidelines (Van Hooren et al., 2020) to minimize this effect. Moreover, we compared the heart rate at the 14 and 15 km h−1 stages to the heart rate that the athlete recorded while running overground at the same speeds, and the values were approximately similar. Further, differences in treadmill surface stiffness (Colino et al., 2020a; Colino et al., 2020b) and shoe use (Hoogkamer et al., 2018) between the current and previous case study could also contribute to physiological differences. Specifically, the athlete in our study was assessed on the shoes he used during the world record performance, and these shoes contained a carbon plate that may improve running economy (Hébert-Losier and Pamment, 2022). In contrast, the shoes used in the previous studies were standard racing shoes without carbon plates (personal communication Dr. Farquhar 29-7–22). Yet, we used a treadmill that likely exhibited a lower surface stiffness and lower energy restitution (Technogym Exite vs GE T2100) that may have offset some of the beneficial effects of the shoes. Another limitation is that we did not measure lactate concentration and thus approximated the lactate threshold and lactate turn point using gas exchange data. However, several studies showed good agreement between the respiratory compensation point and maximal lactate steady state (Keir et al., 2015; Iannetta et al., 2020; Caen et al., 2021), thus indicating some comparability of these methods. Finally, the muscle biopsy was taken 2 years before the present study, and the athlete has since substantially increased his training volume (Table 1). This may have introduced changes in fiber typology and mitochondrial function. However, we expect this impact to be minor, as the athlete had already been performing endurance training with a weekly training volume of >100 km∙w−1 for multiple years before the biopsy. Related to this, mitochondrial respiration shows a high intra-individual variation (∼15% (Jacobs and Lundby, 2021)) and this should therefore be considered when interpreting this data.
5 Conclusion
The new world-record holder marathon runner in the men’s 70–74 age category showed a relatively similar V̇O2max, a lower fractional utilization but a substantially better running economy than the previous record holder. The better running economy is likely a consequence of an almost double weekly training volume compared to the predecessor and a high percentage of type I fibers. His injury resilience enabled him to train every day for the last ∼1.5 years and achieve international performance in his age group category. The high intensity sustained during the race (here, 88.5% of V̇O2max) seems to be a characteristic of elite master marathoners.
Data availability atatement
The original contributions presented in the study are included in the article/Supplementary Materials, further inquiries can be directed to the corresponding author.
Ethics statement
The studies involving human participants were reviewed and approved by Research Ethics Committee of Maastricht University. The patients/participants provided their written informed consent to participate in this study. Written informed consent was obtained from the individual(s) for the publication of any potentially identifiable images or data included in this article.
Author contributions
BH and RL conceived the study, BH collected and analyzed the data, wrote the first draft of the manuscript, and RL provided comments and edits. All authors approved the final version.
Acknowledgments
We would like to thank Pleun van der Lee and Bram Mennen for their assistance with data collection and Lotte Grevendonk, Joris Hoeks and Matthijs Hesselink for providing the muscle biopsy data. The results of the study are presented clearly, honestly, and without fabrication, falsification, or inappropriate data manipulation.
Conflict of interest
The authors declare that the research was conducted in the absence of any commercial or financial relationships that could be construed as a potential conflict of interest.
Publisher’s note
All claims expressed in this article are solely those of the authors and do not necessarily represent those of their affiliated organizations, or those of the publisher, the editors and the reviewers. Any product that may be evaluated in this article, or claim that may be made by its manufacturer, is not guaranteed or endorsed by the publisher.
Supplementary material
The Supplementary Material for this article can be found online at: https://www.frontiersin.org/articles/10.3389/fphys.2023.1122315/full#supplementary-material
References
Aguiar S., Sousa C. V., Sales M. M., Sousa H. G., Santos P. A., Barbosa L. D., et al. (2020). Age-related decrease in performance of male masters athletes in sprint, sprint–endurance, and endurance events. Sport Sci. Health 16 (3), 385–392. doi:10.1007/s11332-019-00613-6
Allen W. K., Seals D. R., Hurley B. F., Ehsani A. A., Hagberg J. M. (1985). Lactate threshold and distance-running performance in young and older endurance athletes. J. Appl. Physiol. (1985) 58 (4), 1281–1284. doi:10.1152/jappl.1985.58.4.1281
American College of Sports Medicine (2013). ACSM's health-related physical fitness assessment manual. Lippincott Williams & Wilkins.
Barnes K. R., Kilding A. E. (2014). Strategies to improve running economy. Sports Med. 45, 37–56. doi:10.1007/s40279-014-0246-y
Beck O. N., Kipp S., Roby J. M., Grabowski A. M., Kram R., Ortega J. D. (2016). Older runners retain youthful running economy despite biomechanical differences. Med. Sci. Sports Exerc 48 (4), 697–704. doi:10.1249/MSS.0000000000000820
Bohm S., Mersmann F., Santuz A., Arampatzis A. (2019). The force-length-velocity potential of the human soleus muscle is related to the energetic cost of running. Proc. R. Soc. B-Biological Sci. 286 (1917), 20192560. doi:10.1098/rspb.2019.2560
Bosco C., Montanari G., Ribacchi R., Giovenali P., Latteri F., Iachelli G., et al. (1987). Relationship between the efficiency of muscular work during jumping and the energetics of running. Eur. J. Appl. Physiol. Occup. Physiol. 56 (2), 138–143. doi:10.1007/BF00640636
Brisswalter J., Nosaka K. (2013). Neuromuscular factors associated with decline in long-distance running performance in master athletes. Sports Med. 43 (1), 51–63. doi:10.1007/s40279-012-0006-9
Burtscher J., Strasser B., Burtscher M., Millet G. P. (2022). The impact of training on the loss of cardiorespiratory fitness in aging masters endurance athletes. Int. J. Environ. Res. Public Health 19 (17), 11050. doi:10.3390/ijerph191711050
Caen K., Pogliaghi S., Lievens M., Vermeire K., Bourgois J. G., Boone J. (2021). Ramp vs. step tests: Valid alternatives to determine the maximal lactate steady-state intensity? Eur. J. Appl. Physiol. 121 (7), 1899–1907. doi:10.1007/s00421-021-04620-9
Colino E., Felipe J. L., Van Hooren B., Gallardo L., Meijer K., Lucia A., et al. (2020). Mechanical properties of treadmill surfaces compared to other overground sport surfaces. Sensors-Basel. 20 (14), 3822. doi:10.3390/s20143822
Colino E., Garcia-Unanue J., Gallardo L., Foster C., Lucia A., Felipe J. L. (2020). Mechanical properties of treadmill surfaces and their effects on endurance running. Int. J. Sports Physiol. Perform. 15 (5), 685–689. doi:10.1123/ijspp.2019-0539
Costill D. L., Thomason H., Roberts E. (1973). Fractional utilization of the aerobic capacity during distance running. Med. Sci. Sports 5 (4), 248–252. doi:10.1249/00005768-197300540-00007
di Prampero P. E., Atchou G., Bruckner J. C., Moia C. (1986). The energetics of endurance running. Eur. J. Appl. Physiol. Occup. Physiol. 55 (3), 259–266. doi:10.1007/BF02343797
dos Anjos Souza V. R., Seffrin A., da Cunha R. A., Vivan L., de Lira C. A. B., Vancini R. L., et al. (2023). Running economy in long-distance runners is positively affected by running experience and negatively by aging. Physiol. Behav. 258, 114032. doi:10.1016/j.physbeh.2022.114032
L. A. Kaminsky, R. Arena, J. Myers, J. E. Peterman, A. R. Bonikowske, M. P. Harberet al. (2022). Updated reference standards for cardiorespiratory fitness measured with cardiopulmonary exercise testing: Data from the fitness registry and the importance of exercise national database (FRIEND). Mayo clinic proceedings (Elsevier).
Fleg J. L., Lakatta E. G. (1988). Role of muscle loss in the age-associated reduction in VO2 max. J. Appl. Physiol. (1985) 65 (3), 1147–1151. doi:10.1152/jappl.1988.65.3.1147
Fletcher J. R., Esau S. P., Macintosh B. R. (2009). Economy of running: Beyond the measurement of oxygen uptake. J. Appl. Physiol. (1985) 107 (6), 1918–1922. doi:10.1152/japplphysiol.00307.2009
Fletcher J. R., Esau S. P., MacIntosh B. R. (2010). Changes in tendon stiffness and running economy in highly trained distance runners. Eur. J. Appl. Physiol. 110 (5), 1037–1046. doi:10.1007/s00421-010-1582-8
Fuchi T., Iwaoka K., Higuchi M., Kobayashi S. (1989). Cardiovascular changes associated with decreased aerobic capacity and aging in long-distance runners. Eur. J. Appl. Physiol. Occup. Physiol. 58 (8), 884–889. doi:10.1007/BF02332223
Ganse B., Degens H. (2021). Current insights in the age-related decline in Sports performance of the older athlete. Int. J. Sports Med. 42 (10), 879–888. doi:10.1055/a-1480-7730
Grevendonk L., Connell N. J., McCrum C., Fealy C. E., Bilet L., Bruls Y. M. H., et al. (2021). Impact of aging and exercise on skeletal muscle mitochondrial capacity, energy metabolism, and physical function. Nat. Commun. 12 (1), 4773. doi:10.1038/s41467-021-24956-2
Hakkinen K., Alen M., Kallinen M., Newton R. U., Kraemer W. J. (2000). Neuromuscular adaptation during prolonged strength training, detraining and re-strength-training in middle-aged and elderly people. Eur. J. Appl. Physiol. 83 (1), 51–62. doi:10.1007/s004210000248
He Z. H., Bottinelli R., Pellegrino M. A., Ferenczi M. A., Reggiani C. (2000). ATP consumption and efficiency of human single muscle fibers with different myosin isoform composition. Biophys. J. 79 (2), 945–961. doi:10.1016/S0006-3495(00)76349-1
Hébert-Losier K., Pamment M. (2022). Advancements in running shoe technology and their effects on running economy and performance–a current concepts overview. Sports Biomech., 1–16. doi:10.1080/14763141.2022.2110512
Hoogkamer W., Kipp S., Frank J. H., Farina E. M., Luo G., Kram R. (2018). A comparison of the energetic cost of running in marathon racing shoes. Sports Med. 48 (4), 1009–1019. doi:10.1007/s40279-017-0811-2
Houmard J. A., Weidner M. L., Gavigan K. E., Tyndall G. L., Hickey M. S., Alshami A. (1998). Fiber type and citrate synthase activity in the human gastrocnemius and vastus lateralis with aging. J. Appl. Physiol. (1985) 85 (4), 1337–1341. doi:10.1152/jappl.1998.85.4.1337
Hunter G. R., McCarthy J. P., Carter S. J., Bamman M. M., Gaddy E. S., Fisher G., et al. (2015). Muscle fiber type, Achilles tendon length, potentiation, and running economy. J. Strength Cond. Res. 29 (5), 1302–1309. doi:10.1519/JSC.0000000000000760
Hunter G. R., Moellering D. R., Windham S. T., Mathis S. L., Bamman M. M., Fisher G. (2019). Relationship between Vo2peak, cycle economy, and mitochondrial respiration in untrained/trained. J. Appl. Physiol. 127 (6), 1562–1568. doi:10.1152/japplphysiol.00223.2019
Iannetta D., Inglis E. C., Pogliaghi S., Murias J. M., Keir D. A. (2020). A "Step-Ramp-Step" protocol to identify the maximal metabolic steady state. Med. Sci. Sports Exerc 52 (9), 2011–2019. doi:10.1249/Mss.0000000000002343
Jacobs R. A., Lundby C. (2021). Contextualizing the biological relevance of standardized high-resolution respirometry to assess mitochondrial function in permeabilized human skeletal muscle. Acta Physiol. (Oxf). 231 (4), e13625. doi:10.1111/apha.13625
Jamnick N. A., Botella J., Pyne D. B., Bishop D. J. (2018). Manipulating graded exercise test variables affects the validity of the lactate threshold and [formula: See text]. PLoS One 13 (7), e0199794. doi:10.1371/journal.pone.0199794
Jeukendrup A. E., Wallis G. A. (2005). Measurement of substrate oxidation during exercise by means of gas exchange measurements. Int. J. Sports Med. 26, S28–S37. doi:10.1055/s-2004-830512
Joyner M. J. (1991). Modeling: Optimal marathon performance on the basis of physiological factors. J. Appl. Physiol. (1985) 70 (2), 683–687. doi:10.1152/jappl.1991.70.2.683
Karamanidis K., Arampatzis A. (2006). Mechanical and morphological properties of human quadriceps femoris and triceps surae muscle-tendon unit in relation to aging and running. J. Biomech. 39 (3), 406–417. doi:10.1016/j.jbiomech.2004.12.017
Keir D. A., Fontana F. Y., Robertson T. C., Murias J. M., Paterson D. H., Kowalchuk J. M., et al. (2015). Exercise intensity thresholds: Identifying the boundaries of sustainable performance. Med. Sci. Sports Exerc 47 (9), 1932–1940. doi:10.1249/MSS.0000000000000613
Keir D. A., Iannetta D., Mattioni Maturana F., Kowalchuk J. M., Murias J. M. (2022). Identification of non-invasive exercise thresholds: Methods, strategies, and an online app. Sports Med. 52 (2), 237–255. doi:10.1007/s40279-021-01581-z
Lannerstrom J., Nilsson L. C., Cardinale D. A., Bjorklund G., Larsen F. J. (2021). Effects of plyometric training on soft and hard surfaces for improving running economy. J. Hum. Kinet. 79 (1), 187–196. doi:10.2478/hukin-2021-0071
LaRoche D. P., Roy S. J., Knight C. A., Dickie J. L. (2008). Elderly women have blunted response to resistance training despite reduced antagonist coactivation. Med. Sci. Sports Exerc 40 (9), 1660–1668. doi:10.1249/MSS.0b013e3181761561
Larsen F. J., Schiffer T. A., Sahlin K., Ekblom B., Weitzberg E., Lundberg J. O. (2011). Mitochondrial oxygen affinity predicts basal metabolic rate in humans. FASEB J. 25 (8), 2843–2852. doi:10.1096/fj.11-182139
Lazarus N. R., Harridge S. D. R. (2017). Declining performance of master athletes: Silhouettes of the trajectory of healthy human ageing? J. Physiol. 595 (9), 2941–2948. doi:10.1113/JP272443
Lepers R., Bontemps B., Louis J. (2020). Physiological profile of a 59-year-old male world record holder marathoner. Med. Sci. Sports Exerc 52 (3), 623–626. doi:10.1249/MSS.0000000000002181
Lepers R., Burfoot A., Stapley P. J. (2021). Sub 3-hour marathon runners for five consecutive decades demonstrate a reduced age-related decline in performance. Front. Physiol. 12, 649282. doi:10.3389/fphys.2021.649282
Lepers R., Stapley P. J. (2016). Master athletes are extending the limits of human endurance. Front. Physiol. 7, 613. doi:10.3389/fphys.2016.00613
Lucia A., Esteve-Lanao J., Olivan J., Gomez-Gallego F., San Juan A. F., Santiago C., et al. (2006). Physiological characteristics of the best Eritrean runners-exceptional running economy. Appl. Physiol. Nutr. Metab. 31 (5), 530–540. doi:10.1139/h06-029
Mademli L., Arampatzis A. (2008). Mechanical and morphological properties of the triceps surae muscle–tendon unit in old and young adults and their interaction with a submaximal fatiguing contraction. J. Electromyogr. Kinesiol 18 (1), 89–98. doi:10.1016/j.jelekin.2006.09.008
McCrum C., Grevendonk L., Schaart G., Moonen-Kornips E., Jörgensen J. A., Gemmink A., et al. (2021). Type II muscle fibre properties are not associated with balance recovery following large perturbations during walking in young and older adults. bioRxiv. doi:10.1101/2021.11.26.470167
Miller J. R., Van Hooren B., Bishop C., Buckley J. D., Willy R. W., Fuller J. T. (2019). A systematic review and meta-analysis of crossover studies comparing physiological, perceptual and performance measures between treadmill and overground running. Sports Med. 49 (5), 763–782. doi:10.1007/s40279-019-01087-9
New York Times (2016). 85-Year-Old marathoner is so fast that even scientists marvel. Available at: https://www.nytimes.com/2016/12/28/sports/ed-whitlock-marathon-running.html (Accessed 1 4, 2023).
Pantoja P. D., Morin J. B., Peyre-Tartaruga L. A., Brisswalter J. (2016). Running energy cost and spring-mass behavior in young versus older trained athletes. Med. Sci. Sports Exerc 48 (9), 1779–1786. doi:10.1249/MSS.0000000000000959
Plasqui G., Boonen A., Geusens P., Kroot E. J., Starmans M., van der Linden S. (2012). Physical activity and body composition in patients with ankylosing spondylitis. Arthritis Care Res. Hob. 64 (1), 101–107. doi:10.1002/acr.20566
Pollock R. D., O'Brien K. A., Daniels L. J., Nielsen K. B., Rowlerson A., Duggal N. A., et al. (2018). Properties of the vastus lateralis muscle in relation to age and physiological function in master cyclists aged 55-79 years. Aging Cell. 17 (2), e12735. doi:10.1111/acel.12735
Quinn T. J., Manley M. J., Aziz J., Padham J. L., MacKenzie A. M. (2011). Aging and factors related to running economy. J. Strength Cond. Res. 25 (11), 2971–2979. doi:10.1519/JSC.0b013e318212dd0e
Rittweger J., di Prampero P. E., Maffulli N., Narici M. V. (2009). Sprint and endurance power and ageing: An analysis of master athletic world records. Proc. Biol. Sci. 276 (1657), 683–689. doi:10.1098/rspb.2008.1319
Robergs R. A., Dwyer D., Astorino T. (2010). Recommendations for improved data processing from expired gas analysis indirect calorimetry. Sports Med. 40 (2), 95–111. doi:10.2165/11319670-000000000-00000
Robinson A. T., Watso J. C., Babcock M. C., Joyner M. J., Farquhar W. B. (2019). Record-breaking performance in a 70-year-old marathoner. N. Engl. J. Med. 380 (15), 1485–1486. doi:10.1056/NEJMc1900771
Sandford G. N., Rogers S. A., Sharma A. P., Kilding A. E., Ross A., Laursen P. B. (2019). Implementing anaerobic speed reserve testing in the field: Validation of vVO2max prediction from 1500-m race performance in elite middle-distance runners. Int. J. Sports Physiol. Perform. 14 (8), 1147–1150. doi:10.1123/ijspp.2018-0553
Schiaffino S., Reggiani C. (2011). Fiber types in mammalian skeletal muscles. Physiol. Rev. 91 (4), 1447–1531. doi:10.1152/physrev.00031.2010
Schiffer T. A., Peleli M., Sundqvist M. L., Ekblom B., Lundberg J. O., Weitzberg E., et al. (2016). Control of human energy expenditure by cytochrome c oxidase subunit IV-2. Am. J. Physiol. Cell. Physiol. 311 (3), C452–C461. doi:10.1152/ajpcell.00099.2016
Shaw A. J., Ingham S. A., Folland J. P. (2014). The valid measurement of running economy in runners. Med. Sci. Sports Exerc 46 (10), 1968–1973. doi:10.1249/MSS.0000000000000311
Shaw A. J., Ingham S. A., Atkinson G., Folland J. P. (2015). The correlation between running economy and maximal oxygen uptake: Cross-sectional and longitudinal relationships in highly trained distance runners. PLoS One 10 (4), e0123101. doi:10.1371/journal.pone.0123101
Tanaka H., Monahan K. D., Seals D. R. (2001). Age-predicted maximal heart rate revisited. J. Am. Coll. Cardiol. 37 (1), 153–156. doi:10.1016/s0735-1097(00)01054-8
Tanaka H., Tarumi T., Rittweger J. (2011). Aging and physiological lessons from master athletes. Compr. Physiol. 10 (1), 261–296. doi:10.1002/cphy.c180041
Toraman N. F. (2005). Short term and long term detraining: Is there any difference between young-old and old people? Br. J. Sports Med. 39 (8), 561–564. doi:10.1136/bjsm.2004.015420
Trappe S. W., Costill D. L., Vukovich M. D., Jones J., Melham T. (1996). Aging among elite distance runners: A 22-yr longitudinal study. J. Appl. Physiol. 80 (1), 285–290. doi:10.1152/jappl.1996.80.1.285
van der Zwaard S., Brocherie F., Jaspers R. T. (2021). Under the hood: Skeletal muscle determinants of endurance performance. Front. Sports Act. Living 3, 719434. doi:10.3389/fspor.2021.719434
Van Hooren B., Fuller J. T., Buckley J. D., Miller J. R., Sewell K., Rao G., et al. (2020). Is motorized treadmill running biomechanically comparable to overground running? A systematic review and meta-analysis of cross-over studies. Sports Med. 50 (4), 785–813. doi:10.1007/s40279-019-01237-z
Van Hooren B., Plasqui G., Lepers R. (2022). Physiological, spatiotemporal, anthropometric, training, and performance characteristics of a 75-year-old multiple world record holder middle-distance runner. Int. J. Sports Physiol. Perform. 18 (2), 204–208. Epub ahead of print:1-5. doi:10.1123/ijspp.2022-0284
Willy R. W., Paquette M. R. (2019). The Physiology and biomechanics of the master runner. Sports Med. Arthrosc. Rev. 27 (1), 15–21. doi:10.1097/JSA.0000000000000212
Keywords: aging, running, master athlete, oxygen consumption, case report, running economy, training characteristics
Citation: Van Hooren B and Lepers R (2023) A physiological comparison of the new—over 70 years of age—marathon record holder and his predecessor: A case report. Front. Physiol. 14:1122315. doi: 10.3389/fphys.2023.1122315
Received: 12 December 2022; Accepted: 30 January 2023;
Published: 13 February 2023.
Edited by:
Jean-Francois Toussaint, Université Paris Cité, FranceReviewed by:
Simone Porcelli, University of Pavia, ItalySandra K Hunter, Marquette University, United States
Leonardo Alexandre Peyré-Tartaruga, Federal University of Rio Grande do Sul, Brazil
Copyright © 2023 Van Hooren and Lepers. This is an open-access article distributed under the terms of the Creative Commons Attribution License (CC BY). The use, distribution or reproduction in other forums is permitted, provided the original author(s) and the copyright owner(s) are credited and that the original publication in this journal is cited, in accordance with accepted academic practice. No use, distribution or reproduction is permitted which does not comply with these terms.
*Correspondence: Bas Van Hooren, basvanhooren@hotmail.com