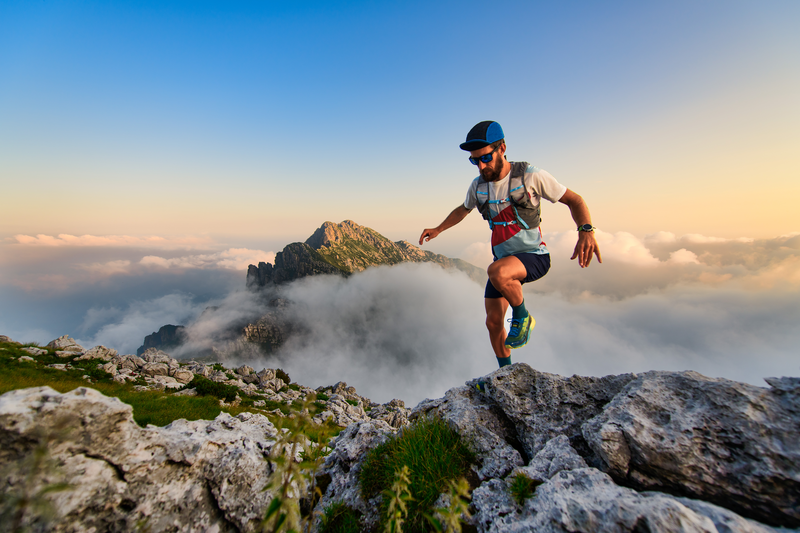
95% of researchers rate our articles as excellent or good
Learn more about the work of our research integrity team to safeguard the quality of each article we publish.
Find out more
ORIGINAL RESEARCH article
Front. Physiol. , 14 February 2023
Sec. Exercise Physiology
Volume 14 - 2023 | https://doi.org/10.3389/fphys.2023.1118127
This article is part of the Research Topic Exercise Friend or Foe? For the Management of Oxidative Stress in Health and Diseases Volume II View all 8 articles
Background: Prolonged and strenuous exercise has been linked to potential exercise-induced myocardial damages. One potential key to unmask the discussed underlying mechanisms of this subclinical cardiac damage could be markers of immunogenic cell damage (ICD). We investigated the kinetics of high-mobility group box 1 protein (HMGB1), soluble receptor for advanced glycation end products (sRAGE), nucleosomes, high sensitive troponin T (hs-TnT) and high sensitive C-reactive protein (hs-CRP) before and up to 12 weeks post-race and described associations with routine laboratory markers and physiological covariates.
Methods: In our prospective longitudinal study, 51 adults (82% males; 43 ± 9 years) were included. All participants underwent a cardiopulmonary evaluation 10–12 weeks pre-race. HMGB1, sRAGE, nucleosomes, hs-TnT and, hs-CRP were analysed 10–12 weeks prior, 1–2 weeks before, immediately, 24 h, 72 h, and 12 weeks post-race.
Results: HMGB1, sRAGE, nucleosomes and hs-TnT increased significantly from pre- to immediately post-race (0.82–2.79 ng/mL; 1132–1388 pg/mL; 9.24–56.65 ng/mL; 6–27 ng/L; p < 0.001) and returned to baseline within 24–72 h. Hs-CRP increased significantly 24 h post-race (0.88–11.5 mg/L; p < 0.001). Change in sRAGE was positively associated with change in hs-TnT (rs = 0.352, p = 0.011). Longer marathon finishing time was significantly associated with decreased levels of sRAGE [−9.2 pg/mL (β = −9.2, SE = 2.2, p < 0.001)].
Conclusion: Prolonged and strenuous exercise increases markers of ICD immediately post-race, followed by a decrease within 72 h. An acute marathon event results in transient alterations of ICD, we assume that this is not solely driven by myocyte damages.
The beneficial effects of regular moderate exercise on the cardiovascular system are well established. Regular exercise improves oxidative capacity (Irving et al., 2015), microvascular collateral formation (Möbius-Winkler et al., 2016) and contractile myocardial function (Nystoriak and Bhatnagar, 2018). Among endurance athletes, there is evidence that prolonged and strenuous exercise is associated with oxidative stress, increased cardiac volume load (Małek Ł et al., 2019) and oxidative DNA damage (Borghini et al., 2015) and may exert exercise-induced myocardial damage and even injury in terms of reversible fatigue or irreversible heart failure (Douglas et al., 1990). Furthermore, an increased risk of exercise-related sudden cardiac death (SCD) has been reported among endurance runners with pre-existing cardiac abnormalities, most likely caused by prolonged strenuous exercise (Albert et al., 2000).
This assumption is supported by studies from the 1990s which reported increased markers of cardiac and muscular damage like troponins (Tn) and creatine kinase (CK) in otherwise healthy individuals after heavy exercise and extreme endurance events such as the Berlin Marathon (Artner-Dworzak et al., 1990; Koller et al., 1995). Additionally, systematic reviews and meta-analyses within the last decade (Regwan et al., 2010; Sedaghat-Hamedani et al., 2015) showed increased Tn values above the 99th percentile (14 ng/L) in 83% of endurance athletes immediately after prolonged and strenuous exercise (Sedaghat-Hamedani et al., 2015). However, our group was able to demonstrate a return of Tn levels to baseline levels within 72 h after a marathon race (Scherr et al., 2011). The time of appearance of the absolute peak value and downslope differ from e.g. myocardial infarction with much faster kinetics pointing to a revesible effect, which leads to the conclusion that these are benign phenomenon. However, recent imaging studies suggest a compromised cardiomyocyte integrity (Aengevaeren et al., 2020).
One potential key to unmask the discussed underlying mechanisms of this subclinical cardiac damage could be markers of immunogenic cell damage (ICD) such as circulating nucleosomes, high-mobility group box 1 protein (HMGB1), and soluble receptor for advanced glycation end-products (sRAGE). Nucleosomes are basic components of nuclear chromatin and are formed by a complex of core histone proteins and DNA that is twisted around them. Linker DNA connects them to a nucleosomal chain (Koyama and Kurumizaka, 2017). After cleavage by specific endonucleases, they are released by dying and stressed cells into the blood circulation. HMGB1 is a nuclear non-histone-DNA-binding protein that stabilizes the nucleosome structure, enables the bending of DNA and facilitates transcription (Balliano et al., 2017). Upon cell stress, HMGB1 can be released in association with nucleosomes or dissociated from them (Bell et al., 2006). RAGE is an important cellular receptor and binding partner for HMGB1, S100 and other danger associated molecular pattern (DAMP) markers transmitting proinflammatory signals on the surface of antigen presenting cells, dendritic cells and macrophages (Pilzweger and Holdenrieder, 2015). However, RAGE can also be shed from these cells and act as a soluble anti-inflammatory decoy receptor by catching HMGB1 and other DAMPs (Bell et al., 2006). These immunogenic markers are released by stressed/damaged cells like muscle, immune, endothelial or liver cells by necrotic, apoptotic or NETosis pathways and therefore, are associated with cardiac cell stress, acute and chronic diseases like diabetes (Volz et al., 2010), arteriosclerosis (Andrassy et al., 2012), cancer (Stoetzer et al., 2012), trauma (Stahl et al., 2016), sepsis (Matsumoto et al., 2015), myocardial infarction (MI) (Sorensen et al., 2011), and heart failure (Volz et al., 2010; Pilzweger and Holdenrieder, 2015).
A recent study conducted by Beko and colleagues including 70 non-professional half marathon and marathon runners reported increased levels of HMGB1 and sRAGE immediately after the race. Both, HMGB1 and sRAGE returned to baseline values after two to 7 days of recovery (Bekos et al., 2016). Beko et al. were not able to detect an increase in sRAGE after a marathon race and they related this to the different training habits. However, they did not further evaluate the association with performance. Consistent with these observations of HMGB1 and sRAGE, circulating cell-free DNA (cfDNA), paralleled that of plasma HMGB1, increased in half marathon runners immediately after the race and decreased to baseline values within 2 h post-race (Atamaniuk et al., 2004). Although, the studies showed mostly an increase of these markers following exercise, many questions remain. To date, no study has evaluated the predictive factors e.g., performance, and laboratory markers associated with the increases of ICD and illuminated the sources of ICD. Therefore, we aimed to evaluate the kinetics of HMGB1, sRAGE, nucleosomes, hs-TnT and hs-CRP before, immediately after, and up to 12 weeks post-race after a marathon race. Furthermore, we identified routine markers of clinical chemistry (e.g., Creatinine and CK) and physiological covariates (exercise capacity, age, BMI and marathon finishing time) associated with post-race concentration of these biomarkers.
We included 51 marathon runners (of the initial 100 participants) of the longitudinal observational ReCaP-Study [Running effects on cognition and plasticity; details published previously (Roeh et al., 2019)] in our analyses. Participants were aged between 18 to 60 years, successfully registered for the Munich Marathon 2017, completed at least one half-marathon prior to the event, had sufficient German language skills and provided written informed consent. Participants with relevant neurological, cardiac or psychiatric diseases, pregnancy, cannabis abuse, and BMI >30 were excluded.
The study has been approved by two local ethic committees: Ludwig-Maximilians University Munich (reference number 17-148) and the University Hospital Klinikum rechts der Isar, Technical University of Munich (reference number 218/17S). The trial was registered at DRKS-German Clinical Trials Register (DRKS-ID: DRKS00012496).
A total of six visits were performed. The first (baseline) visit took place 10–12 weeks (V-1) and the second visit 1–2 weeks (V0) prior to the marathon. The third, fourth, fifth, and sixth were immediately (V1), 24 h (V2.1), 72 h (V2.2) and 12 weeks (V3) after the marathon.
All participants underwent a standardized physical examination and a full cardiac checkup at study site by trained medical staff. Body height and weight were measured via seca scale (Seca, 764, seca GmbH, Hamburg, Germany). Blood pressure (mmHg) was measured after resting in supine position for 5 min. Body fat was measured via calipometry and calculated according to the 7-folder formula of Jackson and Pollock (1978). A standardized 12-lead resting electrocardiography (ECG) was recorded (1 min duration, speed of 50 mm·s−1 and a voltage scale equivalent of 10 mm·mV−1) using Custo cardio 200 (custo diagnostics 3.8; custo med GmbH, Ottobrunn, Germany) in supine position. Additionally, physical fitness and chronotropic competence of all participants were measured via cardiopulmonary exercise test (CPET) including a 12-lead ECG on a treadmill by ramp protocol until voluntary exhaustion. Blood pressure was measured before the test and at maximum load, as well as one and 3 min after the test. Furthermore, a transthoracic echocardiography (IE33, Philips, Amsterdam, Netherlands; standard 2D parasternal short- and long-axis images and apical 2-, 3-, and 4-chamber views) was performed.
At all six visits, blood samples were taken from the antecubital vein using two 10 mL lithium-heparin plasma tube, a 5 mL K2-EDTA plasma tube and a 10 mL gel serum tube (Sarstedt, Nuermbrecht, Germany). Samples were transported (<20 min) to the certified central laboratory of the German Heart Centre Munich where they were centrifuged and analyzed for routine markers of clinical chemistry such as creatinine and creatine kinase (CK) according to national quality standards. Residual samples of heparin, EDTA-plasma and serum were subsequently aliquoted into 2 mL cryotubes and stored at −80°C until further analyses.
HMGB1 and sRAGE concentration was assessed from serum tube by a sandwich enzyme linked immunosorbent assay (ELISA) according to the instructions of the manufacturer (HMGB1 ELISA, ST51011, TECAN IBL International GmbH, Hamburg, Germany; Quantikine® Human RAGE ELISA, R&D Diagnostics, Minneapolis, MN) that was applied on a DS2 automated ELISA processing system (Dynex Technologies, Chantilly, VA, United States). The quantification of the results was done by use of a calibration curve with a measuring range of 0.625–80 ng/mL for HMGB1 and 78–5,000 pg/mL for RAGE.
Nucleosomes were measured from serum tube by the Cell Death Detection ELISAPLUS according to the instructions of the manufacturer (Cat. No. 11774425001, Version 15, Roche Diagnostics, Mannheim, Germany). The quantification of the nucleosomes results was done by use of a calibration curve with a measuring range of 2.83–241.5 ng/mL.
Methods for HMGB1, sRAGE, and nucleosomes were research-use-only (RUO) ELISA assays that were thoroughly validated for their analytical performance and for preanalytically influencing factors before use (Holdenrieder et al., 2001; Lehner et al., 2012; Wittwer et al., 2012). Measurements were done as single determinations. Standard curves and quality controls were included in every run. Serial samples of individuals were run in the same assays. Inter-assay variabilities of the different plates were checked by artificial and serum control materials. Thereby coefficients of variations (CVs) of HMGB1 were between 7.5% and 11.7%, of sRAGE between 5.4% and 8.1% and for nucleosomes at 6.4%.
Hs-TnT was measured from serum tube quantitatively by the highly sensitive electro-chemiluminescence-immunoassay (ECLIA) technology on a Cobas E411 analyser platform (Roche Diagnostics Deutschland GmbH, Mannheim, Germany). The limit of detection (LoD) of the method was 5 ng/L and the limit of quantification (LoQ) 13 ng/L. The reference limit of Hs-TnT in healthy volunteers (99th percentile) was 14 ng/L (Saenger et al., 2011).
Hs-CRP was measured from serum tube by a latex-particle amplified turbidimetry immunoassay method using a Cobas c 501 analyzer (Roche Diagnostics Deutschland GmbH, Mannheim, Germany) with a measurement range of 0.3–200.0 mg/L. The reference limit for adults was <3.0 mg/L (Ridker, 2003).
Methods for hs-cTnT and hs-CRP were IVD-CE labelled methods used in routine diagnostics of the Institute of Laboratory Medicine of the German Heart Centre Munich according the quality control system defined by the guidelines of the German Federal Medical Council (Rili-BÄK).
The statistical analysis was performed with IBM SPSS Statistics for Windows version 25 (IBM Corp., Armonk, NY, United States). The Gaussian distribution was tested using the Kolmogorov–Smirnov test. Normally distributed variables are presented as mean ± standard deviation (SD), non-normally distributed data as median and interquartile range (IQR), categorical variables as absolute (n) and relative (%) frequencies. Distributions of immunogenic markers at different visits were compared using Friedman’s test and between two visits by Wilcoxon signed-rank test; for categorical variables Cochran’s Q-test. Correlations between the variables were assessed using Pearson’s correlation or Spearman’s correlation coefficient. We analyzed the influence of the covariates physical performance, age, BMI and marathon finishing time on the increase of the biomarker with a linear regression model. All tests were performed two-sided with a significance level of α = 0.05.
Out of the initial 100 participants, 51 were included in the present analysis. Reasons for exclusion from final analysis (exclusion reasons during the study period) were: time constraints (n = 18), internal sicknesses (n = 6), orthopedic disease (n = 7), intermediate visit missing for time reasons (n = 10), marathon not finished (n = 4), termination of the study due to personal reasons (n = 1), exclusion criterion for marathon at V-1 (n = 1) and missing blood samples (n = 2). Baseline characteristics of the study population [n = 51, aged 43 ± 9 years (82% male)] are shown in Table 1. A total of 27 (52%) of the included participants had a positive family history of cardiovascular diseases.
Figure 1 shows the kinetics of HMGB1, sRAGE, nucleosomes, hs-TnT and hs-CRP over the different measurement points. Comparison of the data at baseline to immediately after the marathon showed significant increases in sRAGE with medians 1,132 pg/mL (IQR 960–1,430 pg/mL) and 1,388 pg/mL (1,148–1,686 pg/mL, p < 0.001), in HMGB1 with medians of 0.82 ng/mL (IQR 0.62–1.27 ng/mL) and 2.79 ng/mL (1.37–3.87 ng/mL, p < 0.001), in nucleosomes with medians of 9.24 ng/mL (IQR 4.34–15.96 ng/mL) and 56.65 ng/mL (35.12–87.5 ng/mL, p < 0.001), and hs-TnT with medians of 6 ng/L (IQR 5–8 ng/L) and 27 ng/L (19–45 ng/L, p < 0.001). Hs-CRP increased with a peak at 24 h after the marathon (medians 0.88 mg/L (IQR 0.64–1.35 mg/L) and 11.5 mg/L (7.21–16.70 mg/L, p < 0.001) and remained elevated until 72 h 3.8 mg/L (2.34–5.13 mg/L, p < 0.001) after the marathon (Table2). Most remarkably, some runners had very high levels (>100 ng/L) of hs-TnT and/or some also very high levels of sRAGE, HMGB1 and nucleosomes 24 h post-race. In total, HMGB1 and hs-TnT were still slightly elevated after 24 h and returned to baseline 72 h after the marathon while sRAGE and nucleosomes reached baseline levels already after 24 h post-race.
FIGURE 1. HMGB1 (A), sRAGE (B), nucleoseome (C), hs-TnT (D), and hs-CPR (E) concentration 10–12 weeks, 1–2 weeks before the marathon, immediately after the marathon, 24 h, 72 h after the marathon and 12 weeks after the marathon. Boxplots show median and the interquartile range (IQR); *p < 0.05 compared to the baseline values.
HMGB1, sRAGE, nucleosomes, hs-TnT, and hs-CPR concentrations over the study period are summarized in Table 2.
One of the runners had a pre-race (V-1) and 43 runners a post-race (V1) hs-TnT level above the upper reference limit of 14 ng/L (p < 0.001). In one runner (♂, 58 years, BMI 25.5 kg/m2, blood pressure 130/80 mmHg), hs-TnT was elevated at baseline, increased immediately and remained elevated until 72 h after the marathon (Baseline: 24 ng/L; immediately after the marathon: 58 ng/L; 24 h after the marathon: 205 ng/L; 72 h after the marathon: 148 ng/L; 12 weeks after the marathon: 7 ng/L). He revealed neither further cardiovascular risk factors nor symptoms in the course of the study, both initial examinations with cardiopulmonary exercise testing and subsequent examinations were unremarkable.
Overall, none of the runners reported symptoms suggestive of acute myocardial infarction during and/or after the race. Following a comprehensive clinical evaluation at baseline of all included individuals, there was no evidence for any clinically relevant cardiac abnormalities and pathologies.
Regarding the acute effects of marathon running, the following correlations were found: sRAGE immediately after the marathon correlated negatively with the finishing time (r = −0.541; p < 0.001; n = 48, Supplementary Figure S1). Changes in hs-TnT and hs-CRP or CK did not correlate with an increase in HMGB1 or nucleosomes (all p > 0.05). Only, the increase in sRAGE correlated positively with changes in hs-TnT (rs = 0.352, p = 0.011, n = 51), HMGB1 correlated positive with an increase in nucleosomes (r = 0.377, p = 0.006, n = 48), and a hs-TnT increase correlated significantly with an increase in hs-CRP (rs = 0.322, p = 0.021, n = 51).
Linear regression models including age, BMI, marathon finishing time and VO2peak (R2 = 0.271, F (4, 44) = 5.45, p < 0.001) showed that every 1-min increase in marathon finishing time was associated with a sRAGE decrease at visit 1 of −9.2 pg/mL (regression coefficient b = −9.2, standard error = 2.2, p < 0.001), other variables were not significantly associated (BMI: b = −3.0 se = 27.2, p = 0.911; VO2peak: b = −19.72, se = 12.1, p = 0.110; age: b = 1.32, se = 6.6, p = 0.843).
In this prospective observational study, we observed that sRAGE, HMGB1, nucleosomes and hs-TnT increased significantly directly after the marathon. Only hs-CRP increased- as expected- with a delay of 24 h after the marathon. Our findings concerning the kinetics of those markers after marathon complement and extend the work by Beiter et al. (2011); Scherr et al. (2011); Bekos et al. (2016). The three studies showed an increase of HMGB1, nucleosomes or TnT immediately after exhausting exercise in marathon or treadmill runners. Contrary to our results, Bekos et al. did not demonstrate an increase of RAGE immediately after a marathon (Bekos et al., 2016), but only after a half-marathon The author hypothesized that the missing increase of RAGE immediately after the marathon could be the result of training habits or exercise intensity. In this regard, we tested the hypothesis, that training had an influence on the expression of ICD markers, in our analysis. The fact that we found an association between RAGE and marathon finishing time—slower runners had a higher decrease in the RAGE units—suggests that training conditions influence expression of RAGE markers after a marathon. We could not demonstrate further associations between the expression of RAGE and performance parameters. When comparing studies described in the literature regarding training influences on RAGE expression, it shows inhomogeneous results, with studies indicating an inducible decrease of RAGE after training interventions (Kotani et al., 2011; Drosatos et al., 2021). Nevertheless, two studies indicated an increase of RAGE values within training interventions in cardiovascular risk patients (Choi et al., 2012; Sponder et al., 2018). In one randomized controlled trial with type 2 diabetes patients, RAGE levels increased after a 12-week aerobic exercise intervention (60 min at moderate intensity, 5 times/week) (Choi et al., 2012). In a second prospective study, the influence of long-term physical activity (8 months) on serum sRAGE levels in 98 participants was investigated. RAGE levels increased up to 22% in participants with a long-term performance gain of >5% compared to <4.9% (Sponder et al., 2018). Authors of the mentioned studies hypothesized that this variating pattern of decrease and increase in RAGE levels depending on exercise intensity, points towards the capacity of sRAGE to act as a marker for increased or decreased AGEs production (Choi et al., 2012; Sponder et al., 2018). In the present study, we could not demonstrate any association between HMGB1 or Nucleosomes and training intensity. However, previous studies reported that regular training could decrease HMGB1 levels (Gmiat et al., 2017) and that HMGB1 reacts depending on the intensity of the load. This can rather be attributed to an anti-inflammatory process and thus to the chronic long-term effects regarding training. In this case, HMGB1 and nucleosomes are low and sRAGE is rather elevated (as described above). This should be investigated in further studies.
Baseline values such as age, sex or body compositions may contribute to changes in biomarkers released into the circulation. Contrary to the literature, in which more studies indicate an inducible increase in RAGE and nucleosomes in older participants (Pinti et al., 2014; Recabarren-Leiva et al., 2021), we did not find an association between the kinetics of ICD markers and age or sex in our cohort. Only body fat correlated negatively with the increase in post-race sRAGE levels, consistent with existing literature (Dozio et al., 2017; Miranda et al., 2018). A possible explanation for this could be the homogeneous age level (43 ± 9 years) of the participants included in this study. Furthermore, it should be considered that our sample size consists only of 9 females and 42 males, which limits the statistical validity.
It is still unclear whether marathon running can lead to an irreversible cardiac damage or just a reversible cardiac fatigue. Comparing the current results with previous studies assessing the kinetics of TnT and ICM markers in patients, the patterns are significantly different (Apple et al., 1998). In patients after myocardial infarction, TnT and ICM kinetics are characterized by a steep peak increase and followed by prolonged elevation for at least 4–7 days (Apple et al., 1998; Kohno et al., 2009). Furthermore, we found a positive correlation between the increase in sRAGE and hs-TnT (rs = 0.352, p = 0.011), which is a different pattern to that reported in a previous study in patients with non-ST-segment elevation myocardial infarction. This observational study indicated that low levels of sRAGE are associated with high serum levels of cTnI and therefore, suggested that the severity of cardiac damage varies inversely with the levels of sRAGE (McNair et al., 2011). Finally, we plotted the increase in ICD markers from pre to post marathon in relation to the established cardiovascular risk factors (hypertension). We found a significant difference of 0.7 ± 1.0 vs. 2.0 ± 2.0, p = 0.031 in the change of HGMB1 in patients with hypertension compared to without hypertension. However, it should be noted that only two subjects were diagnosed with hypertension.
Since we could not detect any clear cardiac damage, the increases in the markers tend to originate from cells that play a greater role in terms of quantity (muscle cells, endothelia, leukocytes, CRP from liver cells, etc.) and less from perishing cardiac cells. The TnT increase can be explained by a release from muscle cells and the cytosol of the cardiomyocytes (10% of the TnT) (Bleier et al., 1998). The structurally bound fraction, however, is only released after the cardiomyocytes have perished, which can explain the days-long increases in TnT levels after an MI (Pilzweger and Holdenrieder, 2015). Regarding the ICM markers, a distinction must be made with regard to the reaction of the markers to acute and chronic effects. The acute effects only occur immediately after the marathon and are quickly compensated in a good training situation. In the acute phase, all three markers, nucleosomes, HMGB1 and sRAGE, are elevated. After the impact is absent again, the markers will decrease. At least for HMGB1 it is known that–after cessation of the damaging stimulus–it may contribute to regeneration of the tissue and preservation of the organ function on a local level. Therefore, HMGB1 after an acute marathon is hypothesized to be released from inflammatory cells and exerts protective functions for the heart preventing pathological remodeling (Germani et al., 2007). Recent studies suggest that the release of nucleosomes attributes either a rapid, active release mechanism from immune- or endothelial cells, or a passive shear stress-induced detachment mechanism from the cell surface area (Neuberger and Simon, 2022). The typical cell death mechanisms such as necrosis, apoptosis, and “suicidal NETosis (Brinkmann et al., 2004)”, in which chromatin is released from dying neutrophils after chromatin de-condensation and subsequent rupture of the nuclear and cell membrane, requires hours to complete (Neuberger and Simon, 2022). In contrast, the “vital” NETosis, in which detachment occurs passively from the cell surface can happen very rapidly (Pfister, 2022). As described in Pfister, in the vital NETosis, vesicles, containing nuclear DNA from neutrophils, merge with plasma membrane eventually resulting in an alive anuclear cytoplast (Pfister, 2022). Therefore, it seems to be well imaginable that “vital” NETosis is a major mechanism for the release of nucleosomes after marathon. A prolonged increase of nucleosomes following marathon however could originate either from reduced elimination and/or subsequent cell death originating from damaged tissues. In conclusion, the transient increase in these parameters may not reflect a cardiovascular strain. Further studies should investigate the mentioned other originating cell forms.
There are some limitations that should be considered. The sample size of 51 marathon runners is relatively small. However, we investigated our cohort in the context of the training as well as in the recovery period over a after over a 6-month time period. In addition, the recovery period was determined to be 12 weeks after the marathon, which makes it unlikely to detect chronic pathological findings, especially as severe events in a young and healthy study population are rare. Furthermore, cut off values could be addressed only for hs-TnT and CRP. Values for sRAGE, HMGB1 and nucleosome still need to be explored. Future studies should therefore investigate the individual differences over time more closely and examine the cut off values for sRAGE and nucleosomes in order to support clinicians interpreting potential pathological alterations. To evaluate the pathomechanisms of the investigated biomarkers, additional clinical routine markers were taken in account. Nevertheless, specific and unspecific cardiac and pro inflammation pathways with more allocation to a specific organ tend to be more insightful. For example, inclusion of additional biomarkers such as CK-MB, interleukins, myeloperoxidase (MPO), active neutrophil elastase (NE), and proteinase 3 (PR3) should be considered.
In our study, markers of immunogenic cellular damage increased significantly in the early period after prolonged and strenuous exercise and returned to baseline values after a recovery phase of 24–72 h. Based on our results, it seems as if a marathon event does not lead to necrotic alterations but rather points to transient ischemic alterations instead. Therefore, there seems to be no increased risk for persistent cardiac necrosis if no underlying pathology is present. Furthermore, the training condition of active patients should be observed, as more intensive training or a regular training rhythm can influence the expression of the evaluated biomarkers.
The raw data supporting the conclusion of this article will be made available by the authors, without undue reservation.
The studies involving human participants were reviewed and approved by the Ethics Committees of both the Ludwig-Maximilians University Munich (approval number 17-148) and the Technical University Munich (approval number 218/17S). The patients/participants provided their written informed consent to participate in this study.
AR, JS, AH, MH, and PF contributed to the study design. JScho wrote the draft of the manuscript. AR, JS, and SH supervised and manuscript drafting. AR, JS, AH, MH, PF, PK, and BH revised it critically for important intellectual content. KK and SH, contributed to the data acquisition and performed the analysis of the blood samples. JScho, PK, and BH make the statistical analysis for this manuscript. All authors read and gave final approval and agree to be accountable for all aspects of work ensuring integrity and accuracy.
The authors thank the staff of the Department of Prevention, Rehabilitation and Sports Medicine, University Hospital Klinikum rechts der Isar, Technical University of Munich and the Department of Psychiatry and Psychotherapy, University Hospital, Ludwig-Maximilians University Munich. Especially the doctoral students Raffaela Raab, Joanna Moussiopoulou and Viola Landes.
The authors declare that the research was conducted in the absence of any commercial or financial relationships that could be construed as a potential conflict of interest.
All claims expressed in this article are solely those of the authors and do not necessarily represent those of their affiliated organizations, or those of the publisher, the editors and the reviewers. Any product that may be evaluated in this article, or claim that may be made by its manufacturer, is not guaranteed or endorsed by the publisher.
The Supplementary Material for this article can be found online at: https://www.frontiersin.org/articles/10.3389/fphys.2023.1118127/full#supplementary-material
Aengevaeren V. L., Froeling M., Hooijmans M. T., Monte J. R., van den Berg-Faay S., Hopman M. T. E., et al. (2020). Myocardial injury and compromised cardiomyocyte integrity following a marathon run. JACC Cardiovasc. imaging 13 (6), 1445–1447. doi:10.1016/j.jcmg.2019.12.020
Albert C. M., Mittleman M. A., Chae C. U., Lee I. M., Hennekens C. H., Manson J. E. (2000). Triggering of sudden death from cardiac causes by vigorous exertion. N. Engl. J. Med. 343 (19), 1355–1361. doi:10.1056/NEJM200011093431902
Andrassy M., Volz H. C., Maack B., Schuessler A., Gitsioudis G., Hofmann N., et al. (2012). HMGB1 is associated with atherosclerotic plaque composition and burden in patients with stable coronary artery disease. PloS one 7 (12), e52081–e. doi:10.1371/journal.pone.0052081
Apple F. S., Sharkey S. W., Falahati A., Murakami M., Mitha N., Christensen D. (1998). Assessment of left ventricular function using serum cardiac troponin I measurements following myocardial infarction. Clin. chimica acta; Int. J. Clin. Chem. 272 (1), 59–67. doi:10.1016/s0009-8981(97)00252-0
Artner-Dworzak E., Mair J., Seibt I., Koller A., Haid C., Puschendorf B. (1990). Cardiac troponin T identifies unspecific increases of CKMB after physical exercise. Clin. Chem. 36 (10), 1853. doi:10.1093/clinchem/36.10.1853a
Atamaniuk J., Vidotto C., Tschan H., Bachl N., Stuhlmeier K. M., Müller M. M. (2004). Increased concentrations of cell-free plasma DNA after exhaustive exercise. Clin. Chem. 50 (9), 1668–1670. doi:10.1373/clinchem.2004.034553
Balliano A., Hao F., Njeri C., Balakrishnan L., Hayes J. J. (2017). HMGB1 stimulates activity of polymerase β on nucleosome substrates. Biochemistry 56 (4), 647–656. doi:10.1021/acs.biochem.6b00569
Beiter T., Fragasso A., Hudemann J., Niess A. M., Simon P. (2011). Short-term treadmill running as a model for studying cell-free DNA kinetics in vivo. Clin. Chem. 57 (4), 633–636. doi:10.1373/clinchem.2010.158030
Bekos C., Zimmermann M., Unger L., Janik S., Hacker P., Mitterbauer A., et al. (2016). Non-professional marathon running: RAGE axis and ST2 family changes in relation to open-window effect, inflammation and renal function. Sci. Rep. 6, 32315. doi:10.1038/srep32315
Bell C. W., Jiang W., Reich C. F., Pisetsky D. S. (2006). The extracellular release of HMGB1 during apoptotic cell death. Am. J. physiology Cell physiology 291 (6), C1318–C1325. doi:10.1152/ajpcell.00616.2005
Bleier J., Vorderwinkler K. P., Falkensammer J., Mair P., Dapunt O., Puschendorf B., et al. (1998). Different intracellular compartmentations of cardiac troponins and myosin heavy chains: A causal connection to their different early release after myocardial damage. Clin. Chem. 44 (9), 1912–1918. doi:10.1093/clinchem/44.9.1912
Borghini A., Giardini G., Tonacci A., Mastorci F., Mercuri A., Mrakic-Sposta S., et al. (2015). Chronic and acute effects of endurance training on telomere length. Mutagenesis 30 (5), 711–716. doi:10.1093/mutage/gev038
Brinkmann V., Reichard U., Goosmann C., Fauler B., Uhlemann Y., Weiss D. S., et al. (2004). Neutrophil extracellular traps kill bacteria. Sci. (New York, NY) 303 (5663), 1532–1535. doi:10.1126/science.1092385
Choi K. M., Han K. A., Ahn H. J., Hwang S. Y., Hong H. C., Choi H. Y., et al. (2012). Effects of exercise on sRAGE levels and cardiometabolic risk factors in patients with type 2 diabetes: A randomized controlled trial. J. Clin. Endocrinol. Metabolism 97 (10), 3751–3758. doi:10.1210/jc.2012-1951
Douglas P. S., O'Toole M. L., Woolard J. (1990). Regional wall motion abnormalities after prolonged exercise in the normal left ventricle. Circulation 82 (6), 2108–2114. doi:10.1161/01.cir.82.6.2108
Dozio E., Briganti S., Delnevo A., Vianello E., Ermetici F., Secchi F., et al. (2017). Relationship between soluble receptor for advanced glycation end products (sRAGE), body composition and fat distribution in healthy women. Eur. J. Nutr. 56 (8), 2557–2564. doi:10.1007/s00394-016-1291-0
Drosatos I. A., Tsoporis J. N., Izhar S., Gupta S., Tsirebolos G., Sakadakis E., et al. (2021). Differential regulation of circulating soluble receptor for advanced glycation end products (sRAGEs) and its ligands S100a8/A9 four weeks post an exercise intervention in a cohort of young army recruits. Biomolecules 11 (9), 1354. doi:10.3390/biom11091354
Germani A., Limana F., Capogrossi M. C. (2007). Pivotal advances: High-mobility group box 1 protein--a cytokine with a role in cardiac repair. J. Leukoc. Biol. 81 (1), 41–45. doi:10.1189/jlb.0306165
Gmiat A., Mieszkowski J., Prusik K., Prusik K., Kortas J., Kochanowicz A., et al. (2017). Changes in pro-inflammatory markers and leucine concentrations in response to Nordic Walking training combined with vitamin D supplementation in elderly women. Biogerontology 18 (4), 535–548. doi:10.1007/s10522-017-9694-8
Holdenrieder S., Stieber P., Bodenmuller H., Fertig G., Furst H., Schmeller N., et al. (2001). Nucleosomes in serum as a marker for cell death. Clin. Chem. Lab. Med. 39 (7), 596–605. doi:10.1515/CCLM.2001.095
Irving B. A., Lanza I. R., Henderson G. C., Rao R. R., Spiegelman B. M., Nair K. S. (2015). Combined training enhances skeletal muscle mitochondrial oxidative capacity independent of age. J. Clin. Endocrinol. metabolism 100 (4), 1654–1663. doi:10.1210/jc.2014-3081
Jackson A. S., Pollock M. L. (1978). Generalized equations for predicting body density of men. Br. J. Nutr. 40 (3), 497–504. doi:10.1079/bjn19780152
Kohno T., Anzai T., Naito K., Miyasho T., Okamoto M., Yokota H., et al. (2009). Role of high-mobility group box 1 protein in post-infarction healing process and left ventricular remodelling. Cardiovasc Res. 81 (3), 565–573. doi:10.1093/cvr/cvn291
Koller A., Mair J., Mayr M., Calzolari C., Larue C., Puschendorf B. (1995). Diagnosis of myocardial injury in marathon runners. Ann. N. Y. Acad. Sci. 752, 234–235. doi:10.1111/j.1749-6632.1995.tb17431.x
Kotani K., Caccavello R., Sakane N., Yamada T., Taniguchi N., Gugliucci A. (2011). Influence of physical activity intervention on circulating soluble receptor for advanced glycation end products in elderly subjects. J. Clin. Med. Res. 3 (5), 252–257. doi:10.4021/jocmr704w
Koyama M., Kurumizaka H. (2017). Structural diversity of the nucleosome. J. Biochem. 163 (2), 85–95. doi:10.1093/jb/mvx081
Lehner J., Wittwer C., Fersching D., Siegele B., Holdenrieder S., Stoetzer O. J. (2012). Methodological and preanalytical evaluation of an HMGB1 immunoassay. Anticancer Res. 32 (5), 2059–2062.
Małek Ł A., Barczuk-Falęcka M., Werys K., Czajkowska A., Mróz A., Witek K., et al. (2019). Cardiovascular magnetic resonance with parametric mapping in long-term ultra-marathon runners. Eur. J. radiology 117, 89–94. doi:10.1016/j.ejrad.2019.06.001
Matsumoto H., Matsumoto N., Ogura H., Shimazaki J., Yamakawa K., Yamamoto K., et al. (2015). The clinical significance of circulating soluble RAGE in patients with severe sepsis. J. Trauma Acute Care Surg. 78 (6), 1086–1093. doi:10.1097/TA.0000000000000651
McNair E. D., Wells C. R., Qureshi A. M., Pearce C., Caspar-Bell G., Prasad K. (2011). Inverse association between cardiac troponin-I and soluble receptor for advanced glycation end products in patients with non-ST-segment elevation myocardial infarction. Int. J. angiology official Publ. Int. Coll. Angiology, Inc. 20 (1), 49–54. doi:10.1055/s-0031-1272552
Miranda E. R., Fuller K. N. Z., Perkins R. K., Kroeger C. M., Trepanowski J. F., Varady K. A., et al. (2018). Endogenous secretory RAGE increases with improvements in body composition and is associated with markers of adipocyte health. Nutr. Metabolism Cardiovasc. Dis. 28 (11), 1155–1165. doi:10.1016/j.numecd.2018.07.009
Möbius-Winkler S., Uhlemann M., Adams V., Sandri M., Erbs S., Lenk K., et al. (2016). Coronary collateral growth induced by physical exercise: Results of the impact of intensive exercise training on coronary collateral circulation in patients with stable coronary artery disease (EXCITE) trial. Circulation 133 (15), 1438–1448. doi:10.1161/CIRCULATIONAHA.115.016442
Neuberger E. W. I., Simon P. (2022). Cell-free DNA in sports medicine: Implications for clinical laboratory medicine. LaboratoriumsMedizin 46 (4), 295–300. doi:10.1515/labmed-2022-0027
Nystoriak M. A., Bhatnagar A. (2018). Cardiovascular effects and benefits of exercise. Front. cardiovasscular Med. 5, 135. doi:10.3389/fcvm.2018.00135
Pfister H. (2022). Neutrophil extracellular traps and neutrophil-derived extracellular vesicles: Common players in neutrophil effector functions. Diagn. (Basel, Switz. 12 (7), 1715. doi:10.3390/diagnostics12071715
Pilzweger C., Holdenrieder S. (2015). Circulating HMGB1 and RAGE as clinical biomarkers in malignant and autoimmune diseases. Diagnostics 5 (2), 219–253. doi:10.3390/diagnostics5020219
Pinti M., Cevenini E., Nasi M., De Biasi S., Salvioli S., Monti D., et al. (2014). Circulating mitochondrial DNA increases with age and is a familiar trait: Implications for “inflamm-aging”. Eur. J. Immunol. 44 (5), 1552–1562. doi:10.1002/eji.201343921
Recabarren-Leiva D., Burgos C. F., Hernández B., Garcïa-García F. J., Castro R. I., Guzman L., et al. (2021). Effects of the age/rage axis in the platelet activation. Int. J. Biol. Macromol. 166, 1149–1161. doi:10.1016/j.ijbiomac.2020.10.270
Regwan S., Hulten E. A., Martinho S., Slim J., Villines T. C., Mitchell J., et al. (2010). Marathon running as a cause of troponin elevation: A systematic review and meta-analysis. J. interventional Cardiol. 23 (5), 443–450. doi:10.1111/j.1540-8183.2010.00575.x
Ridker P. M. (2003). Clinical application of C-reactive protein for cardiovascular disease detection and prevention. Circulation 107 (3), 363–369. doi:10.1161/01.cir.0000053730.47739.3c
Roeh A., Bunse T., Lembeck M., Handrack M., Pross B., Schoenfeld J., et al. (2019). Running effects on cognition and plasticity (ReCaP): Study protocol of a longitudinal examination of multimodal adaptations of marathon running. Res. sports Med. (Print) 28, 241–255. doi:10.1080/15438627.2019.1647205
Saenger A. K., Beyrau R., Braun S., Cooray R., Dolci A., Freidank H., et al. (2011). Multicenter analytical evaluation of a high-sensitivity troponin T assay. Clin. chimica acta; Int. J. Clin. Chem. 412 (9-10), 748–754. doi:10.1016/j.cca.2010.12.034
Scherr J., Braun S., Schuster T., Hartmann C., Moehlenkamp S., Wolfarth B., et al. (2011). 72-h kinetics of high-sensitive troponin T and inflammatory markers after marathon. Med. Sci. sports Exerc. 43 (10), 1819–1827. doi:10.1249/MSS.0b013e31821b12eb
Sedaghat-Hamedani F., Kayvanpour E., Frankenstein L., Mereles D., Amr A., Buss S., et al. (2015). Biomarker changes after strenuous exercise can mimic pulmonary embolism and cardiac injury--a metaanalysis of 45 studies. Clin. Chem. 61 (10), 1246–1255. doi:10.1373/clinchem.2015.240796
Sorensen M. V., Pedersen S., Mogelvang R., Skov-Jensen J., Flyvbjerg A. (2011). Plasma high-mobility group box 1 levels predict mortality after ST-segment elevation myocardial infarction. JACC Cardiovasc Interv. 4 (3), 281–286. doi:10.1016/j.jcin.2010.10.015
Sponder M., Campean I. A., Emich M., Fritzer-Szekeres M., Litschauer B., Graf S., et al. (2018). Long-term physical activity leads to a significant increase in serum sRAGE levels: A sign of decreased AGE-mediated inflammation due to physical activity? Heart vessels 33 (8), 893–900. doi:10.1007/s00380-018-1125-5
Stahl J. B., Hoecherl E. F., Durner J., Nagel D., Wolf K., Holdenrieder S. (2016). HMGB1, nucleosomes and sRAGE as new prognostic serum markers after multiple trauma. LaboratoriumsMedizin 40 (3), 165–173. doi:10.1515/labmed-2016-0004
Stoetzer O. J., Wittwer C., Lehner J., Fahmueller Y. N., Kohles N., Fersching D. M. I., et al. (2012). Circulating nucleosomes and biomarkers of immunogenic cell death as predictive and prognostic markers in cancer patients undergoing cytotoxic therapy. Expert Opin. Biol. Ther. 12 (Suppl. 1), S217–S224. doi:10.1517/14712598.2012.689280
Volz H. C., Seidel C., Laohachewin D., Kaya Z., Müller O. J., Pleger S. T., et al. (2010). HMGB1: The missing link between diabetes mellitus and heart failure. Basic Res. Cardiol. 105 (6), 805–820. doi:10.1007/s00395-010-0114-3
Keywords: biomarker, necrosis, healthy, HMGB1, sRAGE, exercise
Citation: Schoenfeld J, Roeh A, Holdenrieder S, von Korn P, Haller B, Krueger K, Falkai P, Halle M, Hasan A and Scherr J (2023) High-mobility group box 1 protein, receptor for advanced glycation end products and nucleosomes increases after marathon. Front. Physiol. 14:1118127. doi: 10.3389/fphys.2023.1118127
Received: 07 December 2022; Accepted: 23 January 2023;
Published: 14 February 2023.
Edited by:
Ricardo A. Pinho, Pontifical Catholic University of Parana, BrazilReviewed by:
Elmo Neuberger, Johannes Gutenberg University Mainz, GermanyCopyright © 2023 Schoenfeld, Roeh, Holdenrieder, von Korn, Haller, Krueger, Falkai, Halle, Hasan and Scherr. This is an open-access article distributed under the terms of the Creative Commons Attribution License (CC BY). The use, distribution or reproduction in other forums is permitted, provided the original author(s) and the copyright owner(s) are credited and that the original publication in this journal is cited, in accordance with accepted academic practice. No use, distribution or reproduction is permitted which does not comply with these terms.
*Correspondence: Johannes Scherr, am9oYW5uZXMuc2NoZXJyQGJhbGdyaXN0LmNo
Disclaimer: All claims expressed in this article are solely those of the authors and do not necessarily represent those of their affiliated organizations, or those of the publisher, the editors and the reviewers. Any product that may be evaluated in this article or claim that may be made by its manufacturer is not guaranteed or endorsed by the publisher.
Research integrity at Frontiers
Learn more about the work of our research integrity team to safeguard the quality of each article we publish.