- 1Department of Intervention Research in Exercise Training, German Sport University Cologne, Cologne, Germany
- 2Department of Fitness and Health, IST University of Applied Sciences, Duesseldorf, Germany
- 3Department of Movement and Training Science, University of Wuppertal, Wuppertal, Germany
- 4Department of Sports Science, Bielefeld University, Bielefeld, Germany
The time spent above 90% of maximal oxygen uptake (
1 Introduction
High level endurance training requires large training volumes (Seiler, 2010). In elite athletes, commonly, a high proportion of this training volume is performed at low training intensities (Seiler, 2010). However, to achieve an optimal metabolic training stimulus on maximal oxygen uptake (
In both prospective and cohort studies, a high weekly running volume has been associated with running-related injuries (Macera et al., 1989; Walter et al., 1989). Although the causes of running injuries are multifactorial, in this context, the runner’s interaction with the ground and the resulting reaction force has been considered to be one risk factor (Zadpoor and Nikooyan, 2011; Daoud et al., 2012). Thus, higher loading rates were associated with increased risk of sustaining an injury (Crowell and Davis, 2011; Futrell et al., 2018). More recently, however, in a prospective case control-study in recreational runners, the vertical impact peak and loading rate were not associated with a higher injury rate (Malisoux et al., 2022). Furthermore, in collegiate cross country runners, an higher occurrence rate of bone stress injuries has been linked to a higher step rate, but not higher ground reaction forces (Kliethermes et al., 2021). Nevertheless, besides adequate periodization and polarization models in endurance sports, reducing loading rates is still recommended as an effective means to reduce the risk of developing running injuries (Bowser et al., 2018). In this context, increasing the slope might lead to a significantly lower vertical loading rate during uphill running compared to flat level running (Gottschall and Kram, 2005; Lemire et al., 2022a). Also, increasing the slope from flat level running to 7% was found to reduce flight time and increase floor contact time, in turn resulting in highly significant increases in step frequency (Padulo et al., 2013). Apart from this, previous research revealed an increased energy cost via uphill running compared to horizontal running (Lemire et al., 2022b). Additionally, when running at the same velocity, uphill running is more metabolically demanding than horizontal running (Minetti et al., 2002; Vernillo et al., 2017), hence allowing a similar training stimulus at a lower running velocity.
Against this background, this randomized crossover testing examined the peak
2 Materials and methods
2.1 Participants
G*Power (Version 3.1.9.6) was employed to perform an a priori power analysis. Based on increased metabolic costs via uphill running (Minetti et al., 1994; 2002; Vernillo et al., 2017) moderate effect sizes (standard mean differences (SMD) = 0.60) between horizontal and uphill HIIT running were assumed. A sample size of n = 13 was determined, using the following statistical indicators (α = 0.05; study power (1-β-error) = 0.95; one tail). Assuming moderate dropouts (15%–20%), n = 17 well-trained runners were enrolled in this acute randomized controlled crossover testing. These participants consisted of 8 female (age: 24.4 ± 3.7 yrs; height: 1.69 ± 0.07 m; body mass: 56.6 ± 5.8 kg; body fat: 14.6 ± 4.8%;
2.2 Testing procedures
The measurements were conducted within four lab visits over 3 weeks for each participant. Thereby, horizontal and uphill
Spirometric data during all lab visits were collected using a breath-by-breath system (Zan 600 Oxi USB, Zan Messgeräte, Oberthulba, Germany). This spirometric system was calibrated prior to each test, following the manufacturer’s recommendations. To determine uphill and horizontal-running
2.3 Statistics
Data are presented as means ± standard deviations. Normal distribution was initially tested using Shapiro-Wilk tests (p ≥ 0.1). Variance homogeneity was visually confirmed via plotting sampled residuals vs. theoretical (ideal) residuals (Kozak and Piepho, 2018). Sphericity was verified via Mauchly´s tests. To examine mode differences (horizontal vs. uphill) for the respective outcome measures (
3 Results
3.1 Incremental ramp test
No significant differences (p = 0.100; pes = 0.100; mean difference (MD) = 0.2 ± 0.5 L/min; SMD = 0.28) were found between horizontal (3.9 ± 0.7 L/min) and uphill
3.2 HIIT sessions
rANOVA revealed significant effects (p ≤ 0.012; pes ≥0.351) regarding
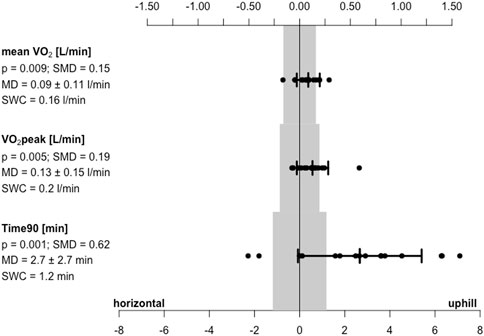
FIGURE 1. Mean difference (MD ± standard deviation) between horizontal and uphill high intensity training protocols for mean oxygen consumption (
No significant mode × time rANOVA interaction effects (p ≥ 0.097; pes ≤0.14) for lactate, HR, RPE and running velocity were found (Figure 2). Nevertheless, running velocity revealed significant time effects (p ≤ 0.001). Subsequently performed post hoc tests (p ≤ 0.001; SMD ≥3.53) revealed higher running velocity during horizontal HIIT (4.47 ± 0.33 to 4.51 ± 0.35 m/s) compared to uphill HIIT (3.17 ± 0.18 to 3.18 ± 0.21 m/s) during all intervals.
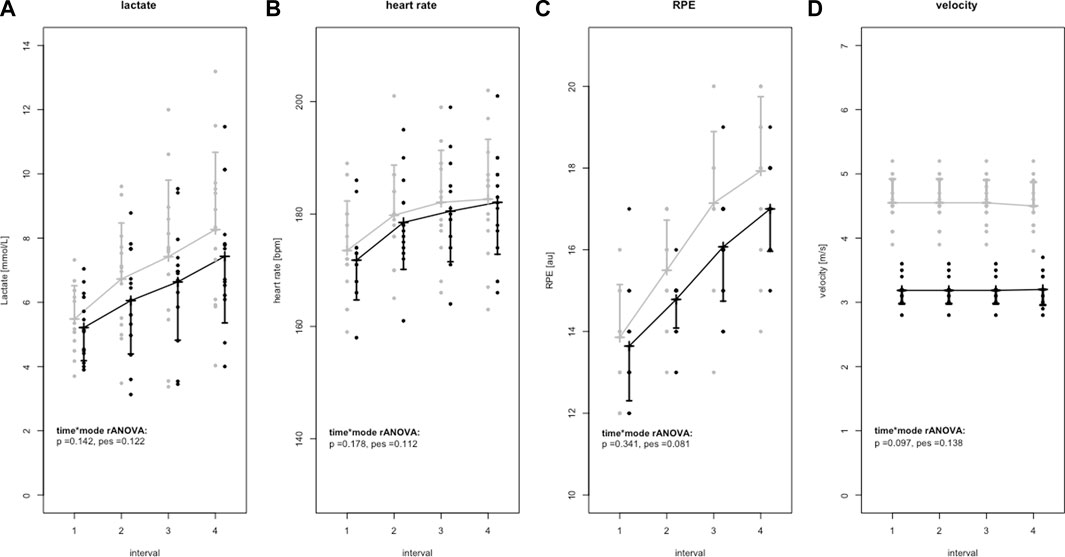
FIGURE 2. Lactate (A), heart rate (B), RPE (C), and running velocity (D) data (mean ± standard deviation) of horizontal (grey) and uphill (black) high intensity training protocols. Individual values are marked as points. In addition, p-values of time*mode interaction effects (p) of the repeated measurement variance analyses (rANOVA) and corresponding effect sizes as partial eta squared (pes) are given.
4 Discussion
To the best of our knowledge, this is the first acute randomized controlled crossover study that examined
A higher acute oxygen consumption during uphill running is commonly explained by the fact that the use of elastic energy may be compromised, so that in turn more mechanical energy (i.e., greater concentric muscle activity) needs to be generated, in order to lift the body’s center of gravity upward and subsequently overcome the slope (Snyder and Farley, 2011). Thus, in the present study, uphill running during a HIIT session notably increased the mean time ≥90%
Previous research revealed that 19%–79% of runners report musculoskeletal injuries of the lower extremities annually (van Gent et al., 2007). Thereby, loading rate and ground reaction force were repeatedly named as relevant risk factors (Crowell and Davis, 2011; Zadpoor and Nikooyan, 2011; Futrell et al., 2018). These relationships, however, were often established based on retrospective, cross-sectional data. More recently, in prospective case control-studies comprising recreational (Malisoux et al., 2022) and collegiate cross country runners (Kliethermes et al., 2021), the vertical impact peak and loading rate were not associated with a higher injury rate. Nevertheless, reducing loading rates is still recommended as an effective means to reduce the risk of developing running injuries (Bowser et al., 2018). In this context, uphill running revealed decreased ground reaction force data compared to horizontal running (Gottschall and Kram, 2005). Furthermore, we observed decreased running velocities during uphill HIIT compared to horizontal HIIT, which additionally decrease loading rate and ground reaction force (Keller et al., 1996). In detail, previous research revealed a 22%–39% ground reaction force decrease via an 6%–9% slope increase (Gottschall and Kram, 2005; Kowalski and Li, 2016). Furthermore, slower running resulted in reduced ground reaction force (Keller et al., 1996). Based on our running velocity differences between horizontal and uphill HIIT, this would result in a ground reaction force reduction of 11%. For the present study a possible reduction of loading rates remains, however, speculative, as these loading rates and ground reaction forces were not measured. Thus, more adequately powered prospective studies are necessary to investigate the association of musculoskeletal injuries of the lower extremities and loading rate as well as the potential prevention effect of uphill running.
Horizontal running has been linked to the stretch-shortening cycle of the muscle-tendon unit of the lower limb (Schöffl et al., 2021), in which part of the mechanical energy of the center of mass (COM) is absorbed during the negative work phase to be restored during the next positive work phase (Nicol et al., 2006). This storage and release of kinetic and potential energy contributes to the acceleration of the body upwards during the propulsive phase and to the reduction of the energy production needed during the concentric phase (Snyder and Farley, 2011; Snyder et al., 2012). In contrast, during uphill running, the center of mass needs to be propelled vertically and does not oscillate around an equilibrium (Dewolf et al., 2016). In detail, the center of mass loses horizontal while simultaneously gaining vertical velocity during the first part of ground contact. Subsequently, during the second part of the contact, a fraction of the energy stored in the elastic elements of the muscle tendon unit is released to increase the kinetic and potential of the center of mass (Dewolf et al., 2016). Accordingly, differences in muscle activation patterns of the lower extremities have been reported between horizontal and uphill running (Yokozawa et al., 2007), with concentric muscle work being dominant during uphill running (Giandolini et al., 2016). Furthermore, to increase the running velocity in flat running conditions, athletes tend to increase their stride length and frequency almost linearly (Ito et al., 1983; Cavanagh and Kram, 1989; Brisswalter and Legros, 1995). Simultaneously, the floor contact time and flight time are reduced (Ito et al., 1983; Cavanagh and Kram, 1989; Brisswalter and Legros, 1995). Even though this pattern is also visible during uphill running compared to flat running, stride length and flight time are significantly reduced, since the foot touches the belt or ground earlier (Padulo et al., 2012; 2013). As the floor contact time does not seem to differ between flat and uphill running, this subsequently leads to a significant reduction in flight time during the uphill running condition (Padulo et al., 2012; 2013). Therefore, it seems possible, that prolonged training sessions running uphill might change the athlete’s kinematics, thus resulting in a reduction in running economy at horizontal conditions. Nevertheless, at least for constant running velocities, experienced athletes select an individual combination of stride length and frequency resulting in the least energy cost (Cavanagh and Kram, 1989; Cavagna et al., 1991), while providing the greatest mechanical efficiency (Morgan et al., 1994). Even though only a small fraction of the overall training time is spent on high-intensity running (Stöggl and Sperlich, 2015), a potential longitudinal effect on running economy induced by prolonged uphill running should be addressed in further research.
A limitation that needs to be addressed is the lack of spatiotemporal running parameters including information on stride length and frequency. Thus, further research should try to disentangle the relationship between spatiotemporal running parameters and oxygen uptake during uphill running. In addition, the potential long-term training effects mentioned above should be examined in appropriate longitudinal intervention studies.
In conclusion, this randomized crossover testing revealed increased mean
Data availability statement
The raw data supporting the conclusions of this article will be made available by the authors, without undue reservation.
Ethics statement
The studies involving human participants were reviewed and approved by Ethical committee of the German Sport University Cologne (approval no. 153/2022). The patients/participants provided their written informed consent to participate in this study.
Author contributions
SH, RG, and LD contributed to the conception and design of the study. RG, TW, and JD led the intervention. LR, SH, and TW performed the statistical analysis. SH wrote the first draft of the manuscript. LR, TW, PW, and LD wrote sections of the manuscript. PW copyedited the draft for content, language, and format, and organized the submission and revision/resubmission process. All authors contributed to the article and approved the submitted version.
Funding
We acknowledge the financial support of the German Research Foundation (DFG) and the Open Access Publication Fund of Bielefeld University for the article processing charge.
Acknowledgments
We appreciatively acknowledge Jonas Hochstrate for his support during the data acquisition phase.
Conflict of interest
The authors declare that the research was conducted in the absence of any commercial or financial relationships that could be construed as a potential conflict of interest.
Publisher’s note
All claims expressed in this article are solely those of the authors and do not necessarily represent those of their affiliated organizations, or those of the publisher, the editors and the reviewers. Any product that may be evaluated in this article, or claim that may be made by its manufacturer, is not guaranteed or endorsed by the publisher.
References
Baumgartner T., Held S., Klatt S., Donath L. (2021). Limitations of foot-worn sensors for assessing running power. Sensors (Basel) 21, 4952. doi:10.3390/s21154952
Bossi A. H., Mesquida C., Passfield L., Rønnestad B. R., Hopker J. G. (2020). Optimizing interval training through power-output variation within the work intervals. Int. J. Sports Physiol. Perform. 15, 982–989. doi:10.1123/ijspp.2019-0260
Bowser B. J., Fellin R., Milner C. E., Pohl M. B., Davis I. S. (2018). Reducing impact loading in runners: A one-year follow-up. Med. Sci. Sports Exerc 50, 2500–2506. doi:10.1249/MSS.0000000000001710
Brisswalter J., Legros P. (1995). Use of energy cost and variability in stride length to assess an optimal running adaptation. Percept. Mot. Ski. 80, 99–104. doi:10.2466/pms.1995.80.1.99
Buchheit M., Laursen P. B. (2013). High-intensity interval training, solutions to the programming puzzle: Part I: Cardiopulmonary emphasis. Sports Med. 43, 313–338. doi:10.1007/s40279-013-0029-x
Cassirame J., Godin A., Chamoux M., Doucende G., Mourot L. (2022). Physiological implication of slope gradient during incremental running test. Int. J. Environ. Res. Public Health 19, 12210. doi:10.3390/ijerph191912210
Cavagna G. A., Willems P. A., Franzetti P., Detrembleur C. (1991). The two power limits conditioning step frequency in human running. J. Physiol. 437, 95–108. doi:10.1113/jphysiol.1991.sp018586
Cavanagh P. R., Kram R. (1989). Stride length in distance running: Velocity, body dimensions, and added mass effects. Med. Sci. Sports Exerc 21, 467–479. doi:10.1249/00005768-198908000-00020
Cohen J. (1988). Statistical power analysis for the behavioral sciences. Hillside: Lawrence Earlbaum Associates.
Crowell H. P., Davis I. S. (2011). Gait retraining to reduce lower extremity loading in runners. Clin. Biomech. (Bristol, Avon) 26, 78–83. doi:10.1016/j.clinbiomech.2010.09.003
Daoud A. I., Geissler G. J., Wang F., Saretsky J., Daoud Y. A., Lieberman D. E. (2012). Foot strike and injury rates in endurance runners: A retrospective study. Med. Sci. Sports Exerc 44, 1325–1334. doi:10.1249/MSS.0b013e3182465115
Dewolf A. H., Peñailillo L. E., Willems P. A. (2016). The rebound of the body during uphill and downhill running at different speeds. J. Exp. Biol. 219, 2276–2288. doi:10.1242/jeb.142976
Foster C., Florhaug J., Franklin J., Gottschall L., Hrovatin L., Parker S., et al. (2001). A new approach to monitoring exercise training. J. Strength Cond. Res. 15, 109–115. doi:10.1519/1533-4287(2001)015<0109:anatme>2.0.co;2
Futrell E. E., Jamison S. T., Tenforde A. S., Davis I. S. (2018). Relationships between habitual cadence, footstrike, and vertical load rates in runners. Med. Sci. Sports Exerc 50, 1837–1841. doi:10.1249/MSS.0000000000001629
Giandolini M., Vernillo G., Samozino P., Horvais N., Edwards W. B., Morin J.-B., et al. (2016). Fatigue associated with prolonged graded running. Eur. J. Appl. Physiol. 116, 1859–1873. doi:10.1007/s00421-016-3437-4
Gottschall J. S., Kram R. (2005). Ground reaction forces during downhill and uphill running. J. Biomech. 38, 445–452. doi:10.1016/j.jbiomech.2004.04.023
Hopkins W. (2004). How to interpret changes in an athletic performance test. Sportscience, 1–7. Available at: https://www.sportsci.org/jour/04/wghtests.htm.
Ito A., Komi P. V., Sjödin B., Bosco C., Karlsson J. (1983). Mechanical efficiency of positive work in running at different speeds. Med. Sci. Sports Exerc 15, 299–308. doi:10.1249/00005768-198315040-00009
Joyner M. J., Coyle E. F. (2008). Endurance exercise performance: The physiology of champions. J. Physiol. 586, 35–44. doi:10.1113/jphysiol.2007.143834
Keller T. S., Weisberger A. M., Ray J. L., Hasan S. S., Shiavi R. G., Spengler D. M. (1996). Relationship between vertical ground reaction force and speed during walking, slow jogging, and running. Clin. Biomech. (Bristol, Avon) 11, 253–259. doi:10.1016/0268-0033(95)00068-2
Kliethermes S. A., Stiffler-Joachim M. R., Wille C. M., Sanfilippo J. L., Zavala P., Heiderscheit B. C. (2021). Lower step rate is associated with a higher risk of bone stress injury: A prospective study of collegiate cross country runners. Br. J. Sports Med. 55, 851–856. doi:10.1136/bjsports-2020-103833
Kowalski E., Li J. X. (2016). Lower limb joint angles and ground reaction forces in forefoot strike and rearfoot strike runners during overground downhill and uphill running. Sports Biomech. 15, 497–512. doi:10.1080/14763141.2016.1185458
Kozak M., Piepho H.-P. (2018). What’s normal anyway? Residual plots are more telling than significance tests when checking ANOVA assumptions. J. Agron. Crop Sci. 204, 86–98. doi:10.1111/jac.12220
Laursen P. B., Jenkins D. G. (2002). The scientific basis for high-intensity interval training: Optimising training programmes and maximising performance in highly trained endurance athletes. Sports Med. 32, 53–73. doi:10.2165/00007256-200232010-00003
Lemire M., Falbriard M., Aminian K., Pavlik E., Millet G. P., Meyer F. (2022a). Correspondence between values of vertical loading rate and oxygen consumption during inclined running. Sports Med. - Open 8, 114. doi:10.1186/s40798-022-00491-2
Lemire M., Hureau T. J., Remetter R., Geny B., Kouassi B. Y. L., Lonsdorfer E., et al. (2020). Trail runners cannot reach
Lemire M., Remetter R., Hureau T. J., Geny B., Lonsdorfer E., Favret F., et al. (2022b). Energy cost of running in well-trained athletes: Toward slope-dependent factors. Int. J. Sports Physiol. Perform. 17, 423–431. doi:10.1123/ijspp.2021-0047
Macera C. A., Pate R. R., Powell K. E., Jackson K. L., Kendrick J. S., Craven T. E. (1989). Predicting lower-extremity injuries among habitual runners. Arch. Intern Med. 149, 2565–2568. doi:10.1001/archinte.149.11.2565
Malisoux L., Gette P., Delattre N., Urhausen A., Theisen D. (2022). Spatiotemporal and ground-reaction force characteristics as risk factors for running-related injury: A secondary analysis of a randomized trial including 800+ recreational runners. Am. J. Sports Med. 50, 537–544. doi:10.1177/03635465211063909
Margaria R., Cerretelli P., Aghemo P., Sassi G. (1963). Energy cost of running. J. Appl. Physiol. 18, 367–370. doi:10.1152/jappl.1963.18.2.367
Midgley A. W., McNaughton L. R., Polman R., Marchant D. (2007). Criteria for determination of maximal oxygen uptake: A brief critique and recommendations for future research. Sports Med. 37, 1019–1028. doi:10.2165/00007256-200737120-00002
Midgley A. W., McNaughton L. R., Wilkinson M. (2006). Is there an optimal training intensity for enhancing the maximal oxygen uptake of distance runners?: Empirical research findings, current opinions, physiological rationale and practical recommendations. Sports Med. 36, 117–132. doi:10.2165/00007256-200636020-00003
Minetti A. E., Ardigò L. P., Saibene F. (1994). Mechanical determinants of the minimum energy cost of gradient running in humans. J. Exp. Biol. 195, 211–225. doi:10.1242/jeb.195.1.211
Minetti A. E., Moia C., Roi G. S., Susta D., Ferretti G. (2002). Energy cost of walking and running at extreme uphill and downhill slopes. J. Appl. Physiol. 93, 1039–1046. doi:10.1152/japplphysiol.01177.2001
Morgan D., Martin P., Craib M., Caruso C., Clifton R., Hopewell R. (1994). Effect of step length optimization on the aerobic demand of running. J. Appl. Physiol. 77, 245–251. doi:10.1152/jappl.1994.77.1.245
Nicol C., Avela J., Komi P. V. (2006). The stretch-shortening cycle: A model to study naturally occurring neuromuscular fatigue. Sports Med. 36, 977–999. doi:10.2165/00007256-200636110-00004
Padulo J., Annino G., Migliaccio G. M., Dʼottavio S., Tihanyi J. (2012). Kinematics of running at different slopes and speeds. J. Strength Cond. Res. 26, 1331–1339. doi:10.1519/JSC.0b013e318231aafa
Padulo J., Powell D., Milia R., Ardigò L. P. (2013). A paradigm of uphill running. PLoS One 8, e69006. doi:10.1371/journal.pone.0069006
Rønnestad B. R., Bakken T. A., Thyli V., Hansen J., Ellefsen S., Hammarstrøm D. (2022). Increasing oxygen uptake in cross-country skiers by speed variation in work intervals. Int. J. Sports Physiol. Perform. 17, 384–390. doi:10.1123/ijspp.2021-0226
Rønnestad B. R., Rømer T., Hansen J. (2019). Increasing oxygen uptake in well-trained cross-country skiers during work intervals with a fast start. Int. J. Sports Physiol. Perform. 1, 383–389. doi:10.1123/ijspp.2018-0360
Schöffl I., Jasinski D., Ehrlich B., Dittrich S., Schöffl V. (2021). Outdoor uphill exercise testing for trail runners, a more suitable method? J. Hum. Kinet. 79, 123–133. doi:10.2478/hukin-2021-0066
Seiler S., Hetlelid K. J. (2005). The impact of rest duration on work intensity and RPE during interval training. Med. Sci. Sports Exerc 37, 1601–1607. doi:10.1249/01.mss.0000177560.18014.d8
Seiler S. (2010). What is best practice for training intensity and duration distribution in endurance athletes? Int. J. sports physiology Perform. 5, 276–291. doi:10.1123/ijspp.5.3.276
Snyder K. L., Farley C. T. (2011). Energetically optimal stride frequency in running: The effects of incline and decline. J. Exp. Biol. 214, 2089–2095. doi:10.1242/jeb.053157
Snyder K. L., Kram R., Gottschall J. S. (2012). The role of elastic energy storage and recovery in downhill and uphill running. J. Exp. Biol. 215, 2283–2287. doi:10.1242/jeb.066332
Stöggl T. L., Sperlich B. (2015). The training intensity distribution among well-trained and elite endurance athletes. Front. Physiology 6. Available at: https://www.frontiersin.org/articles/10.3389/fphys.2015.00295 (Accessed September 14, 2022).
Thevenet D., Tardieu-Berger M., Berthoin S., Prioux J. (2007). Influence of recovery mode (passive vs. active) on time spent at maximal oxygen uptake during an intermittent session in young and endurance-trained athletes. Eur. J. Appl. Physiol. 99, 133–142. doi:10.1007/s00421-006-0327-1
Turnes T., de Aguiar R. A., Cruz R. S. de O., Caputo F. (2016). Interval training in the boundaries of severe domain: Effects on aerobic parameters. Eur. J. Appl. Physiol. 116, 161–169. doi:10.1007/s00421-015-3263-0
van Gent R. N., Siem D., van Middelkoop M., van Os A. G., Bierma-Zeinstra S. M. A., Koes B. W. (2007). Incidence and determinants of lower extremity running injuries in long distance runners: A systematic review. Br. J. Sports Med. 41, 469–480. discussion 480. doi:10.1136/bjsm.2006.033548
Vernillo G., Giandolini M., Edwards W. B., Morin J.-B., Samozino P., Horvais N., et al. (2017). Biomechanics and physiology of uphill and downhill running. Sports Med. 47, 615–629. doi:10.1007/s40279-016-0605-y
Walter S. D., Hart L. E., McIntosh J. M., Sutton J. R. (1989). The Ontario cohort study of running-related injuries. Arch. Intern Med. 149, 2561–2564. doi:10.1001/archinte.149.11.2561
Wenger H. A., Bell G. J. (1986). The interactions of intensity, frequency and duration of exercise training in altering cardiorespiratory fitness. Sports Med. 3, 346–356. doi:10.2165/00007256-198603050-00004
Yokozawa T., Fujii N., Ae M. (2007). Muscle activities of the lower limb during level and uphill running. J. Biomechanics 40, 3467–3475. doi:10.1016/j.jbiomech.2007.05.028
Keywords: incline, intervals, performance, injury, running
Citation: Held S, Rappelt L, Giesen R, Wiedenmann T, Deutsch J-P, Wicker P and Donath L (2023) Increased oxygen uptake in well-trained runners during uphill high intensity running intervals: A randomized crossover testing. Front. Physiol. 14:1117314. doi: 10.3389/fphys.2023.1117314
Received: 06 December 2022; Accepted: 06 February 2023;
Published: 16 February 2023.
Edited by:
Andrea Nicolò, Foro Italico University of Rome, ItalyReviewed by:
Marcel Lemire, University of Upper Alsace, FranceStéphane P Dufour, Université de Strasbourg, France
Copyright © 2023 Held, Rappelt, Giesen, Wiedenmann, Deutsch, Wicker and Donath. This is an open-access article distributed under the terms of the Creative Commons Attribution License (CC BY). The use, distribution or reproduction in other forums is permitted, provided the original author(s) and the copyright owner(s) are credited and that the original publication in this journal is cited, in accordance with accepted academic practice. No use, distribution or reproduction is permitted which does not comply with these terms.
*Correspondence: Pamela Wicker, pamela.wicker@uni-bielefeld.de