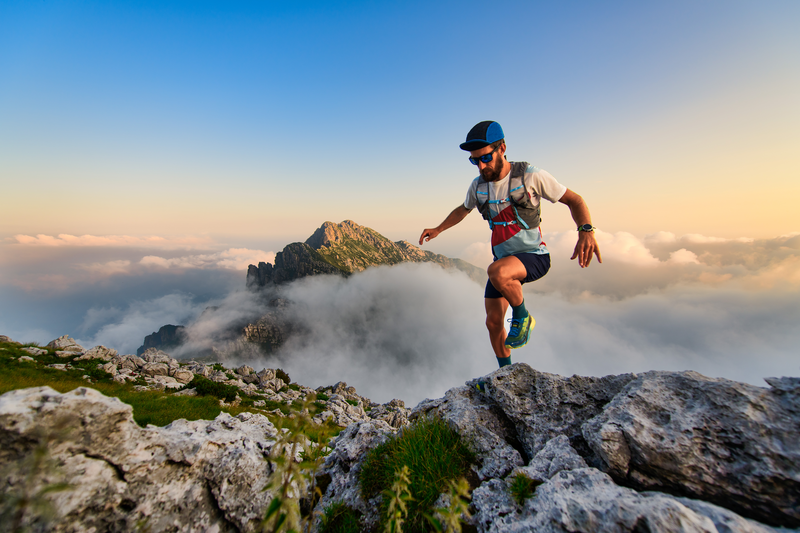
95% of researchers rate our articles as excellent or good
Learn more about the work of our research integrity team to safeguard the quality of each article we publish.
Find out more
BRIEF RESEARCH REPORT article
Front. Physiol. , 21 February 2023
Sec. Cell Physiology
Volume 14 - 2023 | https://doi.org/10.3389/fphys.2023.1113968
Endothelial barrier (EB) disruption contributes to acute lung injury in COVID-19, and levels of both VEGF-A and Ang-2, which are mediators of EB integrity, have been associated with COVID-19 severity. Here we explored the participation of additional mediators of barrier integrity in this process, as well as the potential of serum from COVID-19 patients to induce EB disruption in cell monolayers. In a cohort from a clinical trial consisting of thirty patients with COVID-19 that required hospital admission due to hypoxia we demonstrate that i) levels of soluble Tie2 were increase, and of soluble VE-cadherin were decreased when compared to healthy individuals; ii) sera from these patients induce barrier disruption in monolayers of endothelial cells; and iii) that the magnitude of this effect is proportional to disease severity and to circulating levels of VEGF-A and Ang-2. Our study confirms and extends previous findings on the pathogenesis of acute lung injury in COVID-19, reinforcing the concept that EB is a relevant component of this disease. Our results pave the way for future studies that can refine our understanding of the pathogenesis of acute lung injury in viral respiratory disorders, and contribute to the identification of new biomarkers and therapeutic targets for these conditions.
In COVID-19, acute respiratory distress is mediated by the activation of several components of the inflammatory response in a process referred to as immunothrombosis (Engelmann and Massberg, 2013; Bonaventura et al., 2021). This process involves platelet activation, generation of NETs (neutrophil extracellular traps), tissue factor expression, complement and endothelial activation, which are important elements for pathogen eradication and tissue repair, but that can also mediate tissue damage if activated in a deregulated fashion. Regulation of endothelial barrier (EB) integrity is an essential step of this process, as the disruption of the endothelial line is required for the access of leukocytes to tissues during diapedesis (Seeley et al., 2012).
The physiological mechanisms responsible for EB integrity have been studied for more than a century, with Ernest Starling publishing his model of microvascular fluid dynamics in 1896 which was largely based on differences of oncotic pressure between the intravascular and interstitial space (Starling, 1896; Jacob and Chappell, 2013). During the last century several new concepts were added to our understanding of this process such as the role of the glycocalyx (Michel, 1979) and the discovery of humoral mediators of EB integrity, first identified in studies of angiogenesis during embryonic life (Lomas et al., 1971; Ferrara et al., 2003; Byrne et al., 2005), but later shown to be involved in EB integrity regulation during inflammation. These mediators include VEGF-A and angiopoietins (Ang) 1 and 2, as well as their receptor Tie2 (Gavard and Gutkind, 2006; Schliemann et al., 2006; Park-windhol and Amore, 2016). Interestingly, levels of some of these mediators have been shown to be predictors of disease severity in sepsis (Pickkers et al., 2005; Marx et al., 2013; Page and Liles, 2013). Accordingly, angiogenesis and EB disruption are two intertwined process that can participate in the host response to an infection. Both angiogenesis and EB disruption have been shown to participate in the pathogenesis of COVID-19. The former is evidenced by the clinical observation of intra-alveolar fluid accumulation which is one of the hallmarks of lung pathology in this disease. The latter has been evidenced in human pathology samples showing that intusseptive angiogenesis is present in COVID-19, along with disruption of intercellular junctions (Ackermann et al., 2020), which is also accompanied by evidences of structural destruction of endothelial cell membranes (Magro et al., 2021). Finally, levels of some of the main mediators of EB disruption such as VEGF-A and Ang-2 have been associated with clinical severity in COVID-19 (Pine et al., 2020; Smadja et al., 2020; Rovas et al., 2021; Schmaier et al., 2021; Smadja et al., 2021).
Here we investigated whether levels of components of the Ang/Tie2 pathway and of VEGF-A are associated with EB disruption and with clinical severity in patients with COVID-19.
The study was performed using samples from a previously described population (Henderson et al., 2022), recruited as part of a clinical trial conducted at a tertiary academic hospital from University of Campinas (Mansour et al., 2021). Samples were obtained prior to any intervention, within 24 h from the confirmation of COVID-19 by RT-PCR. Patients were recruited consecutively, and inclusion criteria included the need for hospital admission due to hypoxia and evidence of lung disease in lung CT scans. A group of healthy individuals matched by sex and age was recruited concomitantly from the same geographic area. The study was performed in accordance with the Declaration of Helsinki and approved by the local IRB (protocol CAAE: 36528420.3.0000.5404).
Whole blood samples were collected into EDTA K2 and serum tubes immediately after study enrollment. Samples were processed within 2 h from collection and centrifugation at 2,500 g for 15 min at 22°C for EDTA K2 for plasma, and 1,000 g for 10 min at 10°C for serum. Samples divided in 300 µL aliquots, and immediately frozen at −80°C until analysis.
Clinical and laboratory data were obtained from electronic medical records and from case report forms of the clinical trial.
Plasma levels of EB mediators levels were performed by immunoenzymatic method (ELISA) using commercial kits (R&D) for sTie2 and soluble VE-cadherin (sVEC), and by a customized Luminex immunoassay (Procarta Plex multiplex panel, Thermo-Fischer Scientific) for Ang-1 and VEGF-A; or Ang-2 (Human Angiogenesis/growth factor magnetic bead panel 1 - Merck Millipore), in a Bioplex 200 instrument (Bio-Rad).
Human umbilical vein endothelial cells HUVECs were grown in 75 cm2 flasks in EBM-2 medium supplemented with 10% fetal bovine serum at 37°C in an atmosphere of 5% CO2/95% air. Medium was replaced every 48 h until sub-confluency (approximately 80%–90%) was reached. Human lung endothelial cells (HULECs) were grown in 75 cm2 flasks in DMEM medium supplemented with 10% fetal bovine serum. Cultivation conditions were the same used for HUVECs cells. Medium was replaced every 48 h until reaching the estimated confluence of 80%. All experiments performed on HUVECs and HULECs were between passages 5–7. HUVECs were obtained from Lonza (Walkersville, MD, United States) and HULECs were obtained from ATCC (Manassas, VA, United States).
EB integrity was assessed using ECIS, an electrical cell substrate impedance detection system (ECIS Zθ, Applied BioPhysics, Troy, NY). When added to ECIS arrays and adhered to electrodes, cells behave as insulators, increasing impedance. In monolayers of confluent cells, measured impedance is primarily determined by morphology of the cells, whereas changes in impedance in stimulated cells are caused by changes of EB integrity (Tiruppathi et al., 1992; Szulcek et al., 2014). HUVECs and HULECs were seeded (2.0 × 105 cells/well) and cultured at confluence in eight-well arrays (8WE10+, Applied BioPhysics, Troy, NY) coated with fibronectin (10 μg/mL) specific for this system. After confluence of cell monolayers to the ECIS arrays (normally after 48 h), cells were starved for 2 h, and stimulated with 20% serum from patients or healthy individuals. EB integrity was continuously monitored for 36 hcells.
Data are presented as mean ± standard deviation (SD) or as medians and interquartile range (IQR), as indicated. Differences in continuous variables were analyzed using t-test or Mann-Whitney test for comparison of two variables, or Anova when three or more variables were compared. Data distribution was assessed by the D’Agostino & Pearson normality test. Correlation was calculated using the Pearson or Spearman correlation coefficient. A p-value ≤0.05 was considered statistically significant. All statistical analysis were performed using SPSS version 26 (IBM) or GraphPad Prism 8.0 Software (GraphPad Inc.,).
In total, 30 patients and 30 healthy individuals were included in the study. The characteristics of this cohort have been previously described (Henderson et al., 2022) and are summarized in Table 1.
Patients with COVID-19 presented increased levels of Ang-1, Ang-2, sTie2 and VEGF-A when compared to healthy individuals (p > 0.0001). Patients with COVID-19 had decreased sVEC levels compared to healthy individuals (p = 0.0012) (Figure 1).
FIGURE 1. Plasma levels of (A) Ang-1, (B) Ang-2, (C) sTie2, (D) VEGF-A, and (E) sVEC in patients with COVID-19 and healthy individuals. Horizontal bars indicate median values, and p values are from Mann-Whitney test (n = 28–30 per group).
Correlation analyses demonstrated that levels of some of these EB mediators were associated with clinical and laboratory markers of COVID-19 severity. Specifically, Ang-2 levels were associated with length of intensive care unit (ICU) stay (Rs = 0.409; p = 0.024) and with the extent of lung disease estimated by a CT score (Rs = 0.404; p = 0.026). Similarly, VEGF-A levels were associated with total hospital length of stay (LOS) (Rs = 0.396; p = 0.029) and with the extent of lung disease (Rs = 0.418; p = 0.021) (Figure 2). No additional significant correlation was observed between the other levels of EB mediators and other clinical parameters of disease severity.
FIGURE 2. Correlations of EB integrity mediators with clinical and laboratory markers of COVID-19 severity. (A) Correlation of Ang-2 levels with ICU (intensive care unit) stay; (B) Correlation of Ang-2 levels with a lung CT (computerized tomography) score; (C) Correlation of VEGF-A levels with total hospital length of stay and (D) Correlation of VEGF-A levels with CT score. Spearman correlation coefficient.
We next evaluated whether serum from COVID-19 patients induced changes in EB integrity in monolayers of endothelial cells, using serum from healthy individuals as a control. As shown in Figure 3A, a significant decrease in EB integrity of HUVEC monolayers was evident as early as 15 min after serum stimulation, lasting up to 5 h. Similar data were obtained in HULECs (Figure 3B).
FIGURE 3. In (A) EB integrity of HUVEC monolayers upon stimulation by serum from COVID-19 patients and healthy individuals (n = 27–30 per group). The lower the normalized resistance, the higher the magnitude of EB disruption. Significant differences (* to****) are evident from 15 min to 5 h (Anova corrected for multiple comparisons). (B) EB integrity of HULEC monolayers upon stimulation by serum from COVID-19 patients and healthy individuals (n = 8 per group). Significant differences (* to***) are evident from 15 min to 1 h (Anova corrected for multiple comparisons). In (C) and (D), we shown the correlation of EB disruption with clinically relevant outcomes such days of intensive care unit (ICU) stay and total hospital length of stay respectively. In (E) and (F), Ang-2 and VEGF-A levels was correlated with EB disruption. (n = 57–60 per group). Negative correlations (Spearman test) indicate that the magnitude of EB disruption is associated with worse outcomes. p values: * <0.05; ** <0.01; *** <0.001; **** <0.0001.
Finally, we explored whether the magnitude of EB disruption measured by ECIS was associated with clinically relevant outcomes, and whether it was dependent on circulating levels of mediators of the Ang/Tie2 or VEGF-A pathways. For these analyses we used data obtained 1 h after serum stimulation of endothelial cell monolayers. As shown in Figures 3C, D, significant correlations were observed between the magnitude of EB disruption with length of stay both in the ICU and in as total hospital LOS. Moreover, consistent correlations were observed between EB disruption and serum levels of Ang-2 and VEGF-A (Figures 3E, F).
Both EB disruption and angiogenesis have been shown to contribute to the pathogenesis of inflammatory conditions such as sepsis (Park-windhol and Amore, 2016) and COVID-19 (Ackermann et al., 2020), being considered potential therapeutic targets in these conditions. In this context, the main contribution of our study was the demonstration that i) levels of soluble VEC were decreased, and of soluble Tie2 were increased in COVID-19, and ii) that EB disruption induced by serum from patients with COVID-19 is associated with clinical and laboratory outcomes, and correlated with levels of two key regulators of angiogenesis and EB integrity, Ang-2 and VEGF-A.
The fact that a specific cellular pathway can be both beneficial and detrimental in the pathogenesis of an inflammatory condition forms the basis of the immunothrombosis model (Engelmann and Massberg, 2013). Both EB disruption and angiogenesis are key components of the host response to an infection or tissue damage, as they allow the access of leukocytes to tissues and promote tissue repair (Seeley et al., 2012). Accordingly, circulating levels of mediators of EB integrity and angiogenesis such as VEGF-A and angiopoietins have been associated with the severity of sepsis and septic shock in humans (Alves et al., 2011; Flemming et al., 2015; Seol et al., 2020), and inhibition of these pathways have been shown to improve outcomes in animal models of sepsis and cellular models of COVID-19.
Here we first confirmed previous reports that Ang-2 and VEGF-A levels are increased in COVID-19 (Smadja et al., 2021; Vassiliou et al., 2021), extending these findings to include soluble Tie2 and soluble VEC levels. Significantly higher levels of sTie2 and significantly lower levels of sVEC were demonstrated. The former could be caused either by shedding of this receptor by cellular proteases or by an active secretion process, in a similar mechanism to that described for sFlt-1, in which this soluble form of the VEGF-A receptor functions as a decoy receptor, modulating the effects of its ligand (Kendall et al., 1996). Of note, higher sTie2 levels have been previously described in conditions such as acute myocardial infarction (Wang et al., 2005), acute myeloid leukemia (Attia et al., 2010), neuroendocrine tumors (Melen-Mucha et al., 2012) and obesity (Gozal et al., 2017), among other conditions associated with inflammation. Moreover, sVEC levels were lower in COVID-19, in an interesting contrast with sepsis (Zhang et al., 2010), autoimmune disease (Chen et al., 2014) and atherosclerosis (Soeki et al., 2004), in which this mediator was consistently higher in patients compared to healthy individuals. Of note, while to our knowledge no other study compared sVEC levels in COVID-19 with healthy individuals, a smaller study that compared levels of this mediator between COVID-19 survivors and non-survivors found lower VEC levels in the latter group (Vassiliou et al., 2021). Given the extensive disruption of the alvelo-capillary barrier in COVID-19 compared to sepsis and other inflammatory conditions, and the relevance of VEC internalization in EB regulation (Gavard, 2014), one could speculate that these contrasting results underlie differences in the pathogenesis of acute lung injury in COVID-19, which should be explored in future studies. A possible explanation could be the functional binding of sVEC to an inflamed vasculature and/or circulating cells, analogous to the mechanisms by which extracellular vesicles interact with cells (van Niel et al., 2018). Another notable difference between COVID-19 and sepsis is that the Ang-2/Ang-1 ratio did not increase in the former (data not shown), due to a concomitant and significant increase of the EB-stabilizing mediator Ang-1, observed in our patients. This pattern could represent a response to endothelial damage, and has been described by other authors in COVID-19 (Kaur et al., 2021; Hultström et al., 2022).
Our results also confirm previous reports about the association of Ang-2 and VEGF-A levels with clinical markers of disease severity in COVID-19, namely, length of ICU stay and extent of lung disease (Pine et al., 2020; Smadja et al., 2020; Hultström et al., 2022).
In addition, we explored the effect of sera from COVID-19 patients on EB integrity measured by ECIS, which is considered the gold standard for the in vitro exploration of EB integrity and has been used in key studies of EB biology (Moy et al., 2000; Umapathy et al., 2017; Robilliard et al., 2018). A significant decrease in EB integrity was observed as early as 15 min after serum stimulation, lasting until 5 h thereafter. The reversal of EB disruption after this time-points confirms the transient and functional (i.e., not associated with endothelial cell death) nature of this effect, which has also been observed in studies of sepsis and other inflammatory conditions using this model. The same pattern was confirmed in human lung microvascular endothelial cells, although the effect of serum was slightly less intense and more rapidly reversed. Although the biological relevance of these minor discrepancies cannot be confirmed, one possible explanation is the fact that EB in lungs have been reported to be relatively tighter than other organs (Claesson-Welsh et al., 2021). In fact, HUVECs have been shown to present a higher basal permeability than lung microvascular endothelial cells (van der Heijden et al., 2011). Finally, significant correlations were observed between the magnitude of EB disruption in this assay and clinical markers of disease severity, namely, length of hospital, and ICU stay. The effect of plasma from COVID-19 patients on EB integrity was explored for a recent study showed that effects of plasma from healthy subjects compared to moderate or severe COVID-19 cases resulted in increased endothelial permeability, causing loss of junctional VEC and formation of intercellular gaps within hours of exposure. Loss of junctional proteins has also been demonstrated in human lung tissue (Michalick et al., 2020).
Finally, we also report that the magnitude of this effect is associated with circulating levels of both Ang-2 and VEGF-A, which are well-known mediators of EB integrity during angiogenesis and inflammation both in vitro and in vivo (Folkman and Shing, 1992). Together our results reinforce the concept that EB is a relevant component of the pathogenesis of acute lung injury in COVID-19, and warrant additional mechanistic and interventional studies exploring the role of this process and their mediators in the pathogenesis and treatment of COVID-19 and other forms of viral acute lung injury.
Our study has limitations that need to be acknowledged. First, the sample size is relatively small, due to the limitation for recruitment in the clinical trial from which samples were obtained. On the other hand, the use of data from a clinical trial allowed us to obtain a consecutively recruited sample and high quality samples and clinical/outcome data. Moreover, sample size allowed us to demonstrate a series of relevant changes. Second, as an exploratory study, our results only demonstrate associations, which are not necessarily causal, and need to be explored in future studies, specifically designed for this purpose, addressing the cellular and molecular mechanisms of EB disruption in COVID-19.
In conclusion, levels of EB mediators are modulated in COVID-19, and associated with relevant clinical outcomes of disease severity. Moreover, we demonstrate for the first time that sTie and sVEC are also modulated (up- and down-regulated, respectively) in these patients. Finally, serum from these patients is capable to induce EB disruption in monolayers of endothelial cells, in a magnitude that is proportional to both disease severity and to circulating levels of Ang-2 and VEGF-A.
The original contributions presented in the study are included in the article/Supplementary Material, further inquiries can be directed to the corresponding author.
The studies involving human participants were reviewed and approved by the Ethics and Research Committe—University of Campinas. The patients/participants provided their written informed consent to participate in this study.
CM obtained and processes samples and performed multiplex assays, contributed to data analysis and drafted the manuscript. IB-J performed endothelial cell assays and contributed to data analysis. FD obtained and processed samples and contributed to data analysis. JS performed multiplex assays. BB and AP recruited and managed patients and obtained patient/outcome data. EM and LV designed and conducted the clinical trial from which patients were recruited. FO contributed to study design and data analysis. FC provided endothelial cells for the study and data analysis. ED designed the study, obtained and processed samples, oversaw and provided resources and infrastructure for the assays, reviewed, analyzed data, and drafted the manuscript. All authors contributed to the article and approved the submitted version.
The author(s) disclosed receipt of the following financial support for the research, authorship, and/or publication of this article: This study was financially supported by the Sao Paulo Research Foundation (FAPESP), grants # 2014/14172-6 and 2020/05985-9; Fundo de Apoio ao Ensino, à pesquisa e Extensao (FAEPEX-UNICAMP) 2404/2020 and Coordenacao de Aperfeicoamento de Pessoal de Nivel Superior—Brasil (CAPES)—Finance Code 001.
The authors declare that the research was conducted in the absence of any commercial or financial relationships that could be construed as a potential conflict of interest.
All claims expressed in this article are solely those of the authors and do not necessarily represent those of their affiliated organizations, or those of the publisher, the editors and the reviewers. Any product that may be evaluated in this article, or claim that may be made by its manufacturer, is not guaranteed or endorsed by the publisher.
Ackermann, M., Verleden, S. E., Kuehnel, M., Haverich, A., Welte, T., Laenger, F., et al. (2020). Pulmonary vascular endothelialitis, thrombosis, and angiogenesis in Covid-19. N. Engl. J. Med. 1–9, 120–128. doi:10.1056/NEJMoa2015432
Alves, B. E., Montalvao, S. A. L., Aranha, F. J. P., Lorand-Metze, I., de Souza, C. A., Annichino-Bizzacchi, J. M., et al. (2011). Time-course of sFlt-1 and VEGF-A release in neutropenic patients with sepsis and septic shock: A prospective study. J. Transl. Med. 9, 23–28. doi:10.1186/1479-5876-9-23
Attia, M. A., Hazzaa, S. M., Essa, S. A., and Seleim, M. F. (2010). Prognostic value of soluble angiopoietin-2 and soluble Tie-2 in Egyptian patients with acute myeloid leukemia. Turkish J. Hematol. 27 (4), 282–288. doi:10.5152/tjh.2010.50
Bonaventura, A., Vecchié, A., Dagna, L., Martinod, K., Dixon, D. L., van Tassell, B. W., et al. (2021). Endothelial dysfunction and immunothrombosis as key pathogenic mechanisms in COVID-19. Nat. Rev. Immunol. 21, 319–329. doi:10.1038/s41577-021-00536-9
Byrne, A. M., Bouchier-Hayes, D. J., and Harmey, J. H. (2005). Angiogenic and cell survival functions of vascular endothelial growth factor (VEGF). J. Cell Mol. Med. 9 (4), 777–794. doi:10.1111/j.1582-4934.2005.tb00379.x
Chen, T., Guo, Z. P., Cao, N., Qin, S., Li, M. M., and Jia, R. Z. (2014). Increased serum levels of soluble vascular endothelial-cadherin in patients with systemic vasculitis. Rheumatol. Int. 34 (8), 1139–1143. doi:10.1007/s00296-014-2949-7
Claesson-Welsh, L., Dejana, E., and McDonald, D. M. (2021). Permeability of the endothelial barrier: Identifying and reconciling controversies. Trends Mol. Med. 27, 314–331. doi:10.1016/j.molmed.2020.11.006
Engelmann, B., and Massberg, S. (2013). Thrombosis as an intravascular effector of innate immunity. Nat. Rev. Immunol. 13 (1), 34–45. doi:10.1038/nri3345
Ferrara, N., peter, G. H., and Lecouter, J. (2003). The biology of VEGF and its receptors. Nat. Med. 9 (6), 669–676. doi:10.1038/nm0603-669
Flemming, S., Burkard, N., Renschler, M., Vielmuth, F., Meir, M., Schick, M. A., et al. (2015). Soluble VE-cadherin is involved in endothelial barrier breakdown in systemic inflammation and sepsis. Cardiovasc Res. 107 (1), 32–44. doi:10.1093/cvr/cvv144
Folkman, J., and Shing, Y. (1992). Angiogenesis. J. Biol. Chem. 267, 10931–10934. doi:10.1016/s0021-9258(19)49853-0
Gavard, J. (2014). Endothelial permeability and VE-cadherin: A wacky comradeship. Cell Adh Migr. 8, 158–164. doi:10.4161/cam.29026
Gavard, J., and Gutkind, J. S. (2006). VEGF controls endothelial-cell permeability by promoting the β-arrestin-dependent endocytosis of VE-cadherin. Nat. Cell Biol. 8 (11), 1223–1234. doi:10.1038/ncb1486
Gozal, D., Khalyfa, A., Qiao, Z., Smith, D. L., Philby, M. F., Koren, D., et al. (2017). Angiopoietin-2 and soluble Tie-2 receptor plasma levels in children with obstructive sleep apnea and obesity. Obesity 25 (6), 1083–1090. doi:10.1002/oby.21859
Henderson, M. W., Lima, F., Moraes, C. R. P., Ilich, A., Huber, S. C., Barbosa, M. S., et al. (2022). Contact and intrinsic coagulation pathways are activated and associated with adverse clinical outcomes in COVID-19. Blood Adv. 6 (11), 3367–3377. doi:10.1182/bloodadvances.2021006620
Hultström, M., Fromell, K., Larsson, A., Persson, B., Nilsson, B., Quaggin, S. E., et al. (2022). Angiopoietin-2 inhibition of thrombomodulin-mediated anticoagulation—a novel mechanism that may contribute to hypercoagulation in critically ill COVID-19 patients. Biomedicines 10 (6), 1333. doi:10.3390/biomedicines10061333
Jacob, M., and Chappell, D. (2013). Reappraising starling: The physiology of the microcirculation. Curr. Opin. Crit. Care 19 (4), 282–289. doi:10.1097/MCC.0b013e3283632d5e
Kaur, S., Hussain, S., Kolhe, K., Kumar, G., Tripathi, D. M., Tomar, A., et al. (2021). Elevated plasma ICAM1 levels predict 28-day mortality in cirrhotic patients with COVID-19 or bacterial sepsis. JHEP Rep. 3 (4), 100303. doi:10.1016/j.jhepr.2021.100303
Kendall, R. L., Wang, G., and Thomas, K. A. (1996). Identification of a natural soluble form of the vascular endothelial growth factor receptor, FLT-1, and its heterodimerization with KDR. Biochimical Biophysical Res. Commun. 226, 324–328. doi:10.1006/bbrc.1996.1355
Lomas, J., Anderson, G. M., Domnick-Pierre, K., Vayda, E., and Enkin, M. W. (1971). Tumor angiogenesis: Therapeutic implications. N. Engl. J. Med. 285 (21), 1182–1186. doi:10.1056/nejm197111182852108
Magro, C. M., Mulvey, J., Kubiak, J., Mikhail, S., Suster, D., Crowson, A. N., et al. (2021). Severe COVID-19: A multifaceted viral vasculopathy syndrome. Ann. Diagn Pathol. 50, 151645. doi:10.1016/j.anndiagpath.2020.151645
Mansour, E., Palma, A. C., Ulaf, R. G., Ribeiro, L. C., Bernardes, A. F., Nunes, T. A., et al. (2021). Safety and outcomes associated with the pharmacological inhibition of the kinin – kallikrein system in severe COVID-19. Viruses 13, 309–316. doi:10.3390/v13020309
Marx, M., Fiusa, L., Costa-lima, C., Souza, G. R. de, Vigorito, A. C., Jose, F., et al. (2013). A high angiopoietin-2/angiopoietin-1 ratio is associated with a high risk of septic shock in patients with febrile neutropenia. Crit. Care Med. 17, 1–10. doi:10.1186/cc12848
Melen-Mucha, G., Niedziela, A., Mucha, S., Motylewska, E., Lawnicka, H., Komorowski, J., et al. (2012). Elevated peripheral blood plasma concentrations of tie-2 and angiopoietin 2 in patients with neuroendocrine tumors. Int. J. Mol. Sci. 13 (2), 1444–1460. doi:10.3390/ijms13021444
Michalick, L., Weidenfeld, S., Grimmer, B., Fatykhova, D., Daniel, P., Behrens, F., et al. (2020). Plasma mediators in patients with severe COVID-19 cause lung endothelial barrier failure. Eur. Respir. J. 1–5, 2002384. doi:10.1183/13993003.02384-2020
Michel, C. C. (1979). The investigation of capillary permeability in single vessels. Acta Physiol. Scand. 106, 67.
Moy, A. B., Winter, M., Kamath, A., Blackwell, K., Reyes, G., Giaever, I., et al. (2000). Histamine alters endothelial barrier function at cell-cell and cell-matrix sites. Am. J. Physiol. Lung Cell Mol. Physiol. 278 (5 22-5), L888–L898. doi:10.1152/ajplung.2000.278.5.L888
Page, A. V., and Liles, W. C. (2013). Biomarkers of endothelial activation/dysfunction in infectious diseases. Virulence 4, 507–516. doi:10.4161/viru.24530
Park-windhol, C., and Amore, P. A. D. (2016). Disorders of vascular permeability. Annu. Rev. Pathol. Mech. Dis. 11, 251–281. doi:10.1146/annurev-pathol-012615-044506
Pickkers, P., Sprong, T., Eijk, L. van, Hoeven, H. van der, Smits, P., and Deuren, M. van (2005). Vascular endothelial growth factor is increased during the first 48 hours of human septic shock and correlates with vascular permeability. Shock 24 (6), 508–512. doi:10.1097/01.shk.0000190827.36406.6e
Pine, A. B., Meizlish, M. L., Goshua, G., hong, C. C., Zhang, H., Bishai, J., et al. (2020). Circulating markers of angiogenesis and endotheliopathy in COVID-19. Pulm. Circ. 10, 1–4. doi:10.1177/2045894020966547
Robilliard, L. D., Kho, D. T., Johnson, R. H., Anchan, A., O’Carroll, S. J., and Graham, E. S. (2018). The importance of multifrequency impedance sensing of endothelial barrier formation using ECIS technology for the generation of a strong and durable paracellular barrier. Biosens. (Basel). 8 (3), 64. doi:10.3390/bios8030064
Rovas, A., Osiaevi, I., Buscher, K., Sackarnd, J., Robin, P., Manfred, T., et al. (2021). Microvascular dysfunction in COVID-19: The MYSTIC study. Angiogenesis 24, 145–157. doi:10.1007/s10456-020-09753-7
Schliemann, C., Bieker, R., Padro, T., Kessler, T., Hintelmann, H., Buchner, T., et al. (2006). Expression of angiopoietins and their receptor Tie2 in the bone marrow of patients with acute myeloid leukemia. Haematologica 91 (9), 1203–1211. doi:10.1182/blood.v108.11.1894.1894
Schmaier, A. A., Hurtado, G. M. P., Manickas-Hill, Z. J., Sack, K. D., Chen, S. M., Bhambhani, V., et al. (2021). Tie2 activation protects against prothrombotic endothelial dysfunction in COVID-19. JCI Insight 6, e151527–e151535. doi:10.1172/jci.insight.151527
Seeley, E. J., Matthay, M. A., and Wolters, P. J. (2012). Inflection points in sepsis biology: From local defense to systemic organ injury. Am. J. Physiol. Lung Cell Mol. Physiol. 303 (5), 355–363. doi:10.1152/ajplung.00069.2012
Seol, C. H., Yong, S. H., Shin, J. H., Lee, S. H., Leem, A. Y., Park, S. M., et al. (2020). The ratio of plasma angiopoietin-2 to angiopoietin-1 as a prognostic biomarker in patients with sepsis. Cytokine 129, 155029. doi:10.1016/j.cyto.2020.155029
Smadja, D. M., Guerin, C. L., Chocron, R., Yatim, N., Boussier, J., Gendron, N., et al. (2020). Angiopoietin-2 as a marker of endothelial activation is a good predictor factor for intensive care unit admission of COVID-19 patients. Angiogenesis 23 (4), 611–620. doi:10.1007/s10456-020-09730-0
Smadja, D. M., Philippe, A., Bory, O., Gendron, N., Beauvais, A., Gruest, M., et al. (2021). Placental growth factor level in plasma predicts COVID-19 severity and in-hospital mortality. J. Thromb. Haemost. 19, 1823–1830. doi:10.1111/jth.15339
Soeki, T., Tamura, Y., Shinohara, H., Sakabe, K., Onose, Y., and Fukuda, N. (2004). Elevated concentration of soluble vascular endothelial cadherin is associated with coronary atherosclerosis. Circulation J. 68 (1), 1–5. doi:10.1253/circj.68.1
Starling, E. H. (1896). On the absorption of fluids from the connective tissue spaces. J. Physiol. 19 (4), 312–326. doi:10.1113/jphysiol.1896.sp000596
Szulcek, R., Bogaard, H. J., and van Nieuw Amerongen, G. P. (2014). Electric cell-substrate impedance sensing for the quantification of endothelial proliferation, barrier function, and motility. J. Vis. Exp. 2014(85). doi:10.3791/51300-v
Tiruppathi, C., Malik, A. B., del Vecchio, P. J., Keese, C. R., and Glaever, I. (1992). Electrical method for detection of endothelial cell shape change in real time: Assessment of endothelial barrier function. Proc. Natl. Acad. Sci. U. S. A. 89 (17), 7919–7923. doi:10.1073/pnas.89.17.7919
Umapathy, N. S., Gonzales, J., Makala, L. H., Xu, H., Biddinger, P., and Pace, B. S. (2017). Impaired pulmonary endothelial barrier function in sickle cell mice. Haematologica 102, e26. doi:10.3324/haematol.2016.153098
van der Heijden, M., van Nieuw Amerongen, G. P., van Bezu, J., Paul, M. A., Groeneveld, A. B. J., and van Hinsbergh, V. W. M. (2011). Opposing effects of the angiopoietins on the thrombin-induced permeability of human pulmonary microvascular endothelial cells. PLoS One 6 (8), e23448. doi:10.1371/journal.pone.0023448
van Niel, G., D’Angelo, G., and Raposo, G. (2018). Shedding light on the cell biology of extracellular vesicles. Nat. Rev. Mol. Cell Biol. 19, 213–228. doi:10.1038/nrm.2017.125
Vassiliou, A. G., Keskinidou, C., Jahaj, E., Gallos, P., Dimopoulou, I., Kotanidou, A., et al. (2021). ICU admission levels of endothelial biomarkers as predictors of mortality in critically ill COVID-19 patients. Cells 10 (1), 186–213. doi:10.3390/cells10010186
Wang, C., Fu, P., Li, H., Gao, R., and Xiu, R. (2005). Soluble angiopoietin receptor Tie-2 in patients with acute myocardial infarction and its effects on angiogenesis. Clin. Hemorheol. Microcirc. 33 (1), 1–10.
Keywords: endothelial barrier, COVID-19, angiogenesis, angiopoietin, VEGFA
Citation: Moraes CRP, Borba-Junior IT, De Lima F, Silva JRA, Bombassaro B, Palma AC, Mansour E, Velloso LA, Orsi FA, Costa FTM and De Paula EV (2023) Association of Ang/Tie2 pathway mediators with endothelial barrier integrity and disease severity in COVID-19. Front. Physiol. 14:1113968. doi: 10.3389/fphys.2023.1113968
Received: 01 December 2022; Accepted: 07 February 2023;
Published: 21 February 2023.
Edited by:
Amy L. Ryan, The University of Iowa, United StatesReviewed by:
Ronan Padraic Murphy, Dublin City University, IrelandCopyright © 2023 Moraes, Borba-Junior, De Lima, Silva, Bombassaro, Palma, Mansour, Velloso, Orsi, Costa and De Paula. This is an open-access article distributed under the terms of the Creative Commons Attribution License (CC BY). The use, distribution or reproduction in other forums is permitted, provided the original author(s) and the copyright owner(s) are credited and that the original publication in this journal is cited, in accordance with accepted academic practice. No use, distribution or reproduction is permitted which does not comply with these terms.
*Correspondence: Erich Vinicius De Paula, ZXJpY2hAdW5pY2FtcC5icg==
†These authors have contributed equally to this work and share first authorship
Disclaimer: All claims expressed in this article are solely those of the authors and do not necessarily represent those of their affiliated organizations, or those of the publisher, the editors and the reviewers. Any product that may be evaluated in this article or claim that may be made by its manufacturer is not guaranteed or endorsed by the publisher.
Research integrity at Frontiers
Learn more about the work of our research integrity team to safeguard the quality of each article we publish.