- 1Department of Obstetrics and Gynecology, The Affiliated Hospital of Guizhou Medical University, Guiyang, China
- 2Transformation Engineering Research Center of Chronic Disease Diagnosis and Treatment, Department of Physiology, College of Basic Medicine, Guizhou Medical University, Guiyang, Guizhou, China
- 3Prenatal Diagnosis Center in Guizhou Province, The Affiliated Hospital of Guizhou Medical University, Guiyang, China
- 4College of Basic Medicine, Guizhou Medical University, Guiyang, Guizhou, China
- 5Guizhou Provincial Key Laboratory of Pathogenesis and Drug Research on Common Chronic Diseases, Department of Pathophysiology, School of Basic Medical Sciences, Guizhou Medical University, Guiyang, Guizhou, China
- 6Guizhou Institute of Precision Medicine, Affiliated Hospital of Guizhou Medical University, Guiyang, China
- 7State Key Laboratory of Agrobiotechnology, College of Biological Sciences, China Agricultural University, Beijing, China
Primordial follicles are the starting point of follicular development and the basic functional unit of female reproduction. Primordial follicles are formed around birth, and most of the primordial follicles then enter a dormant state. Since primordial follicles are limited in number and can’t be renewed, dormant primordial follicles cannot be reversed once they enter the growing state. Thus, the orderly occurrence of primordial follicles selective activation directly affects the rate of follicle consumption and thus determines the length of female reproductive lifespan. Studies have found that appropriately inhibiting the activation rate of primordial follicles can effectively slow down the rate of follicle consumption, maintain fertility and delay ovarian aging. Based on the known mechanisms of primordial follicle activation, primordial follicle in vitro activation (IVA) technique has been clinically developed. IVA can help patients with premature ovarian failure, middle-aged infertile women, or infertile women due to gynecological surgery treatment to solve infertility problems. The study of the mechanism of selective activation of primordial follicles can contribute to the development of more efficient and safe IVA techniques. In this paper, recent mechanisms of primordial follicle activation and its clinical application are reviewed.
Introduction
Ovary is an important reproductive and endocrine organ for female mammal. The normal ovarian function provides a fundamental guarantee for the body’s suitable reproductive life and stable endocrine environment. There are two types of follicles in the adult ovarian follicle pool, one is the growing follicle, and the other is large number of primordial follicles as ovarian reserve. The primordial follicle pool is not renewable, and the primordial follicle cannot be reversed once it enters the growing state (Zhang et al., 2015; Zhang and Liu, 2015; Kallen et al., 2018). Therefore, the orderly primordial follicle activation plays a decisive role in maintaining the length of female reproductive life (Reddy et al., 2010; Zhao et al., 2021). There are different stages during follicles development included primordial follicle, primary follicle, secondary follicle, antral follicle and preovulatory follicles in the ovary, but most of the follicles are primordial follicles, and these primordial follicles are in a dormant and static state (Pedersen, 1970; Hsueh et al., 2015; Zhang et al., 2015; Monget et al., 2021) (Figure 1). Primordial follicles consist of a single central oocyte surrounded by multiple pre-granulosa cells. Interestingly, the oocytes are arrest in the first meiosis stage and their growth is relatively static, the cell cycle of the pre-granulosa cells is inhibited (Jaffe and Egbert, 2017; Granados-Aparici et al., 2019). This state can be maintained as long as a year in mice, and up to 50 years in humans. These dormant primordial follicles are recruited from the primordial follicle pool and enter the growth follicle stage. This process is named the initial recruitment, also called primordial follicle activation (Lintern-Moore and Moore, 1979). Primordial follicle initial recruitment is different from cyclic recruitment (Kallen et al., 2018). It is generally believed that cyclic recruitment is regulated by gonadotropins, while initial recruitment is not regulated by gonadotropins (McGee and Hsueh, 2000; Bian et al., 2021). Primordial follicle initial recruitment is mainly regulated by the signals in the pre-granulosa cells and oocyte, as well as by conditions such as growth factors and stress in the primordial follicle microenvironment (Bian et al., 2021). After the primordial follicle is activated, the pre-granulosa cells gradually change from flat to wedge-shaped, then cuboidal, and later called granulosa cells. Meanwhile, oocyte diameter increased (Kallen et al., 2018) (Figure 2). When the primordial follicle is activated to enter the growth follicle stage, it enters the irreversible growth and development process, so the activation of the primordial follicle is equivalent to a gate for follicular development (Hirshfield, 1991; Adhikari et al., 2009). In order to maintain a suitable length of reproductive life and the reproductive health of the body, primordial follicles in the ovaries need to be properly activated at the right time (Zhang and Liu, 2015; Chen et al., 2022). The understanding of the mechanisms of primordial follicle activation is still limited. To better grasp the progress of research on primordial follicle activation, we summarize the currently known key networks that regulate the activation of primordial follicles in this review.
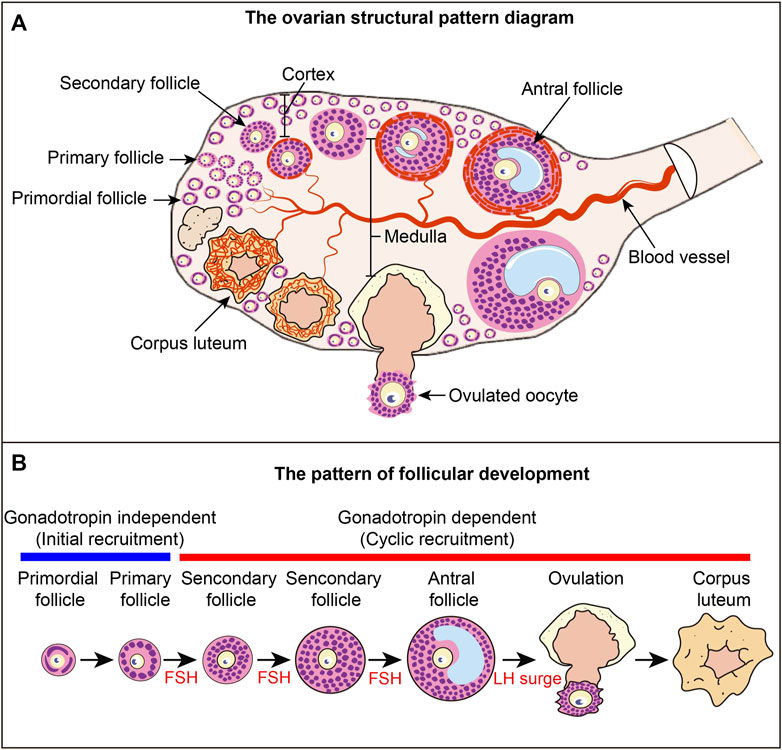
FIGURE 1. Ovarian structure and follicular development pattern diagram (A) Ovarian structural pattern diagram. Follicles at different developmental stages constitute the basic structural and functional unit of the ovary. Primordial follicles are mainly distributed in the cortex, and growing follicles are mainly distributed in the medulla. The blood vessels in the ovary provide nutrients to the ovarian tissue and follicles, and the blood vessels around each follicle are relatively independent. (B) Follicular development pattern. The development of primordial follicles into primary follicles is independent of gonadotropins, a process called primordial follicle activation, also known as initial recruitment. The process from primary follicle development to ovulation is a gonadotropin-dependent stage. The process of primary follicle development into preantral follicles is called cyclic recruitment and is the FSH response phase. Antral follicles ovulate under the stimulation of the LH surge. After ovulation, the granulosa cells luteinize to form the corpus luteum.
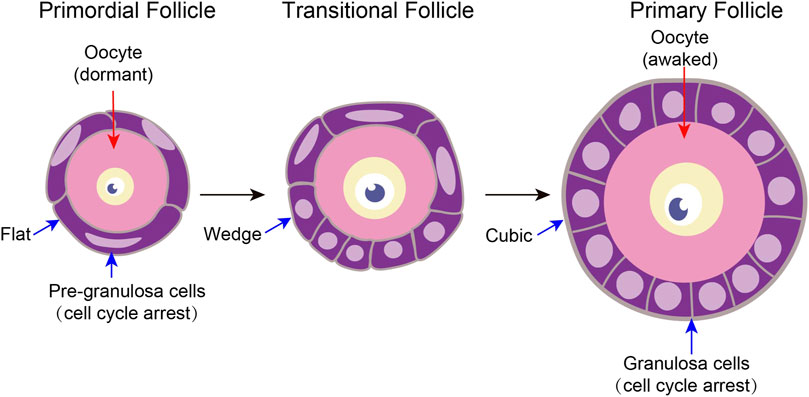
FIGURE 2. Characteristics of tissue structure changes in primordial follicle activation A follicle consists of a single oocyte in the middle and several somatic cells surrounding it. Primitive follicle activation mainly has two characteristics of structural changes. On the one hand, after primordial follicle activation, the pre-granulosa cells slowly changed from flat to wedge-shaped, and then to cubic-shaped. On the other hand, the oocyte diameter increased.
Two waves of primordial follicles activation in mouse
Primordial follicles are formed around birth. The primordial follicles in the ovarian medulla are synchronously activated to become the first wave of activated follicles. The dormant primordial follicles in the cortical region of the ovary are gradually activated into a second wave of activated follicles (Hirshfield, 1991; Mork et al., 2012). Primordial follicles are mainly stored in the cortical area, and growing follicles are mainly stored in the medullary area. The developmental dynamics, functions and mechanisms of the two waves of follicles are different (Zheng et al., 2014; Dai et al., 2022). The activation of the first wave primordial follicles in the medulla is regulated by oocytes and has been determined during the formation of primordial follicles in the embryonic stage (Dai et al., 2022). However, the activation of the second wave primordial follicles in the medulla of the adult ovary may be regulated by pre-granulosa cells (Zhang et al., 2014). The first wave of primordial follicle development later contributes to the onset of puberty. The second wave of primordial follicles contributes to the entire reproductive process in adulthood (Zheng et al., 2014). The current understanding of the mechanism of the two waves of primordial follicle activation is limited, and this will be a fundamental scientific topic that needs attention in the development of primordial follicles.
The signal pathway in oocyte
PTEN-PI3K-AKT signaling
Primordial follicles are composed of only two types of cells: oocytes and pre-granulosa cells. The activation of primordial follicles requires the participation of these two types of cells (Zhang et al., 2015). In the process of primordial follicle activation, two signaling pathways, the phosphatidylinositol-3 kinase (PI3K) signaling pathway and the mechanistic target of rapamycin complex (mechanistic target of rapamycin complex 1, mTORC1) signaling pathway play a key role. PI3K signaling in oocytes is required for primordial follicles to maintain dormant state and follicular reserve (Adhikari and Liu, 2009; Adhikari and Liu, 2010; Zhang et al., 2014; Maidarti et al., 2020; Zhao et al., 2021). Phosphatase and tensin homolog (PTEN) negatively regulate intracellular levels of phosphatidylinositol-3,4,5-trisphosphate (PIP3) in cells and functions as a tumor suppressor by negatively regulating protein kinase B (PKB/AKT) signaling pathway (Worby and Dixon, 2014; Yehia et al., 2020). PTEN-PI3K-AKT is a relatively well-studied and clear signaling pathway during primordial follicle activation (Reddy et al., 2005; Liu et al., 2006). PTEN is mainly localized in dormant primordial follicle oocytes, deletion of Pten in primordial follicle oocytes will lead to excessive activation of the PI3K signaling pathway in oocytes, leading to premature activation of primordial follicles and ultimately premature ovarian failure (Reddy et al., 2008). Pyruvate dehydrogenase kinase 1 (PDK1) activates AKT through co-binding to PIP3 generated by PI3Ks (Gagliardi et al., 2018). Conditional knockout of Pdk1 in primordial follicle oocytes results that the majority of primordial follicles are depleted around the onset of sexual maturity. PTEN-PDK1 signaling in oocytes that controls the survival, loss and activation of primordial follicles (Reddy et al., 2009).
TSC1/TSC2-mTOR signaling in oocyte
mTOR is essential for oogenesis, follicular development, maintenance of follicular reserve, and oocyte maturation (Liu et al., 2018; Correia et al., 2020). TSC complex subunit 1 (TSC1) and TSC complex subunit 2 (TSC2) negatively regulates mammalian target of rapamycin complex 1 (mTORC1) signaling. mTOR is a conserved kinase that mediate cellular responses to stresses such as nutrient deprivation, growth factors and DNA damage (Salussolia et al., 2019). Deletion of Tsc1/2 in primordial follicle oocytes will lead to overactivation of the mTOR signaling pathway in oocytes, which will also lead to the premature activation of primordial follicles and eventually lead to premature ovarian failure (Adhikari et al., 2009). However, primordial follicles are normally activated in the absence of mTOR in primordial follicle oocytes, but subsequent follicle development is arrested, and granulosa cells transform into sertoli-like cells (Guo et al., 2018).
LHX8
LIM homeobox 8 (LHX8) is a member of the LIM homeobox family of proteins (Hobert and Westphal, 2000). In the ovary, LHX8 is specifically expressed in the oocyte nucleus and involved in oogenesis, oocytes differentiation, primordial follicle activation (Choi et al., 2008; Zhao et al., 2022). Lhx8-null mice had abnormally increased level of autophagy in oocytes and increased oocyte DNA damage, resulting in massive oocyte loss (Ren et al., 2015; D’Ignazio et al., 2018). Wang’s study found that the oocyte-specific transcription factors LHX8, FIGLA and SOHLH1 form a transcriptional regulatory network to regulate oogenesis (Wang et al., 2020b). LHX8 directly regulates Lin28a transcription in primordial follicle oocytes to regulate postnatal folliculogenesis (Ren et al., 2015; D’Ignazio et al., 2018).
CDC42
Cell Division Cycle 42 (CDC42) is a small GTPase of the Rho-subfamily, which regulates signaling pathways that control diverse cellular functions including cell morphology, migration, endocytosis and cell cycle progression (Heinrich et al., 2021; Campbell et al., 2022; Wirth et al., 2022). The subcellular localization of CDC42 during primordial follicle activation is interesting. In dormant primordial follicles, CDC42 is specifically expressed in the oocyte cytoplasm. When primordial follicles are activated, the expression of CDC42 on the oocyte membrane is greatly enhanced, the expression of the GTP-active form of CDC42 is enhanced on the oocyte membrane. CDC42 binds to P110-β protein, regulates the activation of PI3K signaling pathway in oocytes, and promotes primordial follicle activation (Yan et al., 2018). In Yan’s study, it was found that the expressions of CDC42 and PTEN in primordial follicle oocytes are mutually exclusive, but the specific regulatory relationship between CDC42 and PTEN is not clear, which needs to be explored in future research.
E-cadherin
Cell adhesion is essential for tissue structure and function. The cadherin family members play a key role in cell-cell recognition and adhesion and interact with intracytoplasmic proteins through adaptor proteins (Collins et al., 2017). E-cadherin, a classical cadherin of the cadherin superfamily, is a calcium-dependent cell adhesion molecule that is involved in the establishment and maintenance of epithelial cell morphology during embryogenesis and adulthood (Zaidel-Bar, 2013). E-cadherin is specifically localized to the cytomembrane of oocytes in primordial follicle. E-cadherin in primordial follicle oocytes plays an indispensable role in the maintenance of the primordial follicle pool by facilitating follicular structural stability and regulating NOBOX expression (Yan et al., 2019). The study also demonstrates that oocyte-derived factors are necessary for the maintenance of follicles.
The signal pathway in pre-granulosa cells
TSC1/TSC2-mTOR signaling in pre-granulosa cells
Interestingly, primordial follicles failed to be activated after deletion of Rptor, a key member of the mTORC1 complex in pre-granulosa cells, and primordial follicles were hyperactivated after deletion of TSC1/2 (Zhang et al., 2014). Studies using multiple transgenic mouse models reveal that pre-granulosa cells initiate and govern the activation of the second wave of primordial follicles (Zhang et al., 2014; Zhang et al., 2015). Under the stimulation of surrounding factors such as hypoxia, nutritional factors, stress and other factors, mTOR in pre-granulosa cells is upregulated, pre-granulosa cells grow and differentiate into granulosa cells, and they secrete more KIT ligands at the same time (Zhang et al., 2014). KIT ligands bind to KIT receptors on the oocyte membrane and activate the PI3K signaling pathway in the oocyte (Kissel et al., 2000; Nilsson and Skinner, 2004; Hutt et al., 2006; Zhang et al., 2014; Saatcioglu et al., 2016). This enables downstream FOXO3A to be phosphorylated, and FOXO3a is transported out of the nucleus to relieve the inhibition of oocyte growth, thereby enabling primordial follicle activation (Castrillon et al., 2003; Zhang et al., 2014; Ezzati et al., 2015). Other studies have also found that CREB, MAPK, HDAC6, NGF and other molecules can regulate the activation of primordial follicles through the mTOR signaling pathway (He et al., 2017; Zhao et al., 2018; Li et al., 2020; Zhang et al., 2021b; Zhang et al., 2022). These studies further illustrate the important role of the mTOR signaling pathway in the activation of primordial follicles.
FOXL2
Foxl2 forkhead box L2 (FOXL2), a forkhead transcription factor, contains a fork-head DNA-binding domain and it may play a role in ovarian development and function (Benayoun et al., 2011; Georges et al., 2013). Expansion of a polyalanine repeat region and other mutations in FOXL2 are a cause of blepharophimosis syndrome, premature ovarian failure and granulosa cell tumour (De Baere et al., 2002; Nallathambi et al., 2007; Pierini et al., 2020). The formation of primordial follicles in Foxl2 knockout mice was not affected, but the pre-granulosa cells failed to differentiate and remained flat, resulting in no growing follicles in the ovary and female mice were sterile (Schmidt et al., 2004). This study also demonstrates that the developmental status of pre-granulosa cells is critical for the activation of primordial follicles and the development of subsequent growing follicles.
SMAD3
SMAD family member 3 (SMAD3) is known to serve as a signaling intermediate for the transforming growth factor beta TGF family (Kawabata et al., 1998) Smad3 knockout mice are viable. Notably, primordial follicle formation was not affected in Smad3 knockout mice but delayed the activation of primordial follicles and development of growing follicles, resulted in reduced fertility (Tomic et al., 2002). The transcription factor Smad3 is expressed in the nucleus of pre-granulosa cells. SMAD3 directly regulates the transcription of CCND2 and inhibits the expression of Myc. CCND2 is bound by p27, thereby arresting the cycle of precursor granulosa cells and maintaining the dormant state of primordial follicles. When the level of TGF-β increases, SMAD3 is transported out of the nucleus, p27 dissociates from CCND2 to relieve the inhibition of the pre-granulosa cell cycle and promote the activation of primordial follicles (Granados-Aparici et al., 2019). From the current research, p27 and SMAD3 play key roles in follicle development and oogenesis, and they may regulate primordial follicle activation mainly by affecting the pre-granulosa cell cycle (Rajareddy et al., 2007). However, p27 and Smad3 knockout mice were used in the current study, but the precise roles and mechanisms of p27 and SMAD3 in primordial follicle activation cannot be fully elucidated, so further research is needed.
AMH
Anti-mullerian hormone (AMH) is a secreted ligand of the TGF-β superfamily (Pepinsky et al., 1988; Howard et al., 2022). AMH is exclusively produced by granulosa cells of ovarian follicles during the early stages of follicle development (Moolhuijsen and Visser, 2020). AMH plasma levels reflect the continuous non-cyclic growth of small follicles, thereby mirroring the size of the resting primordial follicle pool and thus acting as a useful marker of ovarian reserve (Dewailly et al., 2014). AMH is the best measure of ovarian reserve in different clinical conditions at present. (Teede et al., 2019; Shrikhande et al., 2020; Vatansever et al., 2020). AMH supplementation is able to maintain follicular reserve in some ovarian injury models, such as chemotherapy-induced premature ovarian failure, polycystic ovary syndrome (PCOS) (Sonigo et al., 2019; Hoyos et al., 2020; Ou et al., 2021; Rudnicka et al., 2021).
ESR2
Estrogen and its receptors play an integral role in the periodic recruitment of growing follicles, and estrogen receptor knockout mice lead to infertility in female mice due to abnormal meiosis (Shoham and Schachter, 1996; Liu et al., 2017; Tang et al., 2019). A recent study found that disruption of estrogen receptor β (ESR2) signaling results in increased protein level of AKT and mTOR in both granulosa and oocyte factors and leading to increased activation of primordial follicles (Chakravarthi et al., 2020). This study suggests that estrogen receptors may have no effect on the activation of the first wave of primordial follicles but may regulate the activation of the second wave of primordial follicles. It is also possible that the deletion of the estrogen receptor leads to the development of growth follicles, and the changes in the ovarian microenvironment lead to abnormal activation and loss of primordial follicles.
Wnt ligand secretion mediator
Wingless-type MMTV integration site family (WNT) signaling is an evolutionarily conserved system for cell-cell communication (Liu et al., 2022; Rim et al., 2022). WNT is classified broadly into canonical β-catenin dependent, and non-canonical β-catenin-independent pathways (Clevers and Nusse, 2012; Nusse and Clevers, 2017). The WNT signaling pathway is indispensable for primordial germ cell development, oogenesis, follicle development, and maintenance of follicular reserve (Kocer et al., 2008; Zhou et al., 2012; De Cian et al., 2020; Habara et al., 2021). Among the 19 WNT ligands, the mRNAs for Wnt4, Wnt6 and Wnt11 were experssed in the pre-granulosa cells during primordial follicle activation. Wnt2, Wnt2b, Wnt9a, Wnt5b, Wnt11 and Wnt16 was expressed in the oocytes of primordial follicles (Habara et al., 2021). After conditional knockdown of the wntless in pre-granulosa cells, the pre-granulosa cells could not grow and differentiate into cubic granulosa cells, which leads to female infertility (Habara et al., 2021). Wntless in pre-granulosa cells is essential for communication between pre-granulosa cells and oocytes and primordial follicle activation.
Other important molecules and relative signaling pathways
HIPPO
The HIPPO pathway was first discovered in Drosophila melanogaster, the pathway name comes from the fact that Drosophila overgrew like a hippopotamus after mutations in key molecules of the HIPPO pathway in the head and eyes of Drosophila. The HIPPO pathway is highly conserved from Drosophila to mammals (Ma et al., 2019; Moya and Halder, 2019). The upstream membrane protein receptors of the Hippo signaling pathway act as receptors for extracellular growth inhibition signals, and once they sense the extracellular growth inhibition signals, they activate a series of kinase cascade phosphorylation reactions that eventually phosphorylate the downstream effectors Yes-associated protein (YAP) and transcriptional coactivator with PDZ-binding motif (TAZ). And cytoskeletal proteins bind to the phosphorylated YAP and TAZ, causing them to remain in the cytoplasm and reduce its cytosolic activity, thus achieving the regulation of regulation of organ size and volume (Huang et al., 2005; Yu et al., 2015; Ma et al., 2019). After ovarian fragmentation promotes actin polymerization, p-YAP levels decrease and promotes nuclear transfer of YAP. Nuclear localization of YAP further promotes the expression of CCN growth factors and BIRC apoptosis inhibitors, which ultimately promotes follicular overgrowth (Li et al., 2010; Kawamura et al., 2013). The changes in the HIPPO pathway after ovarian fragmentation are a double-edged sword, on the one hand they can lead to follicular overgrowth and premature ovarian failure due to early follicular depletion. On the other hand, this property can be used to promote primordial follicle activation and develop primordial follicle in vitro activation techniques to help infertile patients to conceive children.
HDAC6
Histone deacetylase 6 (HDAC6) is a special histone deacetylase with two deacetylation domains and one ubiquitination domain. HDAC6 plays a center role in several processes, including positive regulation of peptidyl-serine phosphorylation, protein deacetylation, protein destabilization, microtubule stability (Olzmann et al., 2007; Wang et al., 2018; Wang et al., 2020a; Osseni et al., 2020; Wang et al., 2022). Our study showed that histone deacetylase HDAC6 was expressed heterogeneously in different primordial follicles. About 3%–4% of primordial follicles in neonatal and adult mouse ovaries had low HDAC6 expression, and 65% of primordial follicles with low HDAC6 expression will be activated. Further studies found that HDAC6 was transiently downregulated during primordial follicle activation, mediating selective activation of mouse primordial follicles by regulating the expression of mTOR (Zhang et al., 2021b). Interestingly, overexpression of Hdac6 extends fecundity in female mice, longer telomeres and reduced DNA damage may reduce tumorigenesis in Hdac6 overexpression mice (Zhang et al., 2017). Combined with these studies, we speculate that HDCA6 may regulate primordial follicles to selectively activate primordial follicles, prolong follicular cell telomere length and reduce DNA damage, and ultimately prolong female reproductive lifespan.
SIRT1
NAD-dependent protein deacetylase Sirtuin-1 (SIRT1) has been reported to be involved in the regulation of cellular senescence, aging and organism longevity through the acetylation and deacetylation of these substrates altering their transcriptional and enzymatic activities, as well as protein levels (Yao and Rahman, 2012; Lee et al., 2019; Chen et al., 2020). SIRT1 binds directly to the Akt1 and mTOR promoters to promote their transcription, and increased levels of AKT and mTOR expression promote primordial follicle activation. We conducted a clinical translational potential study and found that short-term SIRT1 agonist treatment activates primordial follicles in vitro and these follicles develop normally, both in mice and humans. In vitro fertilization experiments in mice showed that the quality of oocytes obtained by this method was normal. These results suggest that SIRT1 may be a key protein regulating primordial follicle activation and has certain clinical value (Zhang et al., 2019). Interestingly, overexpression of Sirt1 was able to delay ovarian aging, and this effect was the same as that of calorie restriction, Calorie restriction protects fertility in female mice by activating SIRT1 (Long et al., 2019; Zhang et al., 2019).
TGF-β1
TGFB1 transforming growth factor beta 1 (TGF-β1) is a secreted ligand of the TGF-β superfamily. TGF-β binds to various TGF-β receptors leading to recruitment and activation of SMAD family transcription factors that regulate gene expression. The members of TGF-β superfamily, including TGF-β, GDF9, BMP2, BMP4, BMP5, BMP6, BMP7, BMP15, activins and inhibin are expressed by ovarian somatic cells and oocytes in a developmental stage-related manner and function as intraovarian regulators of folliculogenesis (Lee et al., 2001; Hanrahan et al., 2004; Zhao et al., 2016; Vander Ark et al., 2018). Fetal mouse ovary at embryonic stage 18.5 were cultured with the addition of TGF-β ligand for 5–7 days in vitro (Wang W. et al., 2014). The results showed that the primordial follicle reserve was reduced and the primordial follicle activation was inhibited. The opposite result was obtained after incubation with SD208, an inhibitor of TGFβ-R1. Further testing found that TGF-β maintained primordial follicle inventory and primordial follicle dormancy by inhibiting the mTOR signaling pathway. In Wang’s research, TGF-β only affects the mTOR signaling pathway, and has no effect on the PI3K signaling pathway (Wang Z.-P. et al., 2014). Zhang’s research showed that mTOR signaling in precursor granulosa cells initiates and regulates primordial follicle activation, after the mTOR pathway in pre-granulosa cells is activated, PI3K key proteins in oocytes are phosphorylated (Zhang et al., 2014). Combined with the research analysis of Wang’s and Zhang’s, we speculate that long-term addition of TGF-β may maintain primordial follicle pool and primordial follicle activation by regulating the mTOR signaling pathway in oocytes. Interestingly, the 4-day-old mouse ovaries were cultured with TGF-β for 2 h, and the phosphorylation of S6 which is a key downstream of the mTOR pathway was significantly increased, p-AKT was not changed, and SMAD3 nuclear export in pre-granulosa cells was increased, thereby promoting primordial follicle activation (Granados-Aparici et al., 2019). In our study (data not shown), mTOR and PI3K signaling pathways are significantly inhibited after adding SD208 to cultured 2 dpp ovaries of mice for 2 days. TGF-β plays different roles in different stages of follicular development. Long-term upregulation of TGF-β and short-time upregulation of TGF-β may lead to different or even opposite results for primordial follicle development.
NGF
Neurotrophins are growth factors that promote neuronal and non-neuronal cell survival, proliferation and differentiation (Wang W. et al., 2014; Denk et al., 2017). Nerve growth factor (NGF) is a prototype glycoprotein that belongs to the neurotrophins family. NGF contains two classes of receptors: the high affinity receptor tyrosine kinase A (TrkA) and the low-affinity receptor p75 (Chao and Hempstead, 1995; di Mola et al., 2000). The expression of NGF and its receptors is developmentally regulated during folliculogenesis in different mice ovary (Chaves et al., 2010). The number of primordial follicles was not changed, but the number of primary and secondary follicles was significantly reduced in Ngf knockout mice. In the absence of NGF, primordial follicles cannot be activated. It is worth noting that exogenous addition of NGF has no effect on the activation of primordial follicles (Kerr et al., 2009; Dorfman et al., 2014; Dorfman et al., 2014). After the ovaries are mechanically injured, the expression of NGF in the stroma cells near the injury site increases rapidly, NGF induces selective activation of primordial follicles near the injury site, including near the ovulation site through the mTOR signaling pathway (He et al., 2017). However, how the NGF in the stroma cells induces the activation of nearby primordial follicles, as well as the specific signal transduction and molecular mechanisms are still unclear. This is a scientific issue that needs attention in the future.
EGF
Epidermal growth factor (EGF) encodes a member of the epidermal growth factor superfamily, which acts by binding with high affinity to the cell surface receptor, epidermal growth factor receptor (Schneider and Wolf, 2009). In an in vitro ovarian culture model, addition of EGF promotes primordial follicle activation by activating the activity of the PI3K pathway in oocytes, and short-term treatment (30 min) can induce the activation of primordial follicles in humans and mice in vitro. EGF is a highly effective drug target for primordial follicle activation in vitro (Fujihara et al., 2014; Zhang et al., 2020). EGF is highly expressed in zebrafish ovary and testis, EGFRa is expressed in various organs, including the brain, and EGFRb is mainly expressed in the lung and ovary. It is worth noting that only deletion of EGFRa inhibited primordial follicle activation in vivo, whereas primordial follicle activation was not affected by deletion of EGF (Song et al., 2022). This suggests that other growth factors may promote primordial follicle activation through EGFR.
p27
Cyclin-dependent kinase inhibitor 1B (Cdkn1b), also known as p27 or p27Kip1, is a suppressor of cell cycle (Polyak et al., 1994; Chu et al., 2008; Razavipour et al., 2020). The expression of p27 in the ovary during primordial follicle formation and activation is interesting. During primordial follicle formation, p27 is only expressed in the nucleus of somatic cells and not in oocytes. After primordial follicle formation, p27 is expressed in both pre-granulosa cells and oocytes. The expression of p27 is decreased in granulosa cells during primordial follicle activation. In p27 knockout mice, primordial follicles are formed in advance, and the formed primordial follicles are then activated in advance. In addition, a large number of follicles are atresia and eventually lead to premature ovarian failure. In many studies, it was found that PI3K can regulate the expression of p27, but it is interesting that p27 and PI3K are independent in the process of primordial follicle activation (Rajareddy et al., 2007). However, during chemotherapy, dormant primordial follicles are simultaneously overactivated in the ovary via the PI3K/FOXO3a/p27 pathway. Further studies found that in the model of premature ovarian failure induced by cisplatin injection, FOXO3a binds to the promoter of p27 to inhibit its transcription, resulting in excessive activation of primordial follicles. When melatonin and gastrin were injected at the same time, the binding activity of FOXO3a and p27 increased, which promoted the transcription of p27 and saved the over-activation of primitive follicles caused by cisplatin (Jang et al., 2017).
Clinical application of primordial follicle activation in vitro
Primordial follicles (about 1,000) remain in the ovaries of patients with premature ovarian failure, but these primordial follicles are dormant, and their development is not regulated by gonadotropins (Nelson, 2009; De Vos et al., 2010). To utilize the primordial follicle resources in the ovarian tissue of POF patients, the dormant primordial follicles in the ovary must first be activated to develop to a stage when they can respond to gonadotropins, and then use assisted reproductive technology to achieve pregnancy (Telfer and Anderson, 2021). Primordial follicle activation in vitro (IVA) was recently developed based on the mechanism of primordial follicle activation, which can help patients with premature ovarian failure to achieve fertility (Yin et al., 2016). In addition, IVA can also be used in middle-aged women who are infertile or infertile due to treatment, allowing them to use their own oocytes to carry on offspring (Bertoldo et al., 2018).
The HIPPO signaling pathway determines organ size and is conserved from drosophila to mammals (Seo and Kim, 2018; Ma et al., 2019; Wu and Guan, 2021). Following ovarian damage, disruption of the HIPPO pathway accelerates follicle development, including primordial and growing follicles, which results in increased mice ovarian size. Using the feature of HIPPO signaling pathway can promote primordial follicle activation, combined with agonists of the PI3K or mTOR signaling pathway. A method of primordial follicle activation in vitro was developed to help patients with premature ovarian failure successfully have healthy babies (Kawamura et al., 2013; Zhai et al., 2016; Fabregues et al., 2018; Grosbois and Demeestere, 2018; Lee and Chang, 2019; De Roo et al., 2020; Devenutto et al., 2020; Hsueh and Kawamura, 2020; Tanaka et al., 2020; Zhang et al., 2021a). At the same time, factors such as long in vitro processing time, poor in vitro activation efficiency of primordial follicles, and ethical issues have hindered the clinical application of this technology. Several studies are devoted to improving these adverse factors. The combined use of PI3K and mTOR agonists, resveratrol (SIRT1 agonists), and Rac/Cdc42 activator II (CDC42 agonists) can induce mice ovary primordial follicle activation in vitro, which greatly shortens the time of in vitro activation. These drugs may be a potential new in vitro activation target drugs (Sun et al., 2015; Yan et al., 2018; Zhang et al., 2019). Further research found that orthotopic injection of CDC42 agonist into the ovary can promote the activation of primordial follicles in premature ovarian failure mice and induce human ovary primordial follicle activation in vitro. This method by inducing activation directly in vivo avoids the unknown risks associated with in vitro exposure of ovarian tissue (Zhang et al., 2020). Primordial follicle activation in vitro, this new assisted reproductive technology, has been developed to provide new fertility hope for patients with premature ovarian failure.
Conclusion
The rate of primordial follicle activation controls the length of female fertility. The activation of primordial follicles is mutually regulated by various signaling pathways between oocytes and granulosa cells, and is the result of a close interaction between molecules and between cells (Figure 3). The current study shows that the first wave of primordial follicle activation is determined by PI3K signaling in the oocyte and contributes to female puberty. The second wave primordial follicle activation is determined by mTOR signaling in pre-granulosa cells and determines female fertility throughout life. Understanding the mechanism of primordial follicle activation will help us to further analyze the truth of follicle development and promote the progress of in vitro activation technology.
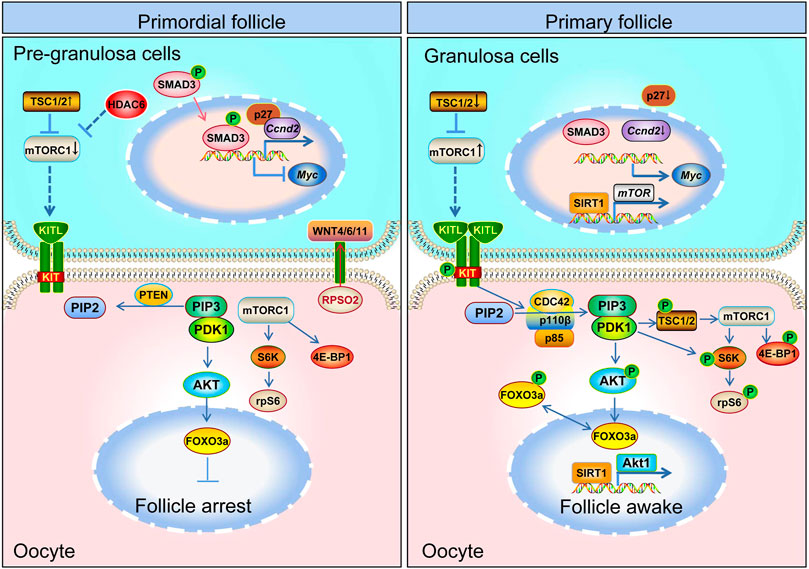
FIGURE 3. Schematic model depicting the mechanisms of primordial follicle activation. Primordial follicle activation is a result of the delicate interaction of pre-granulosa cells and oocytes and the follicular microenvironment. The mTOR and WNT pathways in pre-granulosa cells, the mTOR and PI3K pathway in oocytes, and the communication channel (KITL-KIT) between pre-granulosa cells and oocytes are all necessary for primordial follicle activation. The mTOR signaling pathway in pre-granulosa cells initiates and regulates primordial follicle activation in adult ovary. The mTOR signal in the pre-granulosa cells senses changes in surrounding nutrients, pressure, etc., so that the pre-granulosa cells secrete more KITL. After KITL binds to the receptor on the oocyte membrane, it activates the PI3K signaling pathway in the oocyte, and then promotes the primordial follicle activation.
Author contributions
MH, TZ, JZ, TY, and TC collected the information. MH and TZ wrote the manuscript. TZ, ZX, CW, and ZX revised the manuscript. All authors read and approved the final manuscript.
Funding
This study is funded by the National Natural Science Foundation of China (32100686 to TZ, 32100913 to MH and 82260291 to TZ), Guizhou Provincial Science and Technology Projects [ZK (2022)346 to MH], Science and Technology Fund Project of Guizhou Provincial Health Commission (gzwkj 2021-299 to TZ, gzwkj 2021-527 to MH), China postdoctoral science foundation (2021M700972 to MH and 2022M710919 to TZ), Doctoral Startup Foundation of Guizhou Medical University [(2020)038 to TZ (2020)039 to MH], the National Natural Science Foundation cultivation project of Guizhou Medical University (20NSP031 to TZ, 20NSP008 to MH), innovation and Entrepreneurship Training Program for College Students in Guizhou Province (S202210660136, S202210660093, 202210660090 202110660058), the Continuous Support Fund for Excellent Scientific Research Platform of Colleges and Universities in Guizhou Province [QJJ (2022) 020 to TC], Guizhou Provincial Natural Science Foundation [No (2021)4029 and (2022)4017].
Acknowledgments
We thank the members of TZ’s laboratory for their constructive suggestions in the preparation of the manuscript.
Conflict of interest
The authors declare that the research was conducted in the absence of any commercial or financial relationships that could be construed as a potential conflict of interest.
Publisher’s note
All claims expressed in this article are solely those of the authors and do not necessarily represent those of their affiliated organizations, or those of the publisher, the editors and the reviewers. Any product that may be evaluated in this article, or claim that may be made by its manufacturer, is not guaranteed or endorsed by the publisher.
References
Adhikari, D., Flohr, G., Gorre, N., Shen, Y., Yang, H., Lundin, E., et al. (2009). Disruption of Tsc2 in oocytes leads to overactivation of the entire pool of primordial follicles. Mol. Hum. Reprod. 15, 765–770. doi:10.1093/molehr/gap092
Adhikari, D., and Liu, K. (2009). Molecular mechanisms underlying the activation of mammalian primordial follicles. Endocr. Rev. 30 (5), 438–464. doi:10.1210/er.2008-0048
Adhikari, D., and Liu, K. (2010). mTOR signaling in the control of activation of primordial follicles. Cell cycleGeorget. Tex.) 9 (9), 1673–1674. doi:10.4161/cc.9.9.11626
Benayoun, B. A., Georges, A. B., L'Hôte, D., Andersson, N., Dipietromaria, A., Todeschini, A. L., et al. (2011). Transcription factor FOXL2 protects granulosa cells from stress and delays cell cycle: Role of its regulation by the SIRT1 deacetylase. Hum. Mol. Genet. 20 (9), 1673–1686. doi:10.1093/hmg/ddr042
Bertoldo, M. J., Walters, K. A., Ledger, W. L., Gilchrist, R. B., Mermillod, P., and Locatelli, Y. (2018). In-vitro regulation of primordial follicle activation: Challenges for fertility preservation strategies. Reprod. Biomed. online 36 (5), 491–499. doi:10.1016/j.rbmo.2018.01.014
Bian, X., Xie, Q., Zhou, Y., Wu, H., Cui, J., Jia, L., et al. (2021). Transcriptional changes of mouse ovary during follicle initial or cyclic recruitment mediated by extra hormone treatment. Life Sci. 264, 118654. doi:10.1016/j.lfs.2020.118654
Campbell, B. F., Hercyk, B. S., Williams, A. R., San Miguel, E., Young, H. G., and Das, M. E. (2022). Cdc42 GTPase activating proteins Rga4 and Rga6 coordinate septum synthesis and membrane trafficking at the division plane during cytokinesis. Traffic 23, 478–495. doi:10.1111/tra.12864
Castrillon, D. H., Miao, L., Kollipara, R., Horner, J. W., and DePinho, R. A. (2003). Suppression of ovarian follicle activation in mice by the transcription factor Foxo3a. Science 301, 215–218. doi:10.1126/science.1086336
Chakravarthi, V. P., Ghosh, S., Roby, K. F., Wolfe, M. W., and Rumi, M. A. K. (2020). A gatekeeping role of ESR2 to maintain the primordial follicle reserve. Endocrinology 161, bqaa037. doi:10.1210/endocr/bqaa037
Chao, M. V., and Hempstead, B. L. (1995). p75 and Trk: a two-receptor system. Trends Neurosci. 18 (7), 321–326. doi:10.1016/0166-2236(95)93922-k
Chaves, R. N., Alves, A. M. C. V., Duarte, A. B. G., Araújo, V. R., Celestino, J. J. H., Matos, M. H. T., et al. (2010). Nerve growth factor promotes the survival of goat preantral follicles cultured in vitro. Cells Tissues Organs 192, 272–282. doi:10.1159/000317133
Chen, C., Zhou, M., Ge, Y., and Wang, X. (2020). SIRT1 and aging related signaling pathways. Mech. Ageing Dev. 187, 111215. doi:10.1016/j.mad.2020.111215
Chen, Y.-Y., Russo, D. D., Drake, R. S., Duncan, F. E., Shalek, A. K., Goods, B. A., et al. (2022). Single-cell transcriptomics of staged oocytes and somatic cells reveal novel regulators of follicle activation. Reproduction 164, 55–70. doi:10.1530/REP-22-0053
Choi, Y., Ballow, D. J., Xin, Y., and Rajkovic, A. (2008). Lim homeobox gene, lhx8, is essential for mouse oocyte differentiation and survival. Biol. reproduction 79 (3), 442–449. doi:10.1095/biolreprod.108.069393
Chu, I. M., Hengst, L., and Slingerland, J. M. (2008). The cdk inhibitor p27 in human cancer: Prognostic potential and relevance to anticancer therapy. Nat. Rev. Cancer 8 (4), 253–267. doi:10.1038/nrc2347
Clevers, H., and Nusse, R. (2012). Wnt/β-Catenin signaling and disease. Cell 149, 1192–1205. doi:10.1016/j.cell.2012.05.012
Collins, C., Denisin, A. K., Pruitt, B. L., and Nelson, W. J. (2017). Changes in E-cadherin rigidity sensing regulate cell adhesion. Proc. Natl. Acad. Sci. 114, e5835–e5844. doi:10.1073/pnas.1618676114
Correia, B., Sousa, M. I., and Ramalho-Santos, J. (2020). The mTOR pathway in reproduction: From gonadal function to developmental coordination. Reproduction 159, R173–R188. doi:10.1530/REP-19-0057
Dai, Y., Bo, Y., Wang, P., Xu, X., Singh, M., Jia, L., et al. (2022). Asynchronous embryonic germ cell development leads to a heterogeneity of postnatal ovarian follicle activation and may influence the timing of puberty onset in mice. BMC Biol. 20, 109. doi:10.1186/s12915-022-01318-y
De Baere, E., Lemercier, B., Christin-Maitre, S., Durval, D., Messiaen, L., Fellous, M., et al. (2002). FOXL2 mutation screening in a large panel of POF patients and XX males. J. Med. Genet. 39, e43. doi:10.1136/jmg.39.8.e43
De Cian, M. C., Gregoire, E. P., Le Rolle, M., Lachambre, S., Mondin, M., Bell, S., et al. (2020). R-spondin2 signaling is required for oocyte-driven intercellular communication and follicular growth. Cell death Differ. 27 (10), 2856–2871. doi:10.1038/s41418-020-0547-7
De Roo, C., Lierman, S., Tilleman, K., and De Sutter, P. (2020). In-vitro fragmentation of ovarian tissue activates primordial follicles through the Hippo pathway. Hum. Reprod. Open 2020 (4), hoaa048. doi:10.1093/hropen/hoaa048
De Vos, M., Devroey, P., and Fauser, B. C. (2010). Primary ovarian insufficiency. Lancet (London, Engl. 376 (9744), 911–921. doi:10.1016/S0140-6736(10)60355-8
Denk, F., Bennett, D. L., and McMahon, S. B. (2017). Nerve growth factor and pain mechanisms. Annu. Rev. Neurosci. 40, 307–325. doi:10.1146/annurev-neuro-072116-031121
Devenutto, L., Quintana, R., and Quintana, T. (2020). In vitro activation of ovarian cortex and autologous transplantation: A novel approach to primary ovarian insufficiency and diminished ovarian reserve. Hum. Reprod. Open 2020 (4), hoaa046. doi:10.1093/hropen/hoaa046
Dewailly, D., Andersen, C. Y., Balen, A., Broekmans, F., Dilaver, N., Fanchin, R., et al. (2014). The physiology and clinical utility of anti-Mullerian hormone in women. Hum. Reprod. update 20 (3), 370–385. doi:10.1093/humupd/dmt062
di Mola, F. F., Friess, H., Zhu, Z. W., Koliopanos, A., Bley, T., Di Sebastiano, P., et al. (2000). Nerve growth factor and Trk high affinity receptor (TrkA) gene expression in inflammatory bowel disease. Gut 46 (5), 670–679. doi:10.1136/gut.46.5.670
D’Ignazio, L., Michel, M., Beyer, M., Thompson, K., Forabosco, A., Schlessinger, D., et al. (2018). Lhx8 ablation leads to massive autophagy of mouse oocytes associated with DNA damage. Biol. Reproduction 98, 532–542. doi:10.1093/biolre/iox184
Dorfman, M. D., Garcia-Rudaz, C., Alderman, Z., Kerr, B., Lomniczi, A., Dissen, G. A., et al. (2014). Loss of ntrk2/kiss1r signaling in oocytes causes premature ovarian failure. Endocrinology 155, 3098–3111. doi:10.1210/en.2014-1111
Ezzati, M. M., Baker, M. D., Saatcioglu, H. D., Aloisio, G. M., Pena, C. G., Nakada, Y., et al. (2015). Regulation of FOXO3 subcellular localization by Kit ligand in the neonatal mouse ovary. J. Assisted Reproduction Genet. 32, 1741–1747. doi:10.1007/s10815-015-0589-9
Fabregues, F., Ferreri, J., Calafell, J. M., Moreno, V., Borrás, A., Manau, D., et al. (2018). Pregnancy after drug-free in vitro activation of follicles and fresh tissue autotransplantation in primary ovarian insufficiency patient: A case report and literature review. J. Ovarian Res. 11, 76. doi:10.1186/s13048-018-0447-3
Fujihara, M., Comizzoli, P., Keefer, C. L., Wildt, D. E., and Songsasen, N. (2014). Epidermal Growth Factor (EGF) Sustains in vitro primordial follicle viability by enhancing stromal cell proliferation via MAPK and PI3K Pathways in the prepubertal, but not adult, cat ovary. Biol. Reproduction 90 (4), 86. doi:10.1095/biolreprod.113.115089
Gagliardi, P. A., Puliafito, A., and Primo, L. (2018). PDK1: At the crossroad of cancer signaling pathways. Seminars cancer Biol. 48, 27–35. doi:10.1016/j.semcancer.2017.04.014
Georges, A., Auguste, A., Bessière, L., Vanet, A., Todeschini, A. L., and Veitia, R. A. (2013). FOXL2: A central transcription factor of the ovary. J. Mol. Endocrinol. 52 (1), R17–R33. doi:10.1530/JME-13-0159
Granados-Aparici, S., Hardy, K., Franks, S., Sharum, I. B., Waite, S. L., and Fenwick, M. A. (2019). SMAD3 directly regulates cell cycle genes to maintain arrest in granulosa cells of mouse primordial follicles. Sci. Rep. 9, 6513. doi:10.1038/s41598-019-42878-4
Grosbois, J., and Demeestere, I. (2018). Dynamics of PI3K and Hippo signaling pathways during in vitro human follicle activation. Hum. Reprod. 33, 1705–1714. doi:10.1093/humrep/dey250
Guo, J., Zhang, T., Guo, Y., Sun, T., Li, H., Zhang, X., et al. (2018). Oocyte stage-specific effects of MTOR determine granulosa cell fate and oocyte quality in mice. Proc. Natl. Acad. Sci. 115, e5326–e5333. doi:10.1073/pnas.1800352115
Habara, O., Logan, C. Y., Kanai-Azuma, M., Nusse, R., and Takase, H. M. (2021). WNT signaling in pre-granulosa cells is required for ovarian folliculogenesis and female fertility. Development 148 (9), dev198846. doi:10.1242/dev.198846
Hanrahan, J. P., Gregan, S. M., Mulsant, P., Mullen, M., Davis, G. H., Powell, R., et al. (2004). Mutations in the genes for oocyte-derived growth factors GDF9 and BMP15 are associated with both increased ovulation rate and sterility in cambridge and belclare sheep (Ovis aries). Biol. Reproduction 70, 900–909. doi:10.1095/biolreprod.103.023093
He, Y., Peng, X., Wu, T., Yang, W., Liu, W., Zhang, J., et al. (2017). Restricting the induction of NGF in ovarian stroma engenders selective follicular activation through the mTOR signaling pathway. Cell Death Dis. 8, e2817. doi:10.1038/cddis.2017.168
Heinrich, A., Bhandary, B., Potter, S. J., Ratner, N., and DeFalco, T. (2021). Cdc42 activity in Sertoli cells is essential for maintenance of spermatogenesis. Cell Rep. 37, 109885. doi:10.1016/j.celrep.2021.109885
Hirshfield, A. N. (1991). Development of follicles in the mammalian ovary. Int. Rev. Cytol. 124, 43–101. doi:10.1016/s0074-7696(08)61524-7
Hobert, O., and Westphal, H. (2000). Functions of LIM-homeobox genes. Trends Genet. TIG 16 (2), 75–83. doi:10.1016/s0168-9525(99)01883-1
Howard, J. A., Hart, K. N., and Thompson, T. B. (2022). Molecular mechanisms of AMH signaling. Front. Endocrinol. 13, 927824. doi:10.3389/fendo.2022.927824
Hoyos, L. R., Visser, J. A., McLuskey, A., Chazenbalk, G. D., Grogan, T. R., and Dumesic, D. A. (2020). Loss of anti-Müllerian hormone (AMH) immunoactivity due to a homozygous AMH gene variant rs10417628 in a woman with classical polycystic ovary syndrome (PCOS). Hum. Reprod. 35, 2294–2302. doi:10.1093/humrep/deaa199
Hsueh, A. J., Kawamura, K., Cheng, Y., and Fauser, B. C. (2015). Intraovarian control of early folliculogenesis. Endocr. Rev. 36 (1), 1–24. doi:10.1210/er.2014-1020
Hsueh, A. J. W., and Kawamura, K. (2020). Hippo signaling disruption and ovarian follicle activation in infertile patients. Fertil. Steril. 114, 458–464. doi:10.1016/j.fertnstert.2020.07.031
Huang, J., Wu, S., Barrera, J., Matthews, K., and Pan, D. (2005). The Hippo signaling pathway coordinately regulates cell proliferation and apoptosis by inactivating Yorkie, the Drosophila Homolog of YAP. Cell 122 (3), 421–434. doi:10.1016/j.cell.2005.06.007
Hutt, K. J., McLaughlin, E. A., and Holland, M. K. (2006). KIT/KIT ligand in mammalian oogenesis and folliculogenesis: Roles in rabbit and murine ovarian follicle activation and oocyte growth. Biol. reproduction 75 (3), 421–433. doi:10.1095/biolreprod.106.051516
Jaffe, L. A., and Egbert, J. R. (2017). Regulation of mammalian oocyte meiosis by intercellular communication within the ovarian follicle. Annu. Rev. physiology 79, 237–260. doi:10.1146/annurev-physiol-022516-034102
Jang, H., Na, Y., Hong, K., Lee, S., Moon, S., Cho, M., et al. (2017). Synergistic effect of melatonin and ghrelin in preventing cisplatin-induced ovarian damage via regulation of FOXO3a phosphorylation and binding to the p27Kip1 promoter in primordial follicles. J. Pineal Res. 63, e12432. doi:10.1111/jpi.12432
Kallen, A., Polotsky, A. J., and Johnson, J. (2018). Untapped reserves: Controlling primordial follicle growth activation. Trends Mol. Med. 24, 319–331. doi:10.1016/j.molmed.2018.01.008
Kawabata, M., Inoue, H., Hanyu, A., Imamura, T., and Miyazono, K. (1998). Smad proteins exist as monomers in vivo and undergo homo- and hetero-oligomerization upon activation by serine/threonine kinase receptors. EMBO J. 17 (14), 4056–4065. doi:10.1093/emboj/17.14.4056
Kawamura, K., Cheng, Y., Suzuki, N., Deguchi, M., Sato, Y., Takae, S., et al. (2013). Hippo signaling disruption and Akt stimulation of ovarian follicles for infertility treatment. Proc. Natl. Acad. Sci. 110, 17474–17479. doi:10.1073/pnas.1312830110
Kerr, B., Garcia-Rudaz, C., Dorfman, M., Paredes, A., and Ojeda, S. R. (2009). NTRK1 and NTRK2 receptors facilitate follicle assembly and early follicular development in the mouse ovary. Reproduction 138, 131–140. doi:10.1530/REP-08-0474
Kissel, H., Timokhina, I., Hardy, M. P., Rothschild, G., Tajima, Y., Soares, V., et al. (2000). Point mutation in kit receptor tyrosine kinase reveals essential roles for kit signaling in spermatogenesis and oogenesis without affecting other kit responses. EMBO J. 19 (6), 1312–1326. doi:10.1093/emboj/19.6.1312
Kocer, A., Pinheiro, I., Pannetier, M., Renault, L., Parma, P., Radi, O., et al. (2008). R-spondin1 and FOXL2 act into two distinct cellular types during goat ovarian differentiation. BMC Dev. Biol. 8, 36. doi:10.1186/1471-213X-8-36
Lee, H. N., and Chang, E. M. (2019). Primordial follicle activation as new treatment for primary ovarian insufficiency. Clin. Exp. Reprod. Med. 46, 43–49. doi:10.5653/cerm.2019.46.2.43
Lee, S.-H., Lee, J.-H., Lee, H.-Y., and Min, K.-J. (2019). Sirtuin signaling in cellular senescence and aging. BMB Rep. 52, 24–34. doi:10.5483/BMBRep.2019.52.1.290
Lee, W.-S., Otsuka, F., Moore, R. K., and Shimasaki, S. (2001). Effect of bone morphogenetic protein-7 on folliculogenesis and ovulation in the Rat. Biol. Reproduction 65, 994–999. doi:10.1095/biolreprod65.4.994
Li, J., Kawamura, K., Cheng, Y., Liu, S., Klein, C., Liu, S., et al. (2010). Activation of dormant ovarian follicles to generate mature eggs. Proc. Natl. Acad. Sci. U. S. A. 107 (22), 10280–10284. doi:10.1073/pnas.1001198107
Li, J., Zhang, Y., Zheng, N., Li, B., Yang, J., Zhang, C., et al. (2020). CREB activity is required for mTORC1 signaling-induced primordial follicle activation in mice. Histochem. Cell Biol. 154, 287–299. doi:10.1007/s00418-020-01888-4
Lintern-Moore, S., and Moore, G. P. (1979). The initiation of follicle and oocyte growth in the mouse ovary. Biol. Reprod. 20 (4), 773–778. doi:10.1095/biolreprod20.4.773
Liu, J., Wu, D.-C., Qu, L.-H., Liao, H.-Q., and Li, M.-X. (2018). The role of mTOR in ovarian Neoplasms, polycystic ovary syndrome, and ovarian aging. Clin. Anat. 31, 891–898. doi:10.1002/ca.23211
Liu, J., Xiao, Q., Xiao, J., Niu, C., Li, Y., Zhang, X., et al. (2022). Wnt/β-catenin signalling: Function, biological mechanisms, and therapeutic opportunities. Signal Transduct. Target. Ther. 7 (1), 3. doi:10.1038/s41392-021-00762-6
Liu, K., Rajareddy, S., Liu, L., Jagarlamudi, K., Boman, K., Selstam, G., et al. (2006). Control of mammalian oocyte growth and early follicular development by the oocyte PI3 kinase pathway: New roles for an old timer. Dev. Biol. 299 (1), 1–11. doi:10.1016/j.ydbio.2006.07.038
Liu, W., Xin, Q., Wang, X., Wang, S., Wang, H., Zhang, W., et al. (2017). Estrogen receptors in granulosa cells govern meiotic resumption of pre-ovulatory oocytes in mammals. Cell Death Dis. 8, e2662. doi:10.1038/cddis.2017.82
Long, G.-Y., Yang, J.-Y., Xu, J.-J., Ni, Y.-H., Zhou, X.-L., Ma, J.-Y., et al. (2019). SIRT1 knock-in mice preserve ovarian reserve resembling caloric restriction. Gene 686, 194–202. doi:10.1016/j.gene.2018.10.040
Ma, S., Meng, Z., Chen, R., and Guan, K. L. (2019). The Hippo pathway: Biology and pathophysiology. Annu. Rev. Biochem. 88, 577–604. doi:10.1146/annurev-biochem-013118-111829
Maidarti, M., Anderson, R. A., and Telfer, E. E. (2020). Crosstalk between PTEN/PI3K/Akt signalling and DNA damage in the oocyte: Implications for primordial follicle activation, oocyte quality and ageing. Cells 9, 200. doi:10.3390/cells9010200
McGee, E. A., and Hsueh, A. J. (2000). Initial and cyclic recruitment of ovarian follicles. Endocr. Rev. 21 (2), 200–214. doi:10.1210/edrv.21.2.0394
Monget, P., McNatty, K., and Monniaux, D. (2021). The crazy ovary. Genes 12 (6), 928. doi:10.3390/genes12060928
Moolhuijsen, L. M. E., and Visser, J. A. (2020). Anti-müllerian hormone and ovarian reserve: Update on assessing ovarian function. J. Clin. Endocrinol. metabolism 105 (11), 3361–3373. doi:10.1210/clinem/dgaa513
Mork, L., Maatouk, D. M., McMahon, J. A., Guo, J. J., Zhang, P., McMahon, A. P., et al. (2012). Temporal differences in granulosa cell specification in the ovary reflect distinct follicle fates in mice. Biol. reproduction 86 (2), 37. doi:10.1095/biolreprod.111.095208
Moya, I. M., and Halder, G. (2019). Hippo-YAP/TAZ signalling in organ regeneration and regenerative medicine. Nat. Rev. Mol. cell Biol. 20 (4), 211–226. doi:10.1038/s41580-018-0086-y
Nallathambi, J., Moumné, L., De Baere, E., Beysen, D., Usha, K., Sundaresan, P., et al. (2007). A novel polyalanine expansion in FOXL2: The first evidence for a recessive form of the blepharophimosis syndrome (BPES) associated with ovarian dysfunction. Hum. Genet. 121, 107–112. doi:10.1007/s00439-006-0276-0
Nelson, L. M. (2009). Clinical practice. Primary ovarian insufficiency. N. Engl. J. Med. 360 (6), 606–614. doi:10.1056/NEJMcp0808697
Nilsson, E. E., and Skinner, M. K. (2004). Kit ligand and basic fibroblast growth factor interactions in the induction of ovarian primordial to primary follicle transition. Mol. Cell. Endocrinol. 214 (1-2), 19–25. doi:10.1016/j.mce.2003.12.001
Nusse, R., and Clevers, H. (2017). Wnt/β-Catenin signaling, disease, and emerging therapeutic modalities. Cell 169, 985–999. doi:10.1016/j.cell.2017.05.016
Olzmann, J. A., Li, L., Chudaev, M. V., Chen, J., Perez, F. A., Palmiter, R. D., et al. (2007). Parkin-mediated K63-linked polyubiquitination targets misfolded DJ-1 to aggresomes via binding to HDAC6. J. Cell Biol. 178, 1025–1038. doi:10.1083/jcb.200611128
Osseni, A., Ravel-Chapuis, A., Thomas, J.-L., Gache, V., Schaeffer, L., and Jasmin, B. J. (2020). HDAC6 regulates microtubule stability and clustering of AChRs at neuromuscular junctions. J. Cell Biol. 219 (8), e201901099. doi:10.1083/jcb.201901099
Ou, M., Xu, P., Lin, H., Ma, K., and Liu, M. (2021). AMH is a good predictor of metabolic risk in women with PCOS: A cross-sectional study. Int. J. Endocrinol. 2021, 9511772. doi:10.1155/2021/9511772
Pedersen, T. (1970). Determination of follicle growth rate in the ovary of the immature mouse. J. reproduction Fertil. 21 (1), 81–93. doi:10.1530/jrf.0.0210081
Pepinsky, R. B., Sinclair, L. K., Chow, E. P., Mattaliano, R. J., Manganaro, T. F., Donahoe, P. K., et al. (1988). Proteolytic processing of mullerian inhibiting substance produces a transforming growth factor-beta-like fragment. J. Biol. Chem. 263 (35), 18961–18964. doi:10.1016/s0021-9258(18)37375-7
Pierini, S., Tanyi, J. L., Simpkins, F., George, E., Uribe-Herranz, M., Drapkin, R., et al. (2020). Ovarian granulosa cell tumor characterization identifies FOXL2 as an immunotherapeutic target. JCI insight 5 (16), e136773. doi:10.1172/jci.insight.136773
Polyak, K., Kato, J. Y., Solomon, M. J., Sherr, C. J., Massague, J., Roberts, J. M., et al. (1994). p27Kip1, a cyclin-Cdk inhibitor, links transforming growth factor-beta and contact inhibition to cell cycle arrest. Genes and Dev. 8 (1), 9–22. doi:10.1101/gad.8.1.9
Rajareddy, S., Reddy, P., Du, C., Liu, L., Jagarlamudi, K., Tang, W., et al. (2007). p27kip1 (Cyclin-Dependent Kinase Inhibitor 1B) Controls ovarian development by suppressing follicle endowment and activation and promoting follicle atresia in mice. Mol. Endocrinol. 21, 2189–2202. doi:10.1210/me.2007-0172
Razavipour, S. F., Harikumar, K. B., and Slingerland, J. M. (2020). p27 as a transcriptional regulator: New roles in development and cancer. Cancer Res. 80 (17), 3451–3458. doi:10.1158/0008-5472.CAN-19-3663
Reddy, P., Adhikari, D., Zheng, W., Liang, S., Hämäläinen, T., Tohonen, V., et al. (2009). PDK1 signaling in oocytes controls reproductive aging and lifespan by manipulating the survival of primordial follicles. Hum. Mol. Genet. 18, 2813–2824. doi:10.1093/hmg/ddp217
Reddy, P., Liu, L., Adhikari, D., Jagarlamudi, K., Rajareddy, S., Shen, Y., et al. (2008). Oocyte-specific deletion of Pten causes premature activation of the primordial follicle pool. Science 319, 611–613. doi:10.1126/science.1152257
Reddy, P., Shen, L., Ren, C., Boman, K., Lundin, E., Ottander, U., et al. (2005). Activation of Akt (PKB) and suppression of FKHRL1 in mouse and rat oocytes by stem cell factor during follicular activation and development. Dev. Biol. 281 (2), 160–170. doi:10.1016/j.ydbio.2005.02.013
Reddy, P., Zheng, W., and Liu, K. (2010). Mechanisms maintaining the dormancy and survival of mammalian primordial follicles. Trends Endocrinol. metabolism TEM 21 (2), 96–103. doi:10.1016/j.tem.2009.10.001
Ren, Y., Suzuki, H., Jagarlamudi, K., Golnoski, K., McGuire, M., Lopes, R., et al. (2015). Lhx8 regulates primordial follicle activation and postnatal folliculogenesis. BMC Biol. 13, 39. doi:10.1186/s12915-015-0151-3
Rim, E. Y., Clevers, H., and Nusse, R. (2022). The Wnt pathway: From signaling mechanisms to synthetic modulators. Annu. Rev. Biochem. 91, 571–598. doi:10.1146/annurev-biochem-040320-103615
Rudnicka, E., Kunicki, M., Calik-Ksepka, A., Suchta, K., Duszewska, A., Smolarczyk, K., et al. (2021). Anti-müllerian hormone in pathogenesis, diagnostic and treatment of PCOS. Int. J. Mol. Sci. 22, 12507. doi:10.3390/ijms222212507
Saatcioglu, H. D., Cuevas, I., and Castrillon, D. H. (2016). Control of oocyte reawakening by kit. PLoS Genet. 12 (8), e1006215. doi:10.1371/journal.pgen.1006215
Salussolia, C. L., Klonowska, K., Kwiatkowski, D. J., and Sahin, M. (2019). Genetic etiologies, diagnosis, and treatment of tuberous sclerosis complex. Annu. Rev. Genomics Hum. Genet. 20, 217–240. doi:10.1146/annurev-genom-083118-015354
Schmidt, D., Ovitt, C. E., Anlag, K., Fehsenfeld, S., Gredsted, L., Treier, A.-C., et al. (2004). The murine winged-helix transcription factor Foxl2 is required for granulosa cell differentiation and ovary maintenance. Development 131, 933–942. doi:10.1242/dev.00969
Schneider, M. R., and Wolf, E. (2009). The epidermal growth factor receptor ligands at a glance. J. Cell. physiology 218 (3), 460–466. doi:10.1002/jcp.21635
Seo, J., and Kim, J. (2018). Regulation of Hippo signaling by actin remodeling. BMB Repeports 51, 151–156. doi:10.5483/bmbrep.2018.51.3.012
Shoham, Z., and Schachter, M. (1996). Estrogen biosynthesis-regulation, action, remote effects, and value of monitoring in ovarian stimulation cycles. Fertil. Steril. 65 (4), 687–701. doi:10.1016/s0015-0282(16)58197-7
Shrikhande, L., Shrikhande, B., and Shrikhande, A. (2020). AMH and its clinical implications. J. Obstetrics Gynecol. India 70, 337–341. doi:10.1007/s13224-020-01362-0
Song, Y., Chen, W., Zhu, B., and Ge, W. (2022). Disruption of epidermal growth factor receptor but not EGF blocks follicle activation in zebrafish ovary. Front. Cell Dev. Biol. 9, 750888, doi:10.3389/fcell.2021.750888
Sonigo, C., Beau, I., Grynberg, M., and Binart, N. (2019). AMH prevents primordial ovarian follicle loss and fertility alteration in cyclophosphamide-treated mice. FASEB J. 33, 1278–1287. doi:10.1096/fj.201801089R
Sun, X., Su, Y., He, Y., Zhang, J., Liu, W., Zhang, H., et al. (2015). New strategy for in vitro activation of primordial follicles with mTOR and PI3K stimulators. Cell Cycle 14, 721–731. doi:10.1080/15384101.2014.995496
Tanaka, Y., Hsueh, A. J., and Kawamura, K. (2020). Surgical approaches of drug-free in vitro activation and laparoscopic ovarian incision to treat patients with ovarian infertility. Fertil. Steril. 114, 1355–1357. doi:10.1016/j.fertnstert.2020.07.029
Tang, Z. R., Zhang, R., Lian, Z. X., Deng, S. L., and Yu, K. (2019). Estrogen-receptor expression and function in female reproductive disease. Cells 8 (10), 1123. doi:10.3390/cells8101123
Teede, H., Misso, M., Tassone, E. C., Dewailly, D., Ng, E. H., Azziz, R., et al. (2019). Anti-müllerian hormone in PCOS: A review informing international guidelines. Trends Endocrinol. Metabolism 30, 467–478. doi:10.1016/j.tem.2019.04.006
Telfer, E. E., and Andersen, C. Y. (2021). In vitro growth and maturation of primordial follicles and immature oocytes. Fertil. Steril. 115 (5), 1116–1125. doi:10.1016/j.fertnstert.2021.03.004
Tomic, D., Brodie, S. G., Deng, C., Hickey, R. J., Babus, J. K., Malkas, L. H., et al. (2002). Smad 3 may regulate follicular growth in the mouse ovary. Biol. Reproduction 66, 917–923. doi:10.1095/biolreprod66.4.917
Vander Ark, A., Cao, J., and Li, X. (2018). TGF-β receptors: In and beyond TGF-β signaling. Cell. Signal. 52, 112–120. doi:10.1016/j.cellsig.2018.09.002
Vatansever, D., İncir, S., Bildik, G., Taskiran, C., and Oktem, O. (2020). In-vitro AMH production of ovarian tissue samples in culture correlates with their primordial follicle pool. Eur. J. Obstetrics Gynecol. Reproductive Biol. 254, 138–140. doi:10.1016/j.ejogrb.2020.09.002
Wang, M., Zhou, C., Yu, L., Kong, D., Ma, W., Lv, B., et al. (2022). Upregulation of MDH1 acetylation by HDAC6 inhibition protects against oxidative stress-derived neuronal apoptosis following intracerebral hemorrhage. Cell. Mol. Life Sci. 79, 356. doi:10.1007/s00018-022-04341-y
Wang, R., Tan, J., Chen, T., Han, H., Tian, R., Tan, Y., et al. (2018). ATP13A2 facilitates HDAC6 recruitment to lysosome to promote autophagosome-lysosome fusion. J. Cell Biol. 218, 267–284. doi:10.1083/jcb.201804165
Wang, W., Chen, J., and Guo, X. (2014). The role of nerve growth factor and its receptors in tumorigenesis and cancer pain. Biosci. trends 8 (2), 68–74. doi:10.5582/bst.8.68
Wang, Y., Wang, K., and Fu, J. (2020a). HDAC6 mediates poly (I:C)-Induced TBK1 and Akt phosphorylation in macrophages. Front. Immunol. 11, 1776. doi:10.3389/fimmu.2020.01776
Wang, Z.-P., Mu, X.-Y., Guo, M., Wang, Y.-J., Teng, Z., Mao, G.-P., et al. (2014). Transforming growth factor-β signaling participates in the maintenance of the primordial follicle pool in the mouse ovary. J. Biol. Chem. 289, 8299–8311. doi:10.1074/jbc.M113.532952
Wang, Z., Liu, C.-Y., Zhao, Y., and Dean, J. (2020b). FIGLA, LHX8 and SOHLH1 transcription factor networks regulate mouse oocyte growth and differentiation. Nucleic Acids Res. 48, 3525–3541. doi:10.1093/nar/gkaa101
Wirth, A., Labus, J., Abdel Galil, D., Schill, Y., Schmidt, S., Bunke, T., et al. (2022). Palmitoylation of the small GTPase Cdc42 by DHHC5 modulates spine formation and gene transcription. J. Biol. Chem. 298, 102048. doi:10.1016/j.jbc.2022.102048
Worby, C. A., and Dixon, J. E. (2014). Pten. Annu. Rev. Biochem. 83, 641–669. doi:10.1146/annurev-biochem-082411-113907
Wu, Z., and Guan, K.-L. (2021). Hippo signaling in embryogenesis and development. Trends Biochem. Sci. 46, 51–63. doi:10.1016/j.tibs.2020.08.008
Yan, H., Wen, J., Zhang, T., Zheng, W., He, M., Huang, K., et al. (2019). Oocyte-derived E-cadherin acts as a multiple functional factor maintaining the primordial follicle pool in mice. Cell Death Dis. 10, 160. doi:10.1038/s41419-018-1208-3
Yan, H., Zhang, J., Wen, J., Wang, Y., Niu, W., Teng, Z., et al. (2018). CDC42 controls the activation of primordial follicles by regulating PI3K signaling in mouse oocytes. BMC Biol. 16, 73. doi:10.1186/s12915-018-0541-4
Yao, H., and Rahman, I. (2012). Perspectives on translational and therapeutic aspects of SIRT1 in inflammaging and senescence. Biochem. Pharmacol. 84, 1332–1339. doi:10.1016/j.bcp.2012.06.031
Yehia, L., Keel, E., and Eng, C. (2020). The clinical spectrum of PTEN mutations. Annu. Rev. Med. 71, 103–116. doi:10.1146/annurev-med-052218-125823
Yin, O., Cayton, K., and Segars, J. H. (2016). In vitro activation: A dip into the primordial follicle pool? J. Clin. Endocrinol. metabolism 101 (10), 3568–3570. doi:10.1210/jc.2016-2837
Yu, F. X., Zhao, B., and Guan, K. L. (2015). Hippo pathway in organ size control, tissue homeostasis, and cancer. Cell 163 (4), 811–828. doi:10.1016/j.cell.2015.10.044
Zaidel-Bar, R. (2013). Cadherin adhesome at a glance. J. Cell Sci. 126, 373–378. doi:10.1242/jcs.111559
Zhai, J., Yao, G., Dong, F., Bu, Z., Cheng, Y., Sato, Y., et al. (2016). In vitro activation of follicles and fresh tissue auto-transplantation in primary ovarian insufficiency patients. J. Clin. Endocrinol. Metabolism 101, 4405–4412. doi:10.1210/jc.2016-1589
Zhang, H., and Liu, K. (2015). Cellular and molecular regulation of the activation of mammalian primordial follicles: Somatic cells initiate follicle activation in adulthood. Hum. Reprod. update 21 (6), 779–786. doi:10.1093/humupd/dmv037
Zhang, H., Panula, S., Petropoulos, S., Edsgärd, D., Busayavalasa, K., Liu, L., et al. (2015). Adult human and mouse ovaries lack DDX4-expressing functional oogonial stem cells. Nat. Med. 21, 1116–1118. doi:10.1038/nm.3775
Zhang, H., Risal, S., Gorre, N., Busayavalasa, K., Li, X., Shen, Y., et al. (2014). Somatic cells initiate primordial follicle activation and govern the development of dormant oocytes in mice. Curr. Biol. 24, 2501–2508. doi:10.1016/j.cub.2014.09.023
Zhang, J., Yan, L., Wang, Y., Zhang, S., Xu, X., Dai, Y., et al. (2020). In vivo and in vitro activation of dormant primordial follicles by EGF treatment in mouse and human. Clin. Transl. Med. 10, e182. doi:10.1002/ctm2.182
Zhang, L., Zhang, J., Zhai, J., Liu, X., Deng, W., Wang, H., et al. (2021a). Autotransplantation of the ovarian cortex after in-vitro activation for infertility treatment: A shortened procedure. Hum. Reprod. 36, 2134–2147. doi:10.1093/humrep/deab143
Zhang, T., Du, X., Zhao, L., He, M., Lin, L., Guo, C., et al. (2019). SIRT1 facilitates primordial follicle recruitment independent of deacetylase activity through directly modulating Akt1 and mTOR transcription. FASEB J. 33, 14703–14716. doi:10.1096/fj.201900782R
Zhang, T., He, M., Zhao, L., Qin, S., Zhu, Z., Du, X., et al. (2021b). HDAC6 regulates primordial follicle activation through mTOR signaling pathway. Cell Death Dis. 12, 559. doi:10.1038/s41419-021-03842-1
Zhang, X., Yang, J., Wang, H., Guo, R., Yin, Y., Zhang, D., et al. (2017). Overexpression of Hdac6 extends reproductive lifespan in mice. Protein and Cell 8, 360–364. doi:10.1007/s13238-017-0375-9
Zhang, X., Zhang, W., Wang, Z., Zheng, N., Yuan, F., Li, B., et al. (2022). Enhanced glycolysis in granulosa cells promotes the activation of primordial follicles through mTOR signaling. Cell Death Dis. 13, 87. doi:10.1038/s41419-022-04541-1
Zhao, L., Du, X., Huang, K., Zhang, T., Teng, Z., Niu, W., et al. (2016). Rac1 modulates the formation of primordial follicles by facilitating STAT3-directed Jagged1, GDF9 and BMP15 transcription in mice. Sci. Rep. 6, 23972. doi:10.1038/srep23972
Zhao, L., Li, Q., Kuang, Y., Xu, P., Sun, X., Meng, Q., et al. (2022). Heterozygous loss-of-function variants in LHX8 cause female infertility characterized by oocyte maturation arrest. Genet. Med. official J. Am. Coll. Med. Genet. 24 (11), 2274–2284. doi:10.1016/j.gim.2022.07.027
Zhao, Y., Feng, H., Zhang, Y., Zhang, J. V., Wang, X., Liu, D., et al. (2021). Current understandings of core pathways for the activation of mammalian primordial follicles. Cells 10, 1491. doi:10.3390/cells10061491
Zhao, Y., Zhang, Y., Li, J., Zheng, N., Xu, X., Yang, J., et al. (2018). MAPK3/1 participates in the activation of primordial follicles through mTORC1-KITL signaling. J. Cell. Physiology 233, 226–237. doi:10.1002/jcp.25868
Zheng, W., Zhang, H., Gorre, N., Risal, S., Shen, Y., and Liu, K. (2014). Two classes of ovarian primordial follicles exhibit distinct developmental dynamics and physiological functions. Hum. Mol. Genet. 23, 920–928. doi:10.1093/hmg/ddt486
Keywords: ovary, follicle, primordial follicle activation, In vitro activation, premature ovarian failure
Citation: Zhang T, He M, Zhang J, Tong Y, Chen T, Wang C, Pan W and Xiao Z (2023) Mechanisms of primordial follicle activation and new pregnancy opportunity for premature ovarian failure patients. Front. Physiol. 14:1113684. doi: 10.3389/fphys.2023.1113684
Received: 01 December 2022; Accepted: 20 February 2023;
Published: 28 February 2023.
Edited by:
Bin Liu, Independent Researcher, Shenzhen, ChinaReviewed by:
Shuangbo Kong, Xiamen University, ChinaBunpei Ishizuka, Rose Ladies Clinic, Japan
Yin-Li Zhang, Zhejiang University, China
Copyright © 2023 Zhang, He, Zhang, Tong, Chen, Wang, Pan and Xiao. This is an open-access article distributed under the terms of the Creative Commons Attribution License (CC BY). The use, distribution or reproduction in other forums is permitted, provided the original author(s) and the copyright owner(s) are credited and that the original publication in this journal is cited, in accordance with accepted academic practice. No use, distribution or reproduction is permitted which does not comply with these terms.
*Correspondence: Ziwen Xiao, xzwe@hotmail.com; Wei Pan, pw__6602@163.com; Chao Wang, wangcam@126.com
†ORCID: Tuo Zhang, orcid.org/0000-0003-3256-5930
‡These authors have contributed equally to this work