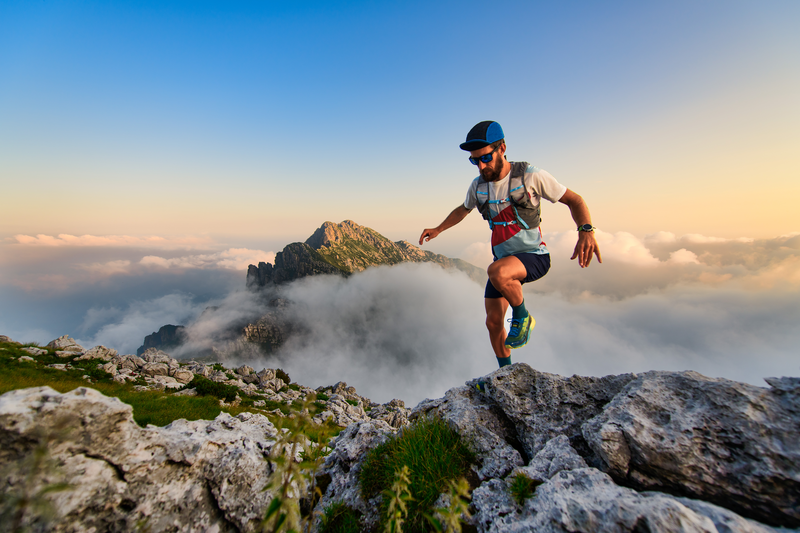
94% of researchers rate our articles as excellent or good
Learn more about the work of our research integrity team to safeguard the quality of each article we publish.
Find out more
ORIGINAL RESEARCH article
Front. Physiol. , 08 March 2023
Sec. Invertebrate Physiology
Volume 14 - 2023 | https://doi.org/10.3389/fphys.2023.1107434
This article is part of the Research Topic The Side Effects of Insecticides on Insects and the Adaptation Mechanisms of Insects to Insecticides View all 15 articles
Maize is an essential crop of China. The recent invasion of Spodoptera frugiperda, also known as fall armyworm (FAW), poses a danger to the country’s ability to maintain a sustainable level of productivity from this core crop. Entomopathogenic fungi (EPF) Metarhizium anisopliae MA, Penicillium citrinum CTD-28 and CTD-2, Cladosporium sp. BM-8, Aspergillus sp. SE-25 and SE-5, Metarhizium sp. CA-7, and Syncephalastrum racemosum SR-23 were tested to determine their effectiveness in causing mortality in second instars, eggs, and neonate larvae. Metarhizium anisopliae MA, P. citrinum CTD-28, and Cladosporium sp. BM-8 caused the highest levels of egg mortality, with 86.0, 75.3, and 70.0%, respectively, followed by Penicillium sp. CTD-2 (60.0%). Additionally, M. anisopliae MA caused the highest neonatal mortality of 57.1%, followed by P. citrinum CTD-28 (40.7%). In addition, M. anisopliae MA, P. citrinum CTD-28, and Penicillium sp. CTD-2 decreased the feeding efficacy of second instar larvae of FAW by 77.8, 75.0, and 68.1%, respectively, followed by Cladosporium sp. BM-8 (59.7%). It is possible that EPF will play an important role as microbial agents against FAW after further research is conducted on the effectiveness of these EPF in the field.
China’s reliance on maize production is evidenced by the fact that the nation now ranks among the world’s top net importers of grain (Tong, 2000). China is the world’s second-largest producer of corn, behind only the United States. The fall armyworm (FAW), also known as Spodoptera frugiperda, J. E. Smith, 1797 (Lepidoptera: Noctuidae), is regarded as a serious and devastating pest of maize. Because of this, maize production continues to be at risk. The American continent, especially its tropical and subtropical parts, is the origin of this pest (Sparks, 1979); it has recently attacked several countries and its presence has had a profoundly negative influence on world food security in all of the locations that it has colonized. FAW was firstly seen in Nigeria and Ghana (Goergen et al., 2016), but it quickly migrates in other African countries (Stokstad, 2017). In 2018, FAW was reported in the state of Karnataka, which is located in the southern portion of India (Sharanabasappa et al., 2018). By the end of 2018, its occurrence was also documented in nations located in Southeast Asia, including Thailand, Bangladesh, and Myanmar (Guo et al., 2018).
The corn strain of FAW was firstly observed in China in December 2018 (Zhang et al., 2019; Sun et al., 2021). Major provinces like Yunnan, were severely attacked by this invasive pest (Zhang et al., 2021), which damages important staple and economical crops but preferably feeds on corn and sorghum in China (Montezano et al., 2018). Significant damage was observed in cornfields of Yunnan during the initial attack of FAW (Yang et al., 2019). The damage losses to the maize crop were up to 22, 67, 32% and 47% in Ghana, Zambia, Ethiopia, and Kenya, respectively (Day et al., 2017; Kumela et al., 2019). Hence, this invasive pest is responsible for causing financial losses of up to 4.66 billion USD dollars in Africa (Rwomushana et al., 2018). FAW causes reduction in yield of 78, 80% and 90% of peanut, barley, and wheat crops, respectively (He et al., 2020; Yang et al., 2020). FAW also causes damage to tobacco crops if its population peaks (Xu et al., 2019).
Recently, the development of biopesticides, including plant extracts and novel insecticides is the serious concern of researchers for reducing crop damage and maximizing agricultural yield (Cook et al., 2004; Idrees et al., 2016; Idrees et al., 2017; Luo et al., 2018; Qadir et al., 2021; Ahmed et al., 2022; Liu et al., 2022; Idrees et al., 2022b). However, regular use of pesticides to control FAW have become a regular practice which is harmful to the ecosystem and natural enemies (Gu et al., 2018; Cai et al., 2017).
The application of entomopathogenic fungi (EPF) is considered to be one of the most common strategies for the control of FAW (Idrees et al., 2021; Idrees et al., 2022a). Because EPF and synthetic insecticides have distinct modes of action, therefore, EPF do not act as fast as synthetic insecticides to kill insect pests (Hajek, 1989; Fargues et al., 1994). EPF can cause infection when spores come in contact with the arthropod host. Fungal spores germinate and breach the insect cuticle through enzymatic degradation and mechanical pressure to gain entry into the insect body under an ideal condition. The EPF have fast multiplication after invading the insect tissues, and emerge from the dead insect to produce more fungal spores (Dara, 2017; Altinok et al., 2019; Ebani and Mancianti, 2021). However, it is worth noting that these EPF serve to minimize crop damage by causing host pest infection, which leads to a decrease in feeding, egg laying, development, and mating and disturbs the physiological function of pests (Thomas et al., 1997). Isolates of EPF exhibited considerable mortality of eggs and neonate larvae of FAW (Akutse et al., 2019) and significantly reduced the feeding efficacy of larvae (Qadir et al., 2021; Idrees et al., 2022a).
A few studies have been performed on the effectiveness of native EPF for the management of FAW in China. Therefore, the purpose of the present research was to evaluate the virulence of EPF against immature stages (eggs, neonate larvae and pupae) as well as on the feeding performance of FAW larvae to develop microbial-based biopesticides at the commercial level against FAW.
Eggs of FAW were collected from an established colony in the laboratory. The eggs were kept in a ventilated rectangular plastic box (28 cm3 × 17 cm3 × 18 cm3). The neonate larvae were fed with fresh maize insecticides free leaves. The first− to third-instar larvae were kept in a rectangular plastic box (28 cm3 × 17 cm3 × 18 cm3), while fourth− to sixth-instar larvae were separately placed in six-well plates to prevent cannibalism until pupation. The new emerging adults were placed in cylindrical glasses. A paper towel was used to cover the top portion of the adult glasses, and sterile cotton balls were placed inside a plastic bottle lid soaked with a 10% concentration of honey. The larvae were kept at 25°C ± 2°C, with a photoperiod of 12:12 (dark: light) and 65% ± 5% relative humidity (RH). Fifty laboratory-reared generations of larvae were used in the present study.
The EPF, Metarhizium anisopliae MA, Penicillium citrinum CTD-28 and CTD-2, Cladosporium sp. BM-8, Aspergillus sp. SE-25 and SE-5, Metarhizium sp. CA-7, and Syncephalastrum racemosum SR-23 were evaluated against immature stages and feeding efficacy of FAW. Detailed information about EPF species is described in Table 1. EPF species were obtained from laboratory collection at IZ-GDAS.
TABLE 1. Information about the fungal isolates evaluated in the present study for the management of fall armyworm.
The fungal isolates were cultured by spreading a small portion of it on Sabouraud dextrose agar media (inoculation) in Petri dished (90 mm in diameter). The Petri dishes were incubated in dark incubator for 2–3 weeks. Fungal conidia were harvested from 2- to 3-week-old sporulated cultures and suspended in 10 mL of distilled water with 0.05% Tween-80 in universal bottles containing glass beads containing six to nine beads (3 mm in diameter) for each bottle. The fungal conidial suspensions were vortexed for 5 minutes at approximately 700 rpm to break up the conidial clumps and verify that the suspension was homogenous. Three conidial concentrations (1 × 106, 1 × 107, and 1 × 108 conidia/mL) were adjusted using hemocytometer before the bioassay.
The viability tests of fungal isolates were further conducted prior to starting the bioassay (Opisa et al., 2018). The eight fungal isolates showed ≥90% germination rates (Supplementary Table S1).
FAW eggs that were one to 2 days old were taken from adult cylindrical glass. Under a light microscope, 50 eggs were divided using a camel hairbrush. A volume of 10 mL of each concentration was sprayed on a batch of 50 eggs using a manually atomized spray bottle (20 mL). A sterile paper towel was placed at the bottom of rectangular box to absorb the extra spore suspension. Sterilized distilled water with 0.05% Tween-80 was considered as a control. The eggs were then air-dried for an hour in a laminar flow hood after exposure to the treatment. The eggs were then put into Petri dishes and incubated at room temperature (25°C ± 2°C) with 65% ± 5% relative humidity (RH). Egg mortality were measured 7 days post treatment. Neonates larvae that emerged from the treated eggs were kept in a perforated rectangular plastic box coated with wet filter paper. Fresh maize leaves were provided to the neonate’s larvae daily. The data of neonate larvae were measured daily up to 14 days post treatment. The whole bioassays were repeated twice and completely randomized design (CRD) was used with three replications for each treatment.
The mortality of the neonate larvae was observed daily for 7 days. The cumulative mortality of both neonates and larvae of FAW was calculated by counting the dead eggs and neonates’ larvae of FAW divided by a total number of eggs at 14 days post-treatment (Idrees et al., 2022a). The cadavers were examined to see whether mycosis was present by the approach of (Aktuse et al., 2019). The dead cadavers were surface sterilized with alcohol (70%) and rinsed three times in a distilled water. The surface-sterilized dead cadavers were kept in Petri dishes containing sterile filter paper. The mortality due to target EPF was tested by observing hyphae and conidia on the body of dead cadavers.
For laboratory bioassays involving EPF evaluations against lepidopterous pests, early second− or third-instar larvae are usually used because these larvae are easy to handle or manipulate during experimentation and are more susceptible to different insecticidal treatments than the other larval instars. In fact, first instar larvae are delicate and soft and are vulnerable to mechanical damage while manipulating or handling, while later (fourth - sixth) instar larvae are somewhat resistant and do not respond well to treatments. Therefore, only early second instar larvae were used in this study.
A group of 30 s instar larvae were transferred on fresh maize leaves unexposed to insecticides in a rectangular plastic box (28 cm3 × 17 cm3 × 18 cm3) covered with perforated lid. The bioassays were laid out according to CRD with three replications for each treatment. Afterwards, 10 mL of each concentration was sprayed on the larvae in each rectangular plastic container using an atomized manual spray bottle (20 mL). Each concentration was sprayed on every second instar larva to ensure that none escaped from the fungal spore suspension by hiding beneath the leaves’ surfaces. A sterile paper towel was put underneath the leaves to absorb the extra fungal suspension. The control larvae were treated with distilled water (0.05% Tween-80). Fresh maize leaves were given daily, and the treated larvae were kept at a temperature of 25°C ± 2°C. Larval mortality was recorded daily for up to 7 days. Mycosis was performed for the dead cadavers by the approach of Akutse et al. (2019).
Using a hand-held atomizer spray bottle (20 mL), 15 FAW second-instar larvae were exposed to 10 mL of an EPF at three different concentrations (Fargues and Maniania, 1992). In a square plastic container, we inserted 12 g of fresh maize leaves. Sterilized distilled water with 0.05% Tween-80 was used to treat the control. The second instar larvae were weighed before and after being given a diet of fresh maize leaves. For this study, we calculated the feeding performance of the larvae in terms of feeding % by dividing the total weight of fresh maize leaf surface in Gram fed to the larvae by the total weight of leaves not eaten by the larvae and then multiplying that number by 100. The feeding performance was observed 24 and 48 h post treatment. The experiment was laid out according to CRD with three replications.
Using manual atomizer spray bottles (20 mL), 10 mL of each concentration of the investigated fungal isolates were applied to the pupae of FAW. Each treatment’s 15 pupae were put inside a 28 cm3 × 17 cm3 × 18 cm3 rectangular plastic box. Sterilized distilled water containing 0.05% Tween-80 was used to treat the control. Pupal mortality was observed for 15 days (Liu et al., 2022). The pupa did not turn black or emerge, or not show any movement upon touch considered as dead. The experiment was laid out according to CRD with three replications.
The Shapiro–Wilk test was used to analyze the normality of all of the stages before they were subjected to a one-way analysis of variance (ANOVA) using Tukey’s highly significant difference (HSD) post hoc test at a 95% level of significance (Shapiro and Wilk, 1965). In addition to graphical depiction, Statistix® Version 8.1 was used to statistically evaluate the data (Analytical Software, Tallahassee, FL). Factorial ANOVA was used to analyze the interaction of the various factors, including the fungal conidial concentrations, treatments and immature stages, and this was followed by Tukey’s highly significant difference (HSD) post hoc test. SPSS (version 22.0) was used to perform for the data analysis.
The results revealed that the isolate of M. anisopliae MA caused 40% egg mortality, followed by the isolates of P. citrinum CTD-28 and Cladosporium sp. BM-8 which caused 36.7% and 35.3% egg mortality, respectively, treated with 1 × 106 conidia/mL at 7 days post treatment (F8, 18 = 19.3; p = 0.0000). The isolate of Penicillium sp. CTD-2 induced 30.0% egg mortality compared to the control with 4.0% (Figure 1; Supplementary Table S2). The isolate of M. anisopliae MA caused the highest egg mortality of 70%, followed by the isolates of P. citrinum CTD-28 and Cladosporium sp. BM-8 which caused 55.3% and 50.0% egg mortality, respectively, treated with 1 × 107 conidia/mL (F8, 18 = 44.1; p = 0.0000). The isolate of Penicillium sp. CTD-2 induced 45.3% egg mortality compared to 4.0% in the control (Figure 1; Supplementary Table S3). The isolates of M. anisopliae MA and P. citrinum CTD-28 caused 86.0% and 75.3% egg mortality, respectively, followed by Cladosporium sp. BM-8 and Penicillium sp. CTD-2 isolates which caused 70.0% and 60.0% egg mortality, respectively, treated with 1 × 108 conidia/mL (F8, 18 = 99.1; p = 0.0000). The lowest egg mortality (24.7%) caused by the isolate of Aspergillus sp. SE-5 over the control with 7.3% (Figure 1; Supplementary Table S4).
FIGURE 1. Fungal isolates induced fall armyworm’s egg mortality treated with different concentrations at 7 days post treatment. Error bars denote the mean ± standard error at the 95% confidence interval. Means followed by the same letters are not significantly different by Tukey’s test at p < 0.05.
The results revealed that the M. anisopliae MA isolate caused 23.3% neonate larvae mortality, followed by Cladosporium sp. BM-8 and Penicillium sp. CTD-2 isolates with 19.6% and 17.1% treated with 1 × 106 conidia/mL at 7 days post treatment (F8, 18 = 4.65; p = 0.0032) (Figure 2; Supplementary Table S2) respectively, over 3.0% in the control. Metarhizium anisopliae MA and Cladosporium sp. BM-8 isolates caused neonate larvae mortality rates of 35.6% and 30.7%, respectively, followed by Penicillium sp. CTD-2 and P. citrinum CTD-28 isolates with 23.2% and 20.9%, respectively, over the control with 1.4% treated with 1 × 107 conidia/mL (F8, 18 = 4.24; p = 0.0,052) (Figure 2; Supplementary Table S3). Metarhizium anisopliae MA and P. citrinum CTD-28 isolates caused neonate mortality rates of 57.1% and 40.7%, respectively, followed by Cladosporium sp. BM-8 and Penicillium sp. CTD-2 isolates with 35.6% and 30.0%, respectively, over the control with 4.5% treated with 1 × 108 conidia/mL (F8, 18 = 3.03; p = 0.0241) (Figure 2; Supplementary Table S4). Furthermore, only 5%–10% of insect cadavers showed mycosis.
FIGURE 2. Fungal isolates induced fall armyworm’s neonate larvae mortality when treated with different concentrations at 7 days post treatment. Error bars denote the mean ± standard error at the 95% confidence interval. Means followed by the same letters are not significantly different by Tukey’s test at p < 0.05.
The results showed that M. anisopliae MA and Cladosporium sp. BM-8 isolates caused cumulative mortality of 54.0% and 48.0%, respectively, followed by P. citrinum CTD-28 and Penicillium sp. CTD-2 isolates, which caused 44.0% and 42.0% cumulative mortality to the eggs and neonates, respectively, compared to the control with 7.3%, respectively, over the control with 3.0% after treated with 1 × 106 conidia/mL at14 days post treatment (F8, 18 = 4.7; p = 0.0000) (Figure 3; Supplementary Table S2). Metarhizium anisopliae MA, Cladosporium sp. BM-8 and P. citrinum CTD-28 isolates caused cumulative mortalities of 80.7%, 65.3%, and 64.7%, respectively, followed by Penicillium sp. CTD-2 with 58.0% over the control (5.3%) treated with 1 × 107 conidia/mL (F8, 18 = 27.4; p = 0.0000) (Figure 3; Supplementary Table S3). Metarhizium anisopliae MA, P. citrinum CTD-28 and Cladosporium sp. BM-8 isolates revealed a significant effect by causing cumulative mortality of 94.0%, 85.3%, and 80.7%, respectively, followed by Penicillium sp. CTD-2 with 72.0% over the control (9.3%) treated with 1 × 108 conidia/mL (F8, 18 = 52.5; p = 0.0000) (Figure 3; Supplementary Table S4).
FIGURE 3. Effects of entomopathogenic fungal isolates on cumulative mortality to the eggs and neonates of fall armyworm treated with different concentrations at 14 days post treatment. Error bars denote the mean ± standard error at the 95% confidence interval. Means followed by the same letters are not significantly different by Tukey’s test at p < 0.05.
The results revealed that among all tested EPF, only Metarhizium anisopilae MA isolates caused 10.0% larval mortality of FAW over the control (1.1%) treated with 1 × 106 conidia/mL at 7 days post treatment (F8, 18 = 3.21; p = 0.0191). There was no significant difference observed by the isolates of M. anisopilae MA and P. citrinum CTD-28 over the control which caused 15.6% and 8.9% larval mortality treated with 1 × 107 conidia/mL (F8, 18 = 2.49; p = 0.0514). The isolate of M. anisopilae MA caused 24.4% larval mortality, followed by P. citrinum CTD-28 with 14.4% over the control (2.21%) treated with 1 × 108 conidia/mL (F8, 18 = 4.02; p = 0.0068). Furthermore, mycosis was observed only in 5% of the dead larvae with effective strains (Figure 4; Supplementary Table S5).
FIGURE 4. Effects of entomopathogenic fungal isolates on larval mortality of fall armyworm treated with different concentrations at 7 days post treatment. Error bars denote the mean ± standard error at the 95% confidence interval. Means followed by the same letters are not significantly different by Tukey’s test at p < 0.05.
The isolates of M. anisopliae MA and P. citrinum CTD-28 were found to be effective by reducing 52.8% and 47.2%, feeding performance of FAW larvae, respectively, followed by Penicillium sp. CTD-2 and Cladosporium sp. BM-8 isolates, which reduced by 44.4% and 40.3% feeding performance, respectively, over the control (4.2%) after treated with 1 × 106 conidia/mL at 48 h post treatment (F8, 18 = 29.5; p = 0.0000). Metarhizium anisopliae MA and P. citrinum CTD-28 isolates significantly reduced the feeding performance by 65.3% and 61.1%, respectively, followed by Penicillium sp. CTD-2 and Cladosporium sp. BM-8 isolates, which reduced the feeding performance of FAW larvae by 52.8% and 50.0%, respectively, compared to the control (4.2%) after treated with 1 × 107 conidia/mL (F8, 18 = 17.2; p = 0.0000). Metarhizium anisopliae MA and P. citrinum CTD-28 isolates outperformed all the other EPF by reducing feeding performance of 77.8% and 75.0%, respectively, followed by 68.1% and 59.7% with Penicillium sp. CTD-2 and Cladosporium sp. BM-8 isolates, respectively, over the control (4.2%) after treated with 1 × 108 conidia/mL (F8, 18 = 38.5; p = 0.0000) (Figure 5; Supplementary Table S6).
FIGURE 5. Effects of entomopathogenic fungal isolates on the feeding performance of second instar larvae of fall armyworm treated with different concentrations at 48 h post treatment. Error bars denote the mean ± standard error at the 95% confidence interval. Means followed by the same letters are not significantly different by Tukey’s test at p < 0.05.
The results indicate that all the tested EPF isolates did not show significant pupal mortality of FAW compared to the control after treated with 1 × 106 conidia/mL at 15 days posttreatment (F8, 18 = 1.19; p = 0.3591). M. anisopilae MA isolate caused pupal mortality of 13.3%, followed by the P. citrinum CTD-28 isolate with 10.0% over the control (0.0%) when treated with 1 × 107 conidia/mL (F8, 18 = 3.65; p = 0.0107). M. anisopilae MA and P. citrinum CTD-28 isolates caused 23.3% and 20.0% pupal mortality, respectively, followed by Penicillium sp. CTD-2 and Cladosporium sp. BM-8 isolates with 13.3% and 10.0% compared to the control (0.0%) treated with 1× 108 conidia/mL (F8, 18 = 5.94; p = 0.0008) (Figure 6; Supplementary Table S7).
FIGURE 6. Effects of EPF on pupal mortality of fall armyworm treated with different concentrations at 14 days post treatment. Error bars denote the mean ± standard error at the 95% confidence interval. Means followed by the same letters are not significantly different by Tukey’s test at p < 0.05.
Factorial analysis of variance revealed a significant effect of the conidial concentrations (F2, 192 = 67.62; p = 0.0000), the treatments (F7, 192 = 59.46; p = 0.0000), the life stages (F3, 192 = 191.46; p = 0.0000), and the significant interaction was observed between concentration and treatment (F14, 192 = 3.18; p = 0.0000), the concentration and life stages (F6, 192 = 5.65; p = 0.0000), the treatment and life stages (F21, 192 = 9.41; p = 0.0000), while non-significant interaction was found among concentrations, treatments and life stages (F42, 192 = 0.40; p = 0.9997) (Table 2; Supplementary Table S8).
TABLE 2. Mortality (Means ± SEs) of immature stages of fall armyworm treated with different concentrations of isolates of entomopathogenic fungi.
There are a variety of microbial pathogens that have been associated with FAW, including fungi, bacteria and viruses (Gardner et al., 1984), but only a few pathogens among them are responsible for causing infection of the pests (Polanczyk et al., 2000; Garcia et al., 2008; Negrisoli et al., 2010b; Negrisoli et al., 2010a; Behle and Popham, 2012; Salvadori et al., 2012; Gómez et al., 2013). FAW nuclear polyhedrosis virus (NPV) is reported to be one of the most important pathogens inducing significant mortality to the pest. Thus, any entomopathogen that is able to cause infection to pests before it reaches its destructive stage might play a key role in the management of insect pests. Hence, the present study focused on screening selected EPF species for the control of FAW by causing infection at any susceptible stage of life.
The eggs are the most susceptible to microbial infection and require maximum nutrients for their development (Tillman, 2010); therefore, eggs are the sensitive stage by pathogenic microorganisms (Kellner, 2002; Trougakos and Margaritis, 2002). The results of the present study revealed that FAW eggs were the most vulnerable to the tested fungal isolates. Our results are supported by previous studies where isolates of M. anisopliae and Cladosporium tenuissimum showed 96.5% and 55.6% FAW egg mortality (Akutse et al., 2019; Idrees et al., 2021). The isolate of Cladosporium sp. was significantly virulent against Helicoverpa armigera egg mortality (Bahar et al., 2011). The findings of our study are in line with those of Idrees et al. (Idrees et al., 2021), who observed that isolates of Aspergillus sp. did not induce FAW egg mortality. The highest egg mortality of Spodoptera litura was observed by Anand and Tiwary (2009), when treated with isolates of Aspergillus sp. Similar to our results, the highest egg mortality of FAW was observed with the isolates of P. citrinum and Pteroptyx bearni (Foo et al., 2017; Idrees et al., 2021). Histopathological research proved that fungal spores can successfully penetrate eggs and cause fungal infections (Pires et al., 2009; Zhang et al., 2014).
The results of our research are supported by previous findings where isolates of M. anisopliae were significantly effective in inducing FAW neonate mortality (Akutse et al., 2019), while the isolates of Aspergillus sp, C. tenuissimum and P. citrimum did not induce significant mortality against neonates of FAW (Idress et al., 2021). The isolates of P. citrinum and C. tenuissimum caused significant cumulative mortality to the eggs and neonates of FAW in the present research. The isolates of Cladosporium aphidis were significantly effective in causing cumulative mortality of aphid species (Gui et al., 2005). Although all host stages are not equally susceptible to pathogen infection, EPF have the potential to cause infection of insect pests at any stage of their life (Opisa et al., 2018).
The tested EPF were not effective against second instar larvae of FAW. The isolates of Aspergillus sp. did not cause significant larval mortality in second instar larvae of FAW and Chilo suppressalis (Idrees et al., 2021; Shahriari et al., 2021). The isolate of P. citrinum was found to be the most effective against second instar larvae of S. litura but ineffective in causing larval mortality of FAW (Herlinda et al., 2020). The isolates of P. citrinum were found to be associated with mosquito larvae (Da et al., 2009) and caused significant larval mortality against Culex quinquefasciatus (Maketon et al., 2014). Interestingly, it was observed that some of the fungal isolates showed significant effectiveness against early instar larvae, while less virulent to mature larvae; for example, the isolate of Cladosporium sp. caused significant mortality of early instar larvae compared with matured larvae of H. armigera (Bahar et al., 2011). Fewer species in the Cladosporium genus were found to be virulent against aphids and whiteflies (Abdel-Baky and Abdel-Salam, 2003; Gui et al., 2005). The isolates of M. anisopliae did not show significant mortality against second instar larvae of FAW (Akutse et al., 2019). The effectiveness level of each fungal isolate varies for causing infection against FAW (Fargues and Maniania, 1992). Therefore, this situation provides a basis for further studies to reveal the mechanisms of higher resistance in FAW larvae to the tested fungal isolates in this study. The high resistance of the mature larvae against fungal infection may be due to the larval integument that does not permit effective penetration of the fungal spore (Bosa et al., 2004). There are minimal chances of fungal infection when the inoculum is lost due to molting, even though molting does not constantly result in the prevention of fungal infection (Meekes, 2001). The low susceptibility of larvae in the present study could be a feature of the tested fungal isolates and might be attributed to genetic diversity or perhaps phenotypic differences between the populations of FAW (Monnerat et al., 2006).
The production of toxic substances by EPF inside the host body leads to mechanical disruption in insect structural integrity, which ultimately reduces the feeding performance of insect pests (Tefera and Pringle, 2003). A previous study reported a significant feeding reduction in the feeding performance of insect pests treated with different fungal isolates (Fargues et al., 1994; Ekesi and Maniania, 2000; Hussain et al., 2009; Migiro et al., 2011; Idrees et al., 2021; Idrees et al., 2022a). The reduction in feeding performance by larvae treated with fungal isolates is one of the key factors in host mortality, indicating virulence of fungal isolates, and requires further investigation to assess the level of pathogenicity or antifeedant effects (Ondiaka et al., 2008).
The previous research finding concluded that EPF did not infect to all the stages of lepidopteran pests equally and in most of the research finds have been observed that EPF have to potential to infect the earliest stages of pest effective compared with later stages (Idrees et al., 2022b). It might be the reason that the resistance developed in later mature stages of pest. The fungal isolates did not show a significant effect in causing pupal mortality of FAW in our research. The previous findings are consistent with our study results, where fungal isolates did not cause significant pupal mortality of S. litura within 14 days post treatment (Anand et al., 2009; Asi et al., 2013). The different life stages of any insect do not response to any pathogen stress the same way. So, it is ‘very normal’ to test EPF on various life stages in order to understand which life stage is most susceptible, or either way, the most resistant to the said fungal isolate. As this would inform users the most appropriate stage of the insect to target for effective biological control programs as in our previous study concluded that eggs are the most susceptible stage for target as compared to the other life stages of FAW (Aktuse et al., 2019; Idrees et al., 2021).
The M. anisopliae, P. citrinum, Penicillium sp. and Cladosporium sp. have potential to infect the immature stages and feeding performance of FAW. Therefore, these EPF could be considered for the development of microbial pesticides against FAW. Further studies are needed to insight the key toxins which are responsible for affecting the physiological function of FAW and slowing down the feeding performance.
The original contributions presented in the study are included in the article/Supplementary Material, further inquiries can be directed to the corresponding author.
All authors listed have made considerable direct or indirect and intellectual contribution to the work and have read and agreed to the manuscript.
This research work was financially supported by the Key-Area Research and Development Program of Guangdong Province (No. 2020B020223004), GDAS Special Project of Science and Technology Development (Nos. 2020GDASYL-20200301003 and 2020GDASYL-20200104025), GDAS Action Capital Project to build a comprehensive industrial technology innovation Center (No. 2022GDASZH-2022010106).
The authors declare that the research was conducted in the absence of any commercial or financial relationships that could be construed as a potential conflict of interest.
All claims expressed in this article are solely those of the authors and do not necessarily represent those of their affiliated organizations, or those of the publisher, the editors and the reviewers. Any product that may be evaluated in this article, or claim that may be made by its manufacturer, is not guaranteed or endorsed by the publisher.
The Supplementary Material for this article can be found online at: https://www.frontiersin.org/articles/10.3389/fphys.2023.1107434/full#supplementary-material
Abdel-Baky, N. F., and Abdel-Salam, A. H. (2003). Natural incidence of Cladosporium spp. as a bio-control agent against whiteflies and aphids in Egypt. J. Appl. Entomol. 127, 228–235. doi:10.1046/j.1439-0418.2003.00662.x
Ahmed, K. S., Idrees, A., Majeed, M. Z., Majeed, M. I., Shehzad, M. Z., Ullah, M. I., et al. (2022). Synergized toxicity of promising plant extracts and synthetic chemicals against fall armyworm Spodoptera frugiperda (JE smith) (Lepidoptera: Noctuidae) in Pakistan. Agronomy 12, 1289. doi:10.3390/agronomy12061289
Akutse, K. S., Kimemia, J. W., Ekesi, S., Khamis, F. M., Ombura, O. L., and Subramanian, S. (2019). Ovicidal effects of entomopathogenic fungal isolates on the invasive Fall armyworm Spodoptera frugiperda (Lepidoptera: Noctuidae). J. Appl. Entomol. 143, 626–634. doi:10.1111/jen.12634
Altinok, H. H., Mahmut, A. A., and Abdurrahman, S. K. (2019). Modes of action of entomopathogenic fungi. Curr. Trends Nat. Sci. 8, 117–124.
Anand, R., Prasad, B., and Tiwary, B. N. (2009). Relative susceptibility of Spodoptera litura pupae to selected entomopathogenic fungi. BioControl 54, 85–92. doi:10.1007/S10526-008-9157-X
Anand, R., and Tiwary, B. N. (2009). Pathogenicity of entomopathogenic fungi to eggs and larvae of Spodoptera litura, the common cutworm. Biocontrol Sci. Technol. 19, 919–929. doi:10.1080/09583150903205069
Asi, M. R., Bashir, M. H., Afzal, M., Zia, K., and Akram, M. (2013). Potential of entomopathogenic fungi for biocontrol of Spodoptera litura fabricius (Lepidoptera: Noctuidae). J. Anim. Plant Sci. 23, 913–918.
Bahar, M. H., Backhouse, D., Gregg, P. C., and Mensah, R. (2011). Efficacy of a Cladosporium sp. fungus against Helicoverpa armigera (Lepidoptera: Noctuidae), other insect pests and beneficial insects of cotton. Biocontrol Sci. Technol. 21, 1387–1397. doi:10.1080/09583157.2011.622036
Behle, R. W., and Popham, H. J. (2012). Laboratory and field evaluations of the efficacy of a fast-killing baculovirus isolate from Spodoptera frugiperda. J. Invertebr. Pathol. 109, 194–200. doi:10.1016/j.jip.2011.11.002
Bosa, C. F., ChÁVez, D., Torres, L., ParÍS, A., Villamizar, L., and Cotes, A. (2004). Evaluation of natíve isolates of Nomuraea rileyi for the control of Spodoptera frugiperda (Lepidoptera: Noctuidae). Rev. Colomb. Entomol. 30, 93–97. doi:10.25100/socolen.v30i1.9535
Cai, P., Gu, X., Yao, M., Zhang, H., Huang, J., Idress, A., et al. (2017). The optimal age and radiation dose for Bactrocera dorsalis (Hendel) (Diptera: Tephritidae) eggs as hosts for mass-reared Fopius arisanus (Sonan) (Hymenoptera: Braconidae). Biol. Control 108, 89–97. doi:10.1016/J.BIOCONTROL.2017.02.006
Cook, D. R., Leonard, B. R., and Gore, J. (2004). Field and laboratory performance of novel insecticides against armyworms (Lepidoptera: Noctuidae). Fla. Entomol. Fla. Entomol. Soc. 87, 433–439. doi:10.1653/0015-4040(2004)087[0433:FALPON]2.0.CO;2
Da, S. P. E., de, M. S. M. I., Ferreira-Keppler, R. L., Hamada, N., and Alencar, Y. B. (2009). Filamentous fungi associated with mosquito larvae (Diptera: Culicidae) in municipalities of the Brazilian amazon. Neotrop. Entomol. 38, 352–359. doi:10.1590/s1519-566x2009000300009
Dara, S. K. (2017). “Entomopathogenic microorganisms: Modes of action and role in IPM. E-Journal of Entomology and Biological,” in E-Journal of Entomology and Biological (United States: University of California Agriculture and natural blogs).
Day, R., Abrahams, P., Bateman, M., Beale, T., Clottey, V., Cock, M., et al. (2017). Fall armyworm: Impacts and implications for Africa. Outlooks Pest Manag. 28, 196–201. doi:10.1564/v28_oct_02
Ebani, V. V., and Mancianti, F. (2021). Entomopathogenic fungi and bacteria in a veterinary perspective. Biology 10, 479. doi:10.3390/biology10060479
Ekesi, S., and Maniania, N. K. (2000). Susceptibility of Megalurothrips sjostedti developmental stages to Metarhizium anisopliae and the effects of infection on feeding, adult fecundity, egg fertility and longevity. Entomol. Exp. Appl. 94, 229–236. doi:10.1046/j.1570-7458.2000.00624.x
Fargues, J., Delmas, J. C., and Lebrun, R. A. (1994). Leaf consumption by larvae of the Colorado potato beetle (Coleoptera: Chrysomelidae) Infected with the Entomopathogen, Beauveria bassiana. J. Econ. Entomol. 87, 67–71. doi:10.1093/jee/87.1.67
Fargues, J., and Maniania, N. K. (1992). Variation in susceptibility of Spodoptera littoralis (Noctuidae) to Nomuraea rileyi (hyphomycetes). Entomophaga 37, 545–554. doi:10.1007/BF02372324
Foo, K., Sathiya Seelan, J. S., and Dawood, M. M. (2017). Microfungi associated with Pteroptyx bearni (Coleoptera: Lampyridae) eggs and larvae from kawang river, sabah (northern borneo). Insects 8, 66. doi:10.3390/insects8030066
Garcia, L. C., Raetano, C. G., and Leite, L. G. (2008). Application technology for the entomopathogenic nematodes Heterorhabditis indica and Steinernema sp. (Rhabditida: Heterorhabditidae and Steinernematidae) to control Spodoptera frugiperda (Smith) (Lepidoptera: Noctuidae) in corn. Neotrop. Entomol. 37, 305–311. doi:10.1590/s1519-566x2008000300010
Gardner, W. A., Noblet, R., and Schwehr, R. D. (1984). The potential of microbial agents in managing populations of the fall armyworm (Lepidoptera: Noctuidae). Fla. Entomol. 67, 325–332.
Goergen, G., Kumar, P. L., Sankung, S. B., Togola, A., and Tamò, M. (2016). First report of outbreaks of the fall armyworm Spodoptera frugiperda (J E Smith) (Lepidoptera, Noctuidae), a new alien invasive pest in West and Central Africa. PLoS One 11, e0165632. doi:10.1371/journal.pone.0165632
Gómez, J., Guevara, J., Cuartas, P., Espinel, C., and Villamizar, L. (2013). Microencapsulated Spodoptera frugiperda nucleopolyhedrovirus: Insecticidal activity and effect on arthropod populations in maize. Biocontrol Sci. Technol. 23, 829–846. doi:10.1080/09583157.2013.802288
Gu, X., Cai, P., Yang, Y., Yang, Q., Yao, M., Idrees, A., et al. (2018). The response of four braconid parasitoid species to methyl eugenol: Optimization of a biocontrol tactic to suppress Bactrocera dorsalis. Biol. Control 122, 101–108. doi:10.1016/J.BIOCONTROL.2018.04.002
Gui, G., Yue, L., Bin, C., and Ying, W. (2005). Virulence of entomophagous fungus Cladosporium aphidis (Thuemen) against 3 aphid species. J. South. Agric. Uni. 27, 184–187.
Guo, J., Zhao, J., He, K., Zhang, F., and Wang, Z. (2018). Potential invasion of the crop-devastating insect pest fall armyworm Spodoptera frugiperda to China. Plant Prot. 44, 1–10.
Hajek, A. E. (1989). Food consumption by Lymantria dispar (Lepidoptera: Lymantriidae) larvae infected with entomophaga maimaiga (zygomycetes: Entomophthorales). Environ. Entomol. 18, 723–727. doi:10.1093/ee/18.4.723
He, L., Zhao, S., and Wu, K. (2020). Study on the damage of fall armyworm, Spodoptera frugiperda to peanut. Plant Prot. 46, 28–33.
Herlinda, S., Efendi, R. A., Suharjo, R., Hasbi, H., Setiawan, A., Elfita, E., et al. (2020). New emerging entomopathogenic fungi Isolated from soil in South Sumatra (Indonesia) and their filtrate and conidial insecticidal activity againts Spodoptera litura. J. Bio. Divers. 21, 5102–5113. doi:10.13057/biodiv/d211115
Hussain, A., Tian, M., He, Y., and Ahmed, S. (2009). Entomopathogenic fungi disturbed the larval growth and feeding performance of Ocinara varians (Lepidoptera: Bombycidae) larvae. Insect Sci. 16, 511–517. doi:10.1111/j.1744-7917.2009.01272.x
Idrees, A., Afzal, A., Qadir, Z. A., and Li, J. (2022a). Bioassays of Beauveria bassiana isolates against the fall armyworm, Spodoptera frugiperda. J. Fungi 8, 717. doi:10.3390/jof8070717
Idrees, A., Qadir, Z. A., Afzal, A., Ranran, Q., and Li, J. (2022b). Laboratory efficacy of selected synthetic insecticides against second instar invasive fall armyworm, Spodoptera frugiperda (Lepidoptera: Noctuidae) larvae. PLoS One 17, e0265265. doi:10.1371/journal.pone.0265265
Idrees, A., Qadir, Z. A., Akutse, K. S., Afzal, A., Hussain, M., Islam, W., et al. (2021). Effectiveness of entomopathogenic fungi on immature stages and feeding performance of fall armyworm, Spodoptera frugiperda (Lepidoptera: Noctuidae) larvae. Insects 12, 1044. doi:10.3390/insects12111044
Idrees, A., Qasim, M., Ali, H., Qadir, Z. A., Idrees, A., Bashir, M. H., et al. (2016). Acaricidal potential of some botanicals against the stored grain mites. Rhizoglyphus tritici. J. Entomology Zoology Stud. 4, 611–617. Available at: https://www.mendeley.com/catalogue/d3ee9586-69e5-343d-90e2-4713ae1007c4/(Accessed November 11, 2021).
Idrees, A., Zhang, H., Luo, M., Thu, M., Cai, P., Islam, W., et al. (2017). Protein baits, volatile compounds and irradiation influence the expression profiles of odorant-binding protein genes in Bactrocera dorsalis (Diptera: Tephritidae). Appl. Ecol. Environ. Res. 15, 1883–1899. doi:10.15666/AEER/1504_18831899
Kellner, R. L. L. (2002). “The role of microorganisms for eggs and progeny,” in Chemoecology of insect eggs and egg deposition. Editor T. M. Monika Hilker (Berlin): Blackwell), 149–164. doi:10.1002/9780470760253.ch6
Kumela, T., Simiyu, J., Sisay, B., Likhayo, P., Mendesil, E., Gohole, L., et al. (2019). Farmers’ knowledge, perceptions, and management practices of the new invasive pest, fall armyworm (Spodoptera frugiperda) in Ethiopia and Kenya. Int. J. Pest Manag. 65, 1–9. doi:10.1080/09670874.2017.1423129
Liu, Z. K., Li, X. L., Tan, X. F., Yang, M. F., Idrees, A., Liu, J. F., et al. (2022). Sublethal effects of emamectin benzoate on fall armyworm, Spodoptera frugiperda (Lepidoptera: Noctuidae). Agriculture 12, 959. doi:10.3390/agriculture12070959
Luo, M., Zhang, H., Du, Y., Idrees, A., He, L., Chen, J., et al. (2018). Molecular identification of cultivable bacteria in the gut of adult Bactrocera tau (Walker) and their trapping effect. Pest Manag. Sci. 74, 2842–2850. doi:10.1002/ps.5074
Maketon, M., Amnuaykanjanasin, A., and Kaysorngup, A. (2014). A rapid knockdown effect of Penicillium citrinum for control of the mosquito Culex quinquefasciatus in Thailand. World J. Microbiol. Biotechnol. 30, 727–736. doi:10.1007/s11274-013-1500-4
Meekes, E. T. M. (2001). Entomopathogenic fungi against whiteflies: Tritrophic interactions between aschersonia species, trialeurodes vaporariorum and bemisia argentifolii, and glasshouse crops. Ann Arbor, MI: Wageningen University and Research ProQuest Dissertations Publishing.
Migiro, L. N., Maniania, N. K., Chabi-Olaye, A., Wanjoya, A., and Vandenberg, J. (2011). Effect of infection by Metarhizium anisopliae (Hypocreales: Clavicipitaceae) on the feeding and oviposition of the pea leafminer Liriomyza huidobrensis (Diptera: Agromyzidae) on different host plants. Biol. Control 56, 179–183. doi:10.1016/j.biocontrol.2010.09.013
Monnerat, R., Martins, E., Queiroz, P., Ordúz, S., Jaramillo, G., Benintende, G., et al. (2006). Genetic variability of Spodoptera frugiperda Smith (Lepidoptera: Noctuidae) populations from Latin America is associated with variations in susceptibility to Bacillus thuringiensis cry toxins. Appl. Environ. Microbiol. 72, 7029–7035. doi:10.1128/aem.01454-06
Montezano, D. G., Sosa-Gómez, D. R., Specht, A., Roque-Specht, V. F., Sousa-Silva, J. C., Paula-Moraes, S. V. de, et al. (2018). Host plants of Spodoptera frugiperda (Lepidoptera: Noctuidae) in the americas. Afr. Entomol. 26, 286–300. doi:10.4001/003.026.0286
Negrisoli, A. S., Garcia, M. S., Barbosa Negrisoli, C. R. C., Bernardi, D., and da Silva, A. (2010a). Efficacy of entomopathogenic nematodes (Nematoda: Rhabditida) and insecticide mixtures to control Spodoptera frugiperda (Smith, 1797) (Lepidoptera: Noctuidae) in corn crops. Crop Prot. 29, 677–683. doi:10.1016/j.cropro.2010.02.002
Negrisoli, A. S., Garcia, M. S., and Negrisoli, C. R. C. B. (2010b). Compatibility of entomopathogenic nematodes (Nematoda: Rhabditida) with registered insecticides for Spodoptera frugiperda (Smith, 1797) (Lepidoptera: Noctuidae) under laboratory conditions. Crop Prot. 29, 545–549. doi:10.1016/j.cropro.2009.12.012
Ondiaka, S., Maniania, N. K., Nyamasyo, G. H. N., and Nderitu, J. H. (2008). Virulence of the entomopathogenic fungi Beauveria bassiana and Metarhizium anisopliae to sweet potato weevil Cylas puncticollis and effects on fecundity and egg viability. Ann. Appl. Biol. 153, 41–48. doi:10.1111/j.1744-7348.2008.00236.x
Opisa, S., du Plessis, H., Akutse, K. S., Fiaboe, K. K. M., and Ekesi, S. (2018). Effects of entomopathogenic fungi and Bacillus thuringiensis-based biopesticides on Spoladea recurvalis (Lepidoptera: Crambidae). J. Appl. Entomol. 142, 617–626. doi:10.1111/jen.12512
Pires, L. M., Marques, E. J., Wanderley-Teixeira, V., Teixeira, Á. A. C., Alves, L. C., and Alves, E. S. B. (2009). Ultrastructure of Tuta absoluta parasitized eggs and the reproductive potential of females after parasitism by Metarhizium anisopliae. Micron 40, 255–261. doi:10.1016/j.micron.2008.07.008
Polanczyk, R. A., Silva, R. F. P. da, and Fiuza, L. M. (2000). Effectiveness of Bacillus thuringiensis strains against Spodoptera frugiperda(Lepidoptera: Noctuidae). Braz. J. Microbiol. 31, 164–166. doi:10.1590/S1517-83822000000300003
Qadir, Z. A., Idrees, A., Mahmood, R., Sarwar, G., Bakar, M. A., Ahmad, S., et al. (2021). Effectiveness of different soft Acaricides against honey bee ectoparasitic mite varroa destructor (Acari: Varroidae). Insects 12, 1032. doi:10.3390/insects12111032
Rwomushana, I., Bateman, M., Beale, T., Beseh, P., Cameron, K., Chiluba, M., et al. (2018). Fall armyworm: Impacts and implications for Africa. Outlooks Pest Manag. 28 (5), 196–201. doi:10.1564/v28_oct_02
Salvadori, J. D. M., Defferrari, M. S., Ligabue-Braun, R., Yamazaki Lau, E., Salvadori, J. R., and Carlini, C. R. (2012). Characterization of entomopathogenic nematodes and symbiotic bacteria active against Spodoptera frugiperda (Lepidoptera: Noctuidae) and contribution of bacterial urease to the insecticidal effect. Biol. Control 63, 253–263. doi:10.1016/j.biocontrol.2012.08.002
Shahriari, M., Zibaee, A., Khodaparast, S. A., and Fazeli-Dinan, M. (2021). Screening and virulence of the entomopathogenic fungi associated with Chilo suppressalis walker. J. Fungi. 7, 34. doi:10.3390/jof7010034
Shapiro, S. S., and Wilk, M. B. (1965). An analysis of variance test for normality (complete samples). Biometrika 52, 591–611. doi:10.2307/2333709
Sharanabasappa, A., Kalleshwaraswamy, C. M., Asokan, R., Swamy, H. M. M., Maruthi, M. S., Pavithra, H. B., et al. (2018). First report of the fall armyworm, Spodoptera frugiperda (JE Smith)(Lepidoptera: Noctuidae), an alien invasive pest on maize in India. Pest Manag. Horti. Eco. GU 24, 23–29. Available at: http://aapmhe.in/816.
Sparks, A. N. (1979). A review of the biology of the Fall Armyworm. Fla. Entomol. 62, 82–87. doi:10.2307/3494083
Stokstad, E. (2017). New crop pest takes Africa at lightning speed. Science 356, 473–474. doi:10.1126/science.356.6337.473
Sun, X., Hu, C., Jia, H., Wu, Q., Shen, X., Zhao, S., et al. (2021). Case study on the first immigration of fall armyworm, Spodoptera frugiperda invading into China. J. Integr. Agric. 20, 664–672. doi:10.1016/s2095-3119(19)62839-x
Tefera, T., and Pringle, K. L. (2003). Food consumption by Chilo partellus (Lepidoptera: Pyralidae) larvae infected with Beauveria bassiana and Metarhizium anisopliae and effects of feeding natural versus artificial diets on mortality and mycosis. J. Invertebr. Pathol. 84, 220–225. doi:10.1016/j.jip.2003.11.001
Thomas, M. B., Blanford, S., and Lomer, C. (1997). Reduction of feeding by the variegated grasshopper, Zonocerus variegatus, following infection by the fungal pathogen. Metarhizium Flavoviride. Biocontrol Sci. Technol. 7, 327–334. doi:10.1080/09583159730730
Tillman, P. G. (2010). Parasitism and predation of stink bug (Heteroptera: Pentatomidae) eggs in Georgia corn fields. Environ. Entomol. 39, 1184–1194. doi:10.1603/en09323
Tong, P. (2000). History of maize science and technology in China. Beijing: Chinese Science and Technology Press.
Trougakos, L. P., and Margaritis, L. H. (2002). “Novel morphological and physiological aspects of insect eggs,” in Chemoecology of insect eggs and egg deposition. Editors M. Hilker, and T. Meiners (Oxford, UK: Blackwell Publishing), 3–31. doi:10.1002/9780470760253.ch1
Xu, P., Zhang, D., Wang, J., Wu, K., Wang, X., Wang, X., et al. (2019). The host preference of Spodoptera frugiperda on maize and tobacco. Plant Prot. 45, 61–64.
Yang, X. L., Liu, Y. C., Luo, M. Z., Li, Y., Wang, W. H., Wan, F., et al. (2019). Spodoptera frugiperda (JE Smith) was first discovered in Jiangcheng county of Yunnan province in southwestern China. Yunnan Agric. 1, 72.
Yang, X., Sun, X., Zhao, S., Li, J., Chi, X., Jiang, Y., et al. (2020). Population occurrence, spatial distribution and sampling technique of fall armyworm Spodoptera frugiperda in wheat fields. Plant Prot. 46, 10–16. doi:10.16688/j.zwbh.2019586-en
Zhang, D., Zhao, S., Wu, Q., Li, Y., and Wu, K. (2021). Cold hardiness of the invasive fall armyworm, Spodoptera frugiperda in China. J. Integr. Agric. 20, 764–771. doi:10.1016/S2095-3119(20)63288-9
Zhang, L., Jin, M., Zhang, D., Jiang, Y., Liu, J., Wu, K., et al. (2019). Molecular identification of invasive fall armyworm Spodoptera frugiperda in Yunnan Province. Plant Prot. 45, 19–24. doi:10.16688/j.zwbh.2019121-en
Keywords: fall army worm, egg mortality, neonate mortality, feeding performance, entomopathogenic fungi
Citation: Idrees A, Afzal A, Qadir ZA and Li J (2023) Virulence of entomopathogenic fungi against fall armyworm, Spodoptera frugiperda (Lepidoptera: Noctuidae) under laboratory conditions. Front. Physiol. 14:1107434. doi: 10.3389/fphys.2023.1107434
Received: 24 November 2022; Accepted: 08 February 2023;
Published: 08 March 2023.
Edited by:
Asad Ali, Abdul Wali Khan University, PakistanReviewed by:
Asem Saad Saad Elabasy, Agricultural Research Center, EgyptCopyright © 2023 Idrees, Afzal, Qadir and Li. This is an open-access article distributed under the terms of the Creative Commons Attribution License (CC BY). The use, distribution or reproduction in other forums is permitted, provided the original author(s) and the copyright owner(s) are credited and that the original publication in this journal is cited, in accordance with accepted academic practice. No use, distribution or reproduction is permitted which does not comply with these terms.
*Correspondence: Jun Li, anVubEBnaWFici5nZC5jbg==
Disclaimer: All claims expressed in this article are solely those of the authors and do not necessarily represent those of their affiliated organizations, or those of the publisher, the editors and the reviewers. Any product that may be evaluated in this article or claim that may be made by its manufacturer is not guaranteed or endorsed by the publisher.
Research integrity at Frontiers
Learn more about the work of our research integrity team to safeguard the quality of each article we publish.