- 1Department of Emergency Medicine, Henry Ford Hospital, Detroit, MI, United States
- 2Department of Internal Medicine, Henry Ford Hospital, Detroit, MI, United States
- 3Department of Medical Education, Indiana University School of Medicine, Notre Dame Campus, South Bend, IN, United States
- 4Department of Surgery, Ernest E. Moore Shock Trauma Center at Denver Health, University of Colorado, Denver, CO, United States
- 5Department of Transplant Surgery, Denver Health and University of Colorado Health Sciences Center, Denver, CO, United States
- 6Division of Hematology and Oncology, Department of Medicine, Northwestern University Feinberg School of Medicine, Chicago, IL, United States
- 7Division of Critical Care, Department of Medicine, Mayo Clinic Arizona, Phoenix, AZ, United States
- 8Division of Pulmonary and Critical Care, Department of Medicine, University of Maryland School of Medicine, Baltimore, MD, United States
- 9Department of Trauma Surgery, Memorial Leighton Trauma Center, South Bend, IN, United States
- 10Department of Medicine, McMaster University, Hamilton, ON, Canada
- 11Thrombosis and Atherosclerosis Research Institute, Hamilton, ON, Canada
- 12Cardinal Flow Assurance LLC, Mishawaka, IN, United States
- 13Departments of Emergency Medicine and Internal Medicine, Saint Joseph Regional Medical Center, Mishawaka, IN, United States
Irrespective of the reason for hypoperfusion, hypocoagulable and/or hyperfibrinolytic hemostatic aberrancies afflict up to one-quarter of critically ill patients in shock. Intensivists and traumatologists have embraced the concept of SHock-INduced Endotheliopathy (SHINE) as a foundational derangement in progressive shock wherein sympatho-adrenal activation may cause systemic endothelial injury. The pro-thrombotic endothelium lends to micro-thrombosis, enacting a cycle of worsening perfusion and increasing catecholamines, endothelial injury, de-endothelialization, and multiple organ failure. The hypocoagulable/hyperfibrinolytic hemostatic phenotype is thought to be driven by endothelial release of anti-thrombogenic mediators to the bloodstream and perivascular sympathetic nerve release of tissue plasminogen activator directly into the microvasculature. In the shock state, this hemostatic phenotype may be a counterbalancing, yet maladaptive, attempt to restore blood flow against a systemically pro-thrombotic endothelium and increased blood viscosity. We therefore review endothelial physiology with emphasis on glycocalyx function, unique biomarkers, and coagulofibrinolytic mediators, setting the stage for understanding the pathophysiology and hemostatic phenotypes of SHINE in various etiologies of shock. We propose that the hyperfibrinolytic phenotype is exemplified in progressive shock whether related to trauma-induced coagulopathy, sepsis-induced coagulopathy, or post-cardiac arrest syndrome-associated coagulopathy. Regardless of the initial insult, SHINE appears to be a catecholamine-driven entity which early in the disease course may manifest as hyper- or hypocoagulopathic and hyper- or hypofibrinolytic hemostatic imbalance. Moreover, these hemostatic derangements may rapidly evolve along the thrombohemorrhagic spectrum depending on the etiology, timing, and methods of resuscitation. Given the intricate hemochemical makeup and changes during these shock states, macroscopic whole blood tests of coagulative kinetics and clot strength serve as clinically useful and simple means for hemostasis phenotyping. We suggest that viscoelastic hemostatic assays such as thromboelastography (TEG) and rotational thromboelastometry (ROTEM) are currently the most applicable clinical tools for assaying global hemostatic function—including fibrinolysis—to enable dynamic resuscitation with blood products and hemostatic adjuncts for those patients with thrombotic and/or hemorrhagic complications in shock states.
1 Introduction: “The machinery of life has been rudely unhinged”
Of the many descriptions of shock, the first by Samuel Gross in the year 1882 as when “the machinery of life has been rudely unhinged” remains concise and accurate (Millham, 2010). This narrative review expands upon the classic descriptions of shock to elucidate the unifying potential for SHock-INduced Endotheliopathy (SHINE) to explain the hemostatic aberrancies observed in all etiologies of progressive shock. Approximately one-quarter of severely injured trauma patients develop hemostatic derangement with an associated increased mortality of three to four times higher than those without coagulopathy. Increased mortality is also noted for critically ill patients in shock with coagulopathies not caused by trauma (Brohi et al., 2003; Adrie et al., 2004; Hess et al., 2008; Johansson et al., 2011a; Gando et al., 2011; Holcomb et al., 2012; Angus and Van der Poll, 2013; Kim et al., 2013; Wada, 2017; Bugaev et al., 2020; Walsh et al., 2020b; Iba and Levy, 2020; Moore et al., 2020). Due to its ubiquitous distribution, substantial surface area, and interfacing role in hemostasis, immunology, and blood flow, the endothelium serves as a common foundation for hemostatic derangement associated with all forms of shock (Fishman, 1982; Aird, 2004; Johansson et al., 2017a).
Here, the physiologic roles of the endothelium are detailed with particular emphasis on coagulofibrinolytic balance, glycocalyx function, and unique biomarkers. Understanding these endothelial functions offers insight to the pathophysiologic anomalies at the level of the endothelium in various forms of shock. Throughout this review, the viscoelastic hemostatic assays (VHAs) thromboelastography (TEG) and rotational thromboelastometry (ROTEM) receive particular attention for their ability to globally assay coagulofibrinolysis, thus enabling a precision-based medicine (PBM) approach to diagnosing and treating the spectrum of coagulopathies associated with SHINE (Stettler et al., 2019). First, we briefly review the classic anatomic and pathophysiologic definitions of shock.
1.1 Epidemiology and classic categorization of shock
Among the etiologies of shock, septic and cardiogenic shock are the most common with an incidence of 171 per 100,000 population and 51.7 per 100,000 population, respectively (Dupuis et al., 2020; Schrage et al., 2021). While the incidence of septic and cardiogenic shock increases annually, the in-hospital mortality rate is slowly decreasing but remains high at 34% and 37%, respectively (Paoli et al., 2018; Osman et al., 2021).
Shock may be diversely defined, all definitions of which approximate the imbalance between tissue oxygen supply and demand (Millham, 2010; Johansson et al., 2017a; Standl et al., 2018). Shock has traditionally been defined by four categories based on fluid compartment volume loss (hypovolemic), volume redistribution (distributive), cardiac pump activity (cardiogenic), and circulatory obstruction (obstructive). These four etiologies of shock are summarized in Figure 1. Briefly, distributive shock pertains to the redistribution of fluid out of the intravascular space and/or away from vital organs without blood or fluid loss. Distributive shock, particularly septic shock, accounts for 59%–66% of all shock presentations (Vincent and De Backer, 2013; Standl et al., 2018). There are three subtypes of distributive shock which include septic, neurogenic, and anaphylactic/anaphylactoid (Standl et al., 2018). Primary endothelial dysfunction (as a root cause of shock) may be a key element of distributive shock and as such, primary endotheliopathy in this type of shock may be further amplified by SHINE. Hypovolemic shock results from a loss of blood or plasma and comprises an estimated 16%–27% of patients in shock (Vincent and De Backer, 2013; Standl et al., 2018). Hypovolemic shock includes four subtypes including hemorrhagic shock, traumatic hemorrhagic shock, plasma loss (non-hemorrhagic) hypovolemic shock, and traumatic hypovolemic shock (Standl et al., 2018). Whole blood loss occurs in hemorrhagic states such as trauma, gastrointestinal bleeds, and obstetrical hemorrhage. Plasma loss occurs with dehydrating conditions such as burns, pancreatitis, and diarrhea. Cardiogenic shock arises from primary pump failure of the heart and accounts for an estimated 13%–16% of shock states (Vincent and De Backer, 2013; Standl et al., 2018). Heart conditions that commonly account for cardiogenic shock include acute myocardial infraction, heart failure, arrythmia, and defective valves. Obstructive shock arises from a blockage of the circulation and accounts for 1%–2% of shock presentations (Vincent and De Backer, 2013; Standl et al., 2018). The defining treatment for obstructive shock is to find the source of obstruction (e.g., cardiac tamponade, tension pneumothorax, or pulmonary embolism) and relieve it.
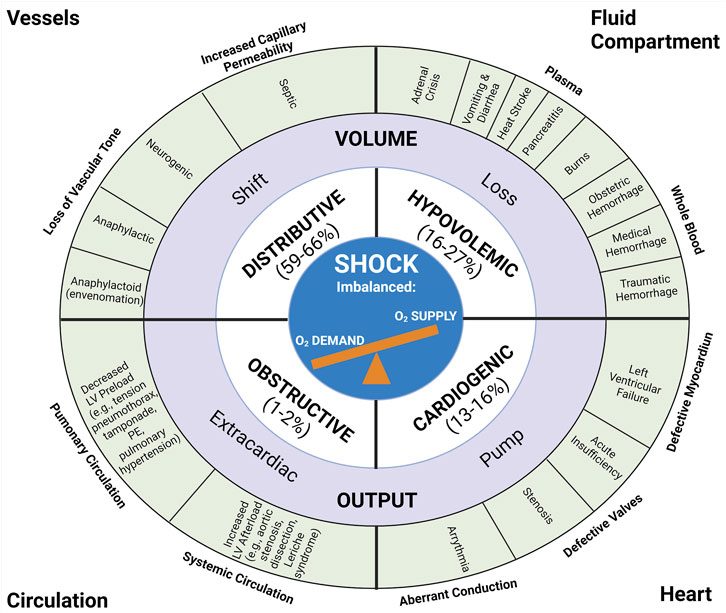
FIGURE 1. Anatomic and Pathophysiologic Categorization of Shock with Relative Frequency of Each Type. The traditional classification of shock includes four main categories: distributive, hypovolemic, obstructive, and cardiogenic shock. The estimated relative frequency of each of the four subcategories are listed with pathophysiologic etiologies defined around the periphery of the diagram (Vincent and De Backer, 2013; Standl et al., 2018). Recreated with permission from (Standl et al., 2018). Created with BioRender.com.
1.2 Limitation of the anatomic and pathophysiologic classification system
This classification precludes a unified approach towards the spectrum of coagulopathies associated with shock. As opposed to the classic anatomic and pathophysiologic definitions, shock may also be described at the level of the endothelium (Fishman, 1982; Aird, 2004). The shared endothelial dysfunction in shock has recently been termed SHINE (Johansson et al., 2017a), whereby the endothelium is acknowledged as an independent “organ” which requires resuscitation in severe injury or disease. SHINE is a catecholamine-driven entity, regardless of the initial anatomic or pathophysiologic insult, which early in the disease course may manifest as hyper- or hypocoagulopathic and hyper- or hypofibrinolytic hemostatic imbalance. Moreover, these hemostatic derangements may rapidly evolve into phenotypes along the thrombohemorrhagic spectrum depending on the etiology, timing, and methods of resuscitation (Johansson et al., 2017a; Wei et al., 2018; Napolitano, 2021; Kregel et al., 2022).
1.3 Physiology of the endothelium
The definition of an organ requires a collection of tissues that form a unit distinct in form and function (Aird, 2004). Endothelial cells uniquely function as stress-sensing, phenotype-switching cells that respond to flow. The endothelium forms an extensive network with a collective weight of around 1 kg. In the brain alone, microvasculature represents 3%–4% of the brain compartment with a cumulative length of 400 miles and a surface area of exchange of 20 m2 between the brain parenchyma and blood (Pardridge, 2002; Deracinois et al., 2015; Goncharov et al., 2017). The endothelium is comprised of a luminal glycocalyx layer maintained by simple squamous endothelial cells held together by complex transmembrane and cytoskeletal components which form intercellular junctions (Reiterer and Branco, 2020). The endothelium has distinct biochemical markers including E-selectin, intercellular adhesion molecule-1 (ICAM-1), the syndecan (Syn) family of four (Syn1-Syn4) heparan sulfate proteoglycans (HSPGs), and angiopoietin (Agpt)-1 and Agpt-2 (Goncharov et al., 2017). The endothelial luminal layer also contains anti-thrombogenic factors such as antithrombin III (AT), thrombomodulin (TM), tissue factor pathway inhibitor (TFPI), and endogenous heparan sulfates (HS) (Reitsma et al., 2007). Far from an inert layer of cells lining all blood and lymphatic vessels, the endothelium plays a vital role in moderating Starling Forces, mounting or attenuating an immune response, modulating vascular resistance, angiogenesis, and regulating coagulofibrinolysis. This is all facilitated by the rapid physical (shear; pressure; contractions/dilations to maintain vascular tone), chemical (manufacture and release of coagulofibrinolytic agents, also in response to physical and electrical stimuli; control of vascular surface/adhesive chemistry and morphology) and electrical (cell activation via central/peripheral nervous system; intercellular communication and response via chemical/ionic configurations) feedback mechanisms central to the endothelium’s role in maintaining hemostasis.
The endothelium is functionally and physically distinct and should thus be regarded as an independent organ system (Aird, 2004). Figure 2 depicts the physiology of the endothelium salient to this review. Table 1 further details biomarkers unique to the endothelial glycocalyx and endothelial-derived coagulofibrinolytic mediators.
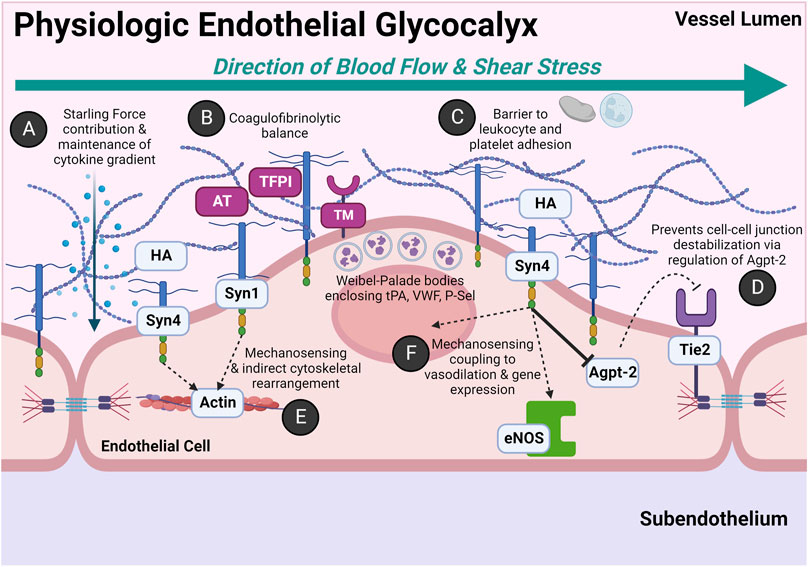
FIGURE 2. Physiologic Roles of the Endothelial Glycocalyx: An Anti-thrombogenic and Anti-adhesive Surface with Rapid Stress-sensing Capability. The glycocalyx is comprised of heparan sulfate proteoglycans (HSPGs) and glycosaminoglycans (GAGs). The HSPGs include the four transmembrane syndecans (Syn1-Syn4) and glypican. The primary GAGs include heparan sulfate (HS) and hyaluronan (HA). HS accounts for ∼50% of the GAG composition in the glycocalyx and covalently bonds to the Syn family and glypican. Other GAGs not pictured include keratan sulfate, dermatan sulfate, and chondroitin sulfate. (A) The negatively charged, hydrophilic moieties of HS and HA have variable cleavage lengths and post-translational modifications which confer significant degrees of specificity for binding cytokines and chemokines. This moderates oncotic and hydrostatic contributions of Starling Forces. Additionally, this creates a gradient of growth factors and signaling molecules which can be indirectly altered by constitutive or stress-induced transient changes in HSPG and GAG composition either by increased synthesis or enzymatic cleavage. (B) The endothelial glycocalyx maintains a gradient of endothelial-synthesized anticoagulant coagulofibrinolytic mediators. In addition to HS, antithrombin III (AT) and tissue factor pathway inhibitor (TFPI) are constitutively expressed in the endothelial glycocalyx. AT complexes with HS to inactivate many coagulation factors, primarily thrombin and Factor X. The anticoagulant TFPI complexes with and inactivates tissue factor (TF)-FVII complex and prothrombinase complex (FVa-FXa). The endothelium also constitutively expresses membrane-bound receptor thrombomodulin (TM), which in the presence of its ligand thrombin, activates circulating plasma protein C. Activated protein C inactivates circulating Factors V and VIII and also inhibits the anti-fibrinolytic, plasminogen activator inhibitor-1 (PAI-1). Weibel-Palade bodies (WPB) also play a significant role in coagulofibrinolytic balance, particularly for the activated endothelium (e.g., by endothelial agonists such as circulating plasma epinephrine). Seminal hemostatic mediators in WPB include tissue plasminogen activator (tPA), von Willebrand Factor (VWF), and P-selectin (P-Sel). (C) The glycocalyx serves as a physical barrier to leukocyte and platelet adhesion in the event adhesion molecule expression is induced on the endothelial luminal surface (e.g., P-selectin, ICAM-1, VCAM-1). (D) Syn4 via intracellular syntenin and synectin indirectly regulates angiopoietin-2 (Agpt-2) activity. High Agpt-2 levels antagonize the tyrosine kinase receptor Tie2, which subsequently destabilizes endothelial cell-cell junctions. Agpt-2 may also be found in WPBs. (E) Syn1 and Syn4 transcellularly signal luminal shear stress to rearrange the endothelial cytoskeleton. (F) Shear stress signaling by the intracellular domain of Syn4 also induces vasodilation via activation of endothelial nitric oxide synthase (eNOS). Shear stress also increases nuclear expression of genes such as those implicated in inflammation. For example, increased shear stress has shown to induce VCAM-1 and ICAM-1 expression. (Lindahl et al., 1998; Kolářová et al., 2014; Rayahin et al., 2015; Leligdowicz et al., 2018; Richter and Richter, 2019; Woodcock and Michel, 2021; Neubauer and Zieger, 2022). Abbreviations: Agpt-2, Angiopoietin-2; AT, Antithrombin III; eNOS, Endothelial Nitric Oxide Synthase; HA, Hyaluronan; Syn1, Syn4, Syndecan; TFPI, Tissue Factor Pathway Inhibitor; Tie2, tyrosine kinase receptor Tie2; TM, Thrombomodulin. Created with BioRender.com.
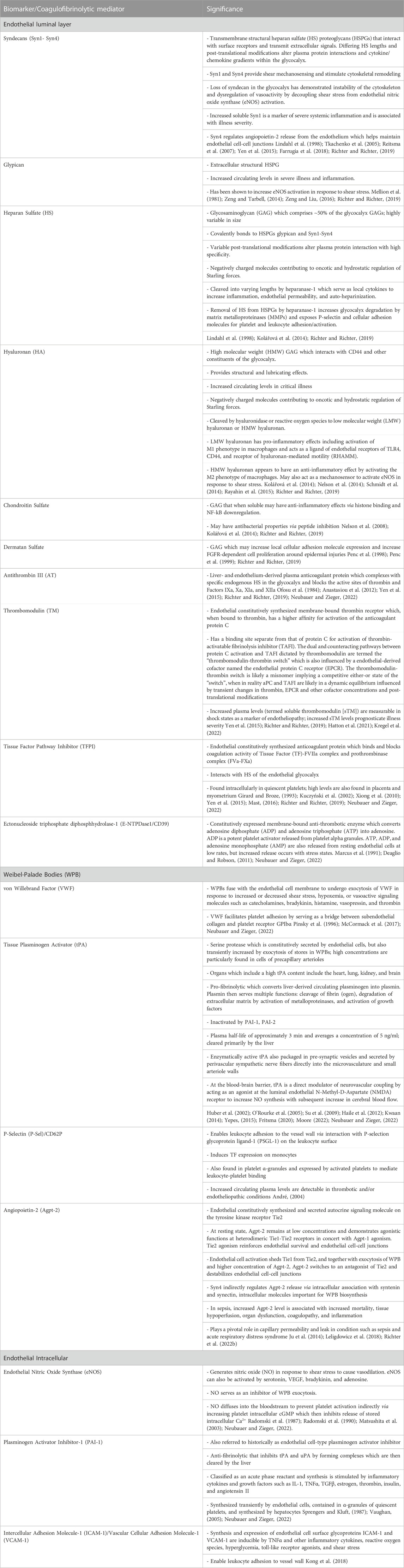
TABLE 1. Endothelial Biomarkers and Coagulofibrinolytic Mediators and their Physiologic, Pathophysiologic, and Clinical Significance.
How this large, complex multifarious system communicates nearly immediately across its vast length and surface area is an impressive capability, the understanding of which is still growing, and which finds importance in the speed with which a patient with SHINE can either deteriorate or respond to early resuscitation. The collective endothelial surface of up to 7,500 m2 transmits messages from one part of the body to another via many pathways (Dobson et al., 2015). The first and most obvious pathway is the transmission of a drop in pressure from one vascular bed to another which is nearly immediate in a closed pressure system. Additionally, it has been demonstrated that direct electrical stimulation of the microvasculature causes local vasoconstriction; however, the endothelium and subendothelial smooth muscle cells propagate the depolarization along the vessel axis to cause long distance vasodilation primarily mediated by voltage-gated calcium channels and increased nitric oxide generation induced by the calcium influx (Figueroa et al., 2007).
The nearly immediate long distance signaling by the endothelium has also been observed for fibrinolysis. Hau Kwaan first noted in the 1950s that stimulation of one venous segment elicited fibrinolytic activities from another vein located far from the site of stimulation (Kwaan, 2014). This implied that there was a possible transmission of hemostatic and inflammatory markers to the intravascular space which can alter endothelial physiology at sites distant from focal injury. However, it later became apparent that perivascular sympathetic pathway activity was another pathway responsible for near-immediate signal transmission (O'Rourke et al., 2005; Kwaan, 2014). Therefore, the transmission of information regarding inflammation and thrombosis in SHINE is a function of not only the standard macrovascular and microvascular pressure gradients, and concomitant shear and blood chemistry, but also of the microvascular Starling forces and sympathetic electrical activity which in concert achieve a delicate immuno-thrombotic balance designed to simultaneously preserve microvascular flow and tissue perfusion during the development of SHINE.
Even though the concept of SHINE has only recently been proposed, earlier literature eloquently defined the endothelium’s importance. In 1982, Alfred Fishman wrote: “In recent years, as the tempo of fresh insights into the complexity of the endothelium has increased, realization has dawned that instead of serving simply as an inert barrier between blood and tissues, the endothelium is a distributed organ of considerable biological potential that not only extends throughout the body in the convenient form of an anti-thrombogenic vascular lining but also performs other distinctive biologic functions at different vascular sites and individual organs” (Fishman, 1982). For example, the endothelium of the pulmonary capillaries has evolved to enhance gas and water exchange while the endothelium of the aorta has evolved to withstand the high pressures exerted by the pumping force of the heart. This “organ” of large surface area has important physiologic functions such as maintaining blood viscosity, providing nutrients, modulating vasomotor function, and participating in immunological surveillance by maintaining innate and acquired immunity while orchestrating a communication between tissue perfusion and tissue flow (Fishman, 1982; Aird, 2006; Johansson et al., 2017a).
2 Pathophysiology of SHINE
Determinants of blood flow include the vessel cross-sectional area, perfusion pressure, and blood viscosity (Rosato et al., 1968). Adaptive responses to decreased perfusion often become maladaptive in the shock state. For example, patients in septic shock experience systemic vasodilation which worsens tissue perfusion and warrants treatment with fluids and vasopressors. Similarly, hypocoagulability and hyperfibrinolysis in patients with shock may represent a maladaptive attempt to restore perfusion by decreasing blood viscosity against a pro-thrombotic endothelium.
Critically ill patients in shock demonstrate endothelial injury and hypocoagulability/hyperfibrinolysis directly proportional to the severity of disease and can be predicted by injury severity score (ISS) or base deficit (BD) in trauma (Johansson et al., 2010; Johansson and Ostrowski, 2010; Moore et al., 2016b; Johansson et al., 2017c), the Sequential Organ Failure Assessment (SOFA) score in septic patients (Ostrowski et al., 2015; Mochizuki et al., 2018; Iba et al., 2019a; Berthelsen et al., 2019; Bestle et al., 2020), and time until return of spontaneous circulation (ROSC) in patients who suffer from PCAS (White et al., 2011; Wada, 2017). Sympatho-adrenal activation (as measured by increased plasma catecholamines) likewise associates with the degree of endothelial injury, increasing injury/disease severity, and mortality risk (Dolgov et al., 1984; Makhmudov et al., 1985; Johansson et al., 2012; Johansson et al., 2015; Jung et al., 2015; Ostrowski et al., 2015; Di Battista et al., 2016; Johansson et al., 2017b). It has been posited that catecholamines, particularly the vasoconstrictive effect of norepinephrine, enact dose-dependent damage upon the endothelium to cause SHINE (Dolgov et al., 1984; Makhmudov et al., 1985; Kristová et al., 1993; Vischer and Wollheim, 1997; Johansson and Ostrowski, 2010). Beyond circulating endocrine catecholamines, peripheral sympathetic nerves may directly activate the endothelium and additional layers of blood vessels via neurotransmitter catecholamines (Peng et al., 2000). Perivascular sympathetic nerves can also release proteins such as tPA into small vessel walls and directly into microcirculation, serving as a backup of the endothelium to balance hemostasis (O'Rourke et al., 2005; Kwaan, 2014). tPA release may also be related to hypoperfusion (and sensing of decreased shear) independent of hypoxemia. Differentiating the effects of circulating catecholamines versus direct microvascular innervation in driving SHINE have not been previously evaluated and warrants future investigation. Additionally, whether increased catecholamines and SHINE are correlational markers of organ injury or causal pathophysiologic drivers remains the subject of further study. Figure 3 represents the prevailing pathophysiologic mechanism of SHINE.
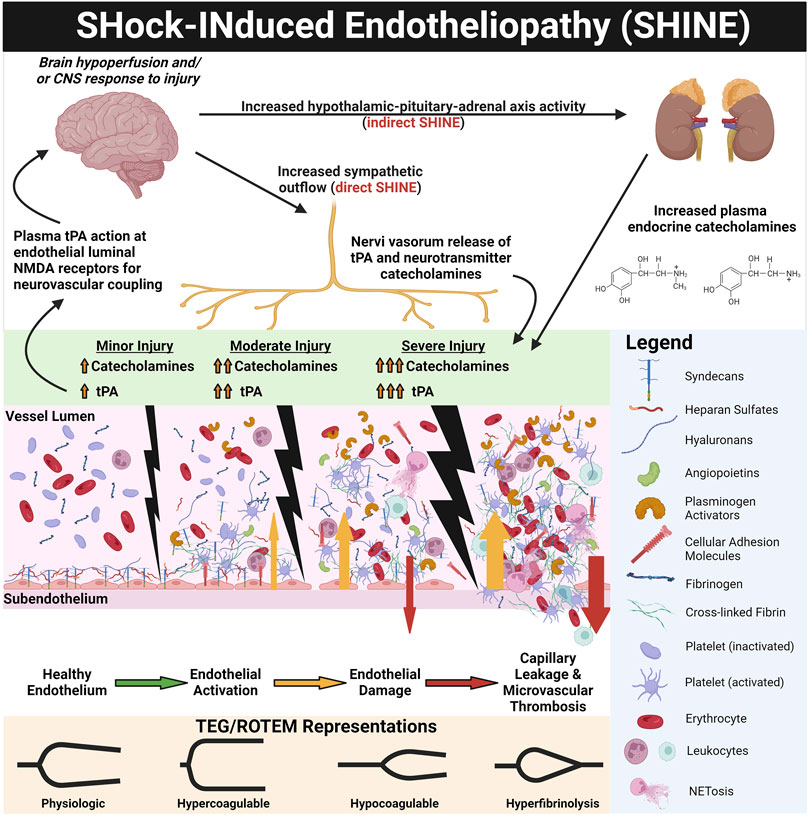
FIGURE 3. SHock-INduced Endotheliopathy (SHINE) as a Reflection of Injury Severity. Increasing sympatho-adrenal activation with increasing injury and shock severity leads to endothelial activation and damage. Increased sympathetic outflow directly provokes SHINE via perivascular sympathetic nerve exocytosis of neurotransmitter catecholamines and enzymatically active tissue plasminogen activator (tPA) into the vessel walls and directly into the microvasculature (O'Rourke et al., 2005; Kwaan, 2014). Hypothalamic-pituitary-adrenal axis activity also increases circulating plasma catecholamines. The corresponding endothelial and hemostatic changes are dose-dependent to injury/shock severity, as measured by endothelial biomarkers (e.g., plasma syndecan-1 and soluble thrombomodulin) and on thromboelastography (TEG) and rotational thromboelastometry (ROTEM) tracings. For example, with trauma, TEG/ROTEM tracings progress from physiologic hemostasis to hypercoagulable in mild trauma, to hypocoagulable in moderate trauma, and finally hyperfibrinolytic in severe trauma (Johansson and Ostrowski, 2010). Genetically preserved responses to critically ill patients inflicted by trauma, burns, and sepsis are similar, suggesting early responses to shock are evolutionarily preserved wherein SHINE may be a unifying mechanism (Xiao et al., 2011; Johansson et al., 2017a). The catecholaminergic surge (in particular the vasoconstrictive action of norepinephrine) causes glycocalyx shedding, endothelial injury, and de-endothelialization of perfused vessels (Dolgov et al., 1984; Makhmudov et al., 1985; Kristová et al., 1993; Vischer and Wollheim, 1997). The activated/injured endothelium promotes thrombosis, causing occlusion of the microvasculature. Together with capillary leak, perivascular edema, and vasoconstriction, these vascular responses provoke a cycle of progressive tissue hypoperfusion, hypovolemia, organ injury, and increasing sympatho-adrenal activation (Opal and Van Der Poll, 2015; Johansson et al., 2017a). It has been hypothesized that the ensuing hypocoagulability and hyperfibrinolysis may be a compensatory counterbalance to the pro-thrombotic endothelium in an attempt to maintain patency of the microvasculature (Johansson and Ostrowski, 2010). Therefore, the two major hemostatic compartments—the endothelium and the blood—may “switch” phenotypes in some progressing shock states. Whereby the physiologic endothelium acts as anti-thrombogenic surface to oppose coagulable blood, in shock, the roles may switch to a pro-thrombotic endothelium with a hypocoagulable/hyperfibrinolytic blood phenotype in attempt to rebalance hemostasis, decrease the blood viscosity, and restore perfusion (Johansson and Ostrowski, 2010) (see Figure 4). Not only does tPA exert pro-fibrinolytic activity via enzymatic activation of plasminogen, but tPA in the brain uniquely acts as a signaling agonist on the N-Methyl-D-Aspartate (NMDA) receptor on the endothelial luminal surface of small cerebrovascular arterioles. The activated NMDA receptor increases synthesis of nitric oxide to cause vasodilation and increase cerebral blood flow (Su et al., 2009; Haile et al., 2012; Yepes, 2015). Thus, increased free tPA (that is, free from complexes with PAI-1 and other inhibitors) in shock states may simultaneously increase systemic perfusion via fibrinolysis of occlusive thrombi and as a neurovascular coupling agent to increase cerebral blood flow (Su et al., 2009; Yepes, 2015). Created with BioRender.com.
Hypocoagulability/hyperfibrinolysis in shock states also correlates to biomarkers of endothelial glycocalyx derangement, such as increased circulating Syn-1, soluble TM (sTM), P-selectin, and an increased ratio of Agpt-2:Agpt-1 (Ostrowski et al., 2015; Johansson et al., 2017a; Johansson et al., 2017b). Glycocalyx disruption and endotheliopathy contributes to a cycle of increasing tissue hypoxia, capillary leak, micro-thrombosis, organ failure, and mortality in patients with shock (Faust et al., 2001; Adrie et al., 2002; Adrie et al., 2004; Neumar et al., 2008; Johansson and Ostrowski, 2010; Gando et al., 2011; Holcomb, 2011; Cohen et al., 2012; Levi et al., 2012; Angus and Van der Poll, 2013; Kim et al., 2013; Cohen et al., 2015; Opal and Van Der Poll, 2015; Johansson et al., 2017a). Thus, the hypocoagulable and hyperfibrinolytic state in progressive shock may be a counterbalance to the pro-thrombotic endothelium in an attempt to restore perfusion to vital organs (Johansson and Ostrowski, 2010). This counterbalance may be viewed as an attempt to rebalance hemostasis by switching phenotypes of the endothelium and blood (Johansson and Ostrowski, 2010). In homeostasis, the endothelium is an anti-thrombogenic and anti-adhesive surface to balance with the coagulable blood. These phenotypes may “switch” in SHINE whereby the hypocoagulable/hyperfibrinolytic blood may counteract the pro-thrombotic endotheliopathy. This may be rationalized as the physiologically sequestered anti-thrombogenic players (e.g., endogenous heparans, TM, tPA) being released from the activated/injured endothelium into the bloodstream (Figure 4). In turn, sTM promotes hypocoagulability via increased activation of protein C to activated protein C (aPC) which then inactivates Factor V (FV) and Factor VIII (FVIII). APC also inhibits plasminogen activator inhibitor-1 (PAI-1). The increase in tPA may also overwhelm circulating PAI-1 levels to provoke a pro-fibrinolytic phenotype. The glycocalyx layer may also become hyperpermeable because of sialidase-mediated disruption of the endothelial border in shock. Although not particularly evaluated for their role in shock, it has been reported that sialic acid residues embedded in the glycocalyx layer regulate the permeability of microvascular structures (Betteridge et al., 2017).
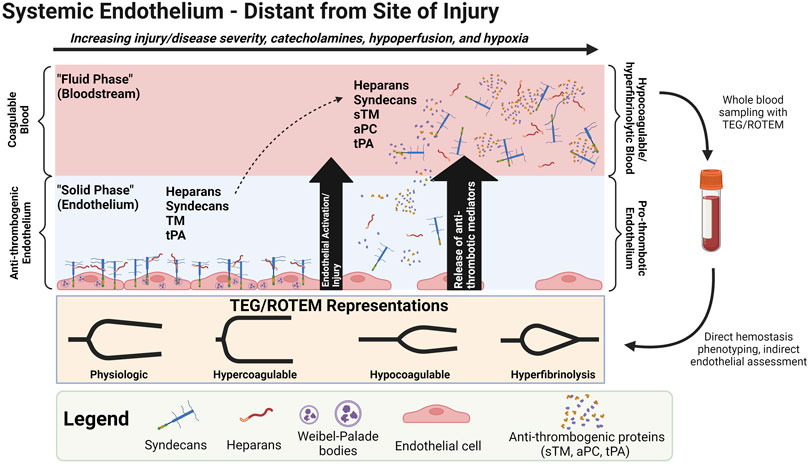
FIGURE 4. Shock-INduced Endotheliopathy (SHINE) “Phenotype Switching” via Release of Anti-thrombogenic Mediators from the Endothelium to the Bloodstream. One possible contributor of the hypocoagulable/hyperfibrinolytic phenotype in progressive shock may be the release of physiologically endothelial-sequestered anti-thrombogenic mediators to the bloodstream during SHINE when the endothelium is systemically activated and/or injured. Note that protein C is physiologically a plasma protein, but increases in soluble thrombomodulin (sTM) may increase the conversion of protein C to activated protein C (aPC). (Johansson and Ostrowski, 2010). Abbreviations: aPC, activated Protein C; ROTEM, Rotational Thromboelastometry; sTM, soluble Thrombomodulin; TEG, Thromboelastography; tPA, tissue Plasminogen Activator. Created with BioRender.com.
Moreover, tPA uniquely in the cerebrovasculature may counteract the vasconstrictive effects of norepinephrine to restore brain perfusion via tPA’s agonist action upon the N-Methyl-D-Aspartate (NMDA) receptor on the endothelial luminal surface of the blood-brain barrier (BBB), causing NO synthesis and vasodilation of cerebral arterioles (Su et al., 2009; Yepes, 2015). Thus, sympatho-adrenal-driven increases in tPA elicits at least two mechanisms to increase perfusion in shock states: 1) increased fibrinolysis to decrease blood viscosity and lyse occlusive thrombi, and 2) increased cerebral blood flow via direct cerebral arteriolar vasodilation.
Recently, increased attention to SHINE has elevated the perspective of the endothelium not merely as an anatomic entity, but as a unique organ with unique functions and biomarkers of injury which requires restoration in severe injury and disease (Aird, 2006; Dobson et al., 2015; Johansson et al., 2017a). The systems hypothesis of trauma (SHOT) has questioned the increasingly reductionist approach to trauma resuscitation, highlighting that hemorrhaging trauma patients are still expiring perhaps due to overemphasis on symptomatic care rather than addressing the underpinning system derangements associated with severe injury (Dobson et al., 2022). SHOT posits the endothelium as the “systems integrator” critical for veno-arterial coupling and preserving the blood-brain barrier essential for maintaining the brain’s privilege over the entire body. Thus, the endothelium may be one system which necessitates resuscitation to switch from the injury phenotype to that of survival (Dobson et al., 2022).
The depth and duration of shock may be evaluated with endothelial biomarker assessment and adjunctive VHAs to guide early restorative therapies. For example, in SHINE associated with TIC, prehospital transfusion of plasma demonstrates a protective effect on the injured endothelium with salutary restitution of the glycocalyx layer for patients who required massive transfusion (MT) (Moore et al., 2018; Sperry et al., 2018; Pusateri et al., 2020). Similar salutary benefit has shown to prevent endothelial damage in early sepsis when these patients are administered therapeutic plasma exchange (TPE) with fresh frozen plasma (Drost et al., 2021; Pape et al., 2021; Stahl et al., 2021). Heparanase-1 is a primary mediator for endothelial injury particularly early in sepsis, whereas heparanase-2 is a protective mediator. Recent studies have shown that shedding of the endothelial glycocalyx, as well as the ratio of heparanase-1 to heparanase-2, is diminished in septic patients who receive early TPE within the first 6 h of presentation. Therefore, it has been proposed that direct endothelial glycocalyx assessment and surrogate assays for shredded endothelial glycocalyx, as well as assays for heparanase-1 and heparanase-2 levels, enable early detection of SHINE to guide earlier antibiotics and targeted endothelial therapy with TPE (Drost et al., 2021; Pape et al., 2021; Stahl et al., 2021). VHAs assist the early detection of coagulopathies associated with SHINE when these patients are treated with plasma whether for trauma or sepsis (Thölking et al., 2015; Moore et al., 2018; Sperry et al., 2018; Pusateri et al., 2020). Recent trauma models adopting VHAs show promise for earlier identification of hemostatic derangement and SHINE, which predicts the need for MT in trauma patients (Thorn and Maegele, 2021). Likewise, early use of VHAs in SIC enables early detection of the depth and duration of shock prior to coagulopathic manifestation by conventional coagulation tests (CCTs) and other standard clinical and biologic markers (Pavoni et al., 2020). Combined with CCTs, VHAs, and clinical and laboratory markers such as lactate and procalcitonin, a PBM approach to assess the depth and duration of shock is becoming a reality whether in TIC or SIC (Vorweg et al., 2013; Müller et al., 2014; Schöchl and Schlimp, 2014; Saraiva et al., 2016; Johansson et al., 2017a; Martínez et al., 2018; Stettler et al., 2019; Walsh et al., 2020b; Scarlatescu et al., 2020; Carge et al., 2021; Drost et al., 2021; Pape et al., 2021; Tuan et al., 2021; Bunch et al., 2022a).
The direction of coagulation is driven not only by the coagulofibrinolytic mediators, but the endothelium is also influenced by the nature and severity of the initial injury, timing and methods of resuscitation, genetic hematologic makeup of the patient, underlying conditions (e.g., age, gender, atherosclerotic risk factors) and medications, all of which determine whether the patient’s endothelium and blood will respond with either a pro- or anti-thrombotic phenotype (Mellion et al., 1981; Kaplanski et al., 1998; Levi and van der Poll, 2008; Park et al., 2014; Dobson et al., 2015; Moore et al., 2016b; Strandin et al., 2016; Moore et al., 2019b; Wernly et al., 2019; Richter et al., 2022a). For example, in hemorrhagic shock, crystalloid resuscitation has demonstrated deleterious effects on the endothelium whereas plasma resuscitation has shown to restore it (Moore et al., 2018; Sperry et al., 2018; Brill et al., 2021). VHAs such as TEG and ROTEM provide a macroscopic, actionable indication of blood hemostatic integrity and severity of endothelial injury (Saini et al., 2019; Kim et al., 2021). Real-time VHA monitoring of coagulopathies associated with SHINE requires an understanding of the history, rationale, and efficacy of these tests.
2.1 VHAs as precision-based medicine tools to treat the spectrum of coagulopathic phenotypes associated with SHINE
Differing shock etiologies cause different early responses by the endothelium. These responses often share hemostatic phenotypes but also demonstrate unique aspects which implicate the need for personalized resuscitation of the endothelium. For example, the hypotensive septic shock patient often manifests acute hypofibrinolysis, or so-called “fibrinolytic shutdown”, and thus does not benefit from administration of the anti-fibrinolytic tranexamic acid (TXA). On the contrary, a patient in severe traumatic hemorrhagic shock with a hyperfibrinolytic phenotype may benefit from TXA (Moore et al., 2020). The hypotensive hypercoagulopathic patient in septic shock and the hypotensive hypocoagulopathic patient in hemorrhage-induced shock represent two opposite extremes along the coagulofibrinolytic spectrum of shock-associated coagulopathies.
SHINE can alter hemostasis on a minute-to-minute basis by endothelial reactions (e.g., shedding of the glycocalyx layer, or “phenotype switching” among pro- or anti-thrombotic and pro- or anti-fibrinolytic), causing worsening microvascular injury and organ malperfusion. Serial bedside VHAs offer more timely goal-directed blood component therapy (BCT) and hemostatic adjunct therapy (HAT) when these coagulopathies may switch phenotypes rapidly. Therefore, assessment of endothelial function and injury pertaining to hemostasis cannot solely rely upon the plasma-based CCTs prothrombin time (PT), activated partial thromboplastin time (aPTT), as well as platelet count, fibrinogen, and D-dimer. Rather, point-of-care global hemostasis assessment with whole blood VHAs, bedside assessment of organ perfusion, and other laboratory markers for hypoperfusion such as serial arterial base deficit and lactate, enable timely physiologic and targeted hemostatic resuscitation of patients in shock (Vorweg et al., 2013; Schöchl et al., 2014; Johansson et al., 2017a; Martínez et al., 2018; Walsh et al., 2020b; Bunch et al., 2022a).
Much as SHINE is a recently proposed framework for classifying patients with shock-associated coagulopathies, a simultaneous expansion has occurred for the use of VHAs to better define the phenotype of these coagulopathies and offer goal-directed therapy (Vorweg et al., 2013; Johansson et al., 2017a; Bugaev et al., 2020; Walsh et al., 2020b). VHAs required decades of guiding resuscitation in liver transplantation, cardiac surgery, and trauma before randomized controlled trials (RCTs) demonstrated the advantage of VHAs over CCTs alone for patients with hemorrhagic shock in these settings (Curry et al., 2018; Bugaev et al., 2020; Walsh et al., 2020b). To date, there remains no robust RCTs demonstrating superiority of VHAs to guide hemostasis management of patients in shock while on extracorporeal membrane oxygenation (ECMO) or with Left Ventricular Assist Devices (LVADs). However, VHAs are overwhelmingly used in these clinical settings (Colman et al., 2019; Bunch et al., 2021; Volod et al., 2022; Volod and Wegner, 2022). The clinician should not be dissuaded from using VHAs because of the absence of large RCTs demonstrating VHA utility to treat the hemostatic derangements caused by SHINE for etiologies other than liver transplantation, cardiac surgery, and trauma. In many other settings, VHAs have demonstrated utility by numerous observational and prospective studies (Adamzik et al., 2011; Brenner et al., 2012; Collins et al., 2014; Tran et al., 2015; Hans and Besser, 2016). The “one-size-fits-all” approach of large RCTs may hinder detection of the “signal from the noise” for the benefits of VHA-guided resuscitation for shock, especially in the care of complex patients because of infrequently met inclusion criteria. On the other hand, PBM allows for personalized treatment based on the patient’s individual phenotype. TEG/ROTEM enable both real-time identification of dynamic hemostatic phenotypes (phenotype switching) and provision of real-time guidance for the treatment of coagulopathies (individualized goal-directed resuscitation). (Maslove et al., 2017; Görlinger et al., 2019; McKinley et al., 2019; Stettler et al., 2019; Walsh et al., 2020b). Hence, VHAs may aid diagnosis and guide treatment for patients with all forms of SHINE. The adherence to the “one-size-fits-all” mandate by which large RCTs must establish clear statistical evidence prior to using a diagnostic test is challenged by the long history and evolution of VHAs as PBM tools in liver transplantation, cardiac surgery, trauma, and most recently, postpartum hemorrhage (PPH), ECMO, and LVAD resuscitation (Collins and Varmus, 2015; Beckmann and Lew, 2016; Letson and Dobson, 2017; Tignanelli and Napolitano, 2018; Stettler et al., 2019; Walsh et al., 2019; Bell et al., 2022).
The remaining review describes the principles of VHAs and applying these principles to the monitoring and treatment of patients in shock afflicted by SHINE. Hemostatic phenotypes associated with SHINE are first delineated by applying the accepted pathophysiologic drivers of endotheliopathy for trauma-induced coagulopathy (TIC) and sepsis-induced coagulopathy (SIC). These prevalent causes of coagulopathy in critical illness set the foundation by which to contextualize the coagulofibrinolytic spectrum of SHINE. In turn, one may rationalize the application of VHAs for the diagnosis and treatment of all shock-associated coagulopathies. Here, we additionally emphasize VHAs for SHINE in post-cardiac arrest syndrome (PCAS), medical causes of hemorrhage, PPH, burns, and venom-induced consumption coagulopathy (VICC) (Vincent and De Backer, 2013; Standl et al., 2018). For many causes of SHINE, whether medical or surgical, the use of VHAs is in its relative infancy. The use of VHAs for these settings may be compared to the early days of liver transplantation which required years of study before large RCTs demonstrated overwhelming benefit (Kang et al., 1985; Starzl, 2002; Walsh et al., 2020b). This physiologic primer likewise serves as the cornerstone for future expansion of trials to determine the benefit of VHAs for management of patients with shock-associated coagulopathies regardless of the etiology. Briefly, we next discuss VHA parameters, interpretation, and goal-directed blood components and hemostatic adjuncts prior to discussing specific etiologies of SHINE shown in Figure 5.
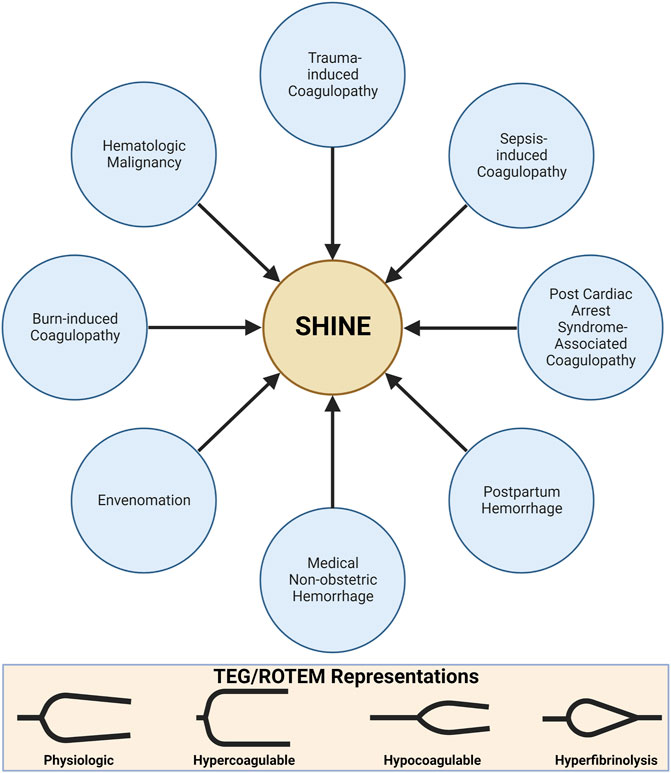
FIGURE 5. SHock-INduced Endotheliopathy (SHINE) as a Unifying Mechanism for Coagulopathies Associated with Critical Illness. In this review, we contextualize SHINE as defined by the viscoelastic hemostatic assays thromboelastography (TEG) and rotational thromboelastometry (ROTEM) within many causes of shock. Created with BioRender.com.
3 Basic principles of VHAs
3.1 VHA tracing and parameters
The mechanics of the cup-and-pin legacy devices (TEG 5000 and ROTEM delta), as well as the Sonic Estimation of Elasticity via Resonance (SEER) technology with SonoClot, and the new generation cartridge-based devices (i.e., ClotPro, Quantra, ROTEM Sigma, and TEG 6s) output tracings that plot the amplitude of clot strength in millimeters on the y-axis versus time in minutes on the x-axis. These tracings evaluate whole blood hemostatic competence by describing clot initiation, amplification, propagation, and termination by fibrinolysis (Curry et al., 2018; Volod et al., 2022). The maximum amplitude (MA) on TEG and maximum clot firmness (MCF) on ROTEM represent the surrogate endpoint of thrombogenesis. MA/MCF correspond to the maximal platelet-fibrin clot contraction strength; declining amplitude following the MA/MCF denotes fibrinolysis. TEG and ROTEM use differing reagents and parameter terminology. However, recognizing the similar pattern between the output tracing of the two tests allows for a broad comparison between the two devices. The differing terminology of the TEG/ROTEM, as well as other VHAs, has been viewed as a barrier to widespread clinical adoption. Figure 6 exemplifies the typical normocoagulable TEG/ROTEM tracing with the respective parameters defined. (Kang et al., 1985; Shore-Lesserson et al., 1999; Luddington, 2005; Spalding et al., 2007; Trzebicki et al., 2010; Sankarankutty et al., 2012; Whiting and DiNardo, 2014; Hunt et al., 2015; Gurbel et al., 2016; Veigas et al., 2016; Curry et al., 2018; Snegovskikh et al., 2018; Field et al., 2019; Gillissen et al., 2019; Schenk et al., 2019; Hartmann et al., 2020; Volod et al., 2022).
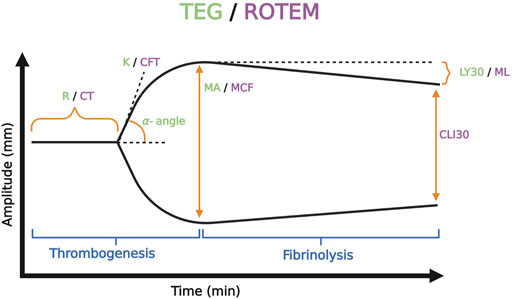
FIGURE 6. Representative Normocoagulable Thromboelastography (TEG) and Rotational Thromboelastometry (ROTEM) Tracing with Their Respective Parameters Defined. TEG and ROTEM parameters are represented by green and purple text, respectively. The time for the clot to reach 2 mm amplitude on the y-axis describes the reaction time (R) for TEG and clotting time (CT) for ROTEM. R and CT correlate to the activated partial thromboplastin time (aPTT) and prothrombin time (PT). The time spanned from 2 to 20 mm amplitude is called the kinetics (K) for TEG and the clot formation time (CFT) for ROTEM; these represent the speed of fibrin buildup. Likewise, alpha-angle measures the rate of fibrin buildup. The maximum amplitude (MA) on TEG and the maximum clot firmness (MCF) on ROTEM reflect crosslinking of fibrin with platelets and correspond to maximum clot retraction strength. Measurements of fibrinolysis include lysis at 30/60 min (LY30/60) which is the percentage decrease from MA achieved at 30/60 min, clot lysis index at 30/60 min (CLI30/60) which is the percentage of clot amplitude remaining relative to the MCF at 30/60 min, and maximum lysis (ML) which is the percentage decrease in MCF at a given length of time (Görlinger et al., 2021; Hartmann and Sikorski, 2021; Volod et al., 2022). Created with BioRender.com.
3.2 The shovel analogy to simplify VHA interpretation
A useful analogy to simplify TEG/ROTEM interpretation embodies the tracing as the shape of a shovel (Figure 7). With hemostatic competence or physiologic hemostasis, the shovel has an ideal handle length (R/CT), blade slope (K/CFT and α-angle), blade width (MA/MCF), and blade tip (LY30/CLI30/ML), shown as the middle shovel in Figure 6. The extremes are represented by different shovel shapes where, for the sake of analogy, the ease of tilling and moving soil corresponds to the ease of moving blood. The hypocoagulable shovel tracing has a long handle with a narrow and pointed blade (top shovel in Figure 6), with which tilling and soil transport becomes less cumbersome but markedly inefficient. The hypercoagulable shovel tracing has a short handle and a wide blade with an absent tapering of the tip (bottom shovel in Figure 6), making it difficult for the earth to be broken up and transported. In the above examples, tilling and soil transport are either difficult (hypercoagulable) or easy but inefficient (hypocoagulable). In summary, in Figure 6, the top shovel represents hypocoagulable disequilibrium, the middle shovel represents physiologic hemostasis, and the bottom shovel represents hypercoagulable disequilibrium. Goal-directed BCT and HAT may be administered based on either the shovel analogy pattern or the numerical values of the TEG/ROTEM parameters (Table 2).
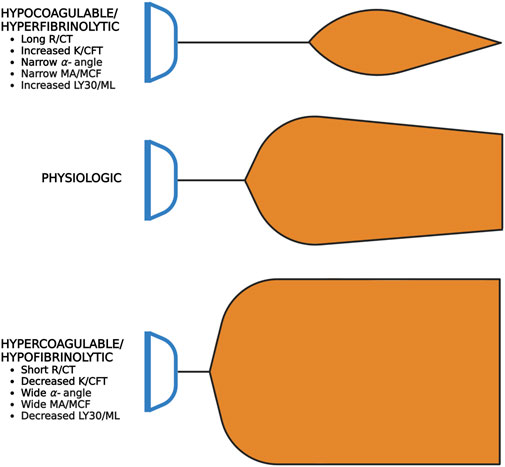
FIGURE 7. Shovel Analogy to Rapidly Interpret TEG/ROTEM Tracings. The top shovel represents the hypocoagulable state marked by a prolonged R/CT, narrow α-angle, narrow MA/MCF, and increased lysis with resultant increased LY30/ML. The middle shovel represents physiologic hemostasis marked by normal R/CT, α-angle, MA/MCF, and LY30/ML. Mild narrowing after the MA demonstrates physiologic fibrinolysis. The bottom shovel represents the hypercoagulable state denoted by decreased R/CT, wide α-angle, wide MA/MCF, and decreased LY30/ML. Abbreviations: R, Reaction time; CT, Clotting Time; K, Kinetics; CFT, Clot Formation Time; MA, Maximum Amplitude; MCF, Maximum Clot Firmness; LY30/60, Lysis at 30/60 min; ML, Maximum Lysis. Created with BioRender.com.
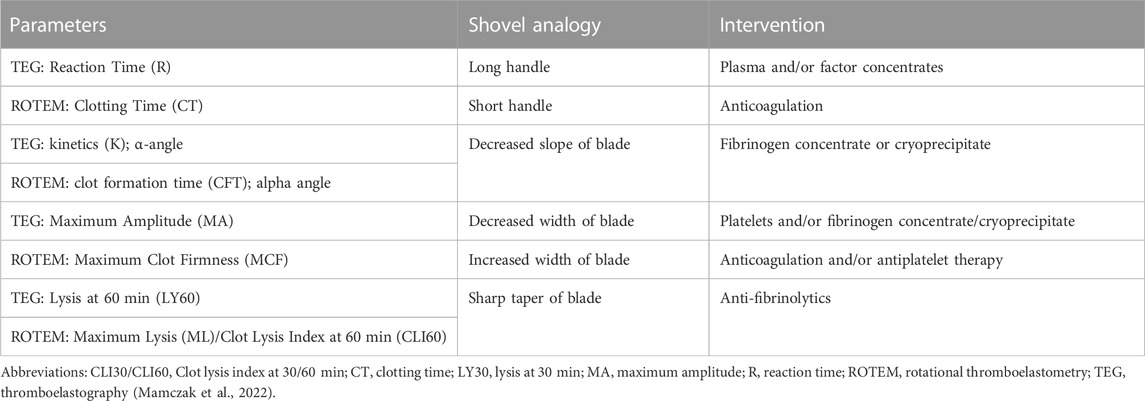
TABLE 2. Goal-directed blood components and hemostatic adjuncts based on the shovel analogy of TEG/ROTEM.
4 VHAs and etiologies of SHINE
4.1 Trauma-induced coagulopathy (TIC)
Uncontrolled hemorrhage accounts for about 25% of deaths after injury, and an estimated one-quarter of these deaths likely have a TIC element (Moore et al., 2021b). TIC is not a single entity, but rather comprises a spectrum of coagulopathic phenotypes that is largely biphasic. ‘Early TIC’ generally characterizes the first 6 h following injury wherein difficulty to achieve hemostasis may lead to hemostasis exhaustion, uncontrolled hemorrhage despite adequate mechanical control of bleeding sites (i.e., coagulopathy), and progressive hemorrhagic shock (Kashuk et al., 1982; Moore et al., 2021a). ‘Late TIC’ generally describes hypercoagulability 24 h or more following the time of injury. Clinically, late TIC manifests micro- and macro-thrombotic complications such as venous thromboemboli, ultimately leading to organ failure (Moore et al., 2021a). Early TIC severity increases proportionally with the magnitude of injury severity, blood loss, and shock. Late TIC correlates to the degree of tissue injury (Moore et al., 2021a).
Following major trauma, the release of tPA from endothelial cells may be involved in the initial activation of fibrinolysis in response to a burst of thrombin and fibrin generation and sympathetic outflow. This fibrinolytic phase ends within several hours by the production of PAI-1 by endothelial cells and platelets. This dynamic change is termed “fibrinolytic shutdown” (Moore et al., 2019b) and may rapidly occur in 40%–50% of patients despite arrival to the hospital within an hour after injury (Moore et al., 2016b). Hemorrhage may invoke physiologic fibrinolysis shutdown to achieve hemostasis at bleeding sites. However, trauma patients with persistent fibrinolytic shutdown at 24 h post-injury have increased mortality (Moore et al., 2019b). On the opposite end of the fibrinolytic spectrum, roughly one-quarter of trauma patients have evidence of prior fibrinolytic activation, but only 7% have active ongoing fibrinolysis at the time of initial blood draw (Moore et al., 2019a). Hyperfibrinolysis as measured by TEG/ROTEM correlates to increasing injury severity, magnitude of shock, catecholamines, and SHINE (Holcomb, 2011; Moore et al., 2016a). Administering TXA empirically to TIC patients without evidence of hyperfibrinolysis may cause early fibrinolysis resistance and increased mortality, necessitating a PBM approach to TXA use guided by VHAs (Moore et al., 2017).
Figure 8 depicts the coagulofibrinolytic balance of TIC as a teeter totter wherein the TM-thrombin complex is one such fulcrum to determine anti- or pro-hemostatic phenotypes. This complex, also mediated by the endothelial protein C receptor (EPCR), activates the aPC anticoagulation pathway resulting in Factor V and Factor VIII degradation and enhanced fibrinolysis via PAI-1 inhibition. On the contrary, the TM-thrombin complex may activate the TAFI pathway resulting in hypercoagulation by inhibiting fibrinolysis (Sillen and Declerck, 2021). Whether the action of the TM-thrombin complex favors increased coagulation via TAFI or a hypocoagulable state mediated by aPC on the activated endothelium depends on the severity of trauma, the presence or absence of shock, endotheliopathy, and the manner, timing, and response to resuscitation (Dobson et al., 2015; Moore et al., 2020). The balance of the two opposing pathways may tip directions within seconds to minutes following trauma and involves either structural and/or posttranslational modifications of different sites on the TM-thrombin complex. Subsequent action by receptors and/or cofactors causes the modified TM-thrombin complex to bind and activate protein C or TAFI (Dobson et al., 2015; Johansson et al., 2017a).
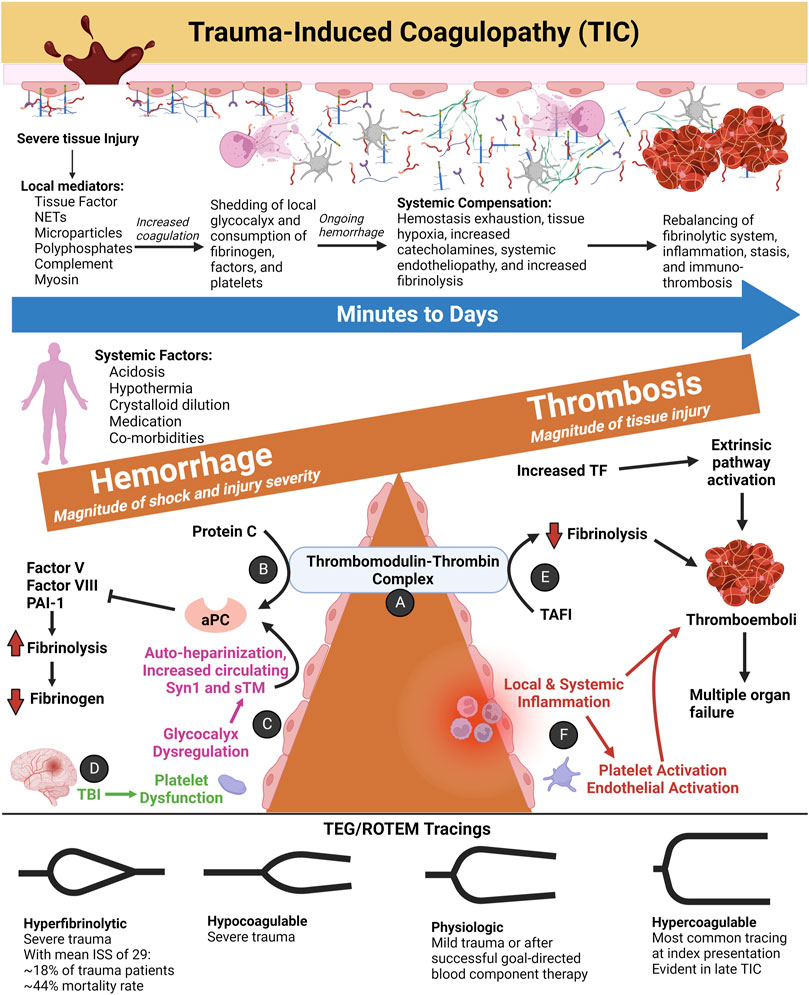
FIGURE 8. The Spectrum of Trauma-Induced Coagulopathy (TIC) as a Function of the Thrombomodulin-Thrombin Complex and SHINE. Hypercoagulability presents most commonly at index trauma presentation according to thromboelastography (TEG) and rotational thromboelastometry (ROTEM) tracings (Johansson and Ostrowski, 2010). As injury severity and the magnitude of hemorrhagic shock increase, the likelihood of hypocoagulability and/or hyperfibrinolysis increases in tandem (Johansson and Ostrowski, 2010; Moore et al., 2016a). Other anti-hemostatic factors at index may include acidosis, hypothermia, crystalloid resuscitation resulting in dilutional coagulopathy, pre-trauma anticoagulant or antiplatelet medications, and co-morbidities (Moore et al., 2021a). After successful initial resuscitation, patients most often demonstrate hypercoagulability and venous thromboembolism in the ensuing days. On the other hand, persistent fibrinolytic shutdown at 24 h post-injury correlates greatest to the magnitude of tissue injury. (A) The thrombomodulin (TM)-thrombin complex is one proposed hypothesis to explain TIC hemostatic phenotypes (Walsh et al., 2019). (B) In its anticoagulant role, the endothelial membrane-bound TM binds with thrombin to convert protein C to activated protein C (aPC). TM-thrombin action on protein C may also be accelerated by endothelial protein C receptor (EPCR, not shown). APC inactivates Factor V, Factor VIII, and plasminogen activator inhibitor-1 (PAI-1) to decrease coagulation and promote tissue plasminogen activator (tPA) activity to convert plasminogen to plasmin (Gando et al., 2018). The resulting fibrinolysis leads to hypofibrinogenemia and a hypocoagulable state as demonstrated by viscoelastic markers. APC and fibrinogen levels share an inverse relationship whereby the TM-thrombin complex increases protein C activation with decreasing fibrinogen levels, leading to a greater anticoagulant and pro-fibrinolytic state (Diez et al., 2006). On the contrary, with increased fibrinogen, the TM–thrombin complex is inhibited from activating protein C. As a result of glycocalyx dysfunction, activation of protein C, enhanced fibrinolysis, and low fibrinogen, the maladaptive response caused by consumption of clotting factors and platelets leads to high fibrin/fibrinogen degradation products (FDPs) with an overall anti-hemostatic state (Diez et al., 2006; Dobson et al., 2015). (C) Tissue hypoperfusion and endothelial injury causes shedding of the endogenous HS of the glycocalyx with subsequent “auto-heparinization” (Ostrowski and Johansson, 2012). The sensitivity of TEG/ROTEM to detect auto-heparinization remains questionable (Zipperle et al., 2022a). Disruption of the endothelial glycocalyx may also be measured by increased circulating syndecan-1 (Syn1) and soluble TM (sTM) levels (Johansson et al., 2011b). (D) Traumatic brain injury produces a unique coagulopathy characterized by platelet dysfunction at the arachidonic acid (AA) and adenosine diphosphate (ADP) receptors as defined by TEG with Platelet Mapping. The relatively high concentrations of von Willebrand Factor (vWF) and Tissue Factor (TF) release from injured brain tissue are thought to cause platelet exhaustion (Castellino et al., 2014; Bradbury et al., 2021). However, the pathophysiology of coagulopathy of traumatic brain injury remains an area of active study. (E) The TM-thrombin complex also activates thrombin-activatable fibrinolysis inhibitor (TAFI) which acts to inhibit tPA binding to fibrin (Marar and Boffa, 2016). (F) Minutes to days after traumatic/surgical-related injury, local and/or systemic inflammation occurs, causing immuno-thrombosis via platelet and endothelial activation. Particularly in the microvasculature, thromboemboli impair organ perfusion and contribute to organ failure (Gando and Otomo, 2015). Abbreviations: aPC, activated Protein C; ISS, Injury Severity Score; NETs, Neutrophil Extracellular Traps; PAI-1, Plasminogen Activator Inhibitor-1; ROTEM, Rotational Thromboelastometry; TAFI, Thrombin-Activatable Fibrinolysis Inhibitor; TEG, Thromboelastography; TF, Tissue Factor; TIC, Trauma-induced Coagulopathy; TBI, Traumatic Brain Injury. Created with BioRender.com.
With vascular injury, a thrombin burst mediates fibrin formation as well as a protection of the fibrin clot from dissolution via activation of TAFI (Lord, 2011; Foley et al., 2013). tPA or uPA cleavage of plasminogen to plasmin, the major fibrinolytic enzyme, then dissolves the fibrin meshwork into soluble fibrin/fibrinogen degradation products (FDPs) which mediate a positive feedback mechanism resulting in fibrinolysis (Silva et al., 2012). PAI-1 primarily prevents hyperfibrinolysis by inhibition of tPA as well as urokinase plasminogen activator (uPA) (Declerck and Gils, 2013; Sillen and Declerck, 2020). In addition, plasmin is inhibited by α2-antiplasmin (Singh et al., 2020). Importantly, activated TAFI (TAFIa) is a zinc-dependent metallocarboxypeptidase which downregulates fibrinolysis by removing C-terminal lysine residues from partially degraded fibrin; thereby preventing the upregulation of plasminogen binding and activation (Declerck, 2011; Vercauteren et al., 2013). Activation of TAFI following the thrombin burst regulates hemostasis with a fibrinolytic shutdown response and has been described as a crucial regulatory link between coagulation and fibrinolysis (Leurs and Hendriks, 2005; Claesen et al., 2021).
Recent studies have demonstrated mortality benefit and cost savings associated with early plasma resuscitation for patients with TIC (Moore et al., 2018; Brill et al., 2021). Early administration of plasma may serve therapeutic and sparing effects on the endothelial glycocalyx layer as demonstrated by decreased Syn-1 levels following plasma administration (Cannon, 2018; Sperry et al., 2018; Gruen et al., 2020; Pusateri et al., 2020; Hrebinko et al., 2021). This reduction of Syn-1 shedding may occur via reduced Tissue Inhibitor of MetalloProteinase (TIMP) activity or decreased activation of A Disintegrin And Metalloproteinase (ADAM). VHAs have been recommended as a method to gauge the adequacy of targeted resuscitation with plasma (Moore et al., 2021a).
Emphasis on resuscitation of the endothelium has led to the use of vasopressin for patients in severe shock associated with trauma (Simmons and Powell, 2016). When used in conjunction with clinical, laboratory, biologic, and standard coagulation tests, adjunctive VHAs reflect the hemostatic milieu of the endothelium and its contribution to hemostatic derangement in patients with SHINE. The combination of these tests provides a holistic view of whole blood hemostatic integrity, enabling goal-directed plasma and/or pressor therapy for patients in hemorrhagic shock (Moore et al., 2021a; Richards et al., 2021).
SHINE and mortality in TIC correlate to blood product administration (Dunne et al., 2004). It has been suggested that the pro-inflammatory extracellular vesicles (EVs) in stored blood products, particularly packed red cells, may cause or contribute to endotheliopathy (Straat et al., 2016). However, a recent observational study of 75 trauma patients demonstrated that red blood cell EVs increased following transfusion yet did not increase Syn1 levels (Dujardin et al., 2022).
Trauma can also be classified as primary or secondary based on pathophysiology (Brohi et al., 2003; Simmons and Powell, 2016), early or late based on timing (Tisherman et al., 2015), hypo- or hyperfibrinolytic based on hemostatic phenotype, and resuscitated or not resuscitated (Gando et al., 1992; Gando et al., 1995; Adrie et al., 2004; Gando et al., 2011; Tauber et al., 2011; Wohlauer et al., 2012; Gando et al., 2013; Moore et al., 2015b; Vogel et al., 2015; Gando and Hayakawa, 2016; Davenport et al., 2017; Leeper et al., 2017; Macko et al., 2017; Meizoso et al., 2017). Without treatment, these patients may progress to a DIC-like syndrome of hyperfibrinolysis in minutes to hours. Therefore, point-of-care testing with VHAs enables hemostatic monitoring to guide diagnosis and individualized ratios of BCT and HATs (Moore et al., 2019b; Moore et al., 2020). It should also be noted that surgical-related coagulopathies, such as damage control surgery or those incurred during liver transplantation and cardiac surgeries which have a long and rooted history of VHA-guided BCT and HAT, may be viewed similarly to TIC. Surgical-related coagulopathies and TIC share traumatic hemorrhagic shock pathophysiology and likewise necessitate goal-directed resuscitation.
4.2 Sepsis-induced coagulopathy (SIC)
Whereas hemorrhagic shock with TIC potentiates uncontrollable bleeding in its early phase, the early hemostatic phenotype of SIC involves a hypercoagulable state of hypofibrinolysis with consequent micro-thrombosis and sequential organ failure (Semeraro et al., 2012). Trauma and surgery patients who survive the early phase of hemorrhage may develop a late hemostatic phenotype that manifests as thrombosis and multiple organ failure like SIC. However, the stimulus of coagulation for each pathologic entity differs. In TIC, tissue factor (TF) release from injured tissue induces coagulation. Micro-thrombus formation, a fundamental event of SIC, is observed in late TIC and is under continued investigation (Vogel et al., 2015; Moore et al., 2020). In SIC, the two main drivers at the level of the endothelium are immuno-thrombosis and suppressed fibrinolysis. The influence of these systems and the mechanisms of their effect on the endothelium are depicted in Figure 9.
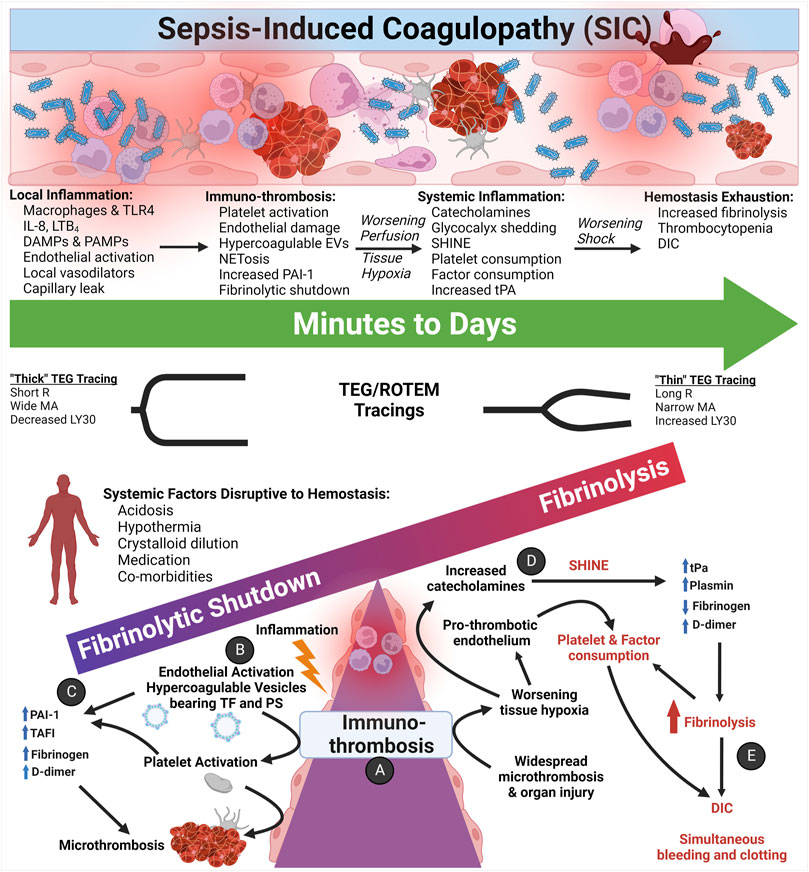
FIGURE 9. The Coagulofibrinolytic Spectrum of Sepsis-induced Coagulopathy (SIC) Pertaining to Immuno-thrombosis and SHINE. (A) Initially, the immuno-thrombosis manifests as microthrombosis within the microvasculature. (B) Inflammation activates the endothelium and, among other mechanisms, activates primary and secondary hemostasis via the endothelial release of hypercoagulable circulating extracellular vesicles (EVs) bearing Tissue Factor (TF) and phosphatidylserine (PS). (C) Most patients with SIC present with hypercoagulopathic, hypofibrinolytic thromboelastography (TEG)/rotational thromboelastometry (ROTEM) tracings with elevated acute phase reactants such as fibrinogen, D-dimer, and plasminogen activator inhibitor-1 (PAI-1). Quiescent platelets contain PAI-1, TAFI, FXIIIa, and α2-antiplasmin in α-granules, and upon activation, platelets release PAI-1 to complex with and inhibit action of tPA. Thrombin may also provoke release of PAI-1 from the endothelium (Huebner et al., 2018). (D) As hypoperfusion and the shock state progresses, increased catecholamines activate and damage the pro-thrombotic endothelium, causing systemic endothelial release of Weibel-Palade bodies containing tPA. Hypoperfusion also increases endothelial calcium influx, resulting in PS exposure on the endothelial luminal surface. (E) Increased circulating tPA tips the scales in favor of fibrinolysis as a counterbalance to the widespread microthrombosis. Thus, a small percentage of septic patients may present and/or progress to a hyperfibrinolytic and consumptive hypocoagulopathic state of disseminated intravascular coagulation (DIC), which requires aggressive resuscitation with primarily blood components as opposed to crystalloid fluids for the hypercoagulopathic SIC patients (Levi and van der Poll, 2017; Iba and Ogura, 2018; Iba et al., 2019b; Bunch et al., 2022b). Abbreviations: DAMPs, Damage-Associated Molecular Patterns; DIC, Disseminated Intravascular Coagulation; EVs, Extracellular Vesicles; IL-8, Interleukin-8; LTB4, Leukotriene B4; LY30, Lysis at 30 min; MA, Maximum Amplitude; PAI-1, Plasminogen Activator Inhibitor-1; PAMPs, Pathogen-Associated Molecular Patterns; PS, PhosphatidylSerine; R, Reaction time; SHINE, SHock-INduced-Endotheliopathy; TAFI, Thrombin-Activatable Fibrinolysis Inhibitor; TF, Tissue Factor; TLR, Toll-Like Receptors; tPA, tissue Plasminogen Activator. Created with BioRender.com.
The mechanisms that initiate SIC have been previously described as both cell-based and humoral-based (Liaw et al., 2016; Iba and Levy, 2018). At the interface of mounting an immune response, the endothelium activates to a pro-thrombotic state in response to numerous inflammatory mediators. Biomarkers that crosstalk between inflammation and endothelial activation include leukotrienes, IL-1, IL-6, IL-8, TNF-α, reactive oxygen species, hydrogen peroxide, complement, histamine, serotonin, and shiga toxin, as well as hypoxia, thrombin, fibrin, and epinephrine (McCormack et al., 2017). The host response to sepsis involves the activation of coagulation by TF on EVs and activated endothelium (Østerud and Bjørklid, 2001). PS expressed on EVs and activated endothelium also activates the extrinsic coagulation cascade (Iba and Ogura, 2018). Among the most salient factors which are involved in the immuno-thrombotic response to sepsis are pathogen-associated molecular patterns (PAMPs), damage-associated molecular patterns (DAMPs), high mobility group box 1 (HMGB 1), DNA, histones, neutrophil extracellular traps (NETs), damaged host cells, and activated immune cells, all of which initiate pro-inflammatory and pro-thrombotic reactions in SIC (Østerud and Bjørklid, 2001; Brinkmann et al., 2004; Adrie et al., 2005; Liaw et al., 2016; Iba and Ogura, 2018; Vulliamy et al., 2019).
FDPs and D-dimer levels have limited use for diagnosing and treating shock in either SIC of TIC. Because of their long half-life these markers do not correlate with PAI-I levels in patients with SIC or TIC. PAI-I levels are not readily available in clinical practice and therefore VHAs have been used to detect fibrinolysis in trauma patients. (Moore et al., 2014). Despite widespread use of VHA to detect fibrinolysis in trauma there is significant debate regarding its sensitivity (Larsen et al., 2012; Hunt et al., 2013; Ramos et al., 2013; Raza et al., 2013; Stettler et al., 2019).
AT is an important anticoagulant that prevents the formation of thrombi (Levy et al., 2016). In addition, prostacyclin, nitric oxide, and TFPI mediate anti-thrombotic effects at the level of the endothelium (Iba and Levy, 2019).
In SIC there is a significant suppression of anti-thrombotic activity which is affected by the methods of treatment as well as the speed of resuscitation. CCTs as well as FDPs and D-dimers do not adequately assay the importance of anti-thrombotic activity in SIC and TIC (Owings et al., 1996; Iba et al., 2012). It is instructive to compare early TIC and late TIC with SIC, whereby the increased release of TM activates protein C whereas late in TIC AT and protein C are depressed (Hess et al., 2008; Zilkens et al., 2008; Yanagida et al., 2013; Choi et al., 2014; Johansson et al., 2017a; Kornblith et al., 2019; Keshari et al., 2020). In sepsis AT levels decline and recent studies have demonstrated the possible utility of AT therapy in septic DIC (Vincent et al., 2019; Egi et al., 2021).
The similarities between SIC and TIC are instructive and are summarized in Table 3. Whereas the hemostatic derangement characteristic of hyperfibrinolytic phenotype associated with early severe TIC in shock is transformed in late TIC into a hypofibrinolytic phenotype characteristic of SIC (Taylor Jr et al., 2001; Gando and Wada, 2019; Vulliamy et al., 2019; Moore, 2022).
4.3 Post-cardiac arrest syndrome (PCAS)-associated coagulopathy
After TIC and SIC, the third most common cause of shock is cardiogenic of which PCAS is a major subtype. Ischemia-reperfusion injury drives the pathophysiology of PCAS-associated coagulopathy. The ensuing tissue necrosis, pro-thrombotic DAMPs, systemic inflammatory response, sympatho-adrenal activation, and SHINE account for the commonly observed hypercoagulability in these patients. The hypercoagulability arises from increased circulating TF, DAMPs, immuno-thrombosis, and an activated pro-thrombotic endothelium. Acutely, systemic hyperfibrinolysis occurs in PCAS because of tPA release from endothelial Weibel-Palade bodies. Hyperfibrinolysis occurs in about one-third to one-half of patients with PCAS, confirming the high incidence of fibrinolysis in patients with the “no-reflow phenomenon” of PCAS (Ames et al., 1968; Schöchl et al., 2013; Kloner et al., 2018). Of note, a recent observational study of 41 patients with cardiac arrest has supported conventional activation of plasminogen—as opposed to pro-inflammatory pathways of fibrinolysis activation—as the cause of hyperfibrinolysis in PCAS-associated coagulopathy (Zipperle et al., 2022b). This study also supported that hyperfibrinolysis in PCAS shares pathophysiologic similarities to TIC wherein hypoperfusion and increased aPC appear to be the incipient drivers. Moreover, it has been observed that cardiac arrest due to hypoxia has a higher incidence of hyperfibrinolysis compared to cardiac arrest from a primarily cardiogenic cause (Wada et al., 2017). Figure 10 depicts the coagulofibrinolytic equilibrium of PCAS-associated coagulopathies wherein ischemia-reperfusion injury determines the balance between hyperfibrinolytic and fibrinolysis shutdown phenotypes.
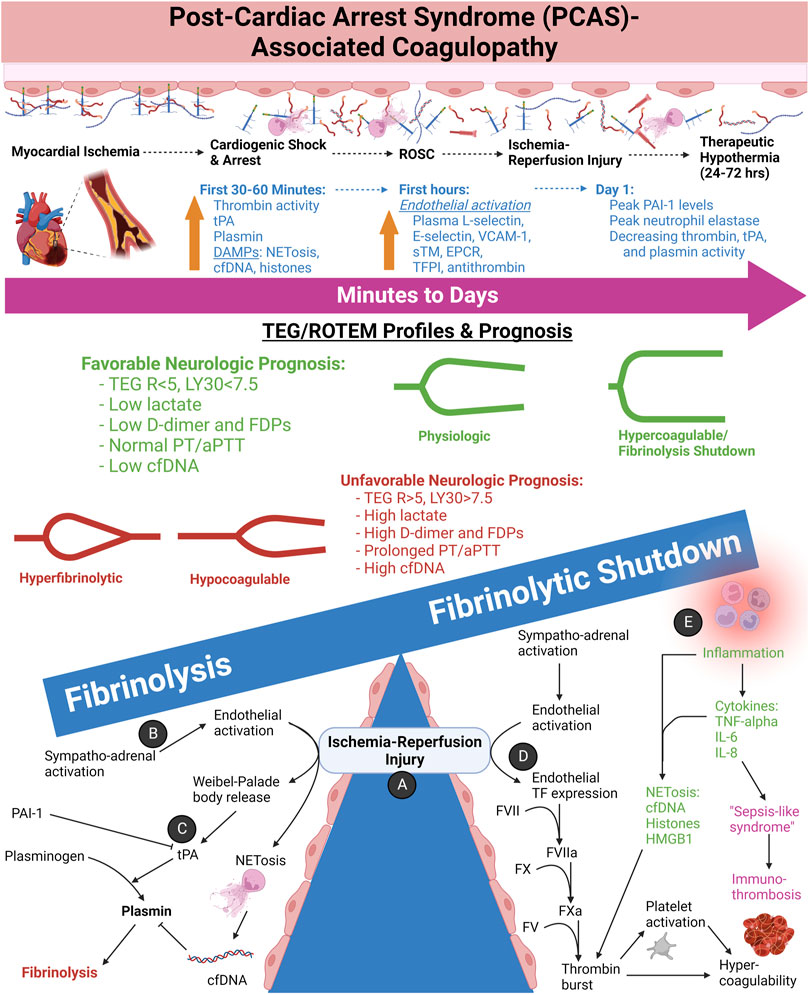
FIGURE 10. The Spectrum of Post-Cardiac Arrest Syndrome (PCAS)-associated Coagulopathies and Neurologic Prognostication by TEG/ROTEM. (A) In cardiac arrest, ischemia afflicts every tissue in the body. Depending on the length of arrest, necrosis results for many tissue types, resulting in an acute inflammatory response. Return of spontaneous circulation (ROSC) further promotes inflammation by reperfusion of oxygen, thereby increasing the generation of reactive oxygen species by the now resident inflammatory cells. (B) As a result of the shock state and epinephrine infusion during resuscitation, the activated endothelium becomes pro-thrombotic and simultaneously fibrinolytic via Weibel-Palade body (WPB) exocytosis as one such mechanism. (C) Widespread release of tissue plasminogen activator (tPA) by the endothelium promotes conversion of plasminogen to plasmin. Circulating cell free DNA (cfDNA), either from neutrophil extracellular traps (NETs) or necrotic cells, has demonstrated to inhibit plasmin activity to a degree. Circulating plasminogen activator inhibitor-1 (PAI-1) also serves to decrease fibrinolytic activity; however, as an acute phase reactant, PAI-1 levels have shown to peak at 24 h following ROSC. Platelet activation and release of α-granule contents PAI-1, TAFI, FXIIIa, and α2-antiplasmin likely also contribute. Hyperfibrinolysis and/or hypocoagulability prognosticate poor neurologic outcomes. These hemostatic phenotypes arise more commonly with longer times to achieve ROSC. TEG measurements of reaction time (R) > 5 min and lysis at 30 min (LY30) >7.5% following ROSC tend to have poor neurologic outcomes. In tandem, prolonged prothrombin time (PT) and activated partial thromboplastin time (aPTT) and increased markers of fibrinolysis (e.g., D-dimer and fibrin [ogen] degradation products) also prognosticate poor outcomes. Increased markers of tissue ischemia and necrosis such as lactate and cfDNA follow a similar worse prognosis. (D) Endothelial activation promotes thrombosis by increased Tissue Factor (TF) expression by both increased extracellular vesicles bearing TF, but also by necrotic cells releasing free TF systemically. (E) The ensuing inflammatory state in response to ischemia promotes immuno-thrombosis via several mechanisms, but namely via NETs catching and activation of circulating platelets as well as pro-thrombotic proteins from necrotic tissues such as cfDNA, histones, and High Mobility Group Box-1 (HMGB-1). The inflammatory state observed clinically in PCAS patients has been aptly termed “Sepsis-like syndrome” because of the systemic inflammatory response syndrome without an infectious source (Wada, 2017; Yu et al., 2020). Important to note, however, that hyperfibrinolysis in PCAS appears to be caused primarily by hypoperfusion rather than inflammation (Zipperle et al., 2022b). Abbreviations: aPTT, activated Partial Thromboplastin Time; cfDNA, cell free DNA; DAMPs, Damage-Associated Molecular Patterns; EPCR, endothelial Protein C Receptor; FDPs, Fibrin(ogen) degradation products; HMGB-1, High Mobility Group Box 1; IL, Interleukin; LY30, Lysis at 30 min; NETs, Neutrophil Extracellular Traps; PAI-1, Plasminogen Activator Inhibitor-1; PT, Prothrombin Time; R, Reaction time; sTM, soluble Thrombomodulin; TFPI, Tissue Factor Pathway Inhibitor; TNF-alpha, Tissue Necrosis Factor-alpha; tPA, tissue Plasminogen Activator; VCAM-1, Vascular Cellular Adhesion Molecule-1. Created with BioRender.com.
Compared to patients without the hyperfibrinolytic phenotype, patients with hyperfibrinolysis required longer CPR times, had elevated aPTT, D-dimer, and hypoperfusion markers including pH, base excess, and lactate. The lysis onset time (LOT) was directly proportional to survival and inversely related to CPR times and lactate. These data confirmed previous observations that the time to onset of clot lysis is an important marker for patient outcomes (Viersen et al., 2012). High lactate levels also predict development of PCAS-associated DIC with hyperfibrinolysis (Wada et al., 2016).
On the contrary, small increases in PAI-1 levels are measurable shortly after ROSC and may be owed to release by activated platelets or endothelium. PAI-1 levels have been shown to peak at 24 h after achieving ROSC, and increased levels correlate to multiple organ dysfunction and worse outcomes (Geppert et al., 2001; Wada, 2017). The initial hypercoagulopathic phase in patients with PCAS reflect similarly to severe TIC with early phase hyperfibrinolysis mediated by tPA and subsequent fibrinolytic shutdown mediated by PAI-1. The unique pathophysiologic moment begins with the “no-reflow phenomenon” which describes reduced antegrade coronary and/or cerebral microcirculatory blood flow despite proximal patency which is commonly seen following cardiac arrest and ROSC (Ames et al., 1968; Kloner et al., 2018). The rapid change from hyperfibrinolysis to hypofibrinolysis occurs with successful and early ROSC. Unlike TIC, these patients do not benefit from anti-fibrinolytic administration which substantiates the lack of similar causality for the coagulopathies associated with PCAS (Ames et al., 1968; Wada, 2017; Yu et al., 2020).
Studies of patients with PCAS who have attained ROSC have demonstrated the utility of TEG and ROTEM to predict intact neurologic survival as a reflection of reduced fibrinolysis. It has been shown that TEG values of R < 5 min or LY30 < 7.5% in early PCAS had more favorable neurologic outcomes. Higher D-dimer levels, PT, aPTT, lactate, and cfDNA were noted in the unfavorable outcome group. Therefore, in the earliest periods following ROSC, a normal hemostatic and fibrinolytic phenotype are early predictors of neurologically intact survival in successfully resuscitated out-of-hospital cardiac arrest patients (Yu et al., 2020). Early ROTEM analysis has likewise revealed a high incidence of hyperfibrinolysis for those patients who had long cardiac arrest times and poor prognosis. Specifically, hyperfibrinolysis criteria have been recorded in 83% of patients with long cardiac arrest times, and these patients also had lower fibrinogen levels with corresponding low levels of FIBTEM MCF (Barea-Mendoza et al., 2019).
The use of mild therapeutic hypothermia (MTH) has demonstrated increased survival. TEG may be a useful technique to evaluate hemostatic integrity in cardiac arrest survivors undergoing MTH. However, the effects of MTH on PCAS-associated coagulopathy requires appreciation for the effect of temperature on fibrin (ogen) concentration and function. When compared to physiologic temperature patients who have survived cardiac arrest, MTH has shown to lengthen TEG R, reduce the coagulation index (CI), and attenuate clot fibrinolysis. Rather than performing the VHAs at 37°C, it is therefore suggested that VHA analysis be performed at 32°C during MTH to increase the accuracy of hypothermic coagulation impairment (Trąbka-Zawicki et al., 2019). Moreover, prolonged MTH has shown to impair thrombin generation as measured by increased CT and prolonged time to maximum velocity of thrombin generation on INTEM (Jeppesen et al., 2017).
4.4 Medical non-obstetrical hemorrhage
Common non-obstetrical causes of shock include gastrointestinal (GI) hemorrhage and, to a lesser extent, retroperitoneal hemorrhage of anticoagulated or hypocoagulopathic patients. The state of the endothelium in many ways reflects TIC where the degree of hemorrhage dictates the hemostatic derangement at the endothelium. However, as in TIC, pre-existing hemostatic phenotypes (e.g., liver failure and antiplatelet or anticoagulant medications) in part determine the evolution and response to therapy which requires VHAs to guide BCT and HAT for these patients (Bunch et al., 2022a). Patients treated with anticoagulants and antiplatelet agents often require replenishment of factors and/or platelets in TIC and medical hemorrhage causing shock (Kang et al., 1985; Shore-Lesserson et al., 1999; Enriquez and Shore-Lesserson, 2009; Ojito et al., 2012; Tanaka et al., 2012; Levin et al., 2014; Tanaka et al., 2014; Weitz and Eikelboom, 2016; Bliden et al., 2017; Douketis et al., 2017; Dubois et al., 2017; Gurbel et al., 2017; Mullins et al., 2018; Artang et al., 2019; Bruckbauer et al., 2019; Dias et al., 2019; Pailleret et al., 2019; Sarode, 2019; Oberladstätter et al., 2021; Pavoni et al., 2022). In patients with liver failure and GI hemorrhage, the rebalanced hemostasis caused by the reduced anticoagulants protein S, protein C, and AT require that VHAs be used in the diagnosis and resuscitation of these patients in shock. Serial hemostatic functional evaluation of a patient in liver failure with shock would not be possible with CCTs, but can be done successfully with VHAs (Groth et al., 1969; Chau et al., 1998; Starzl, 2002; Tripodi and Mannucci, 2011; Agarwal et al., 2012; Stravitz, 2012; Scarlatescu et al., 2018; Stravitz et al., 2021). Interestingly, the use of anti-fibrinolytics in patients with GI hemorrhage and shock has not shown to improve outcomes, further demonstrating the heterogeneity of coagulopathies associated with SHINE due to medical hemorrhage versus traumatic or surgery-related hemorrhage (Roberts et al., 2020).
4.5 Postartum hemorrhage (PPH)
VHA-guided BCT during PPH is expanding. TEG/ROTEM devices can be used to detect and treat clinically significant hypofibrinogenemia, although evidence to support the role of VHAs for guiding fresh frozen plasma and platelet transfusion is less clear (Collis and Collins, 2015; Bamber, 2016; Collis, 2016; Curry et al., 2018). If ROTEM/TEG tracings are normal, clinicians should investigate for another cause of bleeding, and BCT may be withheld. Guidelines support the use of VHAs during PPH if a local algorithm reaches agreement. However, wide consensus does advise that a FIBTEM amplitude at 5 min (A5) of <12 mm with ongoing bleeding necessitates fibrinogen replenishment (Curry et al., 2018; Collins, 2022). Note, however, that patients with PPH may also have reduced thrombin generation (Di Bartolomeo et al., 2017). Guidelines recommend against using VHAs to guide TXA infusion. Rather, TXA should be administered as soon as PPH is diagnosed irrespective of the TEG/ROTEM traces, however patients with PPH are also at high for venous thromboembolism suggesting this issue requires further investigation (Shakur et al., 2010; Roberts et al., 2012; Collins, 2022). The cost-effectiveness of VHAs during PPH needs to be addressed and has formed the foundation for much of the discussion regarding the utility of VHAs to guide BCTs and HATs in patients with severe PPH. (Collis and Collins, 2015; Bamber, 2016; Collis, 2016; Curry et al., 2018; Collins et al., 2019; Liew-Spilger et al., 2021; Collins, 2022).
Severe PPH, such as with amniotic fluid embolism (AFE), often associates with hyperfibrinolysis like severe TIC. However, the etiology and treatment differ significantly from severe TIC and requires VHA-guided resuscitation. The mechanism for AFE-induced coagulopathy is the release of amniotic fluid TF into the systemic and pulmonary circulation, provoking a classic DIC of overwhelming thrombin generation, consumption of clotting factors and platelets, and hyperfibrinolysis (Harnett et al., 2005; Bassily-Marcus et al., 2012).
Treatment of AFE entails the immediate delivery of the fetus, VHA-guided resuscitation of the mother, and emphasis for early administration of fibrinogen to levels that are two to three times higher than normal to outcompete platelet and clotting factor consumption, and fibrinolysis (Kramer et al., 2013; Dahlke et al., 2015; Guasch and Gilsanz, 2016; Pacheco et al., 2016; Henriquez et al., 2018; McDonnell and Browning, 2018; Amgalan et al., 2020; Liew-Spilger et al., 2021). Early PPH not associated with AFE may be diagnosed with modified VHAs designed to detect low levels of fibrinogen, which when properly treated, enable reduced incidence of shock and BCT use in women who suffer PPH (McNamara et al., 2015; Collins et al., 2017; Loughran et al., 2019; Othman et al., 2019; Liew-Spilger et al., 2021). In addition, an endogenous heparin-like substance has been associated with the coagulopathy of severe PPH and subsequent shock as identified by TEG (Wang et al., 2020). The placenta also contains high levels of TFPI (Kuczyński et al., 2002; Xiong et al., 2010).
As with late TIC and SIC as well as late PCAS, fibrinolytic shutdown post-resuscitation of PPH remains a risk factor for venous thromboembolism (VTE). Therefore, VHAs may help guide the intensity and duration of DVT prophylaxis in postpartum women, particularly those who suffered hemorrhagic shock (Maybury et al., 2008).
Figure 11 exemplifies VHA-guided resuscitation for a 35-year-old woman with cardiac arrest due to AFE (Hurwich et al., 2016). Hemostatic management of the obstetrical patient with this severe type of SHINE remains difficult without bedside adjunctive VHAs, whether due to AFE or other causes of PPH such as eclampsia, hemolysis with elevated liver enzymes and low platelets (HELLP Syndrome), or structural anomalies with and without uterine atony (Amorde et al., 2011; Loughran et al., 2019).
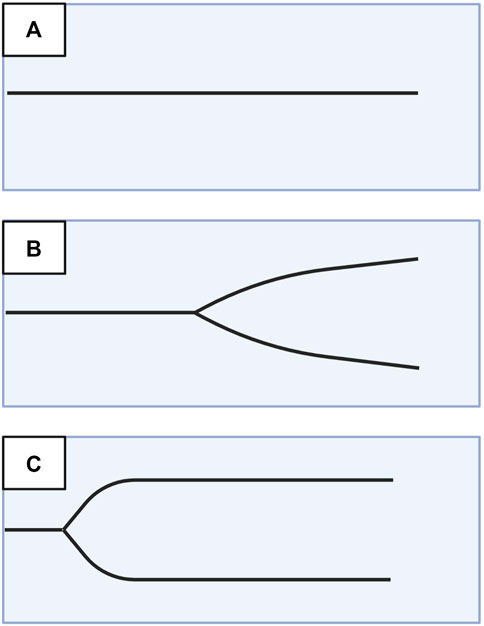
FIGURE 11. Evolution of Thromboelastography (TEG) Tracings During Resuscitation of a Patient with Amniotic Fluid Embolism. During induction of labor, a 35-year-old woman had a sudden cardiac arrest due to amniotic fluid embolism (AFE). She developed immediate disseminated intravascular coagulation, respiratory failure, and renal failure requiring mechanical ventilation and dialysis. Immediate delivery of the fetus by cesarean section was followed by normal APGAR scores at 9 min. Both child and mother were discharged from the hospital with no residual complications. TEG tracing (A) demonstrates a flat line indicating no clot formation. Two hours after the first blood draw, the laboratory called to say that the aPTT was excessively prolonged and must be a laboratory error. TEG tracing (B,C) show gradual improvement of TEG tracings at 2 and 8 h following cardiac arrest. Resuscitation required in total 12 units of packed red blood cells, six units of plasma, three units of platelets, four 10-unit doses of cryoprecipitate, two doses of recombinant factor VIIa at 80 μg/kg/dose, and 2,000 units of prothrombin complex concentrate (Hurwich et al., 2016). Abbreviations: aPTT, activated Partial Thromboplastin Time; TEG, Thromboelastography. Created with BioRender.com.
4.6 Hyperthermia/burns and non-therapeutic environmental hypothermia
Severe hyperthermia, which causes burn-induced coagulopathy (BIC), can present with early hyperfibrinolysis and hypocoagulability. With treatment of shock, these patients most often develop a hypofibrinolytic and hypercoagulable state as manifested by VHAs (Marsden et al., 2017; Borgman et al., 2019). The development of hypofibrinolysis or fibrinolytic shutdown on admission does not affect prognosis, yet at 4 h following thermal injury hyperfibrinolysis as determined by TEG portends a worse prognosis (Pusateri et al., 2020).
Hemostatic profiles of patients with BIC and hyperthermia have been studied with VHAs and demonstrate superiority to CCTs for detecting BIC (Marsden et al., 2017). Additionally, VHAs have shown to be an indispensable tool for identifying the cause of hypocoagulation in patients with severe burn injury (Presnyakova et al., 2021). ROTEM was also found to be useful in providing real-time guidance for the administration of blood products in severe burns (Bugaev et al., 2020). The resuscitation of bleeding during major burn surgery has not been standardized, yet it has been noted that TEG/ROTEM analysis of intraoperative blood samples demonstrates reduced clot strength. Therefore, it has been recommended that resuscitation of patients with burn injuries in shock should aim for normal hemostasis using point-of-care VHA monitoring of hemostatic competence during surgery and resuscitation (Welling et al., 2019). Computational thrombin modeling as well as thrombin generation assays (TGA) have confirmed the validity of VHAs to guide resuscitation for patients with severe BIC who are in shock (Ball et al., 2020). The hypercoagulable state is commonly observed in post-burn patients with BIC. An etiology for this hypercoagulable state has been suggested as the hypermetabolism of fibrinogen with subsequent increase in rebound synthesis of fibrinogen. This rebound as a reaction to enhanced fibrinogen degradation manifests by increased TEG LY60 with increased clotting speed and strength by significantly increased TEG α-angle and MA (Martini et al., 2020). Recent studies of rapid TEG (rTEG) to predict resuscitation volumes and outcomes in patients with BIC have demonstrated that 75% of these patients are hypercoagulable on admission while 25% are hypocoagulable on admission. The use of VHAs to guide the complicated resuscitation of these patients has demonstrated that as much as a five-fold increase in risk of supranormal resuscitation occurs in patients with BIC and abnormally long activated clotting times. For patients with BIC and SHINE, the use of VHAs provides essential guidance for the proper utilization not only of crystalloid, but also of other blood components (Huzar et al., 2018).
An interesting therapeutic phenomenon has been reported whereby hyperthermic patients have been treated with plasma rather than crystalloids in attempt to protect the endothelial glycocalyx. Since plasma has shown to protect the glycocalyx layer of the endothelium for TIC patients, it has been proposed that plasma is a better resuscitation fluid for patients with burn wounds for its dual abilities in restoring intravascular volume and therapeutic effect on the endothelium (Gurney et al., 2019).
At the other extreme, hypothermia alters fibrinogen and platelet function as measured by VHAs. Plasma composition which is reflected by VHAs can determine the type of coagulopathy associated with hypothermia. Even moderate hypothermia can impair thrombin generation as determined by VHA analysis. Significant hypothermia demonstrates inhibition of thrombin generation as manifested by prolonged R/CT, a reduction in the α-angle, and a significant reduction of platelet function as determined by multiple electrode aggregometry (MEA) (Mitrophanov et al., 2014; Wallner et al., 2020).
4.7 Envenomation/intoxication
VICC uncommonly causes hemorrhagic and/or distributive shock in the western world, but much more commonly afflicts rural areas and low-to-middle income countries. However, there is also increasing frequency in urban areas in Western countries due to an interest in exotic pets. In addition to the immediate treatment with antivenom to neutralize lethal toxins, transfusion of plasma, cryoprecipitate, and specific clotting factors has been clarified by using VHAs which have demonstrated procoagulant and anticoagulant effects of snake venom. It has therefore been proposed that VHAs should goal-direct hemostatic resuscitation in patients with VICC (Park et al., 2020). Using TEG with platelet mapping (TEG/PM), a case series of rattlesnake bites in North America revealed inhibition of ADP-induced platelet activation which was reversed by Crotalidae polyvalent immune Fab (ovine). Fibrinolysis was present and resolved in patients for whom serial thromboelastographs were available (Kang and Fisher, 2020).
This clinical finding has been confirmed by a study comparing the efficacy of TEG and CCTs in diagnosing simulated Crotalus atrox envenomation using human whole blood samples. TEG accurately evaluated coagulopathies caused by in vitro pit viper envenomations,confirming that VHAs are useful clinical adjuncts for evaluating VICC (Leffers et al., 2018). Figure 12 demonstrates the benefit of using adjunctive VHAs as well as CCTs to diagnose and treat the coagulopathies of patients in shock caused by envenomation (Leffers et al., 2018).
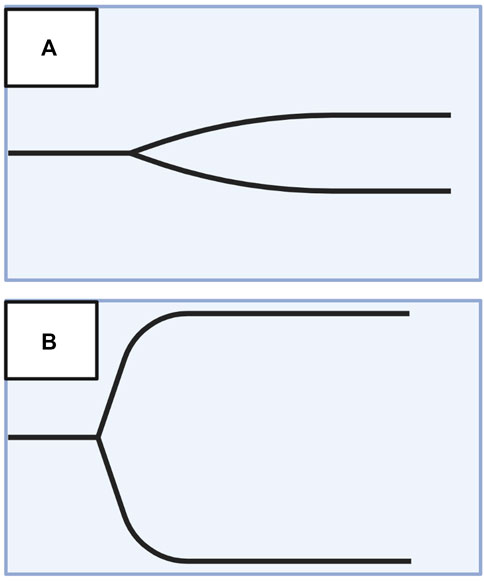
FIGURE 12. Thromboelastography (TEG) Tracings Before and After Administration of Antivenom and Blood Products. Tracing (A) demonstrates venom-induced consumption coagulopathy (VICC) with low α-angle and reduced maximum amplitude of a patient who required multiple rounds of antivenom to achieve hemostatic competence. Tracing (B) demonstrates successful treatment with resolution of VICC. In total, this patient received 24 rounds of antivenom, two units of packed red blood cells, and two units of cryoprecipitate (Leffers et al., 2018). Created with BioRender.com.
4.8 Hematologic coagulopathies
Hematologic malignancies associate with the full spectrum of hemostatic phenotypes. Acute promyelocytic leukemia (APL) and myeloproliferative disorders such as polycythemia vera best exemplify the extremes of this spectrum. APL most often manifests a hypocoagulable hyperfibrinolytic phenotype, whereas as patients with polycythemia vera are hypercoagulable (Walsh et al., 2019; Walsh et al., 2020a). Patients with APL may present with shock associated with hyperfibrinolysis which progresses soon following treatment with all-trans-retinoic acid to a fibrinolytic shutdown phenotype (Tallman and Kwaan, 1992; Zakarija and Kwaan, 2007; Stein et al., 2009; Kwaan and Rego, 2010; Kwaan et al., 2011; Liu et al., 2011; Kwaan and Cull, 2014; Dobson et al., 2015). This pattern of rapid transition from the hyperfibrinolytic phenotype to fibrinolytic shutdown reflects a similar pattern seen in the acute phase of severe TIC which progresses from hyper-to hypo-fibrinolysis within minutes to hours (Tallman and Kwaan, 1992; Zakarija and Kwaan, 2007; Stein et al., 2009; Kwaan and Rego, 2010; Kwaan et al., 2011; Liu et al., 2011; Kwaan and Cull, 2014; Moore et al., 2015a). In APL, the hyperfibrinolytic phenotype is driven by increased expression of tPA, annexin A2, and uPA. Inhibitors of the coagulation cascade such as TFPI are present in APL and may further contribute to this hyperfibrinolytic state (Kwaan and Rego, 2010; Kwaan and Cull, 2014). Therefore, whether caused by SIC or trauma, TEG/ROTEM allow for evaluation of the patient’s phenotype along the spectrum of hypocoagulable/hyperfibrinolytic to hypercoagulable/hypofibrinolytic.
For patients with non-malignant etiologies such as hemophilias or thrombocytopenias who are in shock, VHAs may direct resuscitation by defining the hemostatic phenotype and guiding BCT and HAT (Speybroeck et al., 2020; Bunch et al., 2022a).
4.9 Traumatic brain injury
Coagulopathy of traumatic brain injury (CTBI) perturbs primary hemostasis, and VHAs can be used to monitor the progression of platelet dysfunction (Castellino et al., 2014; Sixta et al., 2015; Maegele et al., 2017; Samuels et al., 2019; Maegele et al., 2020; Webb et al., 2021). Early after traumatic brain injury (TBI), VHAs have shown inhibition of platelet receptors resulting in impaired reactivity at the receptors for ADP, AA (Jacoby et al., 2001; Nekludov et al., 2007; Windelv et al., 2011; Wohlauer et al., 2012; Davis et al., 2013; Castellino et al., 2014), collagen, ristocetin, and thrombin receptor activating peptides (TRAP) (Solomon et al., 2011; Kutcher et al., 2012; Dragan et al., 2021). The severity of TBI correlates to the degree of platelet receptor inhibition. Evidence suggests that CTBI may arise from the disruption of local primary hemostasis and systemic endotheliopathy which are caused primarily by the overwhelming release of von Willebrand Factor (VWF) and TF from the injured brain tissue (Wu et al., 2018; Xu et al., 2020). The circulating supraphysiologic VWF and TF likely activate platelets without adhering to a surface distant from the site of injury, creating a pool of circulating activated but exhausted platelets that are incapable of aggregation (Moore et al., 2021a). VHAs may quantify dysfunction of various platelet receptors and thus, VHAs with specialized function analysis are significantly more effective in monitoring hemostasis and prognostication in CTBI than CCTs, as has been shown for both isolated and multiple systemic trauma (Nekludov et al., 2007; Simard et al., 2009; Lustenberger et al., 2010; Kurland et al., 2012; Laroche et al., 2012; Wohlauer et al., 2012; Davis et al., 2013; Castellino et al., 2014; Nekludov et al., 2014; Hijazi et al., 2015; Medcalf, 2015; Dekker et al., 2016; Di Battista et al., 2016; Foley and Conway, 2016; Yuan et al., 2016; Maegele et al., 2017; Fletcher-Sandersjöö et al., 2020; Bradbury et al., 2021; Cannon et al., 2021). In addition to local and systemic procoagulation, patients with CTBI more commonly present with hypofibrinolysis than hyperfibrinolysis. Coupled with procoagulation, fibrinolytic shutdown leads to the consumption of platelets, coagulation factors, and fibrinogen which ultimately results in bleeding and secondary hemorrhagic progression of TBI in the late phase of CTBI (Hoffman and Monroe, 2009; Tian et al., 2010; Herbert et al., 2017; Maegele et al., 2017; Maegele et al., 2020). However, the sensitivity of VHAs to detect occult fibrinolysis in patients with CTBI is a topic of ongoing discussion (Cotton et al., 2012; Medcalf, 2015; Moore et al., 2016b; Rowell et al., 2020). Resuscitation of patients with TBI who are in shock therefore requires an understanding of the numerous contributing factors to CTBI wherein VHAs provide a greater hemostatic profile.
4.10 Pediatrics
Another area where definition of coagulopathies based on endothelial changes will result in much different treatments than reliance on CCTs alone is the pediatric patient whose endothelium differs significantly from the adult or PPH patient with SHINE. VHAs for decades have guided resuscitation of pediatric patients undergoing liver transplantation, cardiac surgery, cardiac transplantation, and traumatic injury. In addition, VHAs have been monitored hemostasis for patients on ECMO, VADs, continuous veno-venous hemofiltration, and continuous veno-venous plasma filtration (Haas and Faraoni, 2020). The endothelium of the pediatric population undergoes similar changes under conditions of SHINE as with the adult, although the urgency of diagnosis and treatment is much greater and therefore, consideration must be made for the use of these tests for pediatric patients in shock (Richter et al., 2022b).
Endothelial dysfunction has been implicated in pediatric critical illness and is theorized to result from glycocalyx disruption (Puchwein-Schwepcke et al., 2021). As the child develops, the endothelial glycocalyx thins due to blood vessel aging, cumulative exposure to inflammation, and other comorbidities (Richter et al., 2022b). Vascular stiffness increases with age due to physiologic elevations in blood pressure which contributes to the thinning of the glycocalyx via suppression of the glycocalyx core protein glypican 1 (Mahmoud et al., 2021). Additionally, the immune system develops to mount stronger responses to a wide range of pathogens, increasing baseline local and systemic inflammation in the setting of an acute response to infection (Richter et al., 2022b). Comorbid conditions such as cyanotic heart defects, lung vascular malformations, bronchopulmonary dysplasia, and type I diabetes mellitus increase endothelial exposure to reactive oxygen species which drives endothelial cells into premature senescence marked by thinning of the glycocalyx (Erusalimsky and Skene, 2009; Jackson-Weaver et al., 2019). In a critically ill pediatric patient, the joint effect of blood vessel development, immune system maturation, comorbidities, and insults such as trauma or sepsis may cause an acute disruption of the glycocalyx with subsequent vascular pathology and coagulopathies like the progression seen in SHINE. Hence, real-time monitoring of coagulopathies with VHAs may be useful in the care of critically ill pediatric patients (Saini et al., 2019).
It has been shown in pediatric intensive care units that TEG changes the treatment for 47% of patients and provides a better understanding of the hemostatic phenotype in 69% of patients (Carter et al., 2017).
The investigation of endothelial dysfunction in the pediatric population has recently been enhanced by the presence of multisystem inflammatory syndrome in children (MIS-C). Abnormal levels of angiopoietin-2, sE-selectin, and VWF antigen correlate with the vasoactive and inotropic score in patients with severe acute respiratory syndrome coronavirus 2 (SARS-CoV-2)-related MIS-C with shock (Borgel et al., 2021). For MIS-C, inflammatory markers and ROTEM parameters significantly correlate which are indicative of hypercoagulability and elevated fibrinogen activity by FIBTEM MCF and elevated D-dimer. Similar to the adult population with septic shock, pediatric patients with MIS-C who develop shock and SHINE may also benefit from the use of VHAs to guide diagnosis and resuscitation (Al-Ghafry et al., 2021).
5 Conclusion
The appropriate treatment of shock-associated coagulopathies mandates a personalized approach to hemostatic resuscitation. VHAs empower the clinician to appreciate the patient’s position along the spectrum of coagulofibrinolysis, and it is this appreciation of the various hemostatic phenotypes which enable the accurate diagnosis and physiologic treatment of patients with all forms of shock. Until recently, VHAs for hemostatic monitoring of patients in shock was limited to operative settings of liver transplantation and cardiac surgery. Subsequent evidenced-based protocols have validated VHA-guided resuscitation for TIC. VHAs have likewise recently expanded use to treating medical causes of bleeding associated with PPH, GI bleedings, liver failure, and septic shock. Earlier identification of coagulopathic and endotheliopathic patients with VHAs and endothelial biomarkers may enable more timely and targeted resuscitation for those patients in all forms of shock. However, further investigation is needed to elucidate the causal or correlational relationship of endotheliopathy with progressive shock, and whether restorative endothelial therapies manifest a mortality benefit.
Author contributions
Conceptualization: CB, EC, EM, HM, HK, JM, MrW, and MmW; Original Draft Preparation: CB, EC, EM, HM, HK, JM, AT, NZ, and MmW; Writing—Review and Editing: CB, EC, EM, HM, HK, JM, AT, NZ, MA-F, SP, SZ, SH, BP, MM, ST, DZ, DF, PK, DH, MrW, AK, MA, and MmW; Visualization: CB and MmW; Supervision: CB and MmW; All authors contributed to the article and approved the submitted version.
Funding
This review was independently funded among the authors.
Acknowledgments
The authors appreciate Amanda L. Kelly’s medical librarian services from the Sladen Library at Henry Ford Hospital for her assistance in the initial literature search.
Conflict of interest
EM and HM have received research grants from Haemonetics Corporation outside the submitted work. Author MrW is employed by the company Cardinal Flow Assurance LLC.
The remaining authors declare that the research was conducted in the absence of any commercial or financial relationships that could be construed as a potential conflict of interest.
The reviewer, AD, declared a shared parent affiliation with the author MM to the handling editor at the time of review.
Publisher’s note
All claims expressed in this article are solely those of the authors and do not necessarily represent those of their affiliated organizations, or those of the publisher, the editors and the reviewers. Any product that may be evaluated in this article, or claim that may be made by its manufacturer, is not guaranteed or endorsed by the publisher.
References
Adamzik, M., Langemeier, T., Frey, U. H., Görlinger, K., Saner, F., Eggebrecht, H., et al. (2011). Comparison of thrombelastometry with simplified acute physiology score II and sequential organ failure assessment scores for the prediction of 30-day survival: A cohort study. Shock 35 (4), 339–342. doi:10.1097/SHK.0b013e318204bff6
Adrie, C., Adib-Conquy, M., Laurent, I., Monchi, M., Vinsonneau, C., Fitting, C., et al. (2002). Successful cardiopulmonary resuscitation after cardiac arrest as a “sepsis-like” syndrome. Circulation 106 (5), 562–568. doi:10.1161/01.cir.0000023891.80661.ad
Adrie, C., Laurent, I., Monchi, M., Cariou, A., Dhainaou, J.-F., and Spaulding, C. (2004). Postresuscitation disease after cardiac arrest: A sepsis-like syndrome? Curr. Opin. Crit. care 10 (3), 208–212. doi:10.1097/01.ccx.0000126090.06275.fe
Adrie, C., Monchi, M., Laurent, I., Um, S., Yan, S. B., Thuong, M., et al. (2005). Coagulopathy after successful cardiopulmonary resuscitation following cardiac arrest: Implication of the protein C anticoagulant pathway. J. Am. Coll. Cardiol. 46 (1), 21–28. doi:10.1016/j.jacc.2005.03.046
Agarwal, B., Wright, G., Gatt, A., Riddell, A., Vemala, V., Mallett, S., et al. (2012). Evaluation of coagulation abnormalities in acute liver failure. J. Hepatol. 57 (4), 780–786. doi:10.1016/j.jhep.2012.06.020
Aird, W. C. (2004). Endothelium as an organ system. Crit. care Med. 32 (5), S271–S279. doi:10.1097/01.ccm.0000129669.21649.40
Aird, W. C. (2006). Mechanisms of endothelial cell heterogeneity in health and disease. Circ. Res. 98(2):159–162. doi:10.1161/01.RES.0000204553.32549.a7
Al-Ghafry, M., Vagrecha, A., Malik, M., Levine, C., Uster, E., Aygun, B., et al. (2021). Multisystem inflammatory syndrome in children (mis-C) and the prothrombotic state: Coagulation profiles and rotational thromboelastometry in a mis-C cohort. J. Thrombosis Haemostasis 19 (7), 1764–1770. doi:10.1111/jth.15340
Ames, A., Wright, R. L., Kowada, M., Thurston, J. M., and Majno, G. (1968). Cerebral ischemia. II. The no-reflow phenomenon. Am. J. Pathol. 52 (2), 437–453.
Amgalan, A., Allen, T., Othman, M., and Ahmadzia, H. K. (2020). Systematic review of viscoelastic testing (TEG/ROTEM) in obstetrics and recommendations from the Women's SSC of the ISTH. J. Thromb. Haemost. 18 (8), 1813–1838. doi:10.1111/jth.14882
Amorde, R. W., Patel, S. N., and Pagel, P. S. (2011). Management of labor and delivery of a patient with von Willebrand disease type 2a. Int. Anesthesiol. Clin. 49 (2), 74–80. doi:10.1097/AIA.0b013e3181f89bc9
Anastasiou, G., Gialeraki, A., Merkouri, E., Politou, M., and Travlou, A. (2012). Thrombomodulin as a regulator of the anticoagulant pathway: Implication in the development of thrombosis. Blood Coagul. Fibrinolysis 23 (1), 1–10. doi:10.1097/MBC.0b013e32834cb271
André, P. (2004). P-selectin in haemostasis. Br. J. Haematol. 126 (3), 298–306. doi:10.1111/j.1365-2141.2004.05032.x
Angus, D. C., and Van der Poll, T. (2013). Severe sepsis and septic shock. N. Engl. J. Med. 369, 840–851. doi:10.1056/NEJMra1208623
Artang, R., Anderson, M., and Nielsen, J. D. (2019). Fully automated thromboelastograph TEG 6s to measure anticoagulant effects of direct oral anticoagulants in healthy male volunteers. Res. Pract. Thromb. Haemost. 3 (3), 391–396. doi:10.1002/rth2.12206
Ball, R. L., Keyloun, J. W., Brummel-Ziedins, K., Orfeo, T., Palmieri, T. L., Johnson, L. S., et al. (2020). Burn-induced coagulopathies: A comprehensive review. Shock (Augusta, Ga.) 54 (2), 154–167. doi:10.1097/SHK.0000000000001484
Bamber, J. (2016). Point-of-care testing on the labour ward should be mandatory. Int. J. Obstetric Anesth. 27, 69–74. doi:10.1016/j.ijoa.2016.07.001
Barea-Mendoza, J., Terceros-Almanza, L., García-Fuentes, C., Bermejo-Aznárez, S., Del Portillo, I. P., Mudarra-Reche, C., et al. (2019). Rotational thromboelastometry (ROTEM) profile in a cohort of asystole donors. Med. Intensiva Engl. Ed. 43 (7), 410–415. doi:10.1016/j.medin.2018.04.007
Bassily-Marcus, A. M., Yuan, C., Oropello, J., Manasia, A., Kohli-Seth, R., and Benjamin, E. (2012). Pulmonary hypertension in pregnancy: Critical care management. Pulm. Med. 2012, 709407. doi:10.1155/2012/709407
Beckmann, J. S., and Lew, D. (2016). Reconciling evidence-based medicine and precision medicine in the era of big data: Challenges and opportunities. Genome Med. 8 (1), 134. doi:10.1186/s13073-016-0388-7
Bell, S., Roberts, T., Pereira, J. F. M., De Lloyd, L., Amir, Z., James, D., et al. (2022). The sensitivity and specificity of rotational thromboelastometry (ROTEM) to detect coagulopathy during moderate and severe postpartum haemorrhage: A prospective observational study. Int. J. Obstetric Anesth. 49, 103238. doi:10.1016/j.ijoa.2021.103238
Berthelsen, R. E., Ostrowski, S. R., Bestle, M. H., and Johansson, P. I. (2019). Co-Administration of iloprost and eptifibatide in septic shock (CO-ilepss)—A randomised, controlled, double-blind investigator-initiated trial investigating safety and efficacy. Crit. Care 23 (1), 301–310. doi:10.1186/s13054-019-2573-8
Bestle, M. H., Clausen, N. E., Søe-Jensen, P., Kristiansen, K. T., Lange, T., Johansson, P. I., et al. (2020). Efficacy and safety of iloprost in patients with septic shock-induced endotheliopathy—protocol for the multicenter randomized, placebo-controlled, blinded, investigator-initiated trial. Acta Anaesthesiol. Scand. 64 (5), 705–711. doi:10.1111/aas.13546
Betteridge, K. B., Arkill, K. P., Neal, C. R., Harper, S. J., Foster, R. R., Satchell, S. C., et al. (2017). Sialic acids regulate microvessel permeability, revealed by novel in vivo studies of endothelial glycocalyx structure and function. J. Physiol. 595 (15), 5015–5035. doi:10.1113/jp274167
Bliden, K., Zaman, F., Taheri, H., Ilkhanoff, L., Sandesara, C., Blessy, M., et al. (2017). P5598Multicenter observational trial to evaluate TEG6s oral anticoagulant assay in apixaban-treated patients. Eur. Heart J. 38 (1). doi:10.1093/eurheartj/ehx493.p5598
Borgel, D., Chocron, R., Grimaud, M., Philippe, A., Chareyre, J., Brakta, C., et al. (2021). Endothelial dysfunction as a component of severe acute respiratory syndrome coronavirus 2–related multisystem inflammatory syndrome in children with shock. Crit. care Med. 49 (11), e1151–e1156. doi:10.1097/CCM.0000000000005093
Borgman, M. A., Zaar, M., Aden, J. K., Schlader, Z. J., Gagnon, D., Rivas, E., et al. (2019). Hemostatic responses to exercise, dehydration, and simulated bleeding in heat-stressed humans. Am. J. Physiology-Regulatory, Integr. Comp. Physiology 316 (2), R145–R156. doi:10.1152/ajpregu.00223.2018
Bradbury, J. L., Thomas, S. G., Sorg, N. R., Mjaess, N., Berquist, M. R., Brenner, T. J., et al. (2021). Viscoelastic testing and coagulopathy of traumatic brain injury. J. Clin. Med. 10 (21), 5039. doi:10.3390/jcm10215039
Brenner, T., Schmidt, K., Delang, M., Mehrabi, A., Bruckner, T., Lichtenstern, C., et al. (2012). Viscoelastic and aggregometric point-of-care testing in patients with septic shock - cross-links between inflammation and haemostasis. Acta Anaesthesiol. Scand. 56 (10), 1277–1290. doi:10.1111/j.1399-6576.2012.02750.x
Brill, J. B., Cotton, B. A., and Lawless, R. A. (2021). “Plasma transfusion,” in Trauma induced coagulopathy. Editors H. B. Moore, E. E. Moore, and M. D Neal. Second ed (Switzerland: Spring Nature Switzerland AG), 353–371.
Brinkmann, V., Reichard, U., Goosmann, C., Fauler, B., Uhlemann, Y., Weiss, D. S., et al. (2004). Neutrophil extracellular traps kill bacteria. science 303 (5663), 1532–1535. doi:10.1126/science.1092385
Brohi, K., Singh, J., Heron, M., and Coats, T. (2003). Acute traumatic coagulopathy. J. Trauma Acute Care Surg. 54 (6), 1127–1130. doi:10.1097/01.TA.0000069184.82147.06
Bruckbauer, M., Prexl, O., Voelckel, W., Ziegler, B., Grottke, O., Maegele, M., et al. (2019). Impact of direct oral anticoagulants in patients with hip fractures. J. Orthop. Trauma 33 (1), e8–e13. doi:10.1097/BOT.0000000000001329
Bugaev, N., Como, J. J., Golani, G., Freeman, J. J., Sawhney, J. S., Vatsaas, C. J., et al. (2020). Thromboelastography and rotational thromboelastometry in bleeding patients with coagulopathy: Practice management guideline from the Eastern Association for the Surgery of Trauma. J. Trauma Acute Care Surg. 89 (6), 999–1017. doi:10.1097/TA.0000000000002944
Bunch, C. M., Berquist, M., Ansari, A., McCoy, M. L., Langford, J. H., Brenner, T. J., et al. (2022a). The choice between plasma-based common coagulation tests and cell-based viscoelastic tests in monitoring hemostatic competence: Not an either-or proposition. Semin. Thromb. Hemost. 48 (7), 769–784. doi:10.1055/s-0042-1756302
Bunch, C. M., Moore, E. E., Moore, H. B., Neal, M. D., Thomas, A. V., Zackariya, N., et al. (2022b). Immuno-thrombotic complications of COVID-19: Implications for timing of surgery and anticoagulation. Front. Surg. 9, 889999. doi:10.3389/fsurg.2022.889999
Bunch, C. M., Thomas, A. V., Stillson, J. E., Gillespie, L., Khan, R. Z., Zackariya, N., et al. (2021). Preventing thrombohemorrhagic complications of heparinized COVID-19 patients using adjunctive thromboelastography: A retrospective study. J. Clin. Med. 10 (14), 3097. doi:10.3390/jcm10143097
Cannon, J. W., Dias, J. D., Kumar, M. A., Walsh, M., Thomas, S. G., Cotton, B. A., et al. (2021). Use of thromboelastography in the evaluation and management of patients with traumatic brain injury: A systematic review and meta-analysis. Crit. care Explor. 3 (9), e0526. doi:10.1097/CCE.0000000000000526
Cannon, J. W. (2018). Prehospital damage-control resuscitation. N. Engl. J. Med. 379 (4), 387–388. doi:10.1056/NEJMe1805705
Carge, M. J., Liberati, D. M., and Diebel, L. N. (2021). A biomimetic shock model on the effect of endothelial aging on vascular barrier properties. J. Trauma Acute Care Surg. 91 (5), 849–855. doi:10.1097/ta.0000000000003207
Carter, B., Carland, E., Monagle, P., Horton, S., and Butt, W. (2017). Impact of thrombelastography in paediatric intensive care. Anaesth. Intensive Care 45 (5), 589–599. doi:10.1177/0310057X1704500509
Castellino, F. J., Chapman, M. P., Donahue, D. L., Thomas, S., Moore, E. E., Wohlauer, M. V., et al. (2014). Traumatic brain injury causes platelet adenosine diphosphate and arachidonic acid receptor inhibition independent of hemorrhagic shock in humans and rats. J. Trauma Acute Care Surg. 76 (5), 1169–1176. doi:10.1097/TA.0000000000000216
Chau, T., Chan, Y., Patch, D., Tokunaga, S., Greenslade, L., and Burroughs, A. (1998). Thrombelastographic changes and early rebleeding in cirrhotic patients with variceal bleeding. Gut 43 (2), 267–271. doi:10.1136/gut.43.2.267
Choi, Q., Hong, K. H., Kim, J.-E., and Kim, H. K. (2014). Changes in plasma levels of natural anticoagulants in disseminated intravascular coagulation: High prognostic value of antithrombin and protein C in patients with underlying sepsis or severe infection. Ann. laboratory Med. 34 (2), 85–91. doi:10.3343/alm.2014.34.2.85
Claesen, K., Mertens, J. C., Leenaerts, D., and Hendriks, D. (2021). Carboxypeptidase U (CPU, TAFIa, CPB2) in thromboembolic disease: What do we know three decades after its discovery? Int. J. Mol. Sci. 22 (2), 883. doi:10.3390/ijms22020883
Cohen, J., Vincent, J.-L., Adhikari, N. K., Machado, F. R., Angus, D. C., Calandra, T., et al. (2015). Sepsis: A roadmap for future research. Lancet Infect. Dis. 15 (5), 581–614. doi:10.1016/S1473-3099(15)70112-X
Cohen, M. J., Call, M., Nelson, M., Calfee, C. S., Esmon, C. T., Brohi, K., et al. (2012). Critical role of activated protein C in early coagulopathy and later organ failure, infection and death in trauma patients. Ann. Surg. 255 (2), 379–385. doi:10.1097/SLA.0b013e318235d9e6
Collins, F. S., and Varmus, H. (2015). A new initiative on precision medicine. N. Engl. J. Med. 372 (9), 793–795. doi:10.1056/NEJMp1500523
Collins, P. (2022). Point of care coagulation testing for postpartum haemorrhage. Best Pract. Res. Clin. Anaesthesiol. 36, 383–398. doi:10.1016/j.bpa.2022.08.002
Collins, P. W., Bell, S. F., de Lloyd, L., and Collis, R. E. (2019). Management of postpartum haemorrhage: From research into practice, a narrative review of the literature and the cardiff experience. Int. J. Obstet. Anesth. 37, 106–117. doi:10.1016/j.ijoa.2018.08.008
Collins, P. W., Cannings-John, R., Bruynseels, D., Mallaiah, S., Dick, J., Elton, C., et al. (2017). Viscoelastometry guided fresh frozen plasma infusion for postpartum haemorrhage: OBS2, an observational study. Br. J. Anaesth. 119 (3), 422–434. doi:10.1093/bja/aex245
Collins, P. W., Lilley, G., Bruynseels, D., Laurent, D. B., Cannings-John, R., Precious, E., et al. (2014). Fibrin-based clot formation as an early and rapid biomarker for progression of postpartum hemorrhage: A prospective study. Blood 124 (11), 1727–1736. doi:10.1182/blood-2014-04-567891
Collis, R. (2016). Coagulation point-of-care testing on the labour ward should be mandatory. Int. J. Obstetric Anesth. 27, 66–69. doi:10.1016/j.ijoa.2016.06.006
Collis, R. E., and Collins, P. W. (2015). Haemostatic management of obstetric haemorrhage. Anaesthesia 70 (1), 78–86. doi:10.1111/anae.12913
Colman, E., Yin, E. B., Laine, G., Chatterjee, S., Saatee, S., Herlihy, J. P., et al. (2019). Evaluation of a heparin monitoring protocol for extracorporeal membrane oxygenation and review of the literature. J. Thorac. Dis. 11 (8), 3325–3335. doi:10.21037/jtd.2019.08.44
Cotton, B. A., Harvin, J. A., Kostousouv, V., Minei, K. M., Radwan, Z. A., Schöchl, H., et al. (2012). Hyperfibrinolysis at admission is an uncommon but highly lethal event associated with shock and prehospital fluid administration. J. Trauma Acute Care Surg. 73 (2), 365–370. doi:10.1097/TA.0b013e31825c1234
Curry, N. S., Davenport, R., Pavord, S., Mallett, S. V., Kitchen, D., Klein, A. A., et al. (2018). The use of viscoelastic haemostatic assays in the management of major bleeding: A British society for haematology guideline. Br. J. Haematol. 182 (6), 789–806. doi:10.1111/bjh.15524
Dahlke, J. D., Mendez-Figueroa, H., Maggio, L., Hauspurg, A. K., Sperling, J. D., Chauhan, S. P., et al. (2015). Prevention and management of postpartum hemorrhage: A comparison of 4 national guidelines. Am. J. Obstet. Gynecol. 213 (1), 76.e1–76.76.e10. doi:10.1016/j.ajog.2015.02.023
Davenport, R. A., Guerreiro, M., Frith, D., Rourke, C., Platton, S., Cohen, M., et al. (2017). Activated protein C drives the hyperfibrinolysis of acute traumatic coagulopathy. Anesthesiology 126 (1), 115–127. doi:10.1097/ALN.0000000000001428
Davis, P. K., Musunuru, H., Walsh, M., Cassady, R., Yount, R., Losiniecki, A., et al. (2013). Platelet dysfunction is an early marker for traumatic brain injury-induced coagulopathy. Neurocrit Care 18 (2), 201–208. doi:10.1007/s12028-012-9745-6
Deaglio, S., and Robson, S. C. (2011). Ectonucleotidases as regulators of purinergic signaling in thrombosis, inflammation, and immunity. Adv. Pharmacol. 61, 301–332. doi:10.1016/b978-0-12-385526-8.00010-2
Declerck, P. J., and Gils, A. (2013). Three decades of research on plasminogen activator inhibitor-1: A multifaceted serpin. Seminars thrombosis hemostasis 39 (04), 356–364. doi:10.1055/s-0033-1334487
Declerck, P. (2011). Thrombin activatable fibrinolysis inhibitor. Hämostaseologie 31 (03), 165–168. doi:10.5482/ha-1155
Dekker, S. E., Duvekot, A., de Vries, H.-M., Geeraedts, L. M., Peerdeman, S. M., de Waard, M. C., et al. (2016). Relationship between tissue perfusion and coagulopathy in traumatic brain injury. J. Surg. Res. 205 (1), 147–154. doi:10.1016/j.jss.2016.06.023
Deracinois, B., Lenfant, A.-M., Dehouck, M.-P., and Flahaut, C. (2015). Tissue non-specific alkaline phosphatase (TNAP) in vessels of the brain. Subcell. Biochem. 76, 125–151. Neuronal tissue-nonspecific alkaline phosphatase (TNAP). doi:10.1007/978-94-017-7197-9_7
Di Bartolomeo, A., Chauleur, C., Gris, J. C., Chapelle, C., Noblot, E., Laporte, S., et al. (2017). Tissue factor pathway inhibitor for prediction of placenta-mediated adverse pregnancy outcomes in high-risk women: AngioPred study. PLoS One 12 (3), e0173596. doi:10.1371/journal.pone.0173596
Di Battista, A. P., Rizoli, S. B., Lejnieks, B., Min, A., Shiu, M. Y., Peng, H. T., et al. (2016). Sympathoadrenal activation is associated with acute traumatic coagulopathy and endotheliopathy in isolated brain injury. Shock 46 (3), 96–103. doi:10.1097/SHK.0000000000000642
Dias, J. D., Lopez-Espina, C. G., Ippolito, J., Hsiao, L. H., Zaman, F., Muresan, A. A., et al. (2019). Rapid point-of-care detection and classification of direct-acting oral anticoagulants with the TEG 6s: Implications for trauma and acute care surgery. J. Trauma Acute Care Surg. 87 (2), 364–370. doi:10.1097/TA.0000000000002357
Diez, N., Montes, R., Alonso, A., Medina, P., Navarro, S., Espana, F., et al. (2006). Association of increased fibrinogen concentration with impaired activation of anticoagulant protein C. J. Thromb. Haemost. 4 (2), 398–402. doi:10.1111/j.1538-7836.2006.01746.x
Dobson, G. P., Letson, H. L., Sharma, R., Sheppard, F. R., and Cap, A. P. (2015). Mechanisms of early trauma-induced coagulopathy: The clot thickens or not? J. Trauma Acute Care Surg. 79 (2), 301–309. doi:10.1097/TA.0000000000000729
Dobson, G. P., Morris, J. L., and Letson, H. L. (2022). Why are bleeding trauma patients still dying? Towards a systems hypothesis of trauma. Front. Physiol. 13, 990903. doi:10.3389/fphys.2022.990903
Dolgov, V., Makhmudov, R., Bondarenko, M., and Repin, V. (1984). Deleterious action of adrenaline on the endothelial lining of the vessels. Arkhiv Patol. 46 (10), 31–36.
Douketis, J. D., Spyropoulos, A. C., Anderson, J. M., Arnold, D. M., Bates, S. M., Blostein, M., et al. (2017). The perioperative anticoagulant use for surgery evaluation (PAUSE) study for patients on a direct oral anticoagulant who need an elective surgery or procedure: Design and rationale. Thromb. Haemost. 117 (12), 2415–2424. doi:10.1160/th17-08-0553
Dragan, B., Adamik, B., Burzynska, M., Dragan, S. L., and Gozdzik, W. (2021). Platelet receptor activity for predicting survival in patients with intracranial bleeding. J. Clin. Med. 10 (10), 2205. doi:10.3390/jcm10102205
Drost, C. C., Rovas, A., and Kümpers, P. (2021). Protection and rebuilding of the endothelial glycocalyx in sepsis - science or fiction? Matrix Biol. Plus 12, 100091. doi:10.1016/j.mbplus.2021.100091
Dubois, V., Dincq, A.-S., Douxfils, J., Ickx, B., Samama, C.-M., Dogné, J.-M., et al. (2017). Perioperative management of patients on direct oral anticoagulants. Thromb. J. 15 (1), 14–17. doi:10.1186/s12959-017-0137-1
Dujardin, R. W. G., Kisters, J. E. C., Wirtz, M. R., Hajji, N., Tuip-de Boer, A. M., Stensballe, J., et al. (2022). Shock-driven endotheliopathy in trauma patients is associated with leucocyte derived extracellular vesicles. Int. J. Mol. Sci. 23 (24), 15990. doi:10.3390/ijms232415990
Dunne, J. R., Malone, D. L., Tracy, J. K., and Napolitano, L. M. (2004). Allogenic blood transfusion in the first 24 hours after trauma is associated with increased systemic inflammatory response syndrome (SIRS) and death. Surg. Infect. (Larchmt) 5 (4), 395–404. doi:10.1089/sur.2004.5.395
Dupuis, C., Bouadma, L., Ruckly, S., Perozziello, A., Van-Gysel, D., Mageau, A., et al. (2020). Sepsis and septic shock in France: Incidences, outcomes and costs of care. Ann. Intensive Care 10 (1), 145. doi:10.1186/s13613-020-00760-x
Egi, M., Ogura, H., Yatabe, T., Atagi, K., Inoue, S., Iba, T., et al. (2021). The Japanese clinical practice guidelines for management of sepsis and septic shock 2020 (J-SSCG 2020). J. Intensive Care 9 (1), 53–144. doi:10.1186/s40560-021-00555-7
Enriquez, L. J., and Shore-Lesserson, L. (2009). Point-of-care coagulation testing and transfusion algorithms. Br. J. Anaesth. 103 (1), i14–i22. doi:10.1093/bja/aep318
Erusalimsky, J. D., and Skene, C. (2009). Mechanisms of endothelial senescence. Exp. Physiol. 94 (3), 299–304. doi:10.1113/expphysiol.2008.043133
Farrugia, B. L., Lord, M. S., Melrose, J., and Whitelock, J. M. (2018). The role of heparan sulfate in inflammation, and the development of biomimetics as anti-inflammatory strategies. J. Histochem Cytochem 66 (4), 321–336. doi:10.1369/0022155417740881
Faust, S. N., Levin, M., Harrison, O. B., Goldin, R. D., Lockhart, M. S., Kondaveeti, S., et al. (2001). Dysfunction of endothelial protein C activation in severe meningococcal sepsis. N. Engl. J. Med. 345 (6), 408–416. doi:10.1056/NEJM200108093450603
Field, A., Poole, T., and Bamber, J. (2019). ROTEM® sigma reference range validity. Anaesthesia 74 (8), 1063. doi:10.1111/anae.14711
Figueroa, X. F., Chen, C. C., Campbell, K. P., Damon, D. N., Day, K. H., Ramos, S., et al. (2007). Are voltage-dependent ion channels involved in the endothelial cell control of vasomotor tone? Am. J. Physiol. Heart Circ. Physiol. 293 (3), H1371–H1383. doi:10.1152/ajpheart.01368.2006
Fishman, A. P. (1982). Endothelium: A distributed organ of diverse capabilities. Ann. N. Y. Acad. Sci. 401, 1–8. doi:10.1111/j.1749-6632.1982.tb25702.x
Fletcher-Sandersjöö, A., Maegele, M., and Bellander, B.-M. (2020). Does complement-mediated hemostatic disturbance occur in traumatic brain injury? A literature review and observational study protocol. Int. J. Mol. Sci. 21 (5), 1596. doi:10.3390/ijms21051596
Foley, J. H., and Conway, E. M. (2016). Cross talk pathways between coagulation and inflammation. Circ. Res. 118 (9), 1392–1408. doi:10.1161/CIRCRESAHA.116.306853
Foley, J., Kim, P., Mutch, N., and Gils, A. (2013). Insights into thrombin activatable fibrinolysis inhibitor function and regulation. J. Thrombosis Haemostasis 11, 306–315. doi:10.1111/jth.12216
Fritsma, G. (2020). “Laboratory evaluation of hemostasis,” in Rodak's hematology. Editors C. N. O. E M. Keohane, and J M Walenga. 6th ed (Elsevier), 765–792.
Gando, S., and Hayakawa, M. (2016). Pathophysiology of trauma-induced coagulopathy and management of critical bleeding requiring massive transfusion. Semin. Thromb. Hemost. 42 (2), 155–165. doi:10.1055/s-0035-1564831
Gando, S., Mayumi, T., and Ukai, T. (2018). The roles of activated protein C in experimental trauma models. Chin. J. Traumatol. 21 (6), 311–315. doi:10.1016/j.cjtee.2018.07.005
Gando, S., Nakanishi, Y., and Tedo, I. (1995). Cytokines and plasminogen activator inhibitor-1 in posttrauma disseminated intravascular coagulation: Relationship to multiple organ dysfunction syndrome. Crit. Care Med. 23 (11), 1835–1842. doi:10.1097/00003246-199511000-00009
Gando, S., and Otomo, Y. (2015). Local hemostasis, immunothrombosis, and systemic disseminated intravascular coagulation in trauma and traumatic shock. Crit. Care 19 (1), 72. doi:10.1186/s13054-015-0735-x
Gando, S., Sawamura, A., and Hayakawa, M. (2011). Trauma, shock, and disseminated intravascular coagulation: Lessons from the classical literature. Ann. Surg. 254 (1), 10–19. doi:10.1097/SLA.0b013e31821221b1
Gando, S., Tedo, I., and Kubota, M. (1992). Posttrauma coagulation and fibrinolysis. Crit. care Med. 20 (5), 594–600. doi:10.1097/00003246-199205000-00009
Gando, S., Wada, H., Thachil, J., ScientificStandardization Committee on, D. I. C. o. t. I. S. o. T., and Haemostasis, (2013). Differentiating disseminated intravascular coagulation (DIC) with the fibrinolytic phenotype from coagulopathy of trauma and acute coagulopathy of trauma-shock (COT/ACOTS). J. Thromb. Haemost. 11 (5), 826–835. doi:10.1111/jth.12190
Gando, S., and Wada, T. (2019). Disseminated intravascular coagulation in cardiac arrest and resuscitation. J. Thrombosis Haemostasis 17 (8), 1205–1216. doi:10.1111/jth.14480
Geppert, A., Zorn, G., Delle-Karth, G., Heinz, G., Maurer, G., Siostrzonek, P., et al. (2001). Plasminogen activator inhibitor type 1 and outcome after successful cardiopulmonary resuscitation. Crit. Care Med. 29 (9), 1670–1677. doi:10.1097/00003246-200109000-00003
Gillissen, A., van den Akker, T., Caram-Deelder, C., Henriquez, D. D., Bloemenkamp, K. W., Eikenboom, J., et al. (2019). Comparison of thromboelastometry by ROTEM® Delta and ROTEM® Sigma in women with postpartum haemorrhage. Scand. J. Clin. Lab. Invest 79 (1-2), 32–38. doi:10.1080/00365513.2019.1571220
Girard, T. J., and Broze, G. J. (1993). Tissue factor pathway inhibitor. Methods Enzymol. 222, 195–209. doi:10.1016/0076-6879(93)22014-7
Goncharov, N. V., Nadeev, A. D., Jenkins, R. O., and Avdonin, P. V. (2017). Markers and biomarkers of endothelium: When something is rotten in the state. Oxidative Med. Cell. Longev. 2017, 9759735. doi:10.1155/2017/9759735
Görlinger, K., Dirkmann, D., and Hanke, A. A. (2021). “Rotational thromboelastometry (ROTEM®),” in Trauma induced coagulopathy. Editors H. B. Moore, E. E. Moore, and M. D Neal. Second ed (Switzerland: Spring Nature), 279–312.
Görlinger, K., Pérez-Ferrer, A., Dirkmann, D., Saner, F., Maegele, M., Calatayud Á, A. P., et al. (2019). The role of evidence-based algorithms for rotational thromboelastometry-guided bleeding management. Korean J. Anesthesiol. 72 (4), 297–322. doi:10.4097/kja.19169
Groth, C. G., Pechet, L., and Starzl, T. E. (1969). Coagulation during and after orthotopic transplantation of the human liver. Arch. Surg. 98 (1), 31–34. doi:10.1001/archsurg.1969.01340070049006
Gruen, D. S., Brown, J. B., Guyette, F. X., Vodovotz, Y., Johansson, P. I., Stensballe, J., et al. (2020). Prehospital plasma is associated with distinct biomarker expression following injury. JCI insight 5 (8), e135350. doi:10.1172/jci.insight.135350
Guasch, E., and Gilsanz, F. (2016). Massive obstetric hemorrhage: Current approach to management. Med. Intensiva 40 (5), 298–310. doi:10.1016/j.medin.2016.02.010
Gurbel, P. A., Bliden, K. P., Tantry, U. S., Monroe, A. L., Muresan, A. A., Brunner, N. E., et al. (2016). First report of the point-of-care TEG: A technical validation study of the TEG-6S system. Platelets 27 (7), 642–649. doi:10.3109/09537104.2016.1153617
Gurbel, P. A., Bliden, K., Zaman, F., Taheri, H., Ilkhanoff, L., Sandesara, C., et al. (2017). Multicenter observational trial to evaluate TEG6s oral anticoagulant (OAC) assay in apixiban treated patients. Circulation 136 (1), A20555.
Gurney, J. M., Kozar, R. A., and Cancio, L. C. (2019). Plasma for burn shock resuscitation: Is it time to go back to the future? Transfusion 59 (S2), 1578–1586. doi:10.1111/trf.15243
Haas, T., and Faraoni, D. (2020). Viscoelastic testing in pediatric patients. Transfusion 60, S75–S85. doi:10.1111/trf.16076
Haile, W. B., Wu, J., Echeverry, R., Wu, F., An, J., and Yepes, M. (2012). Tissue-type plasminogen activator has a neuroprotective effect in the ischemic brain mediated by neuronal TNF-α. J. Cereb. Blood Flow. Metab. 32 (1), 57–69. doi:10.1038/jcbfm.2011.106
Hans, G. A., and Besser, M. W. (2016). The place of viscoelastic testing in clinical practice. Br. J. Haematol. 173 (1), 37–48. doi:10.1111/bjh.13930
Harnett, M., Hepner, D. L., Datta, S., and Kodali, B. (2005). Effect of amniotic fluid on coagulation and platelet function in pregnancy: An evaluation using thromboelastography. Anaesthesia 60 (11), 1068–1072. doi:10.1111/j.1365-2044.2005.04373.x
Hartmann, J., Murphy, M., and Dias, J. D. (2020). Viscoelastic hemostatic assays: Moving from the laboratory to the site of care-A review of established and emerging technologies. Diagn. (Basel) 10 (2), 118. doi:10.3390/diagnostics10020118
Hartmann, J., and Sikorski, R. A. (2021). “Thromboelastography (TEG® 5000 and TEG® 6s hemostasis analyzers with TEG Manager® software),” in Trauma induced coagulopathy. Editors H. B. Moore, E. E. Moore, and M. D Neal. Second ed (Switzerland: Spring Nature Switzerland AG), 313–331.
Hatton, G. E., Isbell, K. D., Henriksen, H. H., Stensballe, J., Brummerstedt, M., Johansson, P. I., et al. (2021). Endothelial dysfunction is associated with increased incidence, worsened severity, and prolonged duration of acute kidney injury after severe trauma. Shock 55 (3), 311–315. doi:10.1097/shk.0000000000001638
Henriquez, D. D. C. A., Bloemenkamp, K. W. M., and van der Bom, J. G. (2018). Management of postpartum hemorrhage: How to improve maternal outcomes? J. Thromb. Haemost. 8, 1523–1534. doi:10.1111/jth.14200
Herbert, J. P., Guillotte, A. R., Hammer, R. D., and Litofsky, N. S. (2017). Coagulopathy in the setting of mild traumatic brain injury: Truths and consequences. Brain Sci. 7 (7), 92. doi:10.3390/brainsci7070092
Hess, J. R., Brohi, K., Dutton, R. P., Hauser, C. J., Holcomb, J. B., Kluger, Y., et al. (2008). The coagulopathy of trauma: A review of mechanisms. J. Trauma 65 (4), 748–754. doi:10.1097/TA.0b013e3181877a9c
Hijazi, N., Abu Fanne, R., Abramovitch, R., Yarovoi, S., Higazi, M., Abdeen, S., et al. (2015). Endogenous plasminogen activators mediate progressive intracerebral hemorrhage after traumatic brain injury in mice. Blood 125 (16), 2558–2567. doi:10.1182/blood-2014-08-588442
Hoffman, M., and Monroe, D. M. (2009). Tissue factor in brain is not saturated with factor VIIa: Implications for factor VIIa dosing in intracerebral hemorrhage. Stroke 40 (8), 2882–2884. doi:10.1161/STROKEAHA.109.555433
Holcomb, J. B. (2011). A novel and potentially unifying mechanism for shock induced early coagulopathy. Ann. Surg. 254 (2), 201–202. doi:10.1097/SLA.0b013e318226483d
Holcomb, J. B., Minei, K. M., Scerbo, M. L., Radwan, Z. A., Wade, C. E., Kozar, R. A., et al. (2012). Admission rapid thrombelastography can replace conventional coagulation tests in the emergency department: Experience with 1974 consecutive trauma patients. Ann. Surg. 256 (3), 476–486. doi:10.1097/SLA.0b013e3182658180
Hrebinko, K. A., Sperry, J. L., Guyette, F. X., Brown, J. B., Daley, B. J., Miller, R. S., et al. (2021). Evaluating the cost-effectiveness of prehospital plasma transfusion in unstable trauma patients: A secondary analysis of the PAMPer trial. JAMA Surg. 156 (12), 1131–1139. doi:10.1001/jamasurg.2021.4529
Huber, D., Cramer, E. M., Kaufmann, J. E., Meda, P., Massé, J. M., Kruithof, E. K., et al. (2002). Tissue-type plasminogen activator (t-PA) is stored in Weibel-Palade bodies in human endothelial cells both in vitro and in vivo. Blood 99 (10), 3637–3645. doi:10.1182/blood.v99.10.3637
Huebner, B. R., Moore, E. E., Moore, H. B., Stettler, G. R., Nunns, G. R., Lawson, P., et al. (2018). Thrombin provokes degranulation of platelet α-granules leading to the release of active plasminogen activator inhibitor-1 (PAI-1). Shock 50 (6), 671–676. doi:10.1097/shk.0000000000001089
Hunt, B. J., Raza, I., and Brohi, K. (2013). The incidence and magnitude of fibrinolytic activation in trauma patients: A reply to a rebuttal. J. Thromb. Haemost. 11 (7), 1437–1438. doi:10.1111/jth.12296
Hunt, H., Stanworth, S., Curry, N., Woolley, T., Cooper, C., Ukoumunne, O., et al. (2015). Thromboelastography (TEG) and rotational thromboelastometry (ROTEM) for trauma-induced coagulopathy in adult trauma patients with bleeding. Cochrane Database Syst. Rev. 2, CD010438. doi:10.1002/14651858.CD010438.pub2
Hurwich, M., Zimmer, D., Guerra, E., Evans, E., Shire, T., Abernathy, M., et al. (2016). A case of successful thromboelastographic guided resuscitation after postpartum hemorrhage and cardiac arrest. J. Extra-corporeal Technol. 48 (4), 194–197.
Huzar, T. F., Martinez, E., Love, J., George, T. C., Shah, J., Baer, L., et al. (2018). Admission rapid thrombelastography (rTEG®) values predict resuscitation volumes and patient outcomes after thermal injury. J. Burn Care & Res. 39 (3), 345–352. doi:10.1097/BCR.0000000000000593
Iba, T., Arakawa, M., Mochizuki, K., Nishida, O., Wada, H., and Levy, J. H. (2019a). Usefulness of measuring changes in SOFA score for the prediction of 28-day mortality in patients with sepsis-associated disseminated intravascular coagulation. Clin. Appl. Thromb. Hemost. 25, 1076029618824044. doi:10.1177/1076029618824044
Iba, T., and Levy, J. (2019). Derangement of the endothelial glycocalyx in sepsis. J. Thrombosis Haemostasis 17 (2), 283–294. doi:10.1111/jth.14371
Iba, T., and Levy, J. H. (2020). Sepsis-induced coagulopathy and disseminated intravascular coagulation. Anesthesiology 132 (5), 1238–1245. doi:10.1097/ALN.0000000000003122
Iba, T., Levy, J. H., Warkentin, T. E., Thachil, J., van der Poll, T., Levi, M., et al. (2019b). Diagnosis and management of sepsis-induced coagulopathy and disseminated intravascular coagulation. J. Thromb. Haemost. 17 (11), 1989–1994. doi:10.1111/jth.14578
Iba, T., and Levy, J. (2018). Inflammation and thrombosis: Roles of neutrophils, platelets and endothelial cells and their interactions in thrombus formation during sepsis. J. Thrombosis Haemostasis 16 (2), 231–241. doi:10.1111/jth.13911
Iba, T., and Ogura, H. (2018). Role of extracellular vesicles in the development of sepsis-induced coagulopathy. J. Intensive Care 6 (1), 68–12. doi:10.1186/s40560-018-0340-6
Iba, T., Saito, D., Wada, H., and Asakura, H. (2012). Efficacy and bleeding risk of antithrombin supplementation in septic disseminated intravascular coagulation: A prospective multicenter survey. Thrombosis Res. 130 (3), e129–e133. doi:10.1016/j.thromres.2012.03.021
Jackson-Weaver, O., Friedman, J. K., Rodriguez, L. A., Hoof, M. A., Drury, R. H., Packer, J. T., et al. (2019). Hypoxia/reoxygenation decreases endothelial glycocalyx via reactive oxygen species and calcium signaling in a cellular model for shock. J. Trauma Acute Care Surg. 87 (5), 1070–1076. doi:10.1097/TA.0000000000002427
Jacoby, R. C., Owings, J. T., Holmes, J., Battistella, F. D., Gosselin, R. C., and Paglieroni, T. G. (2001). Platelet activation and function after trauma. J. Trauma Acute Care Surg. 51 (4), 639–647. doi:10.1097/00005373-200110000-00003
Jeppesen, A. N., Hvas, A.-M., Duez, C. H. V., Grejs, A. M., Ilkjær, S., and Kirkegaard, H. (2017). Prolonged targeted temperature management compromises thrombin generation: A randomised clinical trial. Resuscitation 118, 126–132. doi:10.1016/j.resuscitation.2017.06.004
Johansson, P. I., Bro-Jeppesen, J., Kjaergaard, J., Wanscher, M., Hassager, C., and Ostrowski, S. R. (2015). Sympathoadrenal activation and endothelial damage are inter correlated and predict increased mortality in patients resuscitated after out-of-hospital cardiac arrest. a post Hoc sub-study of patients from the TTM-trial. PLoS One 10 (3), e0120914. doi:10.1371/journal.pone.0120914
Johansson, P., Stensballe, J., and Ostrowski, S. (2017a). Shock induced endotheliopathy (SHINE) in acute critical illness-a unifying pathophysiologic mechanism. Crit. Care 21 (1), 25. doi:10.1186/s13054-017-1605-5
Johansson, P. I., Henriksen, H. H., Stensballe, J., Gybel-Brask, M., Cardenas, J. C., Baer, L. A., et al. (2017b). Traumatic endotheliopathy: A prospective observational study of 424 severely injured patients. Ann. Surg. 265 (3), 597–603. doi:10.1097/SLA.0000000000001751
Johansson, P. I., Henriksen, H. H., Stensballe, J., Gybel-Brask, M., Cardenas, J. C., Baer, L. A., et al. (2017c). Traumatic endotheliopathy: A prospective observational study of 424 severely injured patients. Ann. Surg. 265 (3), 597–603. doi:10.1097/sla.0000000000001751
Johansson, P. I., and Ostrowski, S. R. (2010). Acute coagulopathy of trauma: Balancing progressive catecholamine induced endothelial activation and damage by fluid phase anticoagulation. Med. Hypotheses 75 (6), 564–567. doi:10.1016/j.mehy.2010.07.031
Johansson, P. I., Sorensen, A. M., Perner, A., Welling, K. L., Wanscher, M., Larsen, C. F., et al. (2011a). Disseminated intravascular coagulation or acute coagulopathy of trauma shock early after trauma? An observational study. Crit. Care 15 (6), R272. doi:10.1186/cc10553
Johansson, P. I., Stensballe, J., Rasmussen, L. S., and Ostrowski, S. R. (2011b). A high admission syndecan-1 level, a marker of endothelial glycocalyx degradation, is associated with inflammation, protein C depletion, fibrinolysis, and increased mortality in trauma patients. Ann. Surg. 254 (2), 194–200. doi:10.1097/SLA.0b013e318226113d
Johansson, P. I., Stensballe, J., Rasmussen, L. S., and Ostrowski, S. R. (2012). High circulating adrenaline levels at admission predict increased mortality after trauma. J. Trauma Acute Care Surg. 72 (2), 428–436. doi:10.1097/ta.0b013e31821e0f93
Johansson, P., Ostrowski, S., and Secher, N. (2010). Management of major blood loss: An update. Acta Anaesthesiol. Scand. 54 (9), 1039–1049. doi:10.1111/j.1399-6576.2010.02265.x
Ju, R., Zhuang, Z. W., Zhang, J., Lanahan, A. A., Kyriakides, T., Sessa, W. C., et al. (2014). Angiopoietin-2 secretion by endothelial cell exosomes: Regulation by the phosphatidylinositol 3-kinase (PI3K)/Akt/endothelial nitric oxide synthase (eNOS) and syndecan-4/syntenin pathways. J. Biol. Chem. 289 (1), 510–519. doi:10.1074/jbc.M113.506899
Jung, C., Fuernau, G., Muench, P., Desch, S., Eitel, I., Schuler, G., et al. (2015). Impairment of the endothelial glycocalyx in cardiogenic shock and its prognostic relevance. Shock 43 (5), 450–455. doi:10.1097/shk.0000000000000329
Kang, A. M., and Fisher, E. S. (2020). Thromboelastography with platelet studies (TEG® with PlateletMapping®) after rattlesnake envenomation in the southwestern United States demonstrates inhibition of ADP-induced platelet activation as well as clot lysis. J. Med. Toxicol. 16 (1), 24–32. doi:10.1007/s13181-019-00729-8
Kang, Y. G., Martin, D. J., Marquez, J., Lewis, J. H., Bontempo, F. A., Shaw, B. W., et al. (1985). Intraoperative changes in blood coagulation and thrombelastographic monitoring in liver transplantation. Anesth. Analg. 64 (9), 888–896. doi:10.1213/00000539-198509000-00008
Kaplanski, G., Marin, V. r., Fabrigoule, M., Boulay, V., Benoliel, A.-M., Bongrand, P., et al. (1998). Thrombin-activated human endothelial cells support monocyte adhesion in vitro following expression of intercellular adhesion molecule-1 (ICAM-1; CD54) and vascular cell adhesion molecule-1 (VCAM-1; CD106). J. Am. Soc. Hematol. 92 (4), 1259–1267. doi:10.1182/blood.v92.4.1259
Kashuk, J. L., Moore, E. E., Millikan, J. S., and Moore, J. B. (1982). Major abdominal vascular trauma--a unified approach. J. trauma 22 (8), 672–679. doi:10.1097/00005373-198208000-00004
Keshari, R. S., Silasi, R., Popescu, N. I., Georgescu, C., Chaaban, H., Lupu, C., et al. (2020). Fondaparinux pentasaccharide reduces sepsis coagulopathy and promotes survival in the baboon model of Escherichia coli sepsis. J. Thrombosis Haemostasis 18 (1), 180–190. doi:10.1111/jth.14642
Kim, J., Kim, K., Lee, J. H., Jo, Y. H., Kim, T., Rhee, J. E., et al. (2013). Prognostic implication of initial coagulopathy in out-of-hospital cardiac arrest. Resuscitation 84 (1), 48–53. doi:10.1016/j.resuscitation.2012.09.003
Kim, S. M., Kim, S. I., Yu, G., Kim, J. S., Hong, S. I., Chae, B., et al. (2021). Role of thromboelastography in the evaluation of septic shock patients with normal prothrombin time and activated partial thromboplastin time. Sci. Rep. 11 (1), 11833. doi:10.1038/s41598-021-91221-3
Kloner, R. A., King, K. S., and Harrington, M. G. (2018). No-reflow phenomenon in the heart and brain. Am. J. Physiol. Heart Circ. Physiol. 315 (3), H550–h562. doi:10.1152/ajpheart.00183.2018
Kolářová, H., Ambrůzová, B., Švihálková Šindlerová, L., Klinke, A., and Kubala, L. (2014). Modulation of endothelial glycocalyx structure under inflammatory conditions. Mediat. Inflamm. 2014, 694312. doi:10.1155/2014/694312
Kong, D. H., Kim, Y. K., Kim, M. R., Jang, J. H., and Lee, S. (2018). Emerging roles of vascular cell adhesion molecule-1 (VCAM-1) in immunological disorders and cancer. Int. J. Mol. Sci. 19 (4), 1057. doi:10.3390/ijms19041057
Kornblith, L. Z., Moore, H. B., and Cohen, M. J. (2019). Trauma-induced coagulopathy: The past, present, and future. J. Thromb. Haemost. 17 (6), 852–862. doi:10.1111/jth.14450
Kramer, M. S., Berg, C., Abenhaim, H., Dahhou, M., Rouleau, J., Mehrabadi, A., et al. (2013). Incidence, risk factors, and temporal trends in severe postpartum hemorrhage. Am. J. Obstet. Gynecol. 209 (5), 449.e1–449.e7. doi:10.1016/j.ajog.2013.07.007
Kregel, H. R., Hatton, G. E., Isbell, K. D., Henriksen, H. H., Stensballe, J., Johansson, P. I., et al. (2022). Shock-induced endothelial dysfunction is present in patients with occult hypoperfusion after trauma. Shock Inj. Inflamm. Sepsis Laboratory Clin. Approaches 57 (1), 106–112. doi:10.1097/SHK.0000000000001866
Kristová, V., Kriska, M., Canová, R., Hejdová, E., Kobzová, D., and Dobrocký, P. (1993). Endothelial changes following repeated effect of vasoconstrictive substances in vitro. Acta Physiol. Hung 81 (4), 363–370.
Kuczyński, J., Uszyński, W., Zekanowska, E., Soszka, T., and Uszyński, M. (2002). Tissue factor (TF) and tissue factor pathway inhibitor (TFPI) in the placenta and myometrium. Eur. J. Obstet. Gynecol. Reprod. Biol. 105 (1), 15–19. doi:10.1016/s0301-2115(02)00113-6
Kurland, D., Hong, C., Aarabi, B., Gerzanich, V., and Simard, J. M. (2012). Hemorrhagic progression of a contusion after traumatic brain injury: A review. J. neurotrauma 29 (1), 19–31. doi:10.1089/neu.2011.2122
Kutcher, M. E., Redick, B. J., McCreery, R. C., Crane, I. M., Greenberg, M. D., Cachola, L. M., et al. (2012). Characterization of platelet dysfunction after trauma. J. trauma acute care Surg. 73 (1), 13–19. doi:10.1097/TA.0b013e318256deab
Kwaan, H. C., and Cull, E. H. (2014). The coagulopathy in acute promyelocytic leukaemia–what have we learned in the past twenty years. Best. Pract. Res. Clin. Haematol. 27 (1), 11–18. doi:10.1016/j.beha.2014.04.005
Kwaan, H. C. (2014). From fibrinolysis to the plasminogen-plasmin system and beyond: A remarkable growth of knowledge, with personal observations on the history of fibrinolysis. Semin. Thromb. Hemost. 40 (5), 585–591. doi:10.1055/s-0034-1383545
Kwaan, H. C., Rego, E. M., McMahon, B., Weiss, I., and Marvin, J. (2011). Microparticles in acute promyelocytic leukemia. Blood 118 (21), 3346. doi:10.1182/blood.v118.21.3346.3346
Kwaan, H. C., and Rego, E. M. (2010). Role of microparticles in the hemostatic dysfunction in acute promyelocytic leukemia. Semin. Thromb. Hemost. 36 (8), 917–924. doi:10.1055/s-0030-1267045
Laroche, M., Kutcher, M. E., Huang, M. C., Cohen, M. J., and Manley, G. T. (2012). Coagulopathy after traumatic brain injury. Neurosurgery 70 (6), 1334–1345. doi:10.1227/NEU.0b013e31824d179b
Larsen, O. H., Fenger-Eriksen, C., Ingerslev, J., and Sørensen, B. (2012). Improved point-of-care identification of hyperfibrinolysis is needed. Thromb. Res. 130 (4), 690–691. doi:10.1016/j.thromres.2012.08.291
Leeper, C. M., Neal, M. D., McKenna, C. J., and Gaines, B. A. (2017). Trending fibrinolytic dysregulation: Fibrinolysis shutdown in the days after injury is associated with poor outcome in severely injured children. Ann. Surg. 266 (3), 508–515. doi:10.1097/SLA.0000000000002355
Leffers, P., Ferreira, J., Sollee, D., and Schauben, J. (2018). Thromboelastography in the management of snakebite-induced coagulopathy: A case series and literature review. Blood Coagulation Fibrinolysis 29 (7), 656–660. doi:10.1097/MBC.0000000000000771
Leligdowicz, A., Richard-Greenblatt, M., Wright, J., Crowley, V. M., and Kain, K. C. (2018). Endothelial activation: The ang/tie Axis in sepsis. Front. Immunol. 9, 838. doi:10.3389/fimmu.2018.00838
Letson, H. L., and Dobson, G. P. (2017). Tranexamic acid for post-partum haemorrhage in the WOMAN trial. Lancet 390 (10102), 1581–1582. doi:10.1016/S0140-6736(17)31947-5
Leurs, J., and Hendriks, D. (2005). Carboxypeptidase U (TAFIa): A metallocarboxypeptidase with a distinct role in haemostasis and a possible risk factor for thrombotic disease. Thrombosis haemostasis 94 (09), 471–487. doi:10.1160/TH04-07-0454
Levi, M., and van der Poll, T. (2017). Coagulation and sepsis. Thromb. Res. 149, 38–44. doi:10.1016/j.thromres.2016.11.007
Levi, M., van der Poll, T., and Schultz, M. (2012). Systemic versus localized coagulation activation contributing to organ failure in critically ill patients. Seminars Immunopathol. 34 (1), 167–179. doi:10.1007/s00281-011-0283-7
Levi, M., and van der Poll, T. (2008). The role of natural anticoagulants in the pathogenesis and management of systemic activation of coagulation and inflammation in critically ill patients. Seminars thrombosis hemostasis 34 (05), 459–468. doi:10.1055/s-0028-1092876
Levin, A. I., Heine, A. M., Coetzee, J. F., and Coetzee, A. (2014). Heparinase thromboelastography compared with activated coagulation time for protamine titration after cardiopulmonary bypass. J. Cardiothorac. Vasc. Anesth. 28 (2), 224–229. doi:10.1053/j.jvca.2013.10.026
Levy, J. H., Sniecinski, R. M., Welsby, I. J., and Levi, M. (2016). Antithrombin: Anti-inflammatory properties and clinical applications. Thrombosis haemostasis 115 (04), 712–728. doi:10.1160/TH15-08-0687
Liaw, P. C., Ito, T., Iba, T., Thachil, J., and Zeerleder, S. (2016). DAMP and DIC: The role of extracellular DNA and DNA-binding proteins in the pathogenesis of DIC. Blood Rev. 30 (4), 257–261. doi:10.1016/j.blre.2015.12.004
Liew-Spilger, A. E., Sorg, N. R., Brenner, T. J., Langford, J. H., Berquist, M., Mark, N. M., et al. (2021). Viscoelastic hemostatic assays for postpartum hemorrhage. J. Clin. Med. 10 (17), 3946. doi:10.3390/jcm10173946
Lindahl, U., Kusche-Gullberg, M., and Kjellén, L. (1998). Regulated diversity of heparan sulfate. J. Biol. Chem. 273 (39), 24979–24982. doi:10.1074/jbc.273.39.24979
Liu, Y., Wang, Z., Jiang, M., Dai, L., Zhang, W., Wu, D., et al. (2011). The expression of annexin II and its role in the fibrinolytic activity in acute promyelocytic leukemia. Leuk. Res. 35 (7), 879–884. doi:10.1016/j.leukres.2010.11.008
Lord, S. T. (2011). Molecular mechanisms affecting fibrin structure and stability. Arteriosclerosis, thrombosis, Vasc. Biol. 31 (3), 494–499. doi:10.1161/ATVBAHA.110.213389
Loughran, J. A., Kitchen, T. L., Sindhakar, S., Ashraf, M., Awad, M., and Kealaher, E. J. (2019). Rotational thromboelastometry (ROTEM®)-guided diagnosis and management of amniotic fluid embolism. Int. J. Obstet. Anesth. 38, 127–130. doi:10.1016/j.ijoa.2018.09.001
Luddington, R. J. (2005). Thrombelastography/thromboelastometry. Clin. Lab. Haematol. 27 (2), 81–90. doi:10.1111/j.1365-2257.2005.00681.x
Lustenberger, T., Talving, P., Kobayashi, L., Barmparas, G., Inaba, K., Lam, L., et al. (2010). Early coagulopathy after isolated severe traumatic brain injury: Relationship with hypoperfusion challenged. J. Trauma Acute Care Surg. 69 (6), 1410–1414. doi:10.1097/TA.0b013e3181cdae81
Macko, A. R., Moore, H. B., Cap, A. P., Meledeo, M. A., Moore, E. E., and Sheppard, F. R. (2017). Tissue injury suppresses fibrinolysis after hemorrhagic shock in nonhuman primates (rhesus macaque). J. trauma acute care Surg. 82 (4), 750–757. doi:10.1097/TA.0000000000001379
Maegele, M., Aversa, J., Marsee, M. K., McCauley, R., Chitta, S. H., Vyakaranam, S., et al. (2020). Changes in coagulation following brain injury. Seminars thrombosis hemostasis 46 (02), 155–166. doi:10.1055/s-0040-1702178
Maegele, M., Schöchl, H., Menovsky, T., Maréchal, H., Marklund, N., Buki, A., et al. (2017). Coagulopathy and haemorrhagic progression in traumatic brain injury: Advances in mechanisms, diagnosis, and management. Lancet Neurology 16 (8), 630–647. doi:10.1016/S1474-4422(17)30197-7
Mahmoud, M., Mayer, M., Cancel, L. M., Bartosch, A. M., Mathews, R., and Tarbell, J. M. (2021). The Glycocalyx core protein Glypican 1 protects vessel wall endothelial cells from stiffness-mediated dysfunction and disease. Cardiovasc. Res. 117 (6), 1592–1605. doi:10.1093/cvr/cvaa201
Makhmudov, R., YaD, M., Dolgov, V., and Repin, V. (1985). Catecholamine-mediated injury to endothelium in rabbit perfused aorta: A quantitative analysis by scanning electron microscopy. Cor Vasa 27 (6), 456–463.
Mamczak, C. N., Speybroeck, J., Stillson, J. E., Dynako, J., Piscoya, A., Peck, E. E., et al. (2022). Viscoelastic hemostatic assays for orthopedic trauma and elective procedures. J. Clin. Med. 11 (14), 4029. doi:10.3390/jcm11144029
Marar, T. T., and Boffa, M. B. (2016). Identification of a thrombomodulin interaction site on thrombin-activatable fibrinolysis inhibitor that mediates accelerated activation by thrombin. J. Thromb. Haemost. 14 (4), 772–783. doi:10.1111/jth.13275
Marcus, A. J., Safier, L. B., Hajjar, K. A., Ullman, H. L., Islam, N., Broekman, M. J., et al. (1991). Inhibition of platelet function by an aspirin-insensitive endothelial cell ADPase. Thromboregulation by endothelial cells. J. Clin. Invest 88 (5), 1690–1696. doi:10.1172/jci115485
Marsden, N. J., Van, M., Dean, S., Azzopardi, E. A., Hemington-Gorse, S., Evans, P. A., et al. (2017). Measuring coagulation in burns: An evidence-based systematic review. Scars, burns Heal. 3, 2059513117728201. doi:10.1177/2059513117728201
Martínez, J. T., Petrone, P., Axelrad, A., and Marini, C. P. (2018). Comparison between thromboelastography and conventional coagulation test: Should we abandon conventional coagulation tests in polytrauma patients? Cirugía Española Engl. Ed. 96 (7), 443–449. doi:10.1016/j.ciresp.2018.04.003
Martini, W. Z., Holcomb, J. B., Yu, Y.-M., Wolf, S. E., Cancio, L. C., Pusateri, A. E., et al. (2020). Hypercoagulation and hypermetabolism of fibrinogen in severely burned adults. J. Burn Care & Res. 41 (1), 23–29. doi:10.1093/jbcr/irz147
Maslove, D. M., Lamontagne, F., Marshall, J. C., and Heyland, D. K. (2017). A path to precision in the ICU. Crit. Care 21 (1), 79–9. doi:10.1186/s13054-017-1653-x
Mast, A. E. (2016). Tissue factor pathway inhibitor: Multiple anticoagulant activities for a single protein. Arterioscler. Thromb. Vasc. Biol. 36 (1), 9–14. doi:10.1161/atvbaha.115.305996
Matsushita, K., Morrell, C. N., Cambien, B., Yang, S. X., Yamakuchi, M., Bao, C., et al. (2003). Nitric oxide regulates exocytosis by S-nitrosylation of N-ethylmaleimide-sensitive factor. Cell 115 (2), 139–150. doi:10.1016/s0092-8674(03)00803-1
Maybury, H., Waugh, J., Gornall, A., and Pavord, S. (2008). There is a return to non-pregnant coagulation parameters after four not six weeks postpartum following spontaneous vaginal delivery. Obstet. Med. 1 (2), 92–94. doi:10.1258/om.2008.080025
McCormack, J. J., Lopes da Silva, M., Ferraro, F., Patella, F., and Cutler, D. F. (2017). Weibel-Palade bodies at a glance. J. Cell Sci. 130 (21), 3611–3617. doi:10.1242/jcs.208033
McDonnell, N. J., and Browning, R. (2018). How to replace fibrinogen in postpartum haemorrhage situations? (Hint: Don't use FFP!). Int. J. Obstet. Anesth. 33, 4–7. doi:10.1016/j.ijoa.2017.08.008
McKinley, T. O., Lisboa, F. A., Horan, A. D., Gaski, G. E., and Mehta, S. (2019). Precision medicine applications to manage multiply injured patients with orthopaedic trauma. J. Orthop. trauma 33, S25–S29. doi:10.1097/BOT.0000000000001468
McNamara, H., Mallaiah, S., Barclay, P., Chevannes, C., and Bhalla, A. (2015). Coagulopathy and placental abruption: Changing management with ROTEM-guided fibrinogen concentrate therapy. Int. J. Obstet. Anesth. 24 (2), 174–179. doi:10.1016/j.ijoa.2014.12.005
Medcalf, R. L. (2015). The traumatic side of fibrinolysis. J. Am. Soc. Hematol. 125 (16), 2457–2458. doi:10.1182/blood-2015-02-629808
Meizoso, J. P., Karcutskie, C. A., Ray, J. J., Namias, N., Schulman, C. I., and Proctor, K. G. (2017). Persistent fibrinolysis shutdown is associated with increased mortality in severely injured trauma patients. J. Am. Coll. Surg. 224 (4), 575–582. doi:10.1016/j.jamcollsurg.2016.12.018
Mellion, B. T., Ignarro, L. J., Ohlstein, E. H., Pontecorvo, E. G., Hyman, A. L., and Kadowitz, P. J. (1981). Evidence for the inhibitory role of guanosine 3′, 5′-monophosphate in ADP-induced human platelet aggregation in the presence of nitric oxide and related vasodilators. Blood 57 (5), 946–955. doi:10.1182/blood.v57.5.946.bloodjournal575946
Millham, F. H. (2010). A brief history of shock. Surgery 148 (5), 1026–1037. doi:10.1016/j.surg.2010.02.014
Mitrophanov, A. Y., Rosendaal, F. R., and Reifman, J. (2014). Computational analysis of the effects of reduced temperature on thrombin generation: The contributions of hypothermia to coagulopathy. Surv. Anesthesiol. 58 (3), 151. doi:10.1097/sa.0000000000000056
Mochizuki, K., Mori, K., Nakamura, Y., Uchimido, R., Kamijo, H., Takeshige, K., et al. (2018). Early changes in the sequential organ failure assessment score among patients with sepsis-induced disseminated intravascular coagulation. Clin. Appl. Thromb. Hemost. 24 (9), 332s–339s. doi:10.1177/1076029618814346
Moore, E. E., Moore, H. B., Gonzalez, E., Chapman, M. P., Hansen, K. C., Sauaia, A., et al. (2015a). Postinjury fibrinolysis shutdown: Rationale for selective tranexamic acid. J. Trauma Acute Care Surg. 78 (6), S65–S69. doi:10.1097/TA.0000000000000634
Moore, H. B., Moore, E. E., Lawson, P. J., Gonzalez, E., Fragoso, M., Morton, A. P., et al. (2015b). Fibrinolysis shutdown phenotype masks changes in rodent coagulation in tissue injury versus hemorrhagic shock. Surgery 158 (2), 386–392. doi:10.1016/j.surg.2015.04.008
Moore, E. E., Moore, H. B., Gonzalez, E., Sauaia, A., Banerjee, A., and Silliman, C. C. (2016a). Rationale for the selective administration of tranexamic acid to inhibit fibrinolysis in the severely injured patient. Transfusion 56 (22), S110–S114. doi:10.1111/trf.13486
Moore, H. B., Moore, E. E., Liras, I. N., Gonzalez, E., Harvin, J. A., Holcomb, J. B., et al. (2016b). Acute fibrinolysis shutdown after injury occurs frequently and increases mortality: A multicenter evaluation of 2,540 severely injured patients. J. Am. Coll. Surg. 222 (4), 347–355. doi:10.1016/j.jamcollsurg.2016.01.006
Moore, E. E., Moore, H. B., Kornblith, L. Z., Neal, M. D., Hoffman, M., Mutch, N. J., et al. (2021a). Trauma-induced coagulopathy. Nat. Rev. Dis. Prim. 7 (1), 30–23. doi:10.1038/s41572-021-00264-3
Moore, H. B., Moore, E. E., and Neal, M. D. (2021b). Trauma induced coagulopathy. Switzerland: Spring Nature Switzerland AG.
Moore, H. B. (2022). Fibrinolysis shutdown and hypofibrinolysis are not synonymous terms: The clinical significance of differentiating low fibrinolytic states. Semin. Thromb. Hemost. doi:10.1055/s-0042-1758057
Moore, H. B., Gando, S., Iba, T., Kim, P. Y., Yeh, C. H., Brohi, K., et al. (2020). Defining trauma-induced coagulopathy with respect to future implications for patient management: Communication from the SSC of the ISTH. J. Thrombosis Haemostasis 18 (3), 740–747. doi:10.1111/jth.14690
Moore, H. B., Moore, E. E., Chapman, M. P., Hansen, K., Cohen, M. J., Pieracci, F. M., et al. (2019a). Does tranexamic acid improve clot strength in severely injured patients who have elevated fibrin degradation products and low fibrinolytic activity, measured by thrombelastography? J. Am. Coll. Surg. 229, 92–101. doi:10.1016/j.jamcollsurg.2019.03.015
Moore, H. B., Moore, E. E., Neal, M. D., Sheppard, F. R., Kornblith, L. Z., Draxler, D. F., et al. (2019b). Fibrinolysis shutdown in trauma: Historical review and clinical implications. Anesth. Analg. 129 (3), 762–773. doi:10.1213/ANE.0000000000004234
Moore, H. B., Moore, E. E., Chapman, M. P., McVaney, K., Bryskiewicz, G., Blechar, R., et al. (2018). Plasma-first resuscitation to treat haemorrhagic shock during emergency ground transportation in an urban area: A randomised trial. Lancet 392 (10144), 283–291. doi:10.1016/S0140-6736(18)31553-8
Moore, H. B., Moore, E. E., Huebner, B. R., Stettler, G. R., Nunns, G. R., Einersen, P. M., et al. (2017). Tranexamic acid is associated with increased mortality in patients with physiological fibrinolysis. J. Surg. Res. 220, 438–443. doi:10.1016/j.jss.2017.04.028
Moore, H., Moore, E., Gonzalez, E., Chapman, M., Chin, T., Silliman, C., et al. (2014). Hyperfibrinolysis, physiologic fibrinolysis, and fibrinolysis shutdown: The spectrum of postinjury fibrinolysis and relevance to antifibrinolytic therapy. J. Trauma Acute Care Surg. 77 (6), 811–817. doi:10.1097/TA.0000000000000341
Müller, M. C., Meijers, J. C., Vroom, M. B., and Juffermans, N. P. (2014). Utility of thromboelastography and/or thromboelastometry in adults with sepsis: A systematic review. Crit. Care 18 (1), R30. doi:10.1186/cc13721
Mullins, B., Akehurst, H., Slattery, D., and Chesser, T. (2018). Should surgery be delayed in patients taking direct oral anticoagulants who suffer a hip fracture? A retrospective, case-controlled observational study at a UK major trauma centre. BMJ Open 8 (4), e020625. doi:10.1136/bmjopen-2017-020625
Napolitano, L. M. (2021). Hemostatic defects in massive transfusion: An update and treatment recommendations. Expert Rev. Hematol. 14 (2), 219–239. doi:10.1080/17474086.2021.1858788
Nekludov, M., Bellander, B.-M., Blombäck, M., and Wallen, H. N. (2007). Platelet dysfunction in patients with severe traumatic brain injury. J. Neurotrauma 24 (11), 1699–1706. doi:10.1089/neu.2007.0322
Nekludov, M., Mobarrez, F., Gryth, D., Bellander, B.-M., and Wallen, H. (2014). Formation of microparticles in the injured brain of patients with severe isolated traumatic brain injury. J. Neurotrauma 31 (23), 1927–1933. doi:10.1089/neu.2013.3168
Nelson, A., Berkestedt, I., and Bodelsson, M. (2014). Circulating glycosaminoglycan species in septic shock. Acta Anaesthesiol. Scand. 58 (1), 36–43. doi:10.1111/aas.12223
Nelson, A., Berkestedt, I., Schmidtchen, A., Ljunggren, L., and Bodelsson, M. (2008). Increased levels of glycosaminoglycans during septic shock: Relation to mortality and the antibacterial actions of plasma. Shock 30 (6), 623–627. doi:10.1097/SHK.0b013e3181777da3
Neubauer, K., and Zieger, B. (2022). Endothelial cells and coagulation. Cell Tissue Res. 387 (3), 391–398. doi:10.1007/s00441-021-03471-2
Neumar, R. W., Nolan, J. P., Adrie, C., Aibiki, M., Berg, R. A., Böttiger, B. W., et al. (2008). Post–cardiac arrest syndrome: Epidemiology, pathophysiology, treatment, and prognostication a consensus statement from the international liaison committee on resuscitation (American heart association, Australian and New Zealand council on resuscitation, European resuscitation council, heart and stroke foundation of Canada, InterAmerican heart foundation, resuscitation council of asia, and the resuscitation council of southern africa); the American heart association emergency cardiovascular care committee; the council on cardiovascular surgery and anesthesia; the council on cardiopulmonary, perioperative, and critical care; the council on clinical cardiology; and the stroke council. Circulation 118 (23), 2452–2483. doi:10.1161/CIRCULATIONAHA.108.190652
O'Rourke, J., Jiang, X., Hao, Z., Cone, R. E., and Hand, A. R. (2005). Distribution of sympathetic tissue plasminogen activator (tPA) to a distant microvasculature. J. Neurosci. Res. 79 (6), 727–733. doi:10.1002/jnr.20366
Oberladstätter, D., Schlimp, C. J., Zipperle, J., Osuchowski, M. F., Voelckel, W., Grottke, O., et al. (2021). Impact of idarucizumab and andexanet alfa on DOAC plasma concentration and ClotPro(®) clotting time: An ex vivo spiking study in A cohort of trauma patients. J. Clin. Med. 10 (16), 3476. doi:10.3390/jcm10163476
Ofosu, F. A., Modi, G. J., Smith, L. M., Cerskus, A. L., Hirsh, J., and Blajchman, M. A. (1984). Heparan sulfate and dermatan sulfate inhibit the generation of thrombin activity in plasma by complementary pathways. Blood 64 (3), 742–747. doi:10.1182/blood.v64.3.742.bloodjournal643742
Ojito, J. W., Hannan, R. L., Burgos, M. M., Lim, H., Huynh, M., Velis, E., et al. (2012). Comparison of point-of-care activated clotting time systems utilized in a single pediatric institution. J. Extra Corpor. Technol. 44 (1), 15–20.
Opal, S., and Van Der Poll, T. (2015). Endothelial barrier dysfunction in septic shock. J. Intern. Med. 277 (3), 277–293. doi:10.1111/joim.12331
Osman, M., Syed, M., Patibandla, S., Sulaiman, S., Kheiri, B., Shah, M. K., et al. (2021). Fifteen-year trends in incidence of cardiogenic shock hospitalization and in-hospital mortality in the United States. J. Am. Heart Assoc. 10 (15), e021061. doi:10.1161/jaha.121.021061
Østerud, B., and Bjørklid, E. (2001). The tissue factor pathway in disseminated intravascular coagulation. Seminars thrombosis hemostasis 27 (06), 605–617. doi:10.1055/s-2001-18866
Ostrowski, S. R., Haase, N., Müller, R. B., Møller, M. H., Pott, F. C., Perner, A., et al. (2015). Association between biomarkers of endothelial injury and hypocoagulability in patients with severe sepsis: A prospective study. Crit. Care 19 (1), 191. doi:10.1186/s13054-015-0918-5
Ostrowski, S. R., and Johansson, P. I. (2012). Endothelial glycocalyx degradation induces endogenous heparinization in patients with severe injury and early traumatic coagulopathy. J. Trauma Acute Care Surg. 73 (1), 60–66. doi:10.1097/TA.0b013e31825b5c10
Othman, M., Han, K., Elbatarny, M., and Abdul-Kadir, R. (2019). The use of viscoelastic hemostatic tests in pregnancy and puerperium: Review of the current evidence-communication from the women's health SSC of the ISTH. J. Thromb. Haemost. 17, 1184–1189. doi:10.1111/jth.14461
Owings, J. T., Bagley, M., Gosselin, R., Romac, D., and Disbrow, E. (1996). Effect of critical injury on plasma antithrombin activity: Low antithrombin levels are associated with thromboembolic complications. J. Trauma Acute Care Surg. 41 (3), 396–405; discussion 405-406. doi:10.1097/00005373-199609000-00004
Pacheco, L. D., Saade, G., Hankins, G. D., Clark, S. L., and Medicine, S. f. M.-F. (2016). Amniotic fluid embolism: Diagnosis and management. Am. J. Obstetrics Gynecol. 215 (2), B16–B24. doi:10.1016/j.ajog.2016.03.012
Pailleret, C., Jourdi, G., Siguret, V., Gouin-Thibault, I., Gandrille, S., Stepanian, A., et al. (2019). Modified ROTEM for the detection of rivaroxaban and apixaban anticoagulant activity in whole blood: A diagnostic test study. Eur. J. Anaesthesiol. 36 (6), 449–456. doi:10.1097/EJA.0000000000000903
Paoli, C. J., Reynolds, M. A., Sinha, M., Gitlin, M., and Crouser, E. (2018). Epidemiology and costs of sepsis in the United States-an analysis based on timing of diagnosis and severity level. Crit. Care Med. 46 (12), 1889–1897. doi:10.1097/ccm.0000000000003342
Pape, T., Hunkemöller, A. M., Kümpers, P., Haller, H., David, S., and Stahl, K. (2021). Targeting the "sweet spot" in septic shock - a perspective on the endothelial glycocalyx regulating proteins Heparanase-1 and -2. Matrix Biol. Plus 12, 100095. doi:10.1016/j.mbplus.2021.100095
Pardridge, W. M. (2002). Drug and gene targeting to the brain with molecular Trojan horses. Nat. Rev. Drug Discov. 1 (2), 131–139. doi:10.1038/nrd725
Park, E. J., Choi, S., Kim, H.-H., and Jung, Y. S. (2020). Novel treatment strategy for patients with venom-induced consumptive coagulopathy from a pit viper bite. Toxins 12 (5), 295. doi:10.3390/toxins12050295
Park, J., Piknova, B., Nghiem, K., Lozier, J., and Schechter, A. (2014). Inhibitory effect of nitrite on coagulation processes demonstrated by thrombelastography. Nitric Oxide 40, 45–51. doi:10.1016/j.niox.2014.05.006
Pavoni, V., Gianesello, L., Conti, D., Ballo, P., Dattolo, P., Prisco, D., et al. (2022). In less than No time”: Feasibility of rotational thromboelastometry to detect anticoagulant drugs activity and to guide reversal therapy. J. Clin. Med. 11 (5), 1407. doi:10.3390/jcm11051407
Pavoni, V., Gianesello, L., Pazzi, M., Stera, C., Meconi, T., and Frigieri, F. C. (2020). Evaluation of coagulation function by rotation thromboelastometry in critically ill patients with severe COVID-19 pneumonia. J. Thromb. Thrombolysis 50 (2), 281–286. doi:10.1007/s11239-020-02130-7
Penc, S. F., Pomahac, B., Eriksson, E., Detmar, M., and Gallo, R. L. (1999). Dermatan sulfate activates nuclear factor-kappab and induces endothelial and circulating intercellular adhesion molecule-1. J. Clin. Invest 103 (9), 1329–1335. doi:10.1172/jci4742
Penc, S. F., Pomahac, B., Winkler, T., Dorschner, R. A., Eriksson, E., Herndon, M., et al. (1998). Dermatan sulfate released after injury is a potent promoter of fibroblast growth factor-2 function. J. Biol. Chem. 273 (43), 28116–28121. doi:10.1074/jbc.273.43.28116
Peng, J., Zhang, L., Drysdale, L., and Fong, G. H. (2000). The transcription factor EPAS-1/hypoxia-inducible factor 2alpha plays an important role in vascular remodeling. Proc. Natl. Acad. Sci. U. S. A. 97 (15), 8386–8391. doi:10.1073/pnas.140087397
Pinsky, D. J., Naka, Y., Liao, H., Oz, M. C., Wagner, D. D., Mayadas, T. N., et al. (1996). Hypoxia-induced exocytosis of endothelial cell Weibel-Palade bodies. A mechanism for rapid neutrophil recruitment after cardiac preservation. J. Clin. Invest 97 (2), 493–500. doi:10.1172/jci118440
Presnyakova, M., Zagrekov, V., Kostina, O., Pushkin, A. S., Kuznetsova, V., and Arefyev, I. Y. (2021). The effect of hyperoxia on the hemostasiological status of severely burned patients. Klin. Lab. Diagn. 66 (11), 666–672. doi:10.51620/0869-2084-2021-66-11-666-672
Puchwein-Schwepcke, A., Genzel-Boroviczény, O., and Nussbaum, C. (2021). The endothelial glycocalyx: Physiology and pathology in neonates, infants and children. Front. Cell Dev. Biol. 9, 733557. doi:10.3389/fcell.2021.733557
Pusateri, A. E., Moore, E. E., Moore, H. B., Le, T. D., Guyette, F. X., Chapman, M. P., et al. (2020). Association of prehospital plasma transfusion with survival in trauma patients with hemorrhagic shock when transport times are longer than 20 minutes: A post hoc analysis of the PAMPer and COMBAT clinical trials. JAMA Surg. 155 (2), e195085. doi:10.1001/jamasurg.2019.5085
Radomski, M. W., Palmer, R. M., and Moncada, S. (1990). An L-arginine/nitric oxide pathway present in human platelets regulates aggregation. Proc. Natl. Acad. Sci. U. S. A. 87 (13), 5193–5197. doi:10.1073/pnas.87.13.5193
Radomski, M. W., Palmer, R. M., and Moncada, S. (1987). Endogenous nitric oxide inhibits human platelet adhesion to vascular endothelium. Lancet 2 (8567), 1057–1058. doi:10.1016/s0140-6736(87)91481-4
Ramos, C. R., Moore, E. E., Manco-Johnson, M. L., Silliman, C. C., Chapman, M. C., and Banerjee, A. (2013). The incidence and magnitude of fibrinolytic activation in trauma patients: A rebuttal. J. Thromb. Haemost. 11 (7), 1435–1437. doi:10.1111/jth.12240
Rayahin, J. E., Buhrman, J. S., Zhang, Y., Koh, T. J., and Gemeinhart, R. A. (2015). High and low molecular weight hyaluronic acid differentially influence macrophage activation. ACS Biomater. Sci. Eng. 1 (7), 481–493. doi:10.1021/acsbiomaterials.5b00181
Raza, I., Davenport, R., Rourke, C., Platton, S., Manson, J., Spoors, C., et al. (2013). The incidence and magnitude of fibrinolytic activation in trauma patients. J. Thromb. Haemost. 11 (2), 307–314. doi:10.1111/jth.12078
Reiterer, M., and Branco, C. M. (2020). Endothelial cells and organ function: Applications and implications of understanding unique and reciprocal remodelling. FEBS J. 287 (6), 1088–1100. doi:10.1111/febs.15143
Reitsma, S., Slaaf, D. W., Vink, H., Van Zandvoort, M. A., and oude Egbrink, M. G. A. (2007). The endothelial glycocalyx: Composition, functions, and visualization. Pflügers Archiv-European J. Physiology 454 (3), 345–359. doi:10.1007/s00424-007-0212-8
Richards, J. E., Harris, T., Dünser, M. W., Bouzat, P., and Gauss, T. (2021). Vasopressors in trauma: A never event? Anesth. Analgesia 133 (1), 68–79. doi:10.1213/ANE.0000000000005552
Richter, R. P., Joiner, D. M., Griffin, R. L., Jansen, J. O., Kerby, J. D., Wade, C. E., et al. (2022a). Endotheliopathy is associated with a 24-hour fibrinolysis phenotype described by low TEG lysis and high D-dimer after trauma: A secondary analysis of the PROPPR study. Ann. Surg. Open 3 (1), e116. doi:10.1097/as9.0000000000000116
Richter, R. P., Payne, G. A., Ambalavanan, N., Gaggar, A., and Richter, J. R. (2022b). The endothelial glycocalyx in critical illness: A pediatric perspective. Matrix Biol. Plus 14, 100106. doi:10.1016/j.mbplus.2022.100106
Richter, R. P., and Richter, J. R. (2019). Glycocalyx gone awry: Pathologic cell signaling during endotheliopathy. Am. J. Biomed. Sci. Res. 5 (2), 118–126. doi:10.34297/ajbsr.2019.05.000891
Roberts, I., Perel, P., Prieto-Merino, D., Shakur, H., Coats, T., Hunt, B. J., et al. (2012). Effect of tranexamic acid on mortality in patients with traumatic bleeding: Prespecified analysis of data from randomised controlled trial. BMJ Br. Med. J. 345, e5839. doi:10.1136/bmj.e5839
Roberts, I., Shakur-Still, H., Afolabi, A., Akere, A., Arribas, M., Brenner, A., et al. (2020). Effects of a high-dose 24-h infusion of tranexamic acid on death and thromboembolic events in patients with acute gastrointestinal bleeding (HALT-IT): An international randomised, double-blind, placebo-controlled trial. Lancet 395 (10241), 1927–1936. doi:10.1016/S0140-6736(20)30848-5
Rosato, F. E., Miller, L. D., and Hebel, M. (1968). A biochemical definition of blood viscosity: Its possible significance in the pathophysiology of shock. Ann. Surg. 168 (1), 61–66. doi:10.1097/00000658-196807000-00007
Rowell, S. E., Meier, E. N., McKnight, B., Kannas, D., May, S., Sheehan, K., et al. (2020). Effect of out-of-hospital tranexamic acid vs placebo on 6-month functional neurologic outcomes in patients with moderate or severe traumatic brain injury. Jama 324 (10), 961–974. doi:10.1001/jama.2020.8958
Saini, A., Spinella, P. C., Ignell, S. P., and Lin, J. C. (2019). Thromboelastography variables, immune markers, and endothelial factors associated with shock and NPMODS in children with severe sepsis. Front. Pediatr. 7, 422. doi:10.3389/fped.2019.00422
Samuels, J. M., Moore, E. E., Silliman, C. C., Banerjee, A., Cohen, M. J., Ghasabyan, A., et al. (2019). Severe traumatic brain injury is associated with a unique coagulopathy phenotype. J. trauma acute care Surg. 86 (4), 686–693. doi:10.1097/TA.0000000000002173
Sankarankutty, A., Nascimento, B., da Luz, L. T., and Rizoli, S. (2012). TEG® and ROTEM® in trauma: Similar test but different results? World J. Emerg. Surg. 7, S3. doi:10.1186/1749-7922-7-S1-S3
Saraiva, I. E., Miranda, P. K., Freitas, J. N., Oliveira, C. R., Ataide, T. L., Nobre, V., et al. (2016). Thromboelastometric evaluation of sepsis associated coagulopathy: A cohort study. Thromb. Res. 147, 124–125. doi:10.1016/j.thromres.2016.10.003
Sarode, R. (2019). Direct oral anticoagulant monitoring: What laboratory tests are available to guide us? Hematol. Am. Soc. Hematol. Educ. Program 2019 (1), 194–197. doi:10.1182/hematology.2019000027
Scarlatescu, E., Lance, M. D., White, N. J., Arama, S. S., and Tomescu, D. R. (2018). Effects of malignancy on blood coagulation in septic intensive care patients. Blood Coagul. Fibrinolysis 29 (1), 92–96. doi:10.1097/MBC.0000000000000680
Scarlatescu, E., White, N. J., and Tomescu, D. R. (2020). Standard and derived rotational thromboelastometry parameters for prediction of disseminated intravascular coagulation in septic patients. Blood Coagul. Fibrinolysis 31 (5), 317–323. doi:10.1097/MBC.0000000000000919
Schenk, B., Görlinger, K., Treml, B., Tauber, H., Fries, D., Niederwanger, C., et al. (2019). A comparison of the new ROTEM® sigma with its predecessor, the ROTEMdelta. Anaesthesia 74 (3), 348–356. doi:10.1111/anae.14542
Schmidt, E. P., Li, G., Li, L., Fu, L., Yang, Y., Overdier, K. H., et al. (2014). The circulating glycosaminoglycan signature of respiratory failure in critically ill adults. J. Biol. Chem. 289 (12), 8194–8202. doi:10.1074/jbc.M113.539452
Schöchl, H., Cadamuro, J., Seidl, S., Franz, A., Solomon, C., Schlimp, C. J., et al. (2013). Hyperfibrinolysis is common in out-of-hospital cardiac arrest: Results from a prospective observational thromboelastometry study. Resuscitation 84 (4), 454–459. doi:10.1016/j.resuscitation.2012.08.318
Schöchl, H., and Schlimp, C. J. (2014). Trauma bleeding management: The concept of goal-directed primary care. Anesth. Analgesia 119 (5), 1064–1073. doi:10.1213/ANE.0b013e318270a6f7
Schöchl, H., Schlimp, C., and Voelckel, W. (2014). Perioperative coagulation management in multiple trauma patients based on viscoelastic test results. Der Unfallchirurg 117 (2), 111–117. doi:10.1007/s00113-013-2490-2
Schrage, B., Becher, P. M., Goßling, A., Savarese, G., Dabboura, S., Yan, I., et al. (2021). Temporal trends in incidence, causes, use of mechanical circulatory support and mortality in cardiogenic shock. Esc. Heart Fail 8 (2), 1295–1303. doi:10.1002/ehf2.13202
Semeraro, N., Ammollo, C. T., Semeraro, F., and Colucci, M. (2012). Sepsis, thrombosis and organ dysfunction. Thromb. Res. 129 (3), 290–295. doi:10.1016/j.thromres.2011.10.013
Shakur, H., Roberts, I., Bautista, R., Caballero, J., Coats, T., Dewan, Y., et al. (2010). Effects of tranexamic acid on death, vascular occlusive events, and blood transfusion in trauma patients with significant haemorrhage (CRASH-2): A randomised, placebo-controlled trial. Lancet 376 (9734), 23–32. doi:10.1016/S0140-6736(10)60835-5
Shore-Lesserson, L., Manspeizer, H. E., DePerio, M., Francis, S., Vela-Cantos, F., and Ergin, M. A. (1999). Thromboelastography-guided transfusion algorithm reduces transfusions in complex cardiac surgery. Anesth. Analg. 88 (2), 312–319. doi:10.1097/00000539-199902000-00016
Sillen, M., and Declerck, P. J. (2020). Targeting PAI-1 in cardiovascular disease: Structural insights into PAI-1 functionality and inhibition. Front. Cardiovasc. Med. 7, 622473. doi:10.3389/fcvm.2020.622473
Sillen, M., and Declerck, P. J. (2021). Thrombin activatable fibrinolysis inhibitor (TAFI): An updated narrative review. Int. J. Mol. Sci. 22 (7), 3670. doi:10.3390/ijms22073670
Silva, M., Thelwell, C., Williams, S., and Longstaff, C. (2012). Regulation of fibrinolysis by C-terminal lysines operates through plasminogen and plasmin but not tissue-type plasminogen activator. J. Thrombosis Haemostasis 10 (11), 2354–2360. doi:10.1111/j.1538-7836.2012.04925.x
Simard, J. M., Kilbourne, M., Tsymbalyuk, O., Tosun, C., Caridi, J., Ivanova, S., et al. (2009). Key role of sulfonylurea receptor 1 in progressive secondary hemorrhage after brain contusion. J. Neurotrauma 26 (12), 2257–2267. doi:10.1089/neu.2009.1021
Simmons, J., and Powell, M. (2016). Acute traumatic coagulopathy: Pathophysiology and resuscitation. BJA Br. J. Anaesth. 117 (3), iii31–iii43. doi:10.1093/bja/aew328
Singh, S., Saleem, S., and Reed, G. L. (2020). Alpha2-antiplasmin: The devil you don't know in cerebrovascular and cardiovascular disease. Front. Cardiovasc. Med. 7, 608899. doi:10.3389/fcvm.2020.608899
Sixta, S. L., Cardenas, J. C., Kitagawa, R., Wade, C. E., Holcomb, J. B., and Cotton, B. A. (2015). Hypocoagulability in traumatic brain injury as measured by traditional means and thrombelastography. J. Neurology Neurophysiology 6 (5), 1–5.
Snegovskikh, D., Souza, D., Walton, Z., Dai, F., Rachler, R., Garay, A., et al. (2018). Point-of-care viscoelastic testing improves the outcome of pregnancies complicated by severe postpartum hemorrhage. J. Clin. Anesth. 44, 50–56. doi:10.1016/j.jclinane.2017.10.003
Solomon, C., Traintinger, S., Ziegler, B., Hanke, A., Rahe-Meyer, N., Voelckel, W., et al. (2011). Platelet function following trauma. A multiple electrode aggregometry study. Thromb. Haemost. 106 (2), 322–330. doi:10.1160/TH11-03-0175
Spalding, G. J., Hartrumpf, M., Sierig, T., Oesberg, N., Kirschke, C. G., and Albes, J. M. (2007). Cost reduction of perioperative coagulation management in cardiac surgery: Value of ‘bedside’ thrombelastography (ROTEM). Eur. J. Cardiothorac. Surg. 31 (6), 1052–1057. doi:10.1016/j.ejcts.2007.02.022
Sperry, J. L., Guyette, F. X., Brown, J. B., Yazer, M. H., Triulzi, D. J., Early-Young, B. J., et al. (2018). Prehospital plasma during air medical transport in trauma patients at risk for hemorrhagic shock. N. Engl. J. Med. 379 (4), 315–326. doi:10.1056/NEJMoa1802345
Speybroeck, J., Marsee, M., Shariff, F., Zackariya, N., Grisoli, A., Lune, S. V., et al. (2020). Viscoelastic testing in benign hematologic disorders: Clinical perspectives and future implications of point-of-care testing to assess hemostatic competence. Transfusion 60, S101–S121. doi:10.1111/trf.16088
Sprengers, E. D., and Kluft, C. (1987). Plasminogen activator inhibitors. Blood 69 (2), 381–387. doi:10.1182/blood.v69.2.381.bloodjournal692381
Stahl, K., Hillebrand, U. C., Kiyan, Y., Seeliger, B., Schmidt, J. J., Schenk, H., et al. (2021). Effects of therapeutic plasma exchange on the endothelial glycocalyx in septic shock. Intensive Care Med. Exp. 9 (1), 57. doi:10.1186/s40635-021-00417-4
Standl, T., Annecke, T., Cascorbi, I., Heller, A. R., Sabashnikov, A., and Teske, W. (2018). The nomenclature, definition and distinction of types of shock. Dtsch. Ärzteblatt Int. 115 (45), 757–768. doi:10.3238/arztebl.2018.0757
Starzl, T. E. (2002). The saga of liver replacement, with particular reference to the reciprocal influence of liver and kidney transplantation (1955-1967). J. Am. Coll. Surg. 195 (5), 587–610. doi:10.1016/s1072-7515(02)01498-9
Stein, E., McMahon, B., Kwaan, H., Altman, J. K., Frankfurt, O., and Tallman, M. S. (2009). The coagulopathy of acute promyelocytic leukaemia revisited. Best. Pract. Res. Clin. Haematol. 22 (1), 153–163. doi:10.1016/j.beha.2008.12.007
Stettler, G. R., Moore, E. E., Moore, H. B., Nunns, G. R., Silliman, C. C., Banerjee, A., et al. (2019). Redefining postinjury fibrinolysis phenotypes using two viscoelastic assays. J. Trauma Acute Care Surg. 86 (4), 679–685. doi:10.1097/TA.0000000000002165
Straat, M., Böing, A. N., Tuip-De Boer, A., Nieuwland, R., and Juffermans, N. P. (2016). Extracellular vesicles from red blood cell products induce a strong pro-inflammatory host response, dependent on both numbers and storage duration. Transfus. Med. Hemother 43 (4), 302–305. doi:10.1159/000442681
Strandin, T., Hepojoki, J., Laine, O., Mäkelä, S., Klingström, J., Lundkvist, Å., et al. (2016). Interferons induce STAT1–dependent expression of tissue plasminogen activator, a pathogenicity factor in puumala hantavirus disease. J. Infect. Dis. 213 (10), 1632–1641. doi:10.1093/infdis/jiv764
Stravitz, R. T., Fontana, R. J., Meinzer, C., Durkalski-Mauldin, V., Hanje, A. J., Olson, J., et al. (2021). Coagulopathy, bleeding events, and outcome according to rotational thromboelastometry in patients with acute liver injury/failure. Hepatology 74 (2), 937–949. doi:10.1002/hep.31767
Stravitz, R. T. (2012). Potential applications of thromboelastography in patients with acute and chronic liver disease. Gastroenterol. Hepatol. (N Y) 8 (8), 513–520.
Su, E. J., Fredriksson, L., Schielke, G. P., Eriksson, U., and Lawrence, D. A. (2009). Tissue plasminogen activator-mediated PDGF signaling and neurovascular coupling in stroke. J. Thromb. Haemost. 7 (1), 155–158. doi:10.1111/j.1538-7836.2009.03402.x
Tallman, M. S., and Kwaan, H. C. (1992). Reassessing the hemostatic disorder associated with acute promyelocytic leukemia [see comments]. Blood 79 (3), 543–553. doi:10.1182/blood.v79.3.543.543
Tanaka, K. A., Bader, S. O., and Görlinger, K. (2014). Novel approaches in management of perioperative coagulopathy. Curr. Opin. Anaesthesiol. 27 (1), 72–80. doi:10.1097/ACO.0000000000000025
Tanaka, K. A., Ogawa, S., and Bolliger, D. (2012). A primer for clinical use of rotational thromboelastometry. Point Care 11 (2), 77–84. doi:10.1097/poc.0b013e318259b18a
Tauber, H., Innerhofer, P., Breitkopf, R., Westermann, I., Beer, R., El Attal, R., et al. (2011). Prevalence and impact of abnormal ROTEM(R) assays in severe blunt trauma: Results of the 'diagnosis and treatment of trauma-induced coagulopathy (DIA-TRE-TIC) study. Br. J. Anaesth. 107 (3), 378–387. doi:10.1093/bja/aer158
Taylor, F. B., Toh, C.-H., Hoots, K. W., Wada, H., and Levi, M. Scientific Subcommittee on Disseminated Intravascular Coagulation DIC of the International Society on Thrombosis and Haemostasis ISTH (2001). Towards definition, clinical and laboratory criteria, and a scoring system for disseminated intravascular coagulation. Thrombosis haemostasis 86 (11), 1327–1330. doi:10.1055/s-0037-1616068
Thölking, G., Mesters, R., Dittrich, R., Pavenstädt, H., Kümpers, P., and Reuter, S. (2015). Assessment of hemostasis after plasma exchange using rotational thrombelastometry (ROTEM). PLoS One 10 (6), e0130402. doi:10.1371/journal.pone.0130402
Thorn, S., and Maegele, M. (2021). “Grading clinical coagulopathy and predicting massive transfusion,” in Trauma induced coagulopathy (Springer), 245–263.
Tian, H.-L., Chen, H., Wu, B.-S., Cao, H.-L., Xu, T., Hu, J., et al. (2010). D-Dimer as a predictor of progressive hemorrhagic injury in patients with traumatic brain injury: Analysis of 194 cases. Neurosurg. Rev. 33 (3), 359–365. doi:10.1007/s10143-010-0251-z
Tignanelli, C. J., and Napolitano, L. M. (2018). The fragility index in randomized clinical trials as a means of optimizing patient care. JAMA Surg. 154, 74–79. doi:10.1001/jamasurg.2018.4318
Tisherman, S. A., Schmicker, R. H., Brasel, K. J., Bulger, E. M., Kerby, J. D., Minei, J. P., et al. (2015). Detailed description of all deaths in both the shock and traumatic brain injury hypertonic saline trials of the Resuscitation Outcomes Consortium. Ann. Surg. 261 (3), 586–590. doi:10.1097/SLA.0000000000000837
Tkachenko, E., Rhodes, J. M., and Simons, M. (2005). Syndecans: New kids on the signaling block. Circ. Res. 96 (5), 488–500. doi:10.1161/01.RES.0000159708.71142.c8
Trąbka-Zawicki, A., Tomala, M., Zeliaś, A., Paszek, E., Zajdel, W., Stępień, E., et al. (2019). Adaptation of global hemostasis to therapeutic hypothermia in patients with out-of-hospital cardiac arrest: Thromboelastography study. Cardiol. J. 26 (1), 77–86. doi:10.5603/CJ.a2017.0080
Tran, H. T. T., Sørensen, B., Bjørnsen, S., Pripp, A. H., Tjønnfjord, G. E., and Andre Holme, P. (2015). Monitoring bypassing agent therapy - a prospective crossover study comparing thromboelastometry and thrombin generation assay. Haemophilia 21 (2), 275–283. doi:10.1111/hae.12570
Tripodi, A., and Mannucci, P. M. (2011). The coagulopathy of chronic liver disease. N. Engl. J. Med. 365 (2), 147–156. doi:10.1056/NEJMra1011170
Trzebicki, J., Flakiewicz, E., Kosieradzki, M., Blaszczyk, B., Kołacz, M., Jureczko, L., et al. (2010). The use of thromboelastometry in the assessment of hemostasis during orthotopic liver transplantation reduces the demand for blood products. Ann. Transpl. 15 (3), 19–24.
Tuan, T. A., Ha, N. T. T., Xoay, T. D., My, T. T. K., Nghiem, L. T., and Dien, T. M. (2021). Hypocoagulable tendency on thromboelastometry associated with severity and anticoagulation timing in pediatric septic shock: A prospective observational study. Front. Pediatr. 9, 676565. doi:10.3389/fped.2021.676565
Vaughan, D. E. (2005). PAI-1 and atherothrombosis. J. Thromb. Haemost. 3 (8), 1879–1883. doi:10.1111/j.1538-7836.2005.01420.x
Veigas, P. V., Callum, J., Rizoli, S., Nascimento, B., and da Luz, L. T. (2016). A systematic review on the rotational thrombelastometry (ROTEM®) values for the diagnosis of coagulopathy, prediction and guidance of blood transfusion and prediction of mortality in trauma patients. Scand. J. Trauma Resusc. Emerg. Med. 24 (1), 114. doi:10.1186/s13049-016-0308-2
Vercauteren, E., Gils, A., and Declerck, P. J. (2013). Thrombin activatable fibrinolysis inhibitor: A putative target to enhance fibrinolysis. Seminars thrombosis hemostasis 39 (04), 365–372. doi:10.1055/s-0033-1334488
Viersen, V., Greuters, S., Korfage, A., Van der Rijst, C., Van Bochove, V., Nanayakkara, P., et al. (2012). Hyperfibrinolysis in out of hospital cardiac arrest is associated with markers of hypoperfusion. Resuscitation 83 (12), 1451–1455. doi:10.1016/j.resuscitation.2012.05.008
Vincent, J.-L., and De Backer, D. (2013). Circulatory shock. N. Engl. J. Med. 369 (18), 1726–1734. doi:10.1056/NEJMra1208943
Vincent, J.-L., Francois, B., Zabolotskikh, I., Daga, M. K., Lascarrou, J.-B., Kirov, M. Y., et al. (2019). Effect of a recombinant human soluble thrombomodulin on mortality in patients with sepsis-associated coagulopathy: The SCARLET randomized clinical trial. Jama 321 (20), 1993–2002. doi:10.1001/jama.2019.5358
Vischer, U. M., and Wollheim, C. B. (1997). Epinephrine induces von Willebrand factor release from cultured endothelial cells: Involvement of cyclic AMP-dependent signalling in exocytosis. Thromb. Haemost. 77 (6), 1182–1188. doi:10.1055/s-0038-1656135
Vogel, S., Bodenstein, R., Chen, Q., Feil, S., Feil, R., Rheinlaender, J., et al. (2015). Platelet-derived HMGB1 is a critical mediator of thrombosis. J. Clin. investigation 125 (12), 4638–4654. doi:10.1172/JCI81660
Volod, O., Bunch, C. M., Zackariya, N., Moore, E. E., Moore, H. B., Kwaan, H. C., et al. (2022). Viscoelastic hemostatic assays: A primer on legacy and new generation devices. J. Clin. Med. 11 (3), 860. doi:10.3390/jcm11030860
Volod, O., and Wegner, J. (2022). Viscoelastic testing in the management of adult patients on mechanical circulatory support devices with focus on extracorporeal membrane oxygenation. Seminars Thrombosis Hemostasis 48 (7), 814–827. doi:10.1055/s-0042-1756303
Vorweg, M., Hanke, A., Gorlinger, K., and Lier, H. (2013). Thromboelastometry guided therapy of severe bleeding. Essener Runde algorithm. Hämostaseologie 33, 51–61. doi:10.5482/HAMO-12-05-0011
Vulliamy, P., Gillespie, S., Armstrong, P. C., Allan, H. E., Warner, T. D., and Brohi, K. (2019). Histone H4 induces platelet ballooning and microparticle release during trauma hemorrhage. Proc. Natl. Acad. Sci. 116 (35), 17444–17449. doi:10.1073/pnas.1904978116
Wada, T. (2017). Coagulofibrinolytic changes in patients with post-cardiac arrest syndrome. Front. Med. 4, 156. doi:10.3389/fmed.2017.00156
Wada, T., Gando, S., Mizugaki, A., Kodate, A., Sadamoto, Y., Murakami, H., et al. (2017). Differences in coagulofibrinolytic changes between post-cardiac arrest syndrome of cardiac causes and hypoxic insults: A pilot study. Acute Med. Surg. 4 (3), 371–372. doi:10.1002/ams2.270
Wada, T., Gando, S., Ono, Y., Maekawa, K., Katabami, K., Hayakawa, M., et al. (2016). Disseminated intravascular coagulation with the fibrinolytic phenotype predicts the outcome of patients with out-of-hospital cardiac arrest. Thromb. J. 14, 43. doi:10.1186/s12959-016-0116-y
Wallner, B., Schenk, B., Hermann, M., Paal, P., Falk, M., Strapazzon, G., et al. (2020). Hypothermia-associated coagulopathy: A comparison of viscoelastic monitoring, platelet function, and real time live confocal microscopy at low blood temperatures, an in vitro experimental study. Front. Physiology 11, 843. doi:10.3389/fphys.2020.00843
Walsh, M., Kwaan, H., McCauley, R., Marsee, M., Speybroeck, J., Thomas, S., et al. (2020a). Viscoelastic testing in oncology patients (including for the diagnosis of fibrinolysis): Review of existing evidence, technology comparison, and clinical utility. Transfusion 60, S86–S100. doi:10.1111/trf.16102
Walsh, M., Moore, E. E., Moore, H., Thomas, S., Lune, S. V., Zimmer, D., et al. (2019). Use of Viscoelastography in malignancy-associated coagulopathy and thrombosis: A review. Semin. Thromb. Hemost. 45 (4), 354–372. doi:10.1055/s-0039-1688497
Walsh, M., Thomas, S., Kwaan, H., Aversa, J., Anderson, S., Sundararajan, R., et al. (2020b). Modern methods for monitoring hemorrhagic resuscitation in the United States: Why the delay? J. Trauma Acute Care Surg. 89 (6), 1018–1022. doi:10.1097/TA.0000000000002977
Wang, S., Qi, C., Liu, Z., Xu, T., and Yao, C. (2020). Endogenous heparin-like substances may cause coagulopathy in a patient with severe postpartum hemorrhage. Transfus. Med. Hemotherapy 47 (4), 337–343. doi:10.1159/000504610
Webb, A. J., Brown, C. S., Naylor, R. M., Rabinstein, A. A., Mara, K. C., and Nei, A. M. (2021). Thromboelastography is a marker for clinically significant progressive hemorrhagic injury in severe traumatic brain injury. Neurocritical Care 35, 738–746. doi:10.1007/s12028-021-01217-0
Wei, S., Kao, L. S., Wang, H. E., Chang, R., Podbielski, J., Holcomb, J. B., et al. (2018). Protocol for a pilot randomized controlled trial comparing plasma with balanced crystalloid resuscitation in surgical and trauma patients with septic shock. Trauma Surg. Acute Care Open 3 (1), e000220. doi:10.1136/tsaco-2018-000220
Weitz, J. I., and Eikelboom, J. W. (2016). Urgent need to measure effects of direct oral anticoagulants. Circulation 134 (3), 186–188. doi:10.1161/CIRCULATIONAHA.116.022307
Welling, H., Ostrowski, S. R., Stensballe, J., Vestergaard, M. R., Partoft, S., White, J., et al. (2019). Management of bleeding in major burn surgery. Burns 45 (4), 755–762. doi:10.1016/j.burns.2018.08.024
Wernly, B., Fuernau, G., Masyuk, M., Muessig, J. M., Pfeiler, S., Bruno, R. R., et al. (2019). Syndecan-1 predicts outcome in patients with ST-segment elevation infarction independent from infarct-related myocardial injury. Sci. Rep. 9 (1), 18367–7. doi:10.1038/s41598-019-54937-x
White, N. J., Leong, B. S., Brueckner, J., Martin, E. J., Brophy, D. F., Peberdy, M. A., et al. (2011). Coagulopathy during cardiac arrest and resuscitation in a swine model of electrically induced ventricular fibrillation. Resuscitation 82 (7), 925–931. doi:10.1016/j.resuscitation.2011.02.034
Whiting, D., and DiNardo, J. A. (2014). TEG and ROTEM: Technology and clinical applications. Am. J. Hematol. 89 (2), 228–232. doi:10.1002/ajh.23599
Windelv, N. A., Welling, K.-L., Ostrowski, S. R., and Johansson, P. I. (2011). The prognostic value of thrombelastography in identifying neurosurgical patients with worse prognosis. Blood coagulation fibrinolysis 22 (5), 416–419. doi:10.1097/MBC.0b013e3283464f53
Wohlauer, M. V., Moore, E. E., Thomas, S., Sauaia, A., Evans, E., Harr, J., et al. (2012). Early platelet dysfunction: An unrecognized role in the acute coagulopathy of trauma. J. Am. Coll. Surg. 214 (5), 739–746. doi:10.1016/j.jamcollsurg.2012.01.050
Woodcock, T. E., and Michel, C. C. (2021). Advances in the starling principle and microvascular fluid exchange; consequences and implications for fluid therapy. Front. Vet. Sci. 8, 623671. doi:10.3389/fvets.2021.623671
Wu, Y., Liu, W., Zhou, Y., Hilton, T., Zhao, Z., Liu, W., et al. (2018). von Willebrand factor enhances microvesicle-induced vascular leakage and coagulopathy in mice with traumatic brain injury. J. Am. Soc. Hematol. 132 (10), 1075–1084. doi:10.1182/blood-2018-03-841932
Xiao, W., Mindrinos, M. N., Seok, J., Cuschieri, J., Cuenca, A. G., Gao, H., et al. (2011). A genomic storm in critically injured humans. J. Exp. Med. 208 (13), 2581–2590. doi:10.1084/jem.20111354
Xiong, Y., Zhou, Q., Jiang, F., Zhou, S., Lou, Y., Guo, Q., et al. (2010). Changes of plasma and placental tissue factor pathway inhibitor-2 in women with preeclampsia and normal pregnancy. Thromb. Res. 125 (6), e317–e322. doi:10.1016/j.thromres.2010.02.017
Xu, X., Kozar, R., Zhang, J., and Dong, J. f. (2020). Diverse activities of von Willebrand factor in traumatic brain injury and associated coagulopathy. J. Thrombosis Haemostasis 18 (12), 3154–3162. doi:10.1111/jth.15096
Yanagida, Y., Gando, S., Sawamura, A., Hayakawa, M., Uegaki, S., Kubota, N., et al. (2013). Normal prothrombinase activity, increased systemic thrombin activity, and lower antithrombin levels in patients with disseminated intravascular coagulation at an early phase of trauma: Comparison with acute coagulopathy of trauma-shock. Surgery 154 (1), 48–57. doi:10.1016/j.surg.2013.02.004
Yen, W., Cai, B., Yang, J., Zhang, L., Zeng, M., Tarbell, J. M., et al. (2015). Endothelial surface glycocalyx can regulate flow-induced nitric oxide production in microvessels in vivo. PLOS ONE 10 (1), e0117133. doi:10.1371/journal.pone.0117133
Yepes, M. (2015). Tissue-type plasminogen activator is a neuroprotectant in the central nervous system. Front. Cell Neurosci. 9, 304. doi:10.3389/fncel.2015.00304
Yu, G., Kim, Y.-J., Kim, J.-s., Kim, S.-I., Ryoo, S. M., Ahn, S., et al. (2020). Prognostic value of repeated thromboelastography measurement for favorable neurologic outcome during targeted temperature management in out-of-hospital cardiac arrest survivors. Resuscitation 155, 65–73. doi:10.1016/j.resuscitation.2020.07.019
Yuan, Q., Sun, Y.-r., Wu, X., Yu, J., Li, Z.-q., Du, Z.-y., et al. (2016). Coagulopathy in traumatic brain injury and its correlation with progressive hemorrhagic injury: A systematic review and meta-analysis. J. Neurotrauma 33 (14), 1279–1291. doi:10.1089/neu.2015.4205
Zakarija, A., and Kwaan, H. C. (2007). Adverse effects on hemostatic function of drugs used in hematologic malignancies. Seminars thrombosis hemostasis 33 (04), 355–364. doi:10.1055/s-2007-976171
Zeng, Y., and Liu, J. (2016). Role of glypican-1 in endothelial NOS activation under various steady shear stress magnitudes. Exp. Cell Res. 348 (2), 184–189. doi:10.1016/j.yexcr.2016.09.017
Zeng, Y., and Tarbell, J. M. (2014). The adaptive remodeling of endothelial glycocalyx in response to fluid shear stress. PLoS One 9 (1), e86249. doi:10.1371/journal.pone.0086249
Zilkens, C., Friese, J., Köller, M., Muhr, G., and Schinkel, C. (2008). HEPATIC FAILURE AFTER INJURY–ACommon PATHOGENESIS WITH SCLEROSING CHOLANGITIS? Eur. J. Med. Res. 13 (7), 309–313.
Zipperle, J., Oberladstätter, D., Weichselbaum, N., Schlimp, C. J., Hofmann, N., Iapichino, G., et al. (2022a). Thromboelastometry fails to detect autoheparinization after major trauma and hemorrhagic shock. J. Trauma Acute Care Surg. 92 (3), 535–541. doi:10.1097/TA.0000000000003464
Zipperle, J., Ziegler, B., Schöchl, H., Voelckel, W., Dungel, P., Cadamuro, J., et al. (2022b). Conventional and pro-inflammatory pathways of fibrinolytic activation in non-traumatic hyperfibrinolysis. J. Clin. Med. 11 (24), 7305. doi:10.3390/jcm11247305
Glossary
AA Arachidonic Acid
ADAM A Disintegrin And Metalloproteinase
ADP Adenosine Diphosphate
AFE Amniotic Fluid Embolism
AMP Adenosine Monophosphate
aPC activated Protein C
APL Acute Promyelocytic Leukemia
aPTT activated Partial Thromboplastin Time
AT Antithrombin III
ATP Adenosine Triphosphate
BCT Blood Component Therapy
BIC Burn-Induced Coagulopathy
CCT Conventional Coagulation Tests
CI Coagulation Index
CTBI Coagulopathy of Traumatic Brain Injury
DAMPs Damage-Associated Molecular Patterns
DIC Disseminated Intravascular Coagulation
ECMO Extracorporeal Membrane Oxygenation
eNOS endothelial Nitric Oxide Synthase
FDP Fibrin/Fibrinogen Degradation Product
GAG Glycosaminoglycan
HAT Hemostatic Adjunct Therapy
HMGB1 High Mobility Group Box 1
HS Heparan Sulfate
HSPG Heparan Sulfate Proteoglycan
ICAM-1 Intercellular Adhesion Molecule-1
ISS Injury Severity Score
LOT Lysis Onset Time
LVAD Left Ventricular Assist Device
MCF Maximum Clotting Firmness
MEA Multiple Electrode Aggregometry
MIS-C Multisystem Inflammatory Syndrome in Children
ML Maximum Lysis
MT Massive Transfusion
MTH Mild Therapeutic Hypothermia
NETs Neutrophil Extracellular Traps
PAI-1 Plasminogen Activator Inhibitor-1
PAMPs Pathogen-Associated Molecular Patterns
PBM Precision-Based Medicine
PCAS Post Cardiac Arrest Syndrome
PPH Postartum Hemorrhage
PT Prothrombin Time
RHAMM Receptor of Hyaluronan-Mediated Motility
ROSC Return Of Spontaneous Circulation
ROTEM Rotational Thromboelastometry
rTEG Rapid Thromboelastography
SHINE Shock-Induced Endotheliopathy
SHOT Systems Hypothesis of Trauma
SIC Sepsis-Induced Coagulopathy
SOFA Sequential Organ Failure Assessment
sTM soluble Thrombomodulin
Syn1 Syndecan-1
Syn4 Syndecan-4
TAFI Thrombin-Activatable Fibrinolysis Inhibitor
TBI Traumatic Brain Injury
TEG Thromboelastography
TF Tissue Factor
TFPI Tissue Factor Pathway Inhibitor
TGA Thrombin Generation Assays
TIC Trauma-Induced Coagulopathy
TIMP Tissue Inhibitor of Metalloproteinase
TLR Toll-Like Receptor
TM Thrombomodulin
tPA tissue Plasminogen Activator
TPE Therapeutic Plasma Exchange
TRAP Thrombin Receptor Activating Peptides
TXA Tranexamic Acid
VHA Viscoelastic Hemostatic Assay
Keywords: critical care, endothelium, glycocalyx, hemostasis, precision medicine, resuscitation, shock, thromboelastography
Citation: Bunch CM, Chang E, Moore EE, Moore HB, Kwaan HC, Miller JB, Al-Fadhl MD, Thomas AV, Zackariya N, Patel SS, Zackariya S, Haidar S, Patel B, McCurdy MT, Thomas SG, Zimmer D, Fulkerson D, Kim PY, Walsh MR, Hake D, Kedar A, Aboukhaled M and Walsh MM (2023) SHock-INduced Endotheliopathy (SHINE): A mechanistic justification for viscoelastography-guided resuscitation of traumatic and non-traumatic shock. Front. Physiol. 14:1094845. doi: 10.3389/fphys.2023.1094845
Received: 10 November 2022; Accepted: 07 February 2023;
Published: 27 February 2023.
Edited by:
Belinda H. McCully, Western University of Health Sciences, United StatesReviewed by:
Amaresh Ranjan, Midwestern University, United StatesAllan Doctor, University of Maryland, United States
Copyright © 2023 Bunch, Chang, Moore, Moore, Kwaan, Miller, Al-Fadhl, Thomas, Zackariya, Patel, Zackariya, Haidar, Patel, McCurdy, Thomas, Zimmer, Fulkerson, Kim, Walsh, Hake, Kedar, Aboukhaled and Walsh. This is an open-access article distributed under the terms of the Creative Commons Attribution License (CC BY). The use, distribution or reproduction in other forums is permitted, provided the original author(s) and the copyright owner(s) are credited and that the original publication in this journal is cited, in accordance with accepted academic practice. No use, distribution or reproduction is permitted which does not comply with these terms.
*Correspondence: Mark M. Walsh, markwalshmd@gmail.com