- 1Laboratory of Vascular Physiology and Medicine, Department of Physiology, Shri B. M. Patil Medical College, Hospital & Research Centre, BLDE (Deemed to be University), Vijayapura, Karnataka, India
- 2Department of Anatomy, Shri B. M. Patil Medical College, Hospital & Research Center, BLDE (Deemed to be University), Vijayapura, Karnataka, India
- 3Department of Biochemistry, Shri B. M. Patil Medical College, Hospital & Research Center, BLDE (Deemed to be University), Vijayapura, Karnataka, India
- 4Department of Surgery, Shri B. M. Patil Medical College, Hospital & Research Center, BLDE (Deemed to be University), Vijayapura, Karnataka, India
- 5Department of Chemistry, BLDEA’s V P Dr PG Halakatti College of Engineering and Technology, Vijayapura, Karnataka, India
Present study aimed to assess effect of pre-treatment with Mucuna pruriens seed extract and its bioactive molecule(s) on NMDAR and Tau protein gene expression in cerebral ischemic rodent model. Methanol extract of M. pruriens seeds was characterized by HPLC, and β-sitosterol was isolated by flash chromatography. In vivo studies to observe the effect of pre-treatment (28 days) with methanol extract of M. pruriens seed and β-sitosterol on the unilateral cerebral ischemic rat model. Cerebral ischemia induced by left common carotid artery occlusion (LCCAO) for 75 min (on day 29) followed by reperfusion for 12 h. Rats (n = 48) divided into four groups. GroupI (control,Untreated + LCCAO)-No pre-treatment + cerebral ischemia; GroupII(β-sitosterol + Sham)-pre-treatment with β-sitosterol, 10 mg/kg/day + sham-operated; GroupIII(β-sitosterol + LCCAO)-pre-treatment with β-sitosterol, 10 mg/kg/day + cerebral ischemia; GroupIV(methanol extract + LCCAO)-pre-treatment with methanol extract of M. pruriens seeds, 50 mg/kg/day + cerebral ischemia. Neurological deficit score was assessed just before sacrifice. Experimental animals were sacrificed after 12 h reperfusion. Brain histopathology was performed. Gene expression of NMDAR and Tau protein of left cerebral hemisphere (occluded side) was performed by RT-PCR. Results revealed that the neurological deficit score was lower in groups III and IV compared to group I. NMDAR and tau protein mRNA expression in left cerebral hemisphere were upregulated in Group I, downregulated in groups III and IV. Histopathology of left cerebral hemisphere (occluded side) in Group I showed features of ischemic brain damage. Groups III and IV, left cerebral hemisphere showed less ischemic damage compared GroupI. Right cerebral hemisphere showed no areas of ischemia-induced brain changes. Pre-treatment with β-sitosterol and methanol extract of M. pruriens seeds may reduce ischemic brain injury following unilateral common carotid artery occlusion in rats.
1 Introduction
With its two subtypes, ischemic and hemorrhagic, stroke is among the leading causes of morbidity and mortality. Ischemic cerebral injury accounts for 87% of all stroke cases (Silva et al., 2017; Wu and Tymianski, 2018). To date, the recanalization of the occluded vessels with tissue plasminogen activator is the widely used therapeutic option. However, the narrow therapeutic time window (within 4.5 h post-stroke) and the severe tissue damage following reperfusion are significant limitations to this treatment modality (Martin and Wang, 2010).
The role of NMDA (N-methyl-d-aspartate) receptors and Tau protein in ‘excitotoxicity’, a mechanism central to neuronal death post cerebral ischemia, have been delineated (Martin and Wang, 2010). Consequently, targeting NMDA receptors and Tau protein could be potentially effective in managing ischemic stroke that can be further explored.
Medicinal plants have long been relied upon in managing several diseases, including cerebrovascular diseases. They have an important place in traditional medicine, including Ayurveda, an Indian system of medicine. The health benefits and therapeutic effects of plants are due to the properties of secondary plant metabolites (phytochemicals) they contain. Phytochemicals have been demonstrated to affect several pathophysiological processes underlying stroke like oxidative stress, inflammation and apoptotic cell death. Hence, phytochemicals are a better, safer, and cost-effective alternative to synthetic drugs (Kim et al., 2016; Hussein et al., 2018; Surguchov et al., 2021). Mucuna pruriens is one such medicinal plant that is widely used in Ayurveda (Pathania et al., 2020).
Mucuna pruriens (M. pruriens), routinely known as Mucuna or velvet bean, is a popular medicinal plant belonging to the legume family and common in tropical and subtropical areas worldwide. In India, it is widely found in the eastern part of the country (Kamkaen et al., 2022). Several studies have reported the anti-Parkinson, antidiabetic, antioxidant, antibacterial, antiepileptic, antineoplastic, male fertility, and aphrodisiac effects of M. pruriens (Rane et al., 2019; Theansungnoen et al., 2022). Phytochemical analysis of M. pruriens seed extract has demonstrated a significant content of L-dopa accounting for its anti-parkinsonism properties. It has been widely used in Ayurveda as a medicinal plant since Vedic times and is used to treat various nervous disorders, probably due to its neuroprotective properties (Rane et al., 2019; Kamkaen et al., 2022). Hence, the primary objective of the present work was to study the possible neuroprotective effect of pretreatment with M. pruriens seed extract and its isolated bioactive molecule(s) in the cerebral ischemic rat model and its impact on NMDA receptor and Tau protein gene expression.
2 Materials and methods
2.1 In vitro studies
In vitro studies involved phytochemical extraction of M. pruriens seeds and determining the total alkaloids, flavonoids, phenols, in the seed extract. The antioxidant property of the seed extract was assayed. Further, the study involved HPLC and flash chromatography to estimate and isolate bioactive molecule(s) from M. pruriens seed extract.
2.1.1 Phytochemical extraction
The seeds of M. pruriens were collected from Karnataka, India. The seed powder was subjected to extraction using methanol, ethanol, petroleum ether, chloroform, and water solvents (Senguttuvan et al., 2014; Kalebar et al., 2020). The qualitative analysis of the solvent extracts revealed that the methanol extract of M. pruriens seeds possessed a superior phytochemical profile additionally supported by the in vitro antioxidant assays (Lampariello et al., 2012; Rane et al., 2019). Hence, in the present study methanol extract of M. pruriens seeds was used. Methanol extract of M. pruriens seed will be denoted as M. pruriens seed extract, henceforth.
Methanol extract of M. pruriens seeds was subjected to determination of total alkaloids, flavonoids, phenols as per standard protocol (Senguttuvan et al., 2014; Kalebar et al., 2020). The antioxidant efficiency of M. pruriens seed extract was determined by hydrogen peroxide scavenging assay and 1,1-Diphenyl-2-Picrylhydrazyl (DPPH) radical scavenging assay as per standard protocol (Brand-Williams et al., 1995; Siddhuraju and Becker, 2003; Keser et al., 2012).
2.1.2 HPLC and flash chromatography analysis
By using HPLC, the bioactive molecules, namely, L-DOPA and β-sitosterol were identified from Mucuna prurines seed extract (Kshirsagar et al., 2016). Waters HPLC system (Waters/Millipore, Milsford, MA, United States) which included a model 515 pump, a model 2,487 dual wavelength absorbance detector was employed. A C18 column was used. The mobile phase was constituted by A (water) and B (acetonitrile). The gradient range varied linearly from 50% to 90% B in 4 min with injection volume 2 mL for the RRHT column. The flow rate was 1.0 mL/min, the column temperature was maintained at 30°C, and the detection wavelength was 280 nm (Pawar et al., 2010). The extraction of bioactive molecule β-sitosterol from M. pruriens seed extract was done by Reversed-Phase Flash Chromatography. The isolation was carried out in Biotage Flash 75 system (Biotage, A Division of Dyax Corporation, Charlottsville, VA, United States) (Herrera et al., 2020).
2.2 In vivo study
In vivo study was undertaken to investigate for the possible neuroprotective effect of M. pruriens seed extract as well as its isolated bioactive molecule in rat model of cerebral ischemia and to understand the underlying molecular mechanism by analyzing the gene expression of brain NMDAR and Tau protein.
2.2.1 Ethical approval
Ethical approval for the study was obtained from Institutional Animal Ethics Committee (IAEC) (No.08/BLDE (DU)/2021). The animal care guidelines set forth by the Committee for the Purpose and Control and Supervision of Experiments on Animals (CPCSEA), Ministry of Environment and Forests (Animal Welfare Division), Government of India were strictly adhered to throughout the study.
2.2.2 Experimental animals
The study involved 48 male Wistar rats, aged 8–10 weeks, weighing 180–250 gm. The experimental animals were maintained at 22°C–24°C and a 12-h light/12-h dark cycle with food and water ad libitum. The animals were acclimatized to the laboratory conditions for 7 days before initiating the experimental protocol.
2.2.3 Study design
The experimental animals (n = 48) were allocated to one of the four groups. Group I (Untreated + LCCAO) served as control. No pre-treatment. Cerebral ischemia induced (on day 29) by LCCAO for 75 min followed by reperfusion for 12 h. Group II (β-sitosterol + Sham)—Pre-treatment with β-sitosterol, 10 mg/kg/day, (Ayaz et al., 2017), oral, 28 days + sham-operated (on day 29). Group III (β-sitosterol + LCCAO) - pre-treatment with β-sitosterol, 10 mg/kg/day, oral, 28 days + cerebral ischemia induced (on day 29) by LCCAO for 75 min followed by reperfusion for 12 h. Group IV (methanol extract + LCCAO) - pre-treatment with methanol extract of Mucuna pruriens seeds, 50 mg/kg/day (Nayak et al., 2017), oral, 28 days + cerebral ischemia induced by LCCAO for 75 min followed by reperfusion for 12 h (on day 29).
The bodyweight of rats was measured using digital weighing machine (Practum 1102-10IN, Germany). The experimental animals in each group were matched for the body weight at the study onset. Daily food consumption of each rat was monitored during the intervention period.
2.2.4 Cerebral ischemia
Cerebral ischemia was induced by left common carotid artery occlusion (LCCAO) for 75 min followed by 12 h reperfusion (Das et al., 2018). The surgical procedure for inducing cerebral ischemia was performed in the morning hours in overnight fasted rats. The animals were subjected to the procedure after the intervention period of 28 days (i.e., on day 29). Strict aseptic precautions were taken during the surgical procedure. The experimental animals were anesthetized with ketamine (60 mg/kg body weight, i. p.) and xylazine (6 mg/kg body weight). An oblique incision was taken alongside sternocleidomastoid in the neck from the base of the mandible to sternum. At the level of greater of cornu of thyroid cartilage, deep fascia and carotid sheath were incised. The common carotid artery was exposed and separated from the fascia, while protecting the vagus and jugular vein from any damage. A nylon thread (0.5 mm) was passed from behind the artery and ligated. The occlusion was maintained for 75 min following which the ligature was released and allowed for 12 h reperfusion. The deep fascia and carotid sheath were closed with proline. Superficial fascia and skin were closed with all aseptic precautions. Sham operation (group II) involved the same surgical steps except for left common carotid artery occlusion. The experimental animals were closely monitored for physiological parameters like heart rate, blood pressure and respiratory rate. Non-Invasive Blood Pressure was recorded with a tail cuff sensor (Biopac NIBP200A model). For heart rate, ECG was recorded using needle subcutaneous electrodes (Biopac Student Lab 4.1). For respiratory rate, pneumogram was recorded using respiratory pad transducer (Biopac Student Lab 4.1). Body temperature was maintained at 36°C–37.5°C using a warm pad. The surgery was uneventful and all the rats recovered following surgery.
2.2.5 Neurological deficit score
Post-ischemic neurological deficit was assessed after 12 h of reperfusion and before sacrifice using a six point scale neurological score: 0 = no neurological deficit; 1 = failure to extend contralateral forelimb fully on lifting the animal by tail; 2 = circling to the contralateral side; 3 = falling to the contralateral side; 4 = no spontaneous walk or in a comatose state; 5 = death (Komatsu et al., 2021).
2.2.6 Analysis of brain NMDA receptor and tau protein gene expression
The experimental animals were sacrificed (n = 6/group) after 12 h reperfusion. The brain was carefully dissected. For gene expression analysis, the left cerebral hemisphere (occluded side) was used. Tissue was immediately stored in RNAlater at −80°C for RT-PCR analysis.
NMDA receptor, tau protein and β-actin (endogenous control) mRNA expressions were assessed by using quantitative reverse transcription-PCR (RTPCR). RNeasy kit was used to extract RNA from left cerebral hemisphere homogenates as per the manufacturer’s protocol. Reverse transcription of RNA was done using SuperScript II Reverse Transcriptase as per the prescribed protocol. cDNA was amplified with quantitative reverse transcription-PCR (Kong et al., 2021). The gene expression of NMDAR and Tau protein are presented as fold change calculated by using the formula 2^-ΔΔCq (Herur et al., 2022). PCR primers specific to each gene are as depicted in Table 1. The PCR conditions are provided as Supplementary Table S1.
2.2.7 Histopathological examination
The animals (n = 6/group) were sacrificed after 12 h reperfusion. Brain was carefully dissected and initially fixed in bouin’s fluid and shifted to 10% neutral buffered formalin 24 h later. For histopathological examination, 2–3 mm thick slices of cerebral hemispheres were made. Bilateral blocks of the brain were embedded in paraffin wax and 2.0 to 3.0 μ sections were cut on a rotary microtome and stained with hematoxylin and eosin (H and E). Blocks included sections from the body of the corpus callosum with coronal section of white matter, splenium of the corpus callosum, posterior limb of the internal capsule with adjacent thalamus, and rostral pons. The stained sections were examined under light microscope (Olympus CH20i) with Samsung Digital Color Camera (Model No. SDC-242, N. J 07094, United States).
3 Statistical analysis
SPSS software (version 20.0) was used for statistical analysis. Data are presented as Mean ± SD. To analyze statistical significant difference across multiple groups, one way ANOVA was used followed by Tukey’s post hoc test to ascertain significant intergroup differences. p < 0.05 was considered statistically significant.
4 Results
4.1 In vitro studies
In the present study the pharmacognostical and phytochemical profile of M. pruriens was screened by sequential phytochemical extraction from its seed powder using different solvents viz., methanol, ethanol, petroleum ether, chloroform, and water. The qualitative analysis of the phytochemical constituents of M. pruriens seed extract with different solvents is provided as Supplementary Table S2. The phytochemical constituents in M. pruriens seed extract is provided as Supplementary Tables S3, S4. The antioxidant activity was evaluated by H2O2 scavenging assay and DPPH radical scavenging assay. The results of DPPH radical scavenging assay and H2O2 scavenging assay are provided as Supplementary Tables S5, S6. The DPPH and hydrogen peroxide scavenging activity of methanol extract of M. pruriens seeds was concentration dependent with greater scavenging at higher concentration. Ascorbic acid was used as a control and the scavenging activity of ascorbic acid was similarly concentration dependent.
4.1.1 HPLC and flash chromatography
HPLC chromatograms of standard solutions of L-DOPA and β-sitosterol and methanol extract of M. pruriens are presented in Figure 1. The calibration curve showed good linearity with correlation coefficients (R2- 0.9975). Based on the data, the estimates of L-DOPA and β-sitosterol in the M. pruriens seed extract was 20mg/100 gm and 12mg/100 gm respectively.
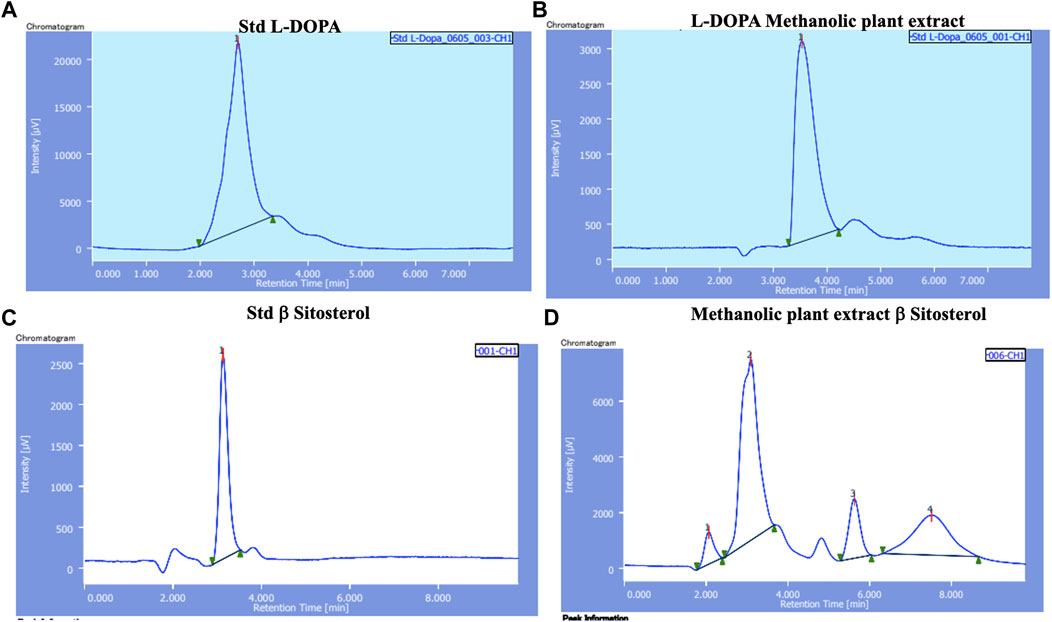
FIGURE 1. HPLC chromatograms of (A) L-DOPA standard; (B) L-DOPA from methanol extract of M. pruriens seed; (C) β-sitosterol standard; (D) β-sitosterol from methanol extract of M. pruriens seed.
4.2 In vivo studies
4.2.1 Physiological parameters
The comparison of physiological parameters before and after surgery as presented as Supplementary Table S7. Heart rate, SBP, DBP and respiratory rate were similar between groups before surgery. After surgery there was an increase in heart rate, SBP, DBP and respiratory rate across all groups. Heart rate and SBP were higher in group I (control) compared to other groups.
4.2.2 Neurological deficit score
Table 2 presents comparison of neurological deficit scores among groups. Neurological deficit score was higher in group I (Control, Untreated + LCCAO), group III (β-sitosterol + LCCAO) and group IV (methanol extract + LCCAO) compared to group II (β-sitosterol + Sham). The scores were lower in group III (β-sitosterol + LCCAO) and IV (methanol extract + LCCAO) compared to group I (Control, Untreated + LCCAO) although not statistically significant.
4.2.3 NMDAR and tau protein gene expression in left cerebral hemisphere homogenate
Table 3 presents Real time PCR results for NMDAR and Tau protein mRNA expression in experimental animals. mRNA expression of NMDAR and Tau protein was higher in Group I (control,Untreated + LCCAO) compared to group II (β-sitosterol + sham). In the pretreated groups, group III (β-sitosterol + LCCAO) and group IV (methanol extract + LCCAO) mRNA expression of NMDAR and Tau protein was lower compared to group 1 (control, Untreated+LCCAO).
4.2.4 Histopathological examination of cerebral hemispheres
Figure 2 presents the photomicrograph of cerebral cortices stained with H&E. In Group I (control, Untreated + LCCAO), left cerebral hemisphere (occluded side) showed cerebral edema (gross alteration), altered microscopic architecture with scattered cellular layers of grey matter and white matter. Neuronal damage (red neurons) with eosinophilic cytoplasm and pyknotic nuclei with a hazy appearance were noticed. Localized cystic infarcts with features of diffuse cerebral infarction were seen (Figure 2A - i, ii). There were no microscopic alterations in the left cerebral hemisphere of group II (β-sitosterol + Sham) (Figure 2B - i, ii). In Group III (β-sitosterol + LCCAO) (Figure 2C - i, ii) and IV (methanol extract + LCCAO) (Figure 2D - i, ii), left cerebral hemisphere (occluded side) showed less ischemic changes compared to group I (control, LCCAO). Right cerebral hemisphere of the brain (non-occluded side) showed no evidence of ischemic changes in all groups (Figures 2A-D - iii, iv). However, mild increase in intracellular space was noticed with no evidence of changes in corticomedullary junction in group I (Figure 2A - iii, iv).
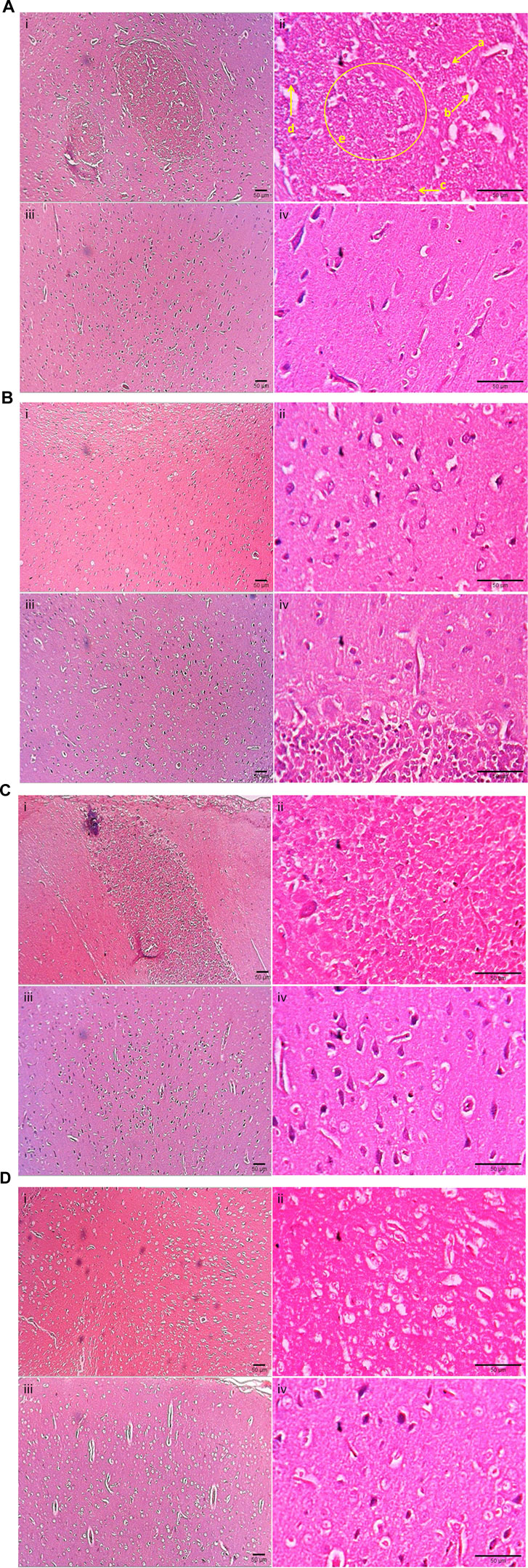
FIGURE 2. Photomicrograph depicting cerebral cortices stained with hematoxylin and eosin of Groups I, II, III, IV. (A): Photomicrograph of cerebral cortices stained with hematoxylin and eosin of Group I (control, Untreated + LCCAO) showing; i) Left (occluded) cerebral cortex (×10), ii) Left (occluded) cerebral cortex (×40), iii) Right (non-occluded) cerebral cortex (×10), iv) Right (non-occluded) cerebral cortex (×40). a - Pyknotic nuclei, b – red neurons with purkinje fibre, c- mild lymphocytic infiltration, d - prominent stained nuclear component, e - multiple hazy nuclei with increased intercapsular space. (B): Photomicrograph of cerebral cortices stained with hematoxylin and eosin of Group II (β-sitosterol + Sham) showing: i) Left (occluded) cerebral cortex (×10), ii) Left (occluded) cerebral cortex (×40), iii) Right (non-occluded) cerebral cortex (×10), iv) Right (non-occluded) cerebral cortex (×40). (C): Photomicrograph of cerebral cortices stained with hematoxylin and eosin of Group III (β-sitosterol + LCCAO) showing; i) Left (occluded) cerebral cortex (×10), ii) Left (occluded) cerebral cortex (×40), iii) Right (non-occluded) cerebral cortex (×10), iv) Right (non-occluded) cerebral cortex (×40). (D): Photomicrograph of cerebral cortices stained with hematoxylin and eosin of Group IV (methanol extract + LCCAO) showing; i) Left (occluded) cerebral cortex (×10), ii) Left (occluded) cerebral cortex (×40), iii) Right (non-occluded) cerebral cortex (×10), iv) Right (non-occluded) cerebral cortex (×40).
5 Discussion
Brain tissue is highly vulnerable to ischemia. Even brief ischemia can trigger a complicated chain of events that could eventually lead to neuronal death (Woodruff et al., 2011). Excitotoxicity is one of the earliest discovered and widely recognized molecular mechanisms of post-ischemic neuronal damage and death. Glutamate and NMDAR (N-methyl-D-aspartate receptor) have an essential role in this mechanism (Shen et al., 2022). Additionally, the role of tau protein in excitotoxicity has been reported (Chen and Jiang, 2019).
Reduced cerebral blood flow during ischemia deranges the normal ionic homeostasis of neurons. The deranged ionic homeostasis depolarizes the neurons initiating a train of events, including the release of excitatory neurotransmitter glutamate into the synaptic cleft while impairing its re-uptake. Consequently, excess glutamate in the extracellular space causes the over-activation of NMDARs on the post-synaptic neurons (Wu and Tymianski, 2018). The excessively activated NMDARs allow uncontrolled Ca2+ influx causing calcium overload and triggering downstream pro-death signalling events that include activation of calpain, generation of reactive oxygen species (ROS), and mitochondrial damage, causing cell necrosis or apoptosis (Martin and Wang, 2010; Wu and Tymianski, 2018). Additionally, excitotoxicity causes tau hyperphosphorylation, significantly reducing its biological activity. There are reports of the critical role of tau in eliciting excitotoxicity. However, the molecular mechanisms underlying tau-induced excitotoxicity are yet to be elucidated (Chen and Jiang, 2019). Given the pivotal role of NMDARs and tau protein in mediating neuronal death in ischemic stroke, interventions directed at NMDAR and tau-mediated pathology could serve as a clinically beneficial approach in the prevention/management of ischemic stroke.
The present study aimed to evaluate the neuroprotective effect of pre-treatment with M. pruriens seed extract and its isolated bioactive molecule, β-sitosterol, in experimental animals subjected to cerebral ischemia by LCCAO. Additionally, the study explores the underlying molecular mechanisms of cerebral ischemia that include expression of NMDAR and Tau protein in experimental cerebral ischemia with or without pre-treatment with M. pruriens seed extract and its isolated bioactive molecule, β-sitosterol.
The current research involved in vitro and in vivo studies. In vitro studies aimed to screen the pharmacognostical and phytochemical profile of M. pruriens. For this, the shade-dried seeds of M. pruriens were subjected to sequential phytochemical extraction using different solvents, viz., methanol, ethanol, petroleum ether, chloroform, and water. The qualitative phytochemical analysis showed the presence of alkaloids, flavonoids, terpenoids, steroids, tannins, saponins and glycosides. Of the different solvents used for phytochemical extraction the methanol extract of M. pruriens seeds had a better profile than others. Hence, the present study proceeded with the methanol extract of M. pruriens seeds. The antioxidant activity of M. pruriens seed extract was demonstrated in vitro by its ability to scavenge DPPH radicals and hydrogen peroxide. Further, M. pruriens seed extract was subjected to HPLC and flash chromatography to identify and isolate its bioactive molecules. L-DOPA and β-sitosterol were identified as the predominant ones. L-DOPA is a predominant constituent in M. pruriens seed and has been used in neurodegenerative diseases like Parkinson’s disease (Rai et al., 2020). β-sitosterol was the next predominant bioactive molecule, estimated at approximately 12mg/100 gm. The present study aimed to explore the role of β-sitosterol in cerebral ischemia-induced neuropathology. β-sitosterol, a bioactive phytosterol, is naturally present in plant cell membranes with a chemical structure similar to mammalian cell-derived cholesterol (Babu and Jayaraman, 2020). β-sitosterol possesses an array of biological functions including antioxidant, antimicrobial, angiogenic, immunomodulatory, antidiabetic, anti-inflammatory, anticancer, and antinociceptive activities (Bin Sayeed et al., 2016).
The results of in vivo study revealed that pretreatment with M. pruriens seed extract and its specific bioactive molecule, β-sitosterol improved neurological deficit score, decreased ischemic brain damage and reduced the gene expression of NMDAR and tau protein in cerebral ischemia induced by LCCAO in experimental animals. The neuroprotective effects of M. pruriens seed extract and its isolated bioactive molecule, β-sitosterol, can be attributed to the downregulation of NMDAR and tau protein gene expression and the downstream signalling pathways, particularly the reactive oxygen species generation. There is increasing evidence that phytochemicals regulate gene expression by modulating the transcriptional activity of genes via specific transcriptional factors or epigenetic mechanisms (Surguchov et al., 2021). A previous study by Fung et al. (2014) demonstrated a cardioprotective effect of pretreatment with M. pruriens seed extract against experimental Naja Sputatrix envenomation due to upregulation of genes related to energy production and metabolism, nervous system, inflammatory response, and apoptosis, among several others in the rat heart. Additionally, the neuroprotective effect of M. pruriens seed extract and β-sitosterol may be partly attributed to their potent antioxidant properties. Since the brain has high oxygen consumption, it is susceptible to the damaging effects of reactive oxygen species generated during ischemia-reperfusion (Lalkovičová and Danielisová, 2016). Studies have reported that pre-treatment with synthetic antioxidants decreased the infarct size in animals subjected to ischemia/reperfusion injury (Duong et al., 2008).
Interestingly, the methanol extract of M. pruriens seeds was more effective than its isolated bioactive molecule, β-sitosterol. Medicinal plant extract may be superior to an equivalent dose of an isolated bioactive molecule. Plant extract contains hundreds or even thousands of different bioactive molecules in variable concentrations that may contribute to its medicinal value. The interaction between these multiple compounds may be synergistic, additive or antagonistic, which may be responsible for the overall activity of the medicinal plant extract (Vaou et al., 2022). L-DOPA was a predominant phytochemical in the seed extract of M. pruriens. L-DOPA might have contributed to better neuroprotection offered by M. pruriens seed extract compared to its isolated bioactive molecule β-sitosterol.
A significant neuroprotective activity of methanol extract of M. pruriens seeds and its isolated bioactive molecule, β-sitosterol suggest a potential for their use in the prevention/reducing the cerebral ischemia induced neuropathology.
6 Conclusion
Pre-treatment with methanol extract of M. pruriens and its bioactive molecule β-sitosterol confers a protection against cerebral ischemic injury by down regulating the gene expression of NMDAR and tau protein that mediate excitotoxicity.
Data availability statement
The raw data supporting the conclusion of this article will be made available by the authors, without undue reservation.
Ethics statement
The animal study was reviewed and approved by Institutional Animal Ethics Committee, Shri B M Patil Medical College, Hospital & Research Centre, BLDE (Deemed to be University), Vijayapura, Karnataka.
Author contributions
PP—acquisition, analysis, or interpretation of data for the work, wrote sections of the manuscript SMP—organized the database, BP— acquisition, analysis, or interpretation of data, wrote sections of the manuscript, RR—acquisition, analysis, or interpretation of data, performed the statistical analysis. IB—acquisition, analysis, or interpretation of data MK—organized the database, performed the statistical analysis SP—organized the database, performed the statistical analysis AP—contributed to the conception and design of the study and provided approval for the publication of the content KD—contributed to the conception and design of the study, provided approval for publication of the content SD—contributed to the conception and design of the study, provided approval for publication of the content SB—acquisition, analysis, or interpretation of data for the work, wrote the first draft of the manuscript. All authors contributed to the manuscript revision and read and approved the submitted version.
Funding
This work was supported by BLDE (Deemed to be University), Vijayapura, Karnataka, India (No DUPO05-0063/21-22 dated 25 Aug 2021).
Conflict of interest
The authors declare that the research was conducted in the absence of any commercial or financial relationships that could be construed as a potential conflict of interest.
Publisher’s note
All claims expressed in this article are solely those of the authors and do not necessarily represent those of their affiliated organizations, or those of the publisher, the editors and the reviewers. Any product that may be evaluated in this article, or claim that may be made by its manufacturer, is not guaranteed or endorsed by the publisher.
Supplementary material
The Supplementary Material for this article can be found online at: https://www.frontiersin.org/articles/10.3389/fphys.2023.1092032/full#supplementary-material
References
Ayaz M., Junaid M., Ullah F., Subhan F., Sadiq A., Ali G., et al. (2017). Anti-Alzheimer's studies on β-sitosterol isolated from Polygonum hydropiper L. Front. Pharmacol. 68, 697. doi:10.3389/fphar.2017.00697
Babu S., Jayaraman S. (2020). An update on β-sitosterol: A potential herbal nutraceutical for diabetic management. Biomed. Pharmacother. 131, 110702. doi:10.1016/j.biopha.2020.110702
Bin Sayeed M. S., Karim S. M. R., Sharmin T., Morshed M. M. (2016). Critical analysis on characterization, systemic effect, and therapeutic potential of beta-sitosterol: A plant-derived orphan phytosterol. Med. (Basel) 3 (4), 29. 15. doi:10.3390/medicines3040029
Brand-Williams W., Cuvelier M. E., Berset C. (1995). Use of a free radical method to evaluate antioxidant activity. LWT-Food Sci. Technol. 28 (1), 25–30. 1. doi:10.1016/S0023-6438(95)80008-5
Chen X., Jiang H. (2019). Tau as a potential therapeutic target for ischemic stroke. Aging (Albany NY) 11 (24), 12827–12843. 16. doi:10.18632/aging.102547
Das K. K., Yendigeri S. M., Patil B. S., Bagoji I. B., Reddy R. C., Bagali S., et al. (2018). Subchronic hypoxia pretreatment on brain pathophysiology in unilateral common carotid artery occluded albino rats. Indian J. Pharmacol. 50 (4), 185–191. doi:10.4103/ijp.IJP_312_17
Duong T. T., Antao S., Ellis N. A., Myers S. J., Witting P. K. (2008). Supplementation with a synthetic polyphenol limits oxidative stress and enhances neuronal cell viability in response to hypoxia-re-oxygenation injury. Brain Res. 1219, 8–18. doi:10.1016/j.brainres.2008.04.044
Fung S. Y., Sim S. M., Kandiah J., Armugam A., Aguiyi J. C., Tan N. H., et al. (2014). Prophylactic effect of Mucuna pruriens linn (velvet bean) seed extract against experimental Naja sputatrix envenomation: Gene expression studies. Indian J. Exp. Biol. 52 (9), 849–859.
Herrera R., Hemming J., Smeds A., Gordobil O., Willför S., Labidi J. (2020). Recovery of bioactive compounds from hazelnuts and walnuts shells: Quantitative-qualitative analysis and chromatographic purification. Biomolecules 10 (10), 1363. doi:10.3390/biom10101363
Herur A., Aithala M., Das K. K., Mallapur A., Hegde R., Kulkarni S. (2022). Interplay of oxidative stress and nitric oxide synthase gene expression on cardiovascular responses in preeclampsia. Rev. Bras. Ginecol. Obstet. 44 (3), 214–219. English. doi:10.1055/s-0042-1742313
Hussein R. A., El-Anssary A. A. (2018). “Plants secondary metabolites: The key drivers of the pharmacological actions of medicinal plants,” in Herbal medicine. Editor P. F. Builders (London: IntechOpen). doi:10.5772/intechopen.76139
Kalebar V. U., Hoskeri J. H., Hiremath S. V., Hiremath M. B. (2020). In-vitro cytotoxic effects of Solanum macranthum fruit. Dunal extract with antioxidant potential. Clin. Phytoscience 6, 24. doi:10.1186/s40816-020-00163-2
Kamkaen N., Chittasupho C., Vorarat S., Tadtong S., Phrompittayarat W., Okonogi S., et al. (2022). Mucuna pruriens seed aqueous extract improved neuroprotective and acetylcholinesterase inhibitory effects compared with synthetic L-dopa. Molecules 27 (10), 3131. 13. doi:10.3390/molecules27103131
Keser S., Celik S., Turkoglu S., Yilmaz O., Turkoglu I. (2012). Hydrogen peroxide radical scavenging and total antioxidant activity of hawthorn. Chem. J. 2 (1), 9–12.
Kim J., Fann D. Y., Seet R. C., Jo D. G., Mattson M. P., Arumugam T. V. (2016). Phytochemicals in ischemic stroke. Neuromolecular Med. 18 (3), 283–305. doi:10.1007/s12017-016-8403-0
Komatsu T., Ohta H., Motegi H., Hata J., Terawaki K., Koizumi M., et al. (2021). A novel model of ischemia in rats with middle cerebral artery occlusion using a microcatheter and zirconia ball under fluoroscopy. Sci. Rep. 11 (1), 12806. doi:10.1038/s41598-021-92321-w
Kong Z. L., Hsu Y. T., Johnson A., Tsai T. H., Miao S., He J. L., et al. (2021). Protective effects of Antrodia camphorata extract against hypoxic cell injury and ischemic stroke brain damage. Phytotherapy Res. 35 (3), 1609–1620. doi:10.1002/ptr.6928
Kshirsagar P. R., Pai S. R., Nimbalkar M. S., Gaikwad N. B. (2016). RP-HPLC analysis of seco-iridoid glycoside swertiamarin from different Swertia species. Nat. Prod. Res. 30 (7), 865–868. doi:10.1080/14786419.2015.1071366
Lalkovičová M., Danielisová V. (2016). Neuroprotection and antioxidants. Neural Regen. Res. 11 (6), 865–874. doi:10.4103/1673-5374.184447
Lampariello L. R., Cortelazzo A., Guerranti R., Sticozzi C., Valacchi G. (2012). The magic velvet bean of Mucuna pruriens. J. Tradit. Complement. Med. 2 (4), 331–339. doi:10.1016/s2225-4110(16)30119-5
Martin H. G., Wang Y. T. (2010). Blocking the deadly effects of the NMDA receptor in stroke. Cell 140 (2), 174–176. 22. doi:10.1016/j.cell.2010.01.014
Nayak V. S., Kumar N., D'Souza A. S., Nayak S. S., Cheruku S. P., Pai K. S. R. (2017). The effects of Mucuna pruriens extract on histopathological and biochemical features in the rat model of ischemia. Neuroreport 28 (18), 1195–1201. doi:10.1097/WNR.0000000000000888
Pathania R., Chawla P., Khan H., Kaushik R., Khan M. A. (2020). An assessment of potential nutritive and medicinal properties of Mucuna pruriens: A natural food legume. 3 Biotech. 10 (6), 261. doi:10.1007/s13205-020-02253-x
Pawar N., Pai S., Nimbalkar M., Dixit G. (2010). RP-HPLC analysis of phenolic antioxidant compound 6-gingerol from different ginger cultivars. Food Chem. 126 (3), 1330–1336. doi:10.1016/j.foodchem.2010.11.090
Rai S. N., Chaturvedi V. K., Singh P., Singh B. K., Singh M. P. (2020). Mucuna pruriens in Parkinson's and in some other diseases: Recent advancement and future prospective. 3 Biotech. 10 (12), 522. doi:10.1007/s13205-020-02532-7
Rane M., Suryawanshi S., Patil R., Aware C., Jadhav R., Gaikwad S., et al. (2019). Exploring the proximate composition, antioxidant, anti-Parkinson's and anti-inflammatory potential of two neglected and underutilized Mucuna species from India. South Afr. J. Bot. 124, 304–310. doi:10.1016/j.sajb.2019.04.030
Senguttuvan J., Paulsamy S., Karthika K. (2014). Phytochemical analysis and evaluation of leaf and root parts of the medicinal herb, Hypochaeris radicata L. for in vitro antioxidant activities. Asian Pac J. Trop. Biomed. 4 (1), S359–S367. doi:10.12980/APJTB.4.2014C1030
Shen Z., Xiang M., Chen C., Ding F., Wang Y., Shang C., et al. (2022). Glutamate excitotoxicity: Potential therapeutic target for ischemic stroke. Biomed. Pharmacother. 151, 113125. doi:10.1016/j.biopha.2022.113125
Siddhuraju P., Becker K. (2003). Antioxidant properties of various solvent extracts of total phenolic constituents from three different agroclimatic origins of drumstick tree (Moringa oleifera Lam.) leaves. J. Agric. Food Chem. 51 (8), 2144–2155. 9. doi:10.1021/jf020444+
Silva C. I., Novais P. C., Rodrigues A. R., Carvalho C. A., Colli B. O., Carlotti C. G., et al. (2017). Expression of NMDA receptor and microRNA-219 in rats submitted to cerebral ischemia associated with alcoholism. Arq. Neuropsiquiatr. 75 (1), 30–35. doi:10.1590/0004-282X20160188
Surguchov A., Bernal L., Surguchev A. A. (2021). Phytochemicals as regulators of genes involved in synucleinopathies. Biomolecules 11 (5), 624. 22. doi:10.3390/biom11050624
Theansungnoen T., Nitthikan N., Wilai M., Chaiwut P., Kiattisin K., Intharuksa A. (2022). Phytochemical analysis and antioxidant, antimicrobial, and antiaging activities of ethanolic seed extracts of four Mucuna species. Cosmetics 9 (1), 14. doi:10.3390/cosmetics9010014
Vaou N., Stavropoulou E., Voidarou C. C., Tsakris Z., Rozos G., Tsigalou C., et al. (2022). Interactions between medical plant-derived bioactive compounds: Focus on antimicrobial combination effects. Antibiot. (Basel) 11 (8), 1014. 28. doi:10.3390/antibiotics11081014
Woodruff T. M., Thundyil J., Tang S. C., Sobey C. G., Taylor S. M., Arumugam T. V. (2011). Pathophysiology, treatment, and animal and cellular models of human ischemic stroke. Mol. Neurodegener. 25 (1), 611. doi:10.1186/1750-1326-6-11
Keywords: Mucuna pruriens, β-sitosterol, left common carotid artery occlusion, NMDA receptor, tau protein, brain histopathology
Citation: Parvatikar PP, Patil SM, Patil BS, Reddy RC, Bagoji I, Kotennavar MS, Patil S, Patil AV, Das KK, Das SN and Bagali S (2023) Effect of Mucuna pruriens on brain NMDA receptor and tau protein gene expression in cerebral ischemic rats. Front. Physiol. 14:1092032. doi: 10.3389/fphys.2023.1092032
Received: 07 November 2022; Accepted: 06 February 2023;
Published: 16 February 2023.
Edited by:
Dewan S. Majid, Tulane University, United StatesReviewed by:
Badrinathan Sridharan, Pukyong National University, Republic of KoreaPrasunpriya Nayak, All India Institute of Medical Sciences Jodhpur, India
Copyright © 2023 Parvatikar, Patil, Patil, Reddy, Bagoji, Kotennavar, Patil, Patil, Das, Das and Bagali. This is an open-access article distributed under the terms of the Creative Commons Attribution License (CC BY). The use, distribution or reproduction in other forums is permitted, provided the original author(s) and the copyright owner(s) are credited and that the original publication in this journal is cited, in accordance with accepted academic practice. No use, distribution or reproduction is permitted which does not comply with these terms.
*Correspondence: Shrilaxmi Bagali, c2hyaWxheG1pLmJhZ2FsaUBibGRlZHUuYWMuaW4=