- Chronobiology Laboratory, School of Biology, Indian Institute of Science Education and Research (IISER), Thiruvananthapuram, Kerala, India
Circadian clocks temporally organize behaviour and physiology of organisms with a rhythmicity of about 24 h. In Drosophila, the circadian clock is composed of mainly four clock genes: period (per), timeless (tim), Clock (Clk) and cycle (cyc) which constitutes the transcription-translation feedback loop. The circadian clock is further regulated via post-transcriptional and post-translational mechanisms among which microRNAs (miRNAs) are well known post-transcriptional regulatory molecules. Here, we identified and characterized the role of miRNA-277 (miR-277) expressed in the clock neurons in regulating the circadian rhythm. Downregulation of miR-277 in the pacemaker neurons expressing circadian neuropeptide, pigment dispersing factor (PDF) advanced the phase of the morning activity peak under 12 h light: 12 h dark cycles (LD) at lower light intensities and these flies exhibited less robust rhythms compared to the controls under constant darkness. In addition, downregulation of miR-277 in the PDF expressing neurons abolished the Clk gene transcript oscillation under LD. Our study points to the potential role of miR-277 in fine tuning the Clk expression and in maintaining the phase of the circadian rhythm in Drosophila.
Introduction
Organisms have evolved with biological timekeeping systems to synchronize various biological processes with the cyclic environmental changes caused by earth’s rotation. The biological clock rhythmically regulates many aspects of metabolism, physiology and behaviour in diverse organisms. The clock genes that control the circadian rhythm were first identified in Drosophila and the fly circadian oscillatory mechanisms have mammalian counterparts (Konopka and Benzer, 1971; Sehgal et al., 1994; Allada et al., 1998; Gekakis et al., 1998; Rutila et al., 1998; Kume et al., 1999). The −24 h period of the endogenous clock is maintained by an interlocked transcription translation feedback loop (TTFL) (Hardin et al., 1990). The two basic helix-loop-helix transcription factors; CLOCK (CLK) and CYCLE (CYC) form a heterodimer and binds to the E-box located at the promoter region of the genes period (per) and timeless (tim) in Drosophila (Taylor and Hardin, 2008). PER and TIM form a heterodimer and upon increased accumulation, this heterodimer enters the nucleus and binds to CLK-CYC dimer through a PER-CLK interaction (Lee et al., 1999). This inhibits the transcription of per and tim by repressing the CLK-CYC transcriptional activity (Menet et al., 2010). This TTFL occurs within a span of approximately 24 h and ensures robust circadian oscillation of clock gene transcripts and their proteins.
Zeitgebers are the cyclic environmental time cues capable of entraining the circadian clock, amongst which, light is considered to be the most potent zeitgeber for many organisms (Schlichting and Helfrich-Förster, 2015). Blue light photoreceptor CRYPTOCHROME (CRY), the compound eyes, ocelli and Hofbauer-Buchner (HB) eyelets are involved in the light mediated clock entrainment in Drosophila (Rieger et al., 2003; Veleri et al., 2007; Yoshii et al., 2016). CRY is present in a subset of the clock neurons as well as in the photoreceptor cells and resets the phase of the clock by leading to the degradation of TIM in a light-dependent manner (Ceriani et al., 1999; Busza et al., 2004). The compound eyes, ocelli and HB eyelets express a variety of rhodopsins (RH1-7), each with distinct spectral sensitivity (Sharkey et al., 2020). The clock neurons, particularly the pigment dispersing factor (PDF)-positive ventral lateral neurons (LNvs), which are responsible for the morning peak (M peak) of activity, have dendritic connections projecting to the accessory medullae (AMe) where they communicate directly with the projections from the HB eyelets and indirectly with the compound eyes via the interneurons (Helfrich-Förster et al., 2002; Li et al., 2018). In addition, the interaction between PDF-positive small ventral lateral neurons (sLNvs) and posterior dorsal neurons (DN1ps) plays an important role in governing the morning anticipatory activity under 12 h light: 12 h dark cycles (LD). The presence of either of these neuronal subsets is sufficient to drive morning anticipation (Stoleru et al., 2004; Zhang et al., 2010). The PDF neuropeptide is critical for proper timing of locomotor activity under LD and for maintenance of rhythm under constant darkness (DD) (Renn et al., 1999). In addition to PDF-positive neurons, the dorsal lateral neurons (LNds), which regulate the evening peak (E peak) of activity also have anatomical connections to the AMe (Helfrich-Förster et al., 2007).
Though the clock neuronal circuitry, as well as the clock gene transcript/protein oscillations are well studied, additional regulatory mechanisms which confer robustness to these circadian oscillations are not well understood. The importance of microRNA (miRNA) mediated posttranscriptional regulation in circadian rhythm has been recognized in previous studies (Kadener et al., 2009). miRNAs are endogenous small (19–25 nucleotides long) noncoding RNAs that repress the translation of mRNA or degrade it by forming an imperfect hybrid at the 3′ untranslated region (3′UTR) of the mRNAs (Carthew and Sontheimer, 2009). Recent studies indicate that posttranscriptional regulation by miRNAs has a potential role in maintaining the robustness of the circadian clock as well as in the proper phasing of the rhythm (Herranz and Cohen, 2010; Ebert and Sharp, 2012; Xue and Zhang, 2018). Although several miRNAs are expressed in clock neurons (Kadener et al., 2009), only a few miRNAs among these were examined systematically in Drosophila to elucidate their specific roles in maintaining the circadian rhythm (Kadener et al., 2009; Luo and Sehgal, 2012; Vodala et al., 2012; Chen et al., 2014; Sun et al., 2015; Garaulet et al., 2016; Zhang et al., 2016; Chen and Rosbash, 2017; Cusumano et al., 2018; Nian et al., 2019; Niu et al., 2019; Xia et al., 2020; Nian et al., 2022).
Our present study was aimed at elucidating the role of miRNA-277 (miR-277) in governing the circadian rhythm in Drosophila. Downregulation of miR-277 in the PDF positive neurons advanced the phase of circadian activity-rest rhythm under LD and amplitude under DD. miR-277 expressed in PDF-positive neurons is demonstrated to be important for proper activity distribution around the morning and evening peak under lower light intensities of LD. In addition, the diurnal oscillation of Clk mRNA was found to be dependent on the optimal expression level of miR-277 in the fly head.
Materials and methodology
Fly lines and maintenance
All the stocks were maintained in a temperature and humidity-controlled incubator (Panasonic MIR-154) at 25°C and 60% ± 5% relative humidity. LD light schedule was maintained in the incubators with a light intensity of 400 lux where the lights came on at Zeitgeber Time 00 (ZT00) and went off at ZT12. The Gal4 (G4) lines used were tubulin G4, tim G4/CyO, Clk856 G4 (Orie Shafer Lab), Pdf G4 (Todd C Holmes Lab). miRNA sponge (SP) line used was the UAS miR-277 Sponge (BL- 61408). This line has multiple (20X) miRNA complementary sequences inserted into specific sites on the 2nd and 3rd chromosomes by phiC31 site-specific integration. Double scrambled sponge (Scrambled Sponge) was used as the control for the sponge line (Fulga et al., 2015). This line also has similar repeats of sequences inserted into the same sites but the sequences are not complementary to any known miRNA. miR-277 was overexpressed using UAS miR-277 (Fly ORF–F002059). The w1118 (BL-5905) was crossed with G4 (driver) and UAS (responder) lines to obtain the respective controls.
Locomotor activity rhythm recording and analysis
Freshly emerged male flies were entrained under LD for 2–3 days before carrying out the locomotor activity-rest rhythm recording. Activity recording of 2–3 day old male flies was carried out under LD at 25°C in a cooled incubator (Panasonic MIR-154) using Drosophila Activity Monitor (Trikinetics, Waltham, MA) with 1 min bin interval. In order to analyze the locomotor activity under LD, the activity of each fly at every 15 min was normalized to the total activity of the day, multiplied by 100 and the average of 28–32 flies for 5 days was plotted as the percentage of activity against the time of the day. The morning and evening anticipation indices were calculated using the equation: sum of activity 3 h prior to light transition/sum of activity 6 h prior to light transition (Sheeba et al., 2010). The phase of the morning peak (M peak) was determined for individual flies by identifying the time point of highest activity between ZT20–02 excluding the startle response around ZT00 (Das et al., 2016). The time window of up to ZT02 was chosen because previous studies showed that the M peak of most of the wild-type strains of Drosophila occurs after lights-on (Helfrich-Förster, 2000). The rhythm parameters of the clock were estimated by recording the locomotor activity under constant darkness (DD) for 10 days. Since miR-277 is reported to have effects on lifespan and ageing (Esslinger et al., 2013), we started the locomotor activity recording with 1-day-old flies, which were entrained for 2 days under LD and then shifted to DD, so that the age of the flies is 13 days by the end of the DD recording. In this way, we ensured that the effect of the microRNA on the free-running period is not influenced by its role in ageing. The free-running period was estimated using Lomb-Scargle periodogram analysis using Actimetrics Clocklab software. The amplitude of the peak corresponding to the free running period value in the periodogram was used to measure the robustness of the rhythm. Flies whose peak value occurred below the threshold of significance level were considered arrhythmic and were not included in the analysis for the estimation of FRP and amplitude of the rhythm.
mRNA isolation and quantification
Five-day old males entrained to LD were used for mRNA isolation. Three biological replicates each with 30 flies were sampled at 6 different time points ZT02, ZT06, ZT10, ZT14, ZT18, and ZT22. 30 fly heads were homogenized by crushing in 1 mL TRIzol reagent (Life Technologies), after which 0.2 mL of chloroform (Sigma-Aldrich) was added and centrifuged at 12,000 rpm for 10 min at 4°C to separate the RNA into the top aqueous layer. The separated aqueous phase containing genetic material was pipetted out and precipitated with 100% Isopropanol (Sigma). After DNase (Invitrogen) treatment and ethanol washes, the precipitate was redissolved in DEPC-treated water (Himedia). cDNA was synthesized from the total RNA using PrimeScript 1st strand cDNA synthesis kit (Takara Bio) following the manufacturer’s instructions. qPCR was performed on Bio-Rad CFX96 Touch Real-Time PCR detection system using TB Green Premix Ex Taq II (Takara) and the housekeeping gene rp49 was used as the reference gene. cry, per, tim, and Clk, mRNA values shown are relative to rp49 mRNA. The primer sequences used are listed in Supplementary Table S1.
TaqMan miRNA assay
The total RNA was isolated from the samples plunged at ZT02 as mentioned in the previous section. Reverse transcription was performed with High-Capacity cDNA Reverse Transcription Kit (Applied Biosystems) using miR-277 (Applied Biosystems, Assay ID-000298) specific primer following manufacturer’s instruction. 2s rRNA (Applied Biosystems, Assay ID-001766) was used as the endogenous control. The resulting cDNA was used for qRT-PCR based quantification of the miRNA. TaqMan Universal Master Mix II, with UNG (Applied Biosystems) was used along with miR-277 or 2s rRNA specific mix of forward primer, reverse primer and TaqMan probe, as per the manufacturer’s instruction. miRNA level was normalized to the 2s rRNA level to obtain the ΔCt value.
Statistical analysis
The phase of the morning peak, free running period and amplitude of DD activity levels were analyzed for normal distribution by the Shapiro-Wilk test. Depending on the result, ANOVA was performed, followed by Tukey’s post hoc multiple comparisons (for normally distributed data) or Kruskal Wallis test was performed, followed by Dunn’s post hoc test (for data that are not normally distributed) to estimate the significant differences between different genotypes. Results were considered statistically significant when p < 0.05. The graphs were plotted with mean and error bars representing the SEM. Plotting as well as statistical tests were performed using GraphPad Prism. All the locomotor activity experiments were conducted thrice with a sample size of 28–32 flies to confirm the results. The results of one such biological replicate are shown in the figures.
The transcript level values were determined by normalizing them with the respective rp49. The values plotted are the mean and SEM of data obtained from 3 biological replicates. The gene expression data obtained by qPCR were analysed for cycling by fitting the expression values at different time points to a sine wave. The cosinor analysis was performed in MATLAB to estimate the peak phase of relative mRNA expression (Nelson et al., 1979). The mRNA is considered to be cycling when the p < 0.05. The peak phase values obtained from individual biological replicates by cosinor analysis were further tested statistically by t-test to confirm if there is any significant difference in peak phase between the genotypes.
Results
Downregulation of miR-277 in the PDF neurons advances the phase of the morning peak
To understand the functional significance of miR-277 expressed in the clock neuron, we downregulated miR-277 in the clock neurons using tim G4 driver and UAS miR-277 Sponge (SP) line. The miR-277 SP line used in this study was obtained from BDSC and has been used in several previous studies (Cerro-Herreros et al., 2016; Zipper et al., 2022). These studies report that this sponge line significantly reduces the miR-277 levels. Under LD with a light intensity of 400 lux, the miR-277 downregulated flies exhibited an increased activity prior to lights-on at ZT23 (Figure 1A). This is possible when there is a phase advance in the morning peak, which was assessed by calculating the phase of the morning peak. It was found that the phase of the M peak was advanced with respect to that of the G4 control and Scrambled Sponge control (Scrambled SP); however, there was no significant advancement with respect to that of the UAS miR-277 SP control (Figure 1B). In addition, miR-277 downregulated flies exhibited an increase in activity after lights-off (ZT13.75–14.50) (Figure 1A). The locomotor activity rhythm of the flies was also recorded under DD to assess the role of miR-277 in the regulation of the free-running period (FRP) and amplitude of the circadian rhythm. Though FRP of the experimental line was not significantly different from that of the controls (Supplementary Figure S1A), the amplitude of DD activity was found to be significantly decreased in the experimental flies (Figure 1C). This suggests that miR-277 present in the clock neuronal circuit helps in maintaining robust rhythms under DD.
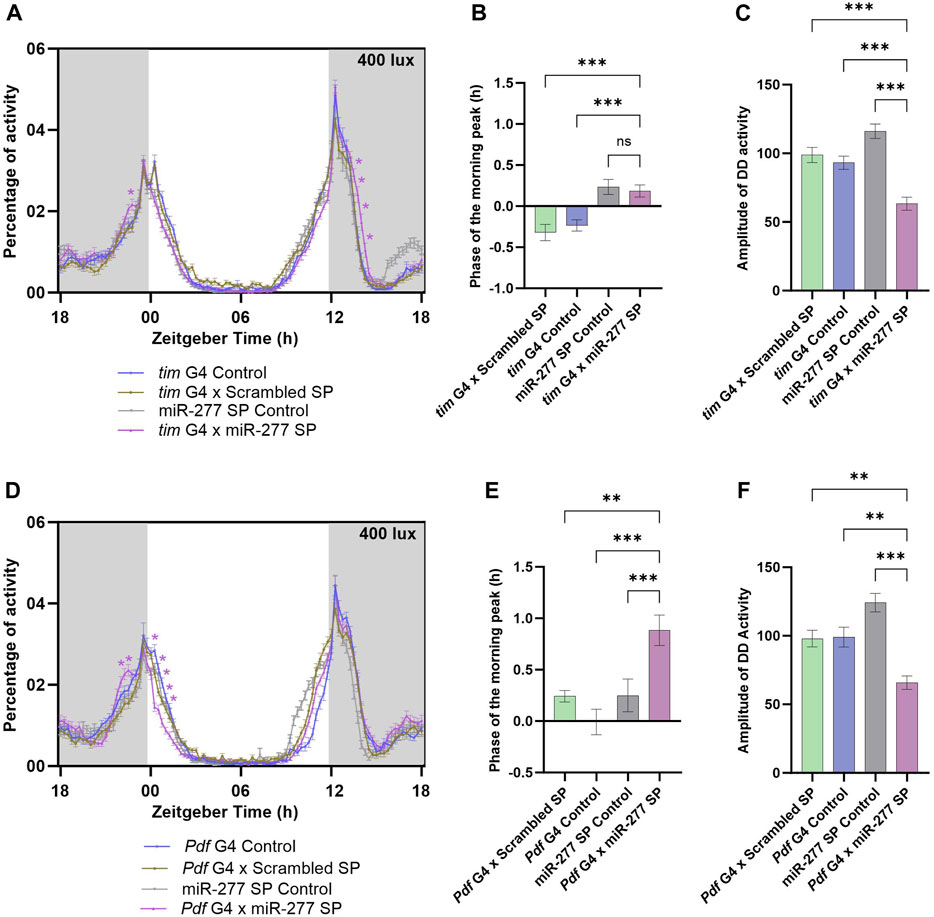
FIGURE 1. Downregulation of miR-277 in the PDF neurons advances the phase of the morning peak. (A) Activity rest rhythm of tim G4 x miR-277 SP compared to tim G4 control, tim G4 x Scrambled SP and UAS miR-277 control under LD. Locomotor activity counts were binned into 15 min intervals and percentage of activity was plotted along the y-axis and Zeitgeber Time in hours along the x-axis. Gray shaded region indicates dark phase under LD. The light intensity was maintained at 400 lux. The asterisks indicate the time points at which the experimental flies showed a significant difference compared to all the three controls. A significant increase in activity was observed at ZT 23 compared to the controls. (Two way ANOVA followed by Tukey’s Honestly Significant Difference (HSD) test. p < 0.05 for time point ZT23). The activity around Evening peak (E peak) also showed significant differences compared to the controls (Two way ANOVA, Tukey’s HSD test. p < 0.05 for time points ZT13.75- ZT14.50). (B) The phase of the morning peak of activity of the tim G4 x Scrambled SP, tim G4 control, UAS miR-277 control and tim G4 x miR-277 SP under LD. A positive value indicates phase advancement and negative value indicates phase delay. Though the phase was significantly advanced in tim G4 x miR-277 SP line compared to two controls, it was not significantly different from that of the UAS miR-277 SP control (Kruskal Wallis test followed by Dunn’s post hoc multiple comparisons. tim G4 x Do. Scr. Sponge vs. tim G4 x miR-277 Sponge p < 0.001, tim G4 Control vs. tim G4 x miR-277 Sponge p < 0.001, UAS miR-277 SP control vs. tim G4 x miR-277 SP p < 0.73). (C) Amplitude of the activity rest rhythm under DD was found to be decreased in tim G4 x miR-277 SP when compared to the controls (ANOVA, Tukey’s HSD test. tim G4 x Scrambled SP vs. tim G4 x miR-277 SP p < 0.001, tim G4 Control vs. tim G4 x miR-277 Sponge p < 0.001, UAS miR-277 SP Control vs. tim G4 x miR-277 SP p < 0.001). (D) Activity-rest rhythm under LD showing increased activity in the time points prior to lights-on in Pdf G4 x miR-277 SP compared to the controls (Two way ANOVA, Tukey’s HSD test. p < 0.05 for time points ZT22.5 and ZT22.75). (E) Phase of the morning peak of activity showing significant advance in Pdf G4 x miR-277 SP compared to the controls (Kruskal Wallis, Dunn’s post hoc multiple comparisons. Pdf G4 x Scrambled SP vs. Pdf G4 x miR-277 SP p < 0.004, Pdf G4 Control vs. Pdf G4 x miR-277 SP p < 0.001, UAS miR-277 SP control vs. Pdf G4 x miR-277 SP p < 0.001). (F) Amplitude of the rhythm under DD showing significant decrease in Pdf G4 x miR-277 SP compared to the controls (ANOVA, Tukey’s HSD test. Pdf G4 x Scrambled SP vs. Pdf G4 x miR-277 SP p < 0.002, Pdf G4 Control vs. Pdf G4 x miR-277 Sponge p < 0.002, UAS miR-277 SP control vs. Pdf G4 x miR-277 SP p < 0.001).
The PDF positive LNvs and DN1ps are the circadian neurons that regulate the morning locomotor activity behaviour in Drosophila (Grima et al., 2004; Stoleru et al., 2004; Zhang et al., 2010). As miR-277 downregulation in clock neurons was found to have an effect on the activity prior to the lights-on, we further restricted the downregulation of miR-277 to PDF neurons. Under LD, the morning activity was increased prior to lights-on (ZT22.5 and 22.75) in the experimental flies compared to the controls (Figure 1D). The phase of the morning peak was also found to be advanced in the experimental flies (Figure 1E). The miR-277 downregulated flies exhibited significantly reduced amplitude of activity rest rhythm under constant darkness (Figure 1F). This reduction in amplitude was observed only when DD recording was conducted using younger (3 day old) flies and not when 9 day old flies were used (Data not shown). However, the FRP of the rhythm was not affected in these flies (Supplementary Figure S1B). Thus, we conclude that miR-277 present in the PDF neurons functions in the proper phasing of the morning peak of activity and maintains robustness of the circadian rhythm under DD in Drosophila.
Overexpression of miR-277 in the clock neurons advances the morning peak phase
The efficiency of the overexpression line used in this study was tested by TaqMan miRNA assay. The miR-277 overexpression line showed a significant increase in miR-277 level when compared to the tubulin G4 and miR-277 OE Controls (Supplementary Figure S1C). The effect of overexpression of miR-277 in the clock neurons was studied by using tim G4 to drive the expression of miR-277. Overexpression of miR-277 using tim G4 resulted in an increased activity prior to lights-on (ZT22.75–23.75) under LD (Figure 2A). The phase of the morning peak was found to be advanced upon phase calculation (Figure 2B). These flies also exhibited an increase in the activity prior to lights-off (ZT10.25–12) (Figure 2A). The FRP and amplitude of these flies were estimated under DD. It was found that the FRP of the experimental flies was significantly increased compared to UAS control and significantly decreased compared to the G4 control, hence no conclusion could be drawn regarding the effect of overexpression in the clock neurons (Supplementary Figure S1D). The amplitude of the rhythm was also not different from that of the controls (Supplementary Figure S1E). The overexpression of miR-277 was then carried out in the PDF positive neurons using Pdf G4. This too resulted in an increased activity prior to lights-on (ZT22.25–23.25) (Figure 2C) and the phase of the morning peak was also found to be significantly advanced in the experimental flies compared to both the controls (Figure 2D). However, overexpression of miR-277 in PDF positive clock neurons did not exert any significant influence on the activity prior to or after lights-off (Figure 2C). The FRP of the miR-277 overexpressed flies was found to be significantly increased and decreased compared to the UAS control and Pdf G4 control respectively, which prevented us from drawing any meaningful conclusion (Supplementary Figure S1F). We did not observe any significant difference in the amplitude of activity-rest rhythm under DD compared to the controls (Supplementary Figure S1G). These results suggest that miR-277 expressed in the clock neurons is involved in the phasing of the morning peak.
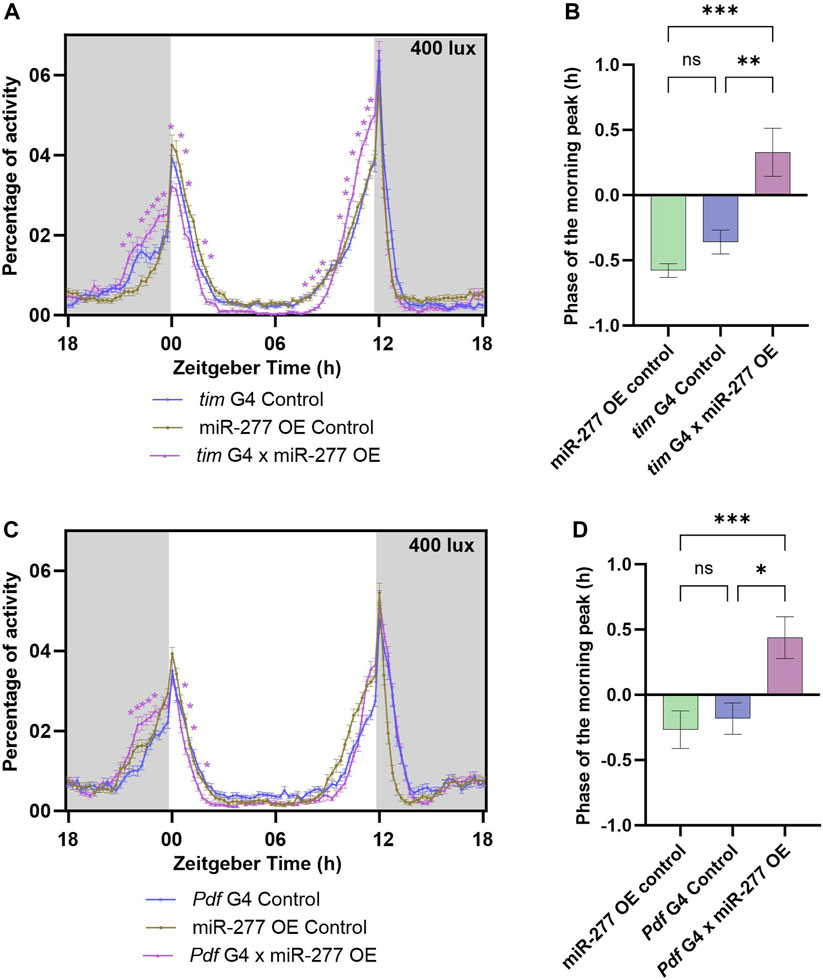
FIGURE 2. Overexpression (OE) of miR-277 in the clock neurons affects the phasing of the morning peak of activity-rest rhythm. (A) Activity rest rhythm of tim G4 x miR-277 OE flies compared to tim G4 control and miR-277 OE control. miR-277 overexpressed flies exhibited increased activity prior to lights on (Two way ANOVA, Tukey’s HSD test. p < 0.05 for time points ZT22.75- ZT23.75). The time points around the E peak also showed significant difference in activity (Two way ANOVA, Tukey’s HSD test. p < 0.05 for time points ZT10.25–12). The asterisks indicate the time points at which the experimental flies showed a significant difference compared to both the G4 and UAS control. (B) The phase of morning peak of the three genotypes under LD. The miR-277 overexpressed flies showed a significant advance in the phase of morning peak compared to the tim G4 and miR-277 OE controls (Kruskal Wallis test, Dunn’s post hoc multiple comparisons. miR-277 OE control vs. tim G4 x miR-277 OE p < 0.001, tim G4 Control vs. tim G4 x miR-277 OE p < 0.01). (C) Activity-rest rhythm of Pdf G4 x miR-277 OE flies compared to Pdf G4 control and miR-277 OE control flies. Pdf G4 x miR-277 OE flies have increased activity prior to lights-on (Two way ANOVA, Tukey’s HSD test. p < 0.05 for time points ZT22.25–23.25). (D) Phase of the morning peak of activity showing a significant advance in the Pdf G4 x miR-277 OE flies compared to Pdf G4 control and miR-277 OE control (Kruskal Wallis test, Dunn’s post hoc multiple comparisons. miR-277 OE control vs. Pdf G4 x miR-277 OE p < 0.001, Pdf G4 Control vs. Pdf G4 x miR-277 OE p < 0.01).
Role of miR-277 in phasing of the morning peak under different light intensities
Since the activity around lights-on is altered when miR-277 was downregulated in the PDF neurons, we decided to check how different light intensities might impact the activity-rest rhythm. To this end, we downregulated miR-277 in the PDF neurons and recorded their activity rest rhythm under LD with three different light intensities viz 10 lux, 100 lux and 1000 lux. Under 10 lux, we observed that the experimental flies failed to have a proper M peak of activity (Figure 3A). Instead, they showed a sustained increase in activity starting nearly 2 hours before lights-on (ZT22.25–23.05). Besides, these flies exhibited a significant reduction in the activity immediately after lights-on and the evening activity onset was delayed (Supplementary Figure S1H). However, the phase of the M peak was not significantly advanced with respect to the UAS miR-277 Sponge control (Figure 3B). Under 100 lux, miR-277 downregulated flies exhibited significant increase in activity at some time points before lights-on (ZT22.75 and 23) (Figure 3C). Upon phase calculation, the M peak was found to be significantly advanced with respect to the controls (Figure 3D). In addition to the altered activity around the M peak, the experimental flies exhibited a significantly decreased activity before lights-off and increased activity after the lights-off under both 10 lux and 100 lux. But no significant difference was found in E activity onset under 100 lux (Figure 3A, C, Supplementary Figure S1I). When we further increased the light intensity to 1000 lux, experimental flies showed a significant increase in activity at ZT 22.75 but decreased activity at ZT 0.50 compared to that of the control flies (Figure 3E). The miR-277 downregulated flies did not exhibit any significant difference in the phase of the morning peak under high light intensity compared to the controls (Figure 3F). The evening activity onset also was not significantly different from the controls (Supplementary Figure S1J). These results suggest that miR-277 present in the PDF neurons is critical for the proper activity distribution around the M peak at various light intensities as well as in the activity regulation around E peak under lower light intensities.
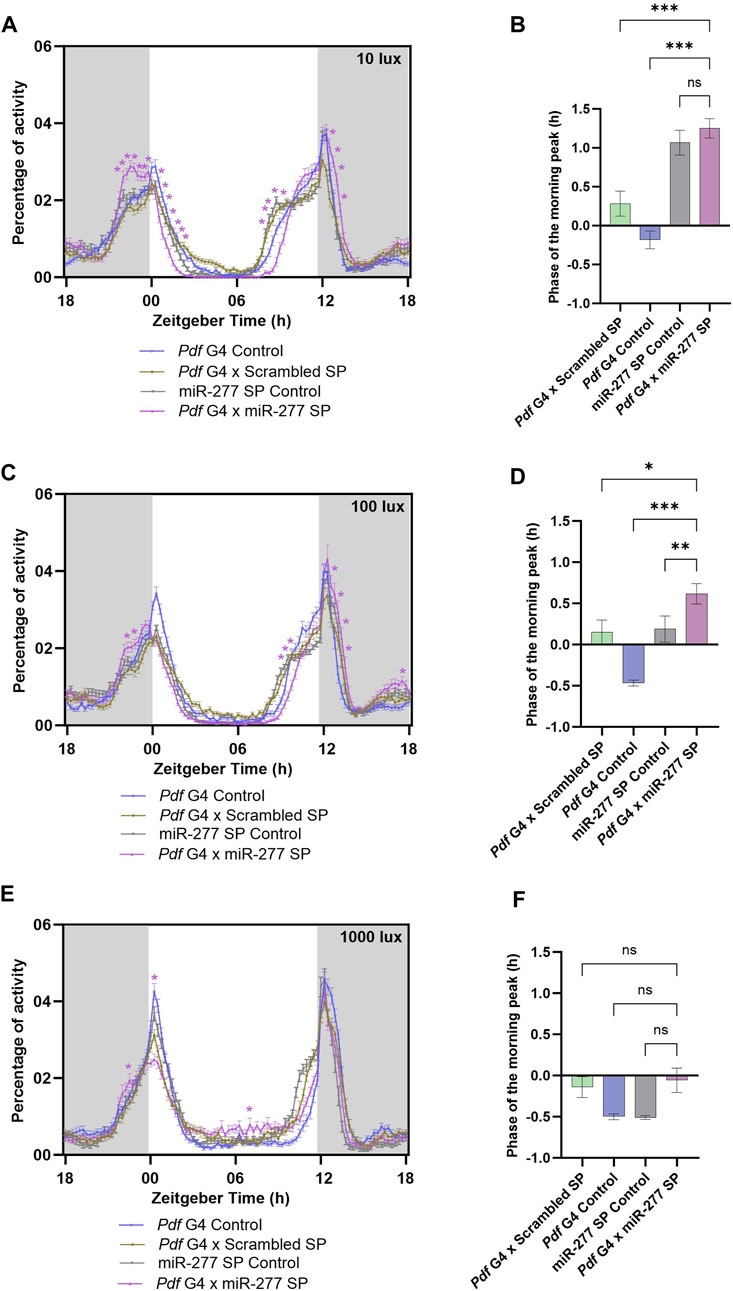
FIGURE 3. (A, C, E) Activity-rest rhythm of Pdf G4 control, Pdf G4 x Scrambled SP, UAS miR-277 SP control and Pdf G4 x miR-277 SP flies under LD with 10 lux, 100 lux and 1000 lux intensities respectively. Pdf G4 x miR-277 SP flies exhibited a significantly increased activity prior to lights-on compared to Pdf G4 control and Pdf G4 x Scrambled SP control under 10 and 100 lux of light intensities (Two way ANOVA, Tukey’s HSD test. p < 0.05 for time points ZT22.25-ZT23.50 under 10 lux. p < 0.05 for time points ZT22.75 and ZT23 under 100 lux). The activity around the E peak also was significantly altered (Two way ANOVA, Tukey’s HSD test. p < 0.05 for time points ZT08.50–09.50 and ZT12.75–13.50under 10 lux, p < 0.05 for time points ZT09.75–10.25 and ZT13.25–13.75 under 100 lux). Under 1000 lux light intensity, Pdf G4 x miR-277 SP flies showed a significant increase in the locomotor activity compared to the controls at ZT 22.75 but a decreased activity at ZT00 and ZT00.25 (Two way ANOVA, Tukey’s HSD test. p < 0.05 for time points ZT22.75, ZT00 and ZT00.25) (B, D) Under 10 lux, the phase of the morning peak of activity was significantly advanced in Pdf G4 x miR-277 SP line compared to two of the controls, but not with respect to the UAS miR-277 SP control (Kruskal Wallis, Dunn’s post hoc multiple comparisons. Pdf G4 x Scrambled SP vs. Pdf G4 x miR-277 SP p < 0.001, Pdf G4 Control vs. Pdf G4 x miR-277 SP p < 0.001, UAS miR-277 SP control vs. Pdf G4 x miR-277 SP p < 0.99). Under 100 lux, the experimental line showed a significant advance in phase of the M peak compared to the three controls (Kruskal Wallis, Dunn’s post hoc multiple comparisons. Pdf G4 x Scrambled SP vs. Pdf G4 x miR-277 SP p < 0.01, Pdf G4 Control vs. Pdf G4 x miR-277 SP p < 0.001, UAS miR-277 SP control vs. Pdf G4 x miR-277 SP p < 0.01). (F) Under 1000 lux light intensity, the phase of morning peak of Pdf G4 x miR-277 SP line does not exhibit any significant difference compared to that of the controls (Kruskal Wallis, Dunn’s post hoc multiple comparisons. Pdf G4 x Do. Scr. Sponge vs. Pdf G4 x miR-277 Sponge p = 0.99, Pdf G4 Control vs. Pdf G4 x miR-277 Sponge p = 0.99, UAS miR-277 SP control vs. Pdf G4 x miR-277 SP p = 0.99).
Downregulation of miR-277 in the PDF neurons abolishes Clk mRNA oscillation
In order to test whether the effect of miR-277 on the phasing of the morning peak is mediated by CRY, we downregulated miR-277 in the PDF neurons and the mRNA level of cry was analysed at 6 different time points across the day with 4h interval. The cry was found to oscillate in the controls as well as in the experimental fly heads with a peak around ZT04 (Figure 4A). The mRNA level of cry at ZT06 was found to be significantly higher in miR-277 downregulated flies compared to the controls (Figure 4A). This might be suggestive of the role of miR-277 in regulating the cry transcript levels in Drosophila under LD.
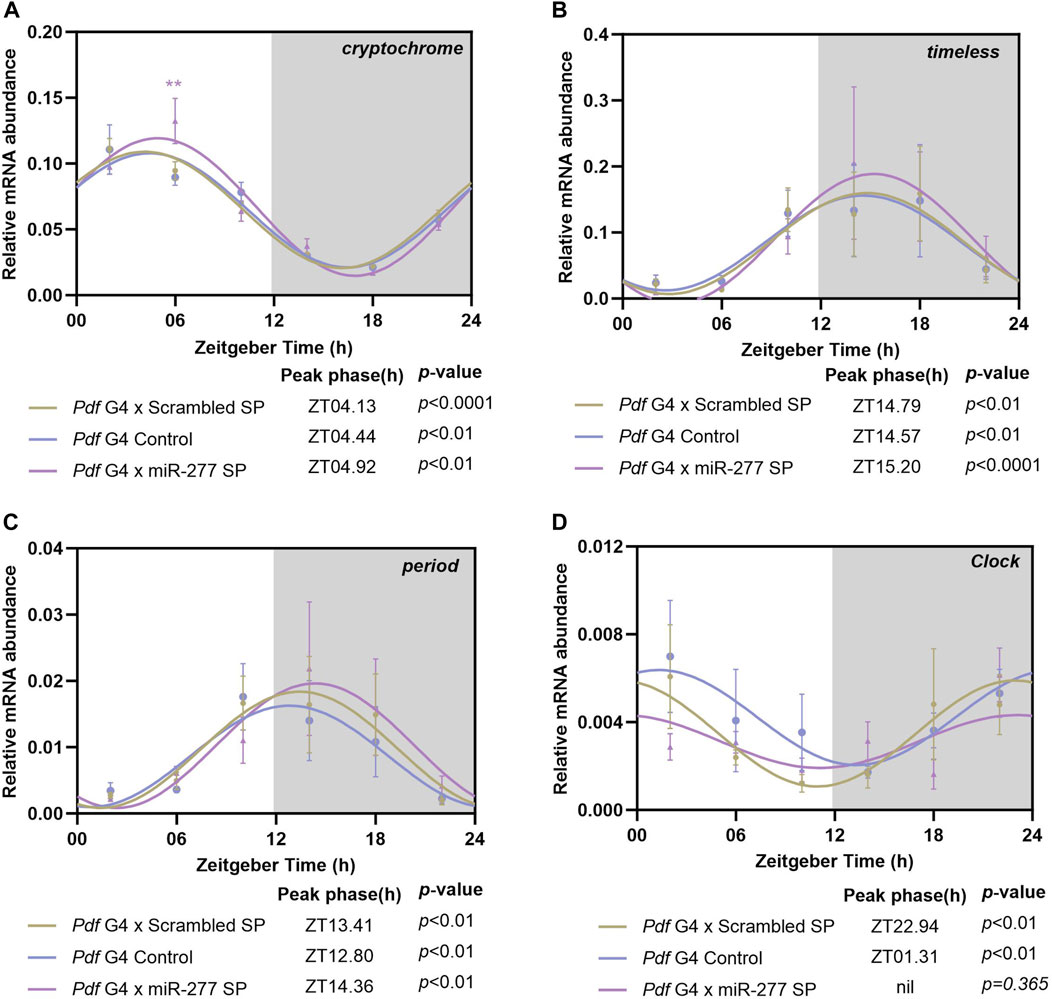
FIGURE 4. Transcript oscillation of various clock genes in the whole head extract of flies with miR-277 downregulation in the PDF neurons under LD. Transcripts were analysed at 6 different time points across the day with 4 h intervals. y-axis represents the transcript value of the clock genes normalised to the respective rp49 value at each time point. Three biological replicates were used for each time point and mean value was plotted with the SEM as the error bar. The values at these time points were fitted into a sine wave to obtain the rhythm parameters such as acrophase, amplitude, etc., p-value less than 0.05 indicates oscillation of the gene. Grey shaded region indicates the dark phase under LD. (A) cryptochrome (cry) transcript level exhibits significant oscillation with peak occurring between ZT04-ZT05 in the experimental as well as the controls (Cosinor analysis. Pdf G4 x Scrambled SP p < 0.001, Pdf G4 Control p < 0.01, Pdf G4 x miR-277 Sponge p < 0.01). The cry transcript level in the experimental fly heads at ZT06 was found to be significantly increased compared to the controls (Two way ANOVA, Tukey’s HSD test. Pdf G4 x Scrambled SP vs. Pdf G4 x miR-277 SP p < 0.01, Pdf G4 Control vs. Pdf G4 x miR-277 SP p < 0.001). (B, C) timeless (tim) and period (per) transcript oscillation in the Pdf G4 x miR-277 SP fly heads compared to Pdf G4 control and Pdf G4 x Scrambled SP control under LD. Both the transcripts exhibit significant cycling with peak between ZT12-15 in the experimental as well as in the control flies. (Cosinor analysis for tim. Pdf G4 x Scrambled SP p < 0.01, Pdf G4 Control p < 0.01, Pdf G4 x miR-277 SP p < 0.001. Cosinor analysis for per. Pdf G4 x Scrambled SP p < 0.01, Pdf G4 Control p < 0.01, Pdf G4 x miR-277 Sponge p < 0.01). (D) Control flies exhibited significant oscillation of Clk mRNA with peak between ZT23-ZT02. However, Clk mRNA diurnal oscillation was abolished in the fly head when miR-277 was downregulated in the PDF expressing clock neurons (Cosinor analysis. Pdf G4 x Scrambled SP p < 0.01, Pdf G4 Control p < 0.01, Pdf G4 x miR-277 SP p = 0.365).
To understand the effect of downregulation of miR-277 in the PDF neurons on the core clock machinery, the mRNA oscillations of tim and per were analysed. Both tim and per mRNAs showed oscillations when miR-277 was downregulated in the PDF neurons (Figures 4B, C), with the peak of the occurring between ZT12-15 in the experimental as well as the control fly heads. We further analysed the transcript oscillation of Clk and it was found that both Gal4 and scrambled sponge controls exhibit oscillations with a peak close to lights-on (Supplementary Figure S1K). However, to our surprise, we found that the Clk mRNA oscillation is abolished in the fly head when miR-277 is downregulated in the PDF neurons (Figure 4D). Thus, we find that presence of miR-277 is critical for the maintenance of oscillating Clk mRNA levels. However, the abolishment of the Clk oscillation was not found to affect the tim and per oscillation.
Discussion
Although miRNAs are important regulators of diverse physiological processes, our understanding of their potential roles in maintaining proper clock function is still limited. Recent studies showed that miRNAs are involved in maintaining the phase, amplitude and period of the circadian clock (Kadener et al., 2009; Mehta and Cheng, 2013; Chen et al., 2014; Garaulet et al., 2016; Xue and Zhang, 2018). In the present study, we assessed the role of miR-277 in regulating the circadian time keeping in Drosophila. In order to investigate the role of miR-277 in the clock tissues including the PDF positive clock neurons, miR-277 was downregulated using tim G4 and Pdf G4. Under LD, downregulation of miR-277 in the entire clock neuronal circuit under tim G4 resulted in an increased activity prior to lights-on at ZT23. These flies also exhibited some increased activity after lights-off. Under DD, the robustness of the rhythm was found to be reduced. Downregulation of miR-277 in the PDF-positive neurons, the principal regulators of the M peak, resulted in the advancement of the morning peak phase by about 40 min. The amplitude of the rhythm was reduced in the experimental flies indicating the importance of miR-277 in maintaining robust rhythms under DD. However, we did not find any significant difference in the free-running period of these flies indicating that the phase advancement observed in the experimental flies is not due to a change in the period of the circadian clock. miR-124 KO flies are known to have a similar effect, where the circadian phase is advanced without any change in the circadian period (Zhang et al., 2016). miR-210 KO flies also have an advancement in the phase of the evening peak while having normal FRP, amplitude and PER cyclic expression (Cusumano et al., 2018). Previous studies have shown that at higher temperatures, DN1p neurons promote the morning anticipatory activity without affecting the free-running period because it is temperature-compensated (Pittendrigh, 1954; Lamaze et al., 2017). Similarly, glass 60j mutants which do not have DN1p are rhythmic under DD but do not exhibit morning anticipatory activity (Helfrich-Förster et al., 2001). The results of our study suggest that the M peak phase advancement observed in flies upon miR-277 downregulation in the PDF neurons is without affecting the speed of the clock. The amplitude of DD rhythm was found to be decreased in the clock/ PDF neurons specific downregulation of miR-277 indicating the importance of miR-277 expression in clock neurons for robust rhythms.
The role of miR-277 in the clock neurons was further investigated by overexpressing miR-277 in the whole clock tissues. This also resulted in a phase advance of the morning peak by a magnitude of approximately 40 min when compared to the controls. In addition, these flies enhanced the activity prior to lights-off. However, when miR-277 was overexpressed in PDF neurons, we found a phase advance in the morning peak of activity without an effect on the activity prior to lights-off. The FRP however was not different for the miR-277 overexpressed flies compared to the controls. This indicates that the overexpression of miR-277 in the PDF neurons might be affecting the PDF signaling from sLNvs to DN1ps and hence altering the activity around M peak without changing the speed of the clock (Zhang et al., 2010). Downregulation as well as upregulation of miR-277 in PDF neurons specific manner producing a similar behavioural phenotype in the phase of morning activity peak is perplexing. Several studies have previously reported similar instances where miRNA downregulation as well as overexpression showing similar phenotype. Downregulation as well as overexpression of miR-276a in the clock neurons is reported to result in decreased rhythmicity (Chen and Rosbash, 2016). Constitutive expression as well as downregulation of miR-277 is known to result in reduced lifespan in flies (Esslinger et al., 2013). This study identified miR-277 as a regulator of branched chain amino acid (BCAA) catabolism and proposed that fine tuning of BCAA catabolism by miR-277 is required for optimal longevity. MicroRNAs are known to fine tune the expression of target genes to physiologically relevant levels (Hobert, 2007; Karres et al., 2007). Hence, we assume that the level of mRNA responsible for phasing of M peak might be fine-tuned to an optimal level by miR-277 and downregulation or overexpression of miR-277 is possibly altering the optimal expression of the target mRNA, leading to phase advancement.
Previous reports suggest that artificially overexpressing miRNAs may cause non-physiological effects (Selbach et al., 2008). Some studies discuss the possibility that overexpression of miRNA could cause downregulation of physiologically non relevant target mRNAs or mRNAs that have weaker affinity binding sites (Schertel et al., 2012). Hence it is possible that the functional target might be different during downregulation and overexpression of the miRNA as the miRNA-target interaction dynamics is dependent on their relative expression levels. Hence the phase advancement observed in downregulation and overexpression of miR-277 might be mediated by two distinct target mRNAs acting on the same or different pathway of M peak phase regulation in the PDF neurons. We also observe that robustness of the rhythm is reduced upon downregulation of miR-277 in the clock neurons but not upon overexpression. This also might be indicative of the fact that two distinct mRNAs are being targeted during downregulation and overexpression.
Since light is the only zeitgeber in an experiment set up with constant temperature and humidity settings, the altered phase under LD condition can be assumed to be the result of changes in the light sensory pathways or due to changes in the core clock components. Blue light photoreceptor CRY and other external photoreceptors are thought to be important in light mediated entrainment (Stanewsky et al., 1998; Helfrich-Förster et al., 2002; Rieger et al., 2003). We assessed the role of miR-277 in governing the phase of behavioural rhythm under various light intensities. Downregulation of miR-277 in PDF-positive neurons altered the activity around the morning peak under LD with various light intensities ranging from 10 to 1000 lux indicating that miR-277 expressed in PDF-positive neurons mediate the proper activity distribution around the morning peak under various light intensities. Whereas we found a difference in the activity around the E peak only at lower light intensities. This might be because the morning oscillator is capable of interacting with the evening oscillator via the PDF neuropeptide and modulates the timing of the evening behaviour (Cusumano et al., 2009). Eventhough both LNds as well as DN1ps are capable of setting the phase of the evening activity, under low light intensities, the morning oscillator enslaves the LNd evening oscillator and this interaction diminishes with increasing light intensity (Chatterjee et al., 2018). miR-277 downregulation in the PDF neuron affected the activity around the evening peak only at lower light intensity and this effect is diminished under high light intensity (1000 lux). Hence, we speculate that miR-277 present in the PDF neurons might be influencing the phasing of the rhythm by controlling the LNv-LNd interaction, possibly via PDF signalling.
The difference in locomotor activity response during light-dark or dark-light transitions under various light intensities of LD might be due to the difference in the entrainment pathways under different light intensities. norpA encoding Phospholipase-C (PLC), which acts downstream of rhodopsin (RH) is critical for entrainment under low light intensities (Zuker, 1996). CRY and 4 Rhodopsin expressing photoreceptors (R1-R6 expressing RH1, yellow R8 inner photoreceptor expressing RH6, pale R7 photoreceptor expressing RH3 and yellow R7 photoreceptor expressing RH4) of compound eyes are also known to be involved in entrainment at low intensities (Stanewsky et al., 1998; Saint-Charles et al., 2016). Entrainment at higher intensities is known to be mediated by HB eyelets possibly with the help of RH6 in a norpA-independent pathway (Schlichting et al., 2019).
Misregulation of miR-277 in the PDF positive neurons did not alter the phase of cry transcript diurnal oscillation, however we found a significant increase in the cry mRNA level at ZT06 in these flies compared to the controls. We further analysed the impact of miR-277 expressed in PDF positive neurons on the central oscillator core clock genes such as per, tim and Clk. When miR-277 was downregulated in PDF positive neurons, we did not find any significant difference in the tim and per transcript oscillation. Nonetheless, downregulation of miR-277 in the PDF neurons abolished Clk mRNA oscillation in the fly head. Such an effect on the Clk oscillation upon downregulation of the miRNA in a very small subset of clock neurons suggests the possibility of miR-277 expressed in the PDF neurons affecting the clock machinery in the visual photoreceptors. Clock as well as clock regulated genes are expressed in the different cell types of Drosophila visual system and circadian neuropeptides such as PDF and Ion transport peptide (ITP) are shown to regulate circadian rhythms in the expression of these genes (Damulewicz et al., 2013; Górska-Andrzejak et al., 2013; Damulewicz et al., 2015). Large LNvs have arborizations extending to the distal medulla and PDF receptors are expressed in the fenestrated glia of the outer retina, suggesting potential PDF signalling to the photoreceptor cells (Im and Taghert, 2010).
When we downregulated miR-277 in PDF positive neurons, Clk mRNA oscillation was abolished but it didn’t affect the per and tim oscillation. Although the Clk mRNA exhibits circadian oscillation in wild type flies, the CLK protein level is not known to exhibit any daily oscillation (Bae et al., 1998; Houl et al., 2006). The ClkARK flies with Clk mRNA expression under the control of per or tim promotor exhibited normal per and tim oscillations and had no effects on the circadian regulation of locomotor activity of the flies except the extended morning activity (Kim et al., 2002). In addition, simulation studies showed that fixing the concentration of the CLK to a constant level or to half of its peak value does not affect the circadian oscillation of per or PER (Smolen et al., 2001; Smolen et al., 2004). Taken together these studies support the observation that Clk mRNA oscillation can be abolished without necessarily impacting the per or tim oscillations.
In order to identify whether Clk is under post-transcriptional regulation of miR-277, we looked at data obtained from previous in vivo study of miRNA regulated transcripts (Xia et al., 2020). The CLEAR-CLIP data does not reveal any interaction between Clk and miR-277. This was further confirmed by in silico analyses. These bioinformatic tools uses various criteria such as complementarity between the seed region of the miRNA and seed match region in the 3′UTR of the mRNA, the free energy change of the hybridisation, evolutionary conservation of the miRNA-mRNA pair, etc., to predict the targets of a miRNA. We have used PicTar, TargetScanFly7.2 and Diana Tools for target prediction. Clk or any of the transcripts in the primary TTFL did not come up in the analyses using these softwares. Hence it is likely that miR-277 does not directly target the Clk and it regulates the Clk oscillation via a mediator. Since Clk gene expression is regulated by PDP1 and VRILLE (VRI) (Cyran et al., 2003), we also checked whether these mRNAs are targets of miR-277. Both CLEAR-CLIP and Diana Tools software suggest pdp1ε as a potential target, suggesting a possible mechanism by which miR-277 might be affecting Clk transcript oscillation.
Studies using the ClkJrk mutants have shown that this mutation abolishes the lights-on locomotor activity response and these flies fail to anticipate light to dark transition under LD. ClkSV40 flies with deleted 3′UTR site exhibit defects in the development of the PDF neurons with unequal number of PDF neurons in both the hemispheres (Lerner et al., 2015). These studies along with our results point to the importance of miR-277 mediated proper Clk expression in the PDF neurons which in turn may govern the clock behavioral output. Although Clk mRNA level was measured in the adult fly head, the Pdf G4 driven miR-277 downregulation was not adult stage specific. Hence we cannot tease apart the developmental and adult stage specific roles of miR-277 in the PDF neurons.
In summary, our study shows a previously unknown role of miR-277 in governing the phase of circadian locomotor activity rest rhythm in Drosophila. The results of our study suggest that miR-277 expressed in the PDF neurons controls circadian rhythms in flies probably by fine tuning the Clk diurnal oscillations.
Data availability statement
The original contributions presented in the study are included in the article/Supplementary Material, further inquiries can be directed to the corresponding author.
Ethics statement
The manuscript presents research on animals that do not require ethical approval for their study.
Author contributions
Conceived and designed the experiments: NK and GA. Performed the experiments: GA and MJ. Analyzed the data: GA and MJ. Wrote the paper: GA and NK. Contributed reagents/materials, Funding acquisition, Project administration: NK. All authors contributed to the article and approved the submitted version.
Funding
This work was supported by the DBT/Wellcome Trust India Alliance Fellowship [IA/E/15/1/502329] awarded to NK and intramural fund from Indian Institute of Science Education and Research, Thiruvananthapuram.
Acknowledgments
We thank Dr. Jishy Varghese for some of the infrastructure facilities and for the fly lines. The authors very much appreciate the support by Namitha TJ, Jervis Fernandes, Swetha Gopalakrishnan and Aishwarya Segu during the manuscript revision.
Conflict of interest
The authors declare that the research was conducted in the absence of any commercial or financial relationships that could be construed as a potential conflict of interest.
Publisher’s note
All claims expressed in this article are solely those of the authors and do not necessarily represent those of their affiliated organizations, or those of the publisher, the editors and the reviewers. Any product that may be evaluated in this article, or claim that may be made by its manufacturer, is not guaranteed or endorsed by the publisher.
Supplementary material
The Supplementary Material for this article can be found online at: https://www.frontiersin.org/articles/10.3389/fphys.2023.1082866/full#supplementary-material
References
Allada R., White N. E., So W. V., Hall J. C., Rosbash M. (1998). A mutant Drosophila homolog of mammalian Clock disrupts circadian rhythms and transcription of period and timeless. Cell 93 (5), 791–804. doi:10.1016/s0092-8674(00)81440-3
Bae K., Lee C., Sidote D., Chuang K. Y., Edery I. (1998). Circadian regulation of a Drosophila homolog of the mammalian Clock gene: PER and TIM function as positive regulators. Mol. Cell Biol. 18 (10), 6142–6151. doi:10.1128/mcb.18.10.6142
Busza A., Emery-Le M., Rosbash M., Emery P. (2004). Roles of the two Drosophila CRYPTOCHROME structural domains in circadian photoreception. Science 304 (5676), 1503–1506. doi:10.1126/science.1096973
Carthew R. W., Sontheimer E. J. (2009). Origins and Mechanisms of miRNAs and siRNAs. Cell 136 (4), 642–655. doi:10.1016/j.cell.2009.01.035
Ceriani M. F., Darlington T. K., Staknis D., Más P., Petti A. A., Weitz C. J., et al. (1999). Light-dependent sequestration of TIMELESS by CRYPTOCHROME. Science 285 (5427), 553–556. doi:10.1126/science.285.5427.553
Cerro-Herreros E., Fernandez-Costa J. M., Sabater-Arcis M., Llamusi B., Artero R. (2016). Derepressing muscleblind expression by miRNA sponges ameliorates myotonic dystrophy-like phenotypes in Drosophila. Sci. Rep. 6 (1), 36230. doi:10.1038/srep36230
Chatterjee A., Lamaze A., De J., Mena W., Chélot E., Martin B., et al. (2018). Reconfiguration of a multi-oscillator network by light in the Drosophila circadian clock. Curr. Biol. 28 (13), 2007–2017. doi:10.1016/j.cub.2018.04.064
Chen W., Liu Z., Li T., Zhang R., Xue Y., Zhong Y., et al. (2014). Regulation of Drosophila circadian rhythms by miRNA let-7 is mediated by a regulatory cycle. Nat. Commun. 5, 5549. doi:10.1038/ncomms6549
Chen X., Rosbash M. (2016). mir-276a strengthens Drosophila circadian rhythms by regulating timeless expression. Proc. Natl. Acad. Sci. 113 (21), E2965–E2972. doi:10.1073/pnas.1605837113
Chen X., Rosbash M. (2017). MicroRNA-92a is a circadian modulator of neuronal excitability in Drosophila. Nat. Commun. 8, 14707. doi:10.1038/ncomms14707
Cusumano P., Biscontin A., Sandrelli F., Mazzotta G. M., Tregnago C., De Pittà C., et al. (2018). Modulation of miR-210 alters phasing of circadian locomotor activity and impairs projections of PDF clock neurons in Drosophila melanogaster. PLoS Genet. 14 (7), e1007500. doi:10.1371/journal.pgen.1007500
Cusumano P., Klarsfeld A., Chélot E., Picot M., Richier B., Rouyer F. (2009). PDF-modulated visual inputs and cryptochrome define diurnal behavior in Drosophila. Nat. Neurosci. 12 (11), 1431–1437. doi:10.1038/nn.2429
Cyran S. A., Buchsbaum A. M., Reddy K. L., Lin M. C., Glossop N. R., Hardin P. E., et al. (2003). vrille, Pdp1, and dClock form a second feedback loop in the Drosophila circadian clock. Cell 112 (3), 329–341. doi:10.1016/s0092-8674(03)00074-6
Damulewicz M., Loboda A., Bukowska-Strakova K., Jozkowicz A., Dulak J., Pyza E. (2015). Clock and clock-controlled genes are differently expressed in the retina, lamina and in selected cells of the visual system of Drosophila melanogaster. Front. Cell Neurosci. 9, 353. doi:10.3389/fncel.2015.00353
Damulewicz M., Rosato E., Pyza E. (2013). Circadian regulation of the Na+/K+-ATPase alpha subunit in the visual system is mediated by the pacemaker and by retina photoreceptors in Drosophila melanogaster. PLoS One 8 (9), e73690. doi:10.1371/journal.pone.0073690
Das A., Holmes T. C., Sheeba V. (2016). dTRPA1 in non-circadian neurons modulates temperature-dependent rhythmic activity in Drosophila melanogaster. J. Biol. Rhythms 31 (3), 272–288. doi:10.1177/0748730415627037
Ebert M. S., Sharp P. A. (2012). Roles for microRNAs in conferring robustness to biological processes. Cell 149 (3), 515–524. doi:10.1016/j.cell.2012.04.005
Esslinger S. M., Schwalb B., Helfer S., Michalik K. M., Witte H., Maier K. C., et al. (2013). Drosophila miR-277 controls branched-chain amino acid catabolism and affects lifespan. RNA Biol. 10 (6), 1042–1056. doi:10.4161/rna.24810
Fulga T. A., McNeill E. M., Binari R., Yelick J., Blanche A., Booker M., et al. (2015). A transgenic resource for conditional competitive inhibition of conserved Drosophila microRNAs. Nat. Commun. 6, 7279. doi:10.1038/ncomms8279
Garaulet D. L., Sun K., Li W., Wen J., Panzarino A. M., O'Neil J. L., et al. (2016). miR-124 regulates diverse aspects of rhythmic behavior in Drosophila. J. Neurosci. 36 (12), 3414–3421. doi:10.1523/JNEUROSCI.3287-15.2016
Gekakis N., Staknis D., Nguyen H. B., Davis F. C., Wilsbacher L. D., King D. P., et al. (1998). Role of the CLOCK protein in the mammalian circadian mechanism. Science 280 (5369), 1564–1569. doi:10.1126/science.280.5369.1564
Górska-Andrzejak J., Makuch R., Stefan J., Görlich A., Semik D., Pyza E. (2013). Circadian expression of the presynaptic active zone protein bruchpilot in the lamina of Drosophila melanogaster. Dev. Neurobiol. 73 (1), 14–26. doi:10.1002/dneu.22032
Grima B., Chélot E., Xia R., Rouyer F. (2004). Morning and evening peaks of activity rely on different clock neurons of the Drosophila brain. Nature 431 (7010), 869–873. doi:10.1038/nature02935
Hardin P. E., Hall J. C., Rosbash M. (1990). Feedback of the Drosophila period gene product on circadian cycling of its messenger RNA levels. Nature 343 (6258), 536–540. doi:10.1038/343536a0
Helfrich-Förster C. (2000). Differential control of morning and evening components in the activity rhythm of Drosophila melanogaster--sex-specific differences suggest a different quality of activity. J. Biol. Rhythms 15 (2), 135–154. doi:10.1177/074873040001500208
Helfrich-Förster C., Edwards T., Yasuyama K., Wisotzki B., Schneuwly S., Stanewsky R., et al. (2002). The extraretinal eyelet of Drosophila: development, ultrastructure, and putative circadian function. J. Neurosci. 22 (21), 9255–9266. doi:10.1523/JNEUROSCI.22-21-09255.2002
Helfrich-Förster C., Shafer O. T., Wülbeck C., Grieshaber E., Rieger D., Taghert P. (2007). Development and morphology of the clock-gene-expressing lateral neurons of Drosophila melanogaster. J. Comp. Neurol. 500 (1), 47–70. doi:10.1002/cne.21146
Helfrich-Förster C., Winter C., Hofbauer A., Hall J. C., Stanewsky R. (2001). The circadian clock of fruit flies is blind after elimination of all known photoreceptors. Neuron 30 (1), 249–261. doi:10.1016/s0896-6273(01)00277-x
Herranz H., Cohen S. M. (2010). MicroRNAs and gene regulatory networks: managing the impact of noise in biological systems. Genes Dev. 24 (13), 1339–1344. doi:10.1101/gad.1937010
Houl J. H., Yu W., Dudek S. M., Hardin P. E. (2006). Drosophila CLOCK is constitutively expressed in circadian oscillator and non-oscillator cells. J. Biol. Rhythms 21 (2), 93–103. doi:10.1177/0748730405283697
Im S. H., Taghert P. H. (2010). PDF receptor expression reveals direct interactions between circadian oscillators in Drosophila. J. Comp. Neurol. 518 (11), 1925–1945. doi:10.1002/cne.22311
Kadener S., Menet J. S., Sugino K., Horwich M. D., Weissbein U., Nawathean P., et al. (2009). A role for microRNAs in the Drosophila circadian clock. Genes Dev. 23 (18), 2179–2191. doi:10.1101/gad.1819509
Karres J. S., Hilgers V., Carrera I., Treisman J., Cohen S. M. (2007). The conserved microRNA MiR-8 tunes atrophin levels to prevent neurodegeneration in Drosophila. Cell 131 (1), 136–145. doi:10.1016/j.cell.2007.09.020
Kim E. Y., Bae K., Ng F. S., Glossop N. R., Hardin P. E., Edery I. (2002). Drosophila CLOCK protein is under posttranscriptional control and influences light-induced activity. Neuron 34 (1), 69–81. doi:10.1016/s0896-6273(02)00639-6
Konopka R. J., Benzer S. (1971). Clock mutants of Drosophila melanogaster. Proc. Natl. Acad. Sci. U. S. A. 68 (9), 2112–2116. doi:10.1073/pnas.68.9.2112
Kume K., Zylka M. J., Sriram S., Shearman L. P., Weaver D. R., Jin X., et al. (1999). mCRY1 and mCRY2 are essential components of the negative limb of the circadian clock feedback loop. Cell 98 (2), 193–205. doi:10.1016/s0092-8674(00)81014-4
Lamaze A., Öztürk-Çolak A., Fischer R., Peschel N., Koh K., Jepson J. E. C. (2017). Regulation of sleep plasticity by a thermo-sensitive circuit in Drosophila. Sci. Rep. 7, 40304. doi:10.1038/srep40304
Lee C., Bae K., Edery I. (1999). PER and TIM inhibit the DNA binding activity of a Drosophila CLOCK-CYC/dBMAL1 heterodimer without disrupting formation of the heterodimer: a basis for circadian transcription. Mol. Cell Biol. 19 (8), 5316–5325. doi:10.1128/mcb.19.8.5316
Lerner I., Bartok O., Wolfson V., Menet J. S., Weissbein U., Afik S., et al. (2015). Clk post-transcriptional control denoises circadian transcription both temporally and spatially. Nat. Commun. 6 (1), 7056. doi:10.1038/ncomms8056
Li M. T., Cao L. H., Xiao N., Tang M., Deng B., Yang T., et al. (2018). Hub-organized parallel circuits of central circadian pacemaker neurons for visual photoentrainment in Drosophila. Nat. Commun. 9 (1), 4247. doi:10.1038/s41467-018-06506-5
Luo W., Sehgal A. (2012). Regulation of circadian behavioral output via a MicroRNA-JAK/STAT circuit. Cell 148 (4), 765–779. doi:10.1016/j.cell.2011.12.024
Mehta N., Cheng H. Y. (2013). Micro-managing the circadian clock: the role of microRNAs in biological timekeeping. J. Mol. Biol. 425 (19), 3609–3624. doi:10.1016/j.jmb.2012.10.022
Menet J. S., Abruzzi K. C., Desrochers J., Rodriguez J., Rosbash M. (2010). Dynamic PER repression mechanisms in the Drosophila circadian clock: from on-DNA to off-DNA. Genes Dev. 24 (4), 358–367. doi:10.1101/gad.1883910
Nelson W., Tong Y. L., Lee J. K., Halberg F. (1979). Methods for cosinor-rhythmometry. Chronobiologia 6 (4), 305–323.
Nian X., Chen W., Bai W., Zhao Z. (2022). Regulation of circadian locomotor rhythm by miR-263a. Biol. Rhythm Res. 53 (1), 148–158. doi:10.1080/09291016.2020.1726049
Nian X., Chen W., Bai W., Zhao Z., Zhang Y. (2019). miR-263b controls circadian behavior and the structural plasticity of pacemaker neurons by regulating the LIM-only protein beadex. Cells 8 (8), 923. doi:10.3390/cells8080923
Niu Y., Liu Z., Nian X., Xu X., Zhang Y. (2019). miR-210 controls the evening phase of circadian locomotor rhythms through repression of Fasciclin 2. PLoS Genet. 15 (7), e1007655. doi:10.1371/journal.pgen.1007655
Pittendrigh C. S. (1954). On Temperature Independence in the clock system controlling emergence time in drosophila. Proc. Natl. Acad. Sci. U. S. A. 40 (10), 1018–1029. doi:10.1073/pnas.40.10.1018
Renn S. C., Park J. H., Rosbash M., Hall J. C., Taghert P. H. (1999). A pdf neuropeptide gene mutation and ablation of PDF neurons each cause severe abnormalities of behavioral circadian rhythms in Drosophila. Cell 99 (7), 791–802. doi:10.1016/s0092-8674(00)81676-1
Rieger D., Stanewsky R., Helfrich-Förster C. (2003). Cryptochrome, compound eyes, Hofbauer-Buchner eyelets, and ocelli play different roles in the entrainment and masking pathway of the locomotor activity rhythm in the fruit fly Drosophila melanogaster. J. Biol. Rhythms 18 (5), 377–391. doi:10.1177/0748730403256997
Rutila J. E., Suri V., Le M., So W. V., Rosbash M., Hall J. C. (1998). CYCLE is a second bHLH-PAS clock protein essential for circadian rhythmicity and transcription of Drosophila period and timeless. Cell 93 (5), 805–814. doi:10.1016/s0092-8674(00)81441-5
Saint-Charles A., Michard-Vanhée C., Alejevski F., Chélot E., Boivin A., Rouyer F. (2016). Four of the six Drosophila rhodopsin-expressing photoreceptors can mediate circadian entrainment in low light. J. Comp. Neurol. 524 (14), 2828–2844. doi:10.1002/cne.23994
Schertel C., Rutishauser T., Förstemann K., Basler K. (2012). Functional characterization of Drosophila microRNAs by a novel in vivo library. Genetics 192 (4), 1543–1552. doi:10.1534/genetics.112.145383
Schlichting M., Helfrich-Förster C. (2015). Photic entrainment in Drosophila assessed by locomotor activity recordings. Methods Enzymol. 552, 105–123. doi:10.1016/bs.mie.2014.10.017
Schlichting M., Menegazzi P., Rosbash M., Helfrich-Förster C. (2019). A distinct visual pathway mediates high-intensity light adaptation of the circadian clock in Drosophila. J. Neurosci. 39 (9), 1621–1630. doi:10.1523/JNEUROSCI.1497-18.2018
Sehgal A., Price J. L., Man B., Young M. W. (1994). Loss of circadian behavioral rhythms and per RNA oscillations in the Drosophila mutant timeless. Science 263 (5153), 1603–1606. doi:10.1126/science.8128246
Selbach M., Schwanhäusser B., Thierfelder N., Fang Z., Khanin R., Rajewsky N. (2008). Widespread changes in protein synthesis induced by microRNAs. Nature 455 (7209), 58–63. doi:10.1038/nature07228
Sharkey C. R., Blanco J., Leibowitz M. M., Pinto-Benito D., Wardill T. J. (2020). The spectral sensitivity of Drosophila photoreceptors. Sci. Rep. 10 (1), 18242. doi:10.1038/s41598-020-74742-1
Sheeba V., Fogle K. J., Holmes T. C. (2010). Persistence of morning anticipation behavior and high amplitude morning startle response following functional loss of small ventral lateral neurons in Drosophila. PLoS One 5 (7), e11628. doi:10.1371/journal.pone.0011628
Smolen P., Baxter D. A., Byrne J. H. (2001). Modeling circadian oscillations with interlocking positive and negative feedback loops. J. Neurosci. 21 (17), 6644–6656. doi:10.1523/JNEUROSCI.21-17-06644.2001
Smolen P., Hardin P. E., Lo B. S., Baxter D. A., Byrne J. H. (2004). Simulation of Drosophila circadian oscillations, mutations, and light responses by a model with VRI, PDP-1, and CLK. Biophys. J. 86 (5), 2786–2802. doi:10.1016/S0006-3495(04)74332-5
Stanewsky R., Kaneko M., Emery P., Beretta B., Wager-Smith K., Kay S. A., et al. (1998). The cryb mutation identifies cryptochrome as a circadian photoreceptor in Drosophila. Cell 95 (5), 681–692. doi:10.1016/s0092-8674(00)81638-4
Stoleru D., Peng Y., Agosto J., Rosbash M. (2004). Coupled oscillators control morning and evening locomotor behaviour of Drosophila. Nature 431 (7010), 862–868. doi:10.1038/nature02926
Sun K., Jee D., de Navas L. F., Duan H., Lai E. C. (2015). Multiple in vivo biological processes are mediated by functionally redundant activities of Drosophila mir-279 and mir-996. PLoS Genet. 11 (6), e1005245. doi:10.1371/journal.pgen.1005245
Taylor P., Hardin P. E. (2008). Rhythmic E-box binding by CLK-CYC controls daily cycles in per and tim transcription and chromatin modifications. Mol. Cell Biol. 28 (14), 4642–4652. doi:10.1128/MCB.01612-07
Veleri S., Rieger D., Helfrich-Förster C., Stanewsky R. (2007). Hofbauer-Buchner eyelet affects circadian photosensitivity and coordinates TIM and PER expression in Drosophila clock neurons. J. Biol. Rhythms 22 (1), 29–42. doi:10.1177/0748730406295754
Vodala S., Pescatore S., Rodriguez J., Buescher M., Chen Y. W., Weng R., et al. (2012). The oscillating miRNA 959-964 cluster impacts Drosophila feeding time and other circadian outputs. Cell Metab. 16 (5), 601–612. doi:10.1016/j.cmet.2012.10.002
Xia X., Fu X., Du J., Wu B., Zhao X., Zhu J., et al. (2020). Regulation of circadian rhythm and sleep by miR-375-timeless interaction in Drosophila. Faseb J. 34 (12), 16536–16551. doi:10.1096/fj.202001107R
Xue Y., Zhang Y. (2018). Emerging roles for microRNA in the regulation of Drosophila circadian clock. BMC Neurosci. 19 (1), 1. doi:10.1186/s12868-018-0401-8
Yoshii T., Hermann-Luibl C., Helfrich-Forster C. (2016). Circadian light-input pathways in Drosophila. Commun. Integr. Biol. 9 (1), e1102805. doi:10.1080/19420889.2015.1102805
Zhang L., Chung B. Y., Lear B. C., Kilman V. L., Liu Y., Mahesh G., et al. (2010). DN1p circadian neurons coordinate acute light and PDF inputs to produce robust daily behavior in Drosophila. Curr. Biol. 20 (7), 591–599. doi:10.1016/j.cub.2010.02.056
Zhang Y., Lamba P., Guo P., Emery P. (2016). miR-124 regulates the phase of Drosophila circadian locomotor behavior. J. Neurosci. 36 (6), 2007–2013. doi:10.1523/JNEUROSCI.3286-15.2016
Zipper L., Batchu S., Kaya N. H., Antonello Z. A., Reiff T. (2022). The MicroRNA miR-277 controls physiology and pathology of the adult Drosophila midgut by regulating the expression of fatty acid β-oxidation-related genes in intestinal stem cells. Metabolites 12 (4), 315. doi:10.3390/metabo12040315
Keywords: circadian, phase, amplitude, free running period, miR-277, light intensity, Clock gene
Citation: Anna G, John M and Kannan NN (2023) miR-277 regulates the phase of circadian activity-rest rhythm in Drosophila melanogaster. Front. Physiol. 14:1082866. doi: 10.3389/fphys.2023.1082866
Received: 28 October 2022; Accepted: 07 November 2023;
Published: 28 November 2023.
Edited by:
Joanna C. Chiu, University of California, Davis, United StatesReviewed by:
Yong Zhang, Soochow University, ChinaYao Drummer Cai, University of California, Davis, United States
Copyright © 2023 Anna, John and Kannan. This is an open-access article distributed under the terms of the Creative Commons Attribution License (CC BY). The use, distribution or reproduction in other forums is permitted, provided the original author(s) and the copyright owner(s) are credited and that the original publication in this journal is cited, in accordance with accepted academic practice. No use, distribution or reproduction is permitted which does not comply with these terms.
*Correspondence: Nisha N. Kannan, bmlzaGFua2FubmFuQGlpc2VydHZtLmFjLmlu
†Present address: Maria John, Department of Biological Sciences, University of Bergen, Norway