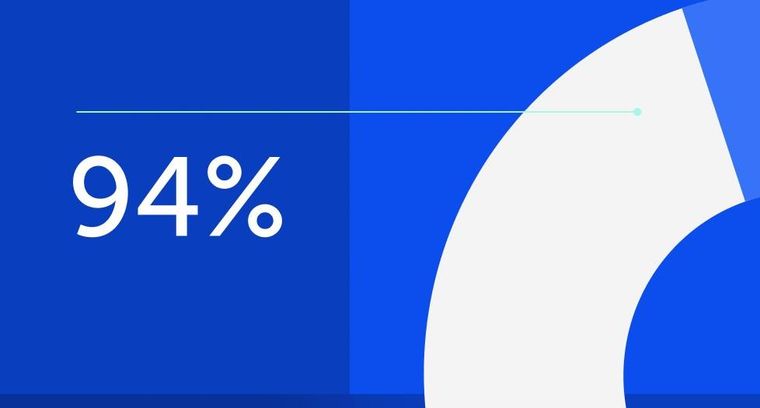
94% of researchers rate our articles as excellent or good
Learn more about the work of our research integrity team to safeguard the quality of each article we publish.
Find out more
ORIGINAL RESEARCH article
Front. Physiol., 19 January 2023
Sec. Exercise Physiology
Volume 14 - 2023 | https://doi.org/10.3389/fphys.2023.1070653
Objective: This study aims to explore the dose-response relationship between the daily duration of moderate to vigorous physical activity and the improvement of cardiometabolic risk indicators in obese children and adolescents.
Methods: Seventy-seven obese children and adolescents aged 10–17 years were randomly recruited for a 4-week exercise intervention in a closed camp during 2019–2021, physical activity was monitored by ActiGraph GT3X + to obtain daily MVPA duration, and the improvement of CMR indicators were reflected by the changes (Δ) of waist circumference, systolic blood pressure, diastolic blood pressure, total cholesterol, triglyceride , high-density lipoprotein cholesterol, low-density lipoprotein cholesterol, fasting insulin, fasting plasma glucose, and homeostasis model assessment of insulin resistance before and after the intervention, calculated as ‘‘Δ+indicator” = values after intervention–values before intervention. The groups were divided into different doses of Q1∼Q3 according to the daily MVPA duration from low to high. The differences in the improvement of different dose groups were compared by one-way analysis of covariance, and the dose-response relationship between MVPA duration and CMR indicators improvement was analyzed by linear regression and piecewise regression. The nonlinear relationship was analyzed by restricted cubic spline.
Results: 1) Compared with indicators before the intervention, WC, SBP, DBP, TC, TG, HDL-C, LDL-C, FINS, and HOMA-IR were significantly lower after the intervention (p-value < 0.05). 2) The dose-response relationship between MVPA and LDL-C improvement was non-linear (P-Nonlinear < 0.05). When MVPA >77.1min/day, ΔLDL-C further decreased with the increase of MVPA duration [β = −0.009, 95% confidence interval (CI): −0.013, −0.005], and when MVPA ≤77.1min/day, increasing the MVPA duration did not increase the improvement of ΔLDL-C.
Conclusion: There was a nonlinear dose-response relationship between the daily MVPA duration and LDL-C improvement in obese children and adolescents. In order to obtain more significant improvement in LDL-C through increased MVPA duration, MVPA duration should be higher than 77.1 min/day.
The incidence of childhood and adolescent obesity has been on the rise globally over the last half-century, and this has placed a huge burden on the current global public health system (Afshin et al., 2017). As of 2017, the detection rate of obesity among children and adolescents aged 9–17 years in China has exceeded 10% (Zhu et al., 2019), while globally, more than 100 million children and adolescents have obesity problems, and if no effective measures are taken, the total number of overweight and obese children worldwide is expected to exceed 250 million (Shah et al., 2020). Obesity can lead to an increased risk of heart disease by aggregating cardiometabolic risk (CMR) factors such as hypertension, insulin resistance, dyslipidemia, and type 2 diabetes (Chung et al., 2018). In addition, the development of obesity in childhood and adolescence can have a profound negative impact on physical health status in adulthood (Suglia et al., 2018), and evidence from cross-sectional and prospective studies suggests that obesity in childhood and adolescence is associated with the development of atherosclerosis in adulthood (Berenson et al., 1998; Cote et al., 2015). The significance of early intervention in obese children and adolescents to improve cardiometabolic health cannot be overstated.
Physical activity (PA) not only improves the physical shape of obese children and adolescents but also its effect on cardiometabolic health has been confirmed by previous studies (Väistö et al., 2014; Júdice et al., 2020). The correlation between moderate to vigorous physical activity (MVPA) and improved cardiometabolic health is considered to be high, while the correlation between light physical activity (LPA) and improved cardiometabolic health is negligible (Hansen et al., 2018; Väistö et al., 2019). Current physical activity guidelines issued by national and international authorities recommend that children and adolescents should engage in MVPA for at least 60 min per day to achieve significant health benefits (Misra et al., 2012; Kahlmeier et al., 2015; Zhan et al., 2017; Piercy et al., 2018; Bull et al., 2020; Clutterbuck and Johnston, 2021), which gives children and adolescents the basis for exercising in their daily lives. Obese children and adolescents are at higher risk of injury when exercising than normal-weight ones, and to balance safety and efficiency, exercise needs to be planned precisely according to the dose-response relationship between physical activity and health effects, thus ensuring higher health improvement by increasing physical activity within a reasonable range. However, existing physical activity guidelines and related studies have not clarified the quantitative dose-response relationship between MVPA duration and cardiometabolic health improvement in obese children and adolescents. Therefore, this study tried to investigate the dose-response relationship between physical activity and CMR indicators in obese children and adolescents by monitoring and evaluating the effect of physical activity and CMR indicators and analyze whether there was a non-linear relationship and threshold effect, aiming to provide a scientific and accurate theoretical basis for improving cardiometabolic health through exercise in obese children and adolescents.
Participants were randomly recruited between 2019 and 2021 through the Shenzhen Weight Watchers Boot Camp. Inclusion criteria: 1) complying with the obesity criteria in the National Health and Family Planning Commission (NHFPC) WS/T586-2018 (for both boys and girls aged 6–17 years, overweight was defined as BMI ≥85th percentile whereas obesity was defined as BMI ≥95th percentile, stratified by gender and age. For example, for 10-year-old boys, BMI ≥19.2 or higher was considered overweight and BMI ≥21.9 was considered obese, whereas for 11-year-old boys, the BMI cut-off points for overweight and obesity were 19.9 and 23.0 respectively, the specific BMI cut-off points depend on age and gender, further details are provided in the supplementary material.); 2) aged 10–17 years; 3) participant’s parents or guardians were informed of the study and signed an informed consent form. Exclusion criteria: 1) those who were taking medication for obesity; 2) those with cardiopulmonary insufficiency and exercise limitation; 3) those who were overweight or normal weight status. Finally, a total of 77 participants were included.
The height and weight of the participants were measured in centimeters (cm) and kilograms (kg), respectively, using the equipment specified for national physical fitness monitoring, and the results were accurate to one decimal point. Body mass index (BMI) was calculated from the measured height and weight, with BMI = weight (kg)/height (m2). Waist circumference (WC) was measured using a leather tape measure, and the test method was performed according to the requirements of National Health and Family Planning Commission (NHFPC) WS/T611-2018.
The systolic blood pressure (SBP) and diastolic blood pressure (DBP) of the participants were measured using a Omron electronic blood pressure monitor (OMRON HEM-1020, China), and the test was performed according to the requirements of National Health and Family Planning Commission (NHFPC) WS/T610-2018.
Participants’ physical activity during camp was recorded by wearing an ActiGraph GT3X + triaxial motion accelerometer (ActiGraph, Pensacola, FL, United States), which has been shown to be applicable to physical activity measurements in children and adolescents (Mora-Gonzalez et al., 2019; Mayorga-Vega et al., 2021). All participants were asked to wear the accelerometer at the intersection of the midline of the right axilla and the horizontal line of the iliac spine (Xing et al., 2021). Testers set the sampling frequency of 30Hz and the sampling interval of the 60s in advance, and the participants wore the accelerometer 6 days a week for 4 weeks and were not allowed to remove it except for activities such as sleeping and bathing and swimming, and it was effectively worn for at least 8 h a day (Cain et al., 2013; Rich et al., 2013; Migueles et al., 2017). The accelerometers were distributed by the testers at 09:00 each morning and collected at 21:00 that evening, and the instrument was set to record data during that period for 12 h. Data were recorded, exported, and analyzed by using ActiLife Version 6.13.3 software, and the period in which no physical activity data were monitored for 60 min in a row was set as not effectively wearing the accelerometer before exporting the analysis (Skrede et al., 2017), and participants had at least 2 weeks and ≥3 days per week of effective wearing days before being included in the analysis. The cut point for MVPA selected for this study was the cut point criterion previously established by our team for obese children and adolescents, and VM3 ≥ 3,687 counts/min was determined to be MVPA (Liu et al., 2021). Participants performed 4 h of aerobic exercise based training sessions with a coach each day, 2 h in the morning and 2 h in the afternoon, participants were free to engage in physical activity outside of training sessions, 6 days per week, for a total of 4 weeks.
The participants blood samples were collected at the designated hospital at 8:00 a.m. on the second day of the camp, and all participants fasted for at least 12 h before the blood collection. Total cholesterol (TC), triglyceride (TG), high density lipoprotein cholesterol (HDL-C), low density lipoprotein (LDL-C), were measured using a fully automated biochemical analyzer (AU5800, Beckman, Japan). Fasting plasma glucose (FPG) was measured by the glucose oxidase method, and fasting insulin (FINS) was measured by electrochemiluminescence immunoassay. The homeostasis model assessment of insulin resistance (HOMA-IR) = FPG (mmol/L) × FINS (mIU/L)/22.5 (Matthews et al., 1985).
The resting energy consumption of the participants was obtained by indirect calorimetry, which is considered the gold standard for energy consumption testing (Boullata et al., 2007). The Cortex Meta Max 3B portable gas metabolism analyzer was used to measure the resting energy consumption of the participants. All participants completed the resting energy test within 1 week before the official start of exercise training and were asked to refrain from drinking coffee and alcoholic beverages for 24 h before the test and to avoid the influence of food-specific kinetic effects on the test results by having an interval of more than 2 h between the official test and the meal. During the test, the participant was sedentary for 10–15 min in a stable room temperature test site, and then the tester put on the apparatus and performed the resting energy test for 15 min in a quiet and lying position, during which the participant was awake. The amount of O2 inhaled (mL/min) and CO2 exhaled (mL/min) in each breath were recorded, and then the resting energy consumption was calculated according to the formula of weir (Weir, 1949).
All participants were put on a diet by a professional dietitian hired by the camp, and the daily caloric intake through diet was calculated based on the resting energy expenditure (REE) of the participants.REE (kcal/min) = 3.9*VO2(L/min)+1.1*VCO2(L/min); REE (kcal/day) = REE (kcal/min)*1,440. In order to match energy expenditure with REE, nutrition experts designed the diet plan in accordance with the Chinese Food Composition Table compiled by the Chinese Center for Disease and Prevention. The ratio of calorie distribution among the three meals a day was approximately 3:4:3, with the proportion of carbohydrate, protein, and fat supplying 55%–65%, 10%–15%, and 20%–35% of the total energy of each meal, respectively. The main types of food included cereals, meat, eggs, vegetables, and fruits.
Continuous variables were expressed as mean ± standard deviation (
No participants dropped out halfway through the study, and a total of 77 participants were included in the statistical analysis, including 43 males and 34 females, aged 13.0 ± 1.7 years old, and the basic information is shown in Table 1.
WC, SBP, DBP, TC, TG, HDL-C, LDL-C, FINS, and HOMA-IR after intervention were significantly lower than those before intervention (p < 0.05). There was a decreasing trend in FPG, however, without statistical significance (p > 0.05). As shown in Table 2, there was a significant difference in the improvement of LDL-C among Q1∼Q3 groups (p < 0.05), and post hoc analysis revealed that the degree of improvement in the Q3 group was significantly higher than that in the Q1 groups (p < 0. 05).
TABLE 2. Comparison of the improvement degree of cardiometabolic risk indicators in different dose groups.
Linear regression was used to analyze the dose-response relationship between daily MVPA and improvement in CMR indicators, finding that for each 1 min/day increase in MVPA duration in the unadjusted model, ΔLDL-C decreased by 0.003 mmol/L [95% confidence interval (CI): −0.005, −0.001]. After adjusting for pre-test value, age, gender, and baseline BMI, ΔLDL decreased by 0.002 mmol/L (95% CI: −0.004, 0.001) for each 1 min/day increase in MVPA duration. In addition, no linear dose-response relationship was found between MVPA duration and other CMR indicators (p > 0.05, Table 3).
TABLE 3. Linear dose-response relationship analysis of MVPA and improvement in cardiometabolic risk indicators.
The results of the RCS analysis showed that there was a nonlinear relationship between MVPA and LDL-C improvement (P-Nonlinear < 0.05) and threshold effect (Figure 1), while the nonlinear dose-response relationship between MVPA and other indicators was not found (P-Nonlinear > 0.05) (Supplementary Figures S1–S9). The results of piecewise regression showed (Table 4) that when MVPA>77.1 min/day, increasing the daily MVPA duration resulted in higher improvement, and each 1 min/day increase in MVPA duration in the unadjusted model resulted in a 0.009 mmol/L (95% CI: −0.013, −0.005) decrease in ΔLDL-C. After adjusting for pre-test value, age, gender, and baseline BMI, ΔLDL-C decreased by 0.008 mmol/L (95% CI: −0.012, −0.004) for each 1 min/day increase in MVPA duration. When MVPA was ≤77.1min/day, increasing the daily MVPA duration did not increase the improvement of LDL-C.
FIGURE 1. Nonlinear relationship between MVPA and LDL-C improvement. The red solid line shows the fitted curves, the red shaded areas show the 95% confidence intervals.
TABLE 4. Nonlinear dose-response and threshold-effect relationship analysis of MVPA and LDL-C improvement.
This study aimed to explore the dose-response relationship between daily MVPA duration and the improvement of CMR indicators in obese children and adolescents.
The results showed a non-linear dose-response relationship between daily MVPA duration and LDL-C improvement in obese children and adolescents. Only when MVPA is higher than 77.1 min/day, increasing the MVPA duration can obtain a higher LDL-C improvement effect. In clinical practice, WC is the evaluation indicator of abdominal obesity, SBP and DBP are the evaluation indicators of hypertension, and blood lipid level is used to judge whether dyslipidemia occurs, in addition, FPG, FINS and HOMA-IR are indicators of glucose metabolism (Li et al., 2022). All of these cardiometabolic risk indicators were associated with an increased risk of cardiovascular disease (CVD) (Park and Choi, 2022). Excessive accumulation of fat associated with obesity is an important cause of increased CMR (Yan et al., 2019). Previous studies have found that exercise intervention can effectively reduce the levels of weight, BMI, body fat percentage, TG, TC, LDL-C, FINS, SBP, DBP, and other CMR indicators in obese children and adolescents (Guo et al., 2011; Sigal et al., 2014; Liu et al., 2021). Similar to previous studies, the present study found significant improvements in BMI, WC, SBP, DBP, FINS, and lipids in obese children and adolescents after a 4-week exercise intervention. Notably, unlike previous studies (Wong et al., 2008; González-Ruíz et al., 2022; Meng et al., 2022) with longer intervention periods (12 weeks or longer), the obese children and adolescents in this study achieved significant health improvement after 4 weeks of exercise, suggesting that even short-period exercise interventions can achieve improved cardiometabolic health in obese children and adolescents. Elevated LDL-C is a necessary condition for atherogenesis induction, and no evidence of any clinically significant harm no matter how low the LDL-C level (Makover et al., 2022). Intervention such as exercise to reduce LDL-C levels in obese children and adolescents is crucial for the prevention of CVD. HDL-C is considered to be a cardiovascular risk protective factor, and higher HDL-C levels are associated with a lower risk of cardiovascular disease. The present study found a significant decrease in HDL-C levels after a 4-week exercise intervention, and similar results were observed in previous studies as well (Wang et al., 2011; Santos and Lavie, 2021). The reason for the HDL-C decrease may be related to the decrease in fat intake associated with dietary control during the intervention, as fatty acids are substrates of HDL-C (Santos and Lavie, 2021). Furthermore, Aicher et al. (Aicher et al., 2012) showed that although HDL-C levels decreased significantly after exercise intervention, cholesterol efflux capacity (CEC), an indicator reflecting the HDL-C’s ability of reverse cholesterol transport (RCT), did not change significantly, whereas CEC is considered to be an important indicator of HDL-C function (Rosenson, 2016) and its predictive value for the risk of cardiovascular disease is higher than that of low HDL-C (Khera et al., 2011). Therefore, this decrease in HDL-C due to the combination of exercise and dietary control is not associated with increased cardiovascular risk.
Since the 1990s, scholars have been studying the dose-response relationship between physical activity and health promotion to clarify the health effects of exercise doses produced by different combinations of exercise intensity, duration, and frequency (Haapanen et al., 1996; Kraus et al., 2001; Thompson et al., 2010). In the randomized controlled trial conducted by Davis et al. (Davis et al., 2012) on overweight/obese adolescents, the improvement of insulin sensitivity was more significant in participants of the high exercise dose group than in those of the low exercise dose group. Wu et al. (2021) found that increased levels of physical activity were associated with improved cardiometabolic risk, with a 0.359 mmol/L decrease in triglyceride and a 0.290 mmol/L increase in HDL-C for every 1METs increase in physical activity. Strizich et al. (Strizich et al., 2018) examined the relationship between objectively measured MVPA duration and cardiometabolic risk in 1,426 adolescents in a cross-sectional study and found that increased MVPA duration was associated with decreased triglycerides, and this relationship remained significant after adjustment for BMI and waist circumference. As with the previous studies mentioned above, the present study found the dose-response relationship between daily MVPA duration and improved CMR indicator in obese children and adolescents. In contrast to these previous studies, this paper found a significant nonlinearity in the dose-response relationship between MVPA and CMR indicator improvement, i.e., the improvement did not increase consistently with increasing MVPA duration. Several recent studies have also shown that the dose-response relationship between physical activity and health effects is not always linear. Sriram et al. (Sriram et al., 2021) found in a cross-sectional study consisting of normal weight adolescents that higher MVPA duration was not associated with lower SBP when the weekly MVPA duration exceeded 150 min and 90 min for boys and girls, respectively. Dipietro et al. (Dipietro et al., 2020) analyzed the relationship between different MVPA duration and the incidence of CMR aggregation in obese young adults and found that compared to participants with MVPA <150 min/week, those with MVPA between 150 and 300 min/week had a 66% lower incidence of CMR aggregation, whereas the incidence of CMR aggregation was only 61% lower in participants with MVPA >300 min/week, and the incidence of CMR aggregation did not decrease further with increasing MVPA time. In this study, we used RCS to analyze the dose-response relationship between the improvement of various CMR indicators (such as WC, SBP, DBP, FPG, TC, TG, etc.) and MVPA, but only the dose-response relationship between LDL-C and MVPA was found. As shown in Figure 1, the dose-response curve remained nearly stable at first, and then decreased rapidly after exceeding the threshold, reflecting two different trends of LDL-C improvement before and after the threshold point. The present study showed that when MVPA ≤77.1 min/day, increasing MVPA did not result in higher LDL-C improvement, while when MVPA >77.1min/day, a higher amount of MVPA resulted in more substantial improvement. Of course, not all previous studies are similar to the results of our study. Jenkins et al. (Jenkins et al., 2017) found that there was no dose-response relationship between the proportion of MVPA in the total time of physical activity and LDL-C level. The differences between the studies could be due to differences in indicators of physical activity, the participants’ weight status and ethnicity. In addition, the pre-post design used in our study also contributed to the difference between the results of previous cross-sectional studies.
Unlike previous studies, we illustrated the dose-response relationship between MVPA and CMR indicators in obese children and adolescents by pre-post quasi-experimental study design, and our findings might provide a certain reference for the development of precise exercise intervention strategies to improve cardiometabolic health in obese children and adolescents. This study has the following advantages: 1) it adopts the pre-post design with its control instead of a cross-sectional design, which avoids the limitation of cross-sectional design that cannot explore the causal relationship; and 2) it uses RCS to analyze whether there is a nonlinear relationship in the dose-response relationship, which avoids the limitation of simple linear regression that cannot explore the nonlinear relationship. Of course, this study has some limitations: 1) we only found a non-linear dose-response relationship between MVPA and LDL-C, but no dose-response relationship between other CMR indicators and MVPA was observed, which may be limited by the relatively short 4-week intervention period, and the intervention period should be extended for further study in the future; and 2) the participants were obese children and adolescents, and the closed centralized intervention led to difficulty in recruiting participants, thus limiting the further expansion of the sample size, which should be further expanded in the future for more far-reaching studies. 3) The absence of a control group may have affected the interpretation of the results to some extent.
There is a nonlinear dose-response relationship between MVPA and LDL-C improvement in obese children and adolescents. To achieve higher LDL-C improvement efficiency by increasing MVPA duration, daily MVPA duration should be higher than 77.1 min.
The raw data supporting the conclusion of this article will be made available by the authors, without undue reservation.
The studies involving human participants were reviewed and approved by Ethics Committee of Guangzhou Sports University (Approval number: 2018LCLL-008). Written informed consent to participate in this study was provided by the participants’ legal guardian/next of kin.
ZC and LZ conceived the experiments. ZC and LZ analyzed the data. ZC and LZ carried out experiments. All authors were involved in writing the paper and had final approval of the submitted and published versions.
Guangdong Planning Fund of Philosophy and Social Science (GD21CTY01); Guangdong Province Universities and Colleges Pearl River Scholar Funded Scheme (2019).
The authors declare that the research was conducted in the absence of any commercial or financial relationships that could be construed as a potential conflict of interest.
All claims expressed in this article are solely those of the authors and do not necessarily represent those of their affiliated organizations, or those of the publisher, the editors and the reviewers. Any product that may be evaluated in this article, or claim that may be made by its manufacturer, is not guaranteed or endorsed by the publisher.
The Supplementary Material for this article can be found online at: https://www.frontiersin.org/articles/10.3389/fphys.2023.1070653/full#supplementary-material
Afshin A., Forouzanfar M. H., Reitsma M. B., Sur P., Estep K., Lee A., et al. (2017). Health effects of overweight and obesity in 195 countries over 25 years. N. Engl. J. Med. 377 (1), 13–27. doi:10.1056/NEJMoa1614362
Aicher B. O., Haser E. K., Freeman L. A., Carnie A. V., Stonik J. A., Wang X., et al. (2012). Diet-induced weight loss in overweight or obese women and changes in high-density lipoprotein levels and function. Obes. (Silver Spring) 20 (10), 2057–2062. doi:10.1038/oby.2012.56
Berenson G. S., Srinivasan S. R., Bao W., Newman W. P., Tracy R. E., Wattigney W. A. (1998). Association between multiple cardiovascular risk factors and atherosclerosis in children and young adults. The Bogalusa Heart Study. N. Engl. J. Med. 338 (23), 1650–1656. doi:10.1056/nejm199806043382302
Boullata J., Williams J., Cottrell F., Hudson L., Compher C. (2007). Accurate determination of energy needs in hospitalized patients. J. Am. Diet. Assoc. 107 (3), 393–401. doi:10.1016/j.jada.2006.12.014
Bull F. C., Al-Ansari S. S., Biddle S., Borodulin K., Buman M. P., Cardon G., et al. (2020). World Health Organization 2020 guidelines on physical activity and sedentary behaviour. Br. J. Sports Med. 54 (24), 1451–1462. doi:10.1136/bjsports-2020-102955
Cain K. L., Sallis J. F., Conway T. L., Van Dyck D., Calhoon L. (2013). Using accelerometers in youth physical activity studies: A review of methods. J. Phys. Act. Health 10 (3), 437–450. doi:10.1123/jpah.10.3.437
Chung S. T., Onuzuruike A. U., Magge S. N. (2018). Cardiometabolic risk in obese children. Ann. N. Y. Acad. Sci. 1411 (1), 166–183. doi:10.1111/nyas.13602
Clutterbuck G. L., Johnston L. M. (2021). Appraisal of clinical practice guideline: Canadian 24-hour movement guidelines for children and youth: An integration of physical activity, sedentary behaviour, and sleep. J. Physiother. 67 (3), 223. doi:10.1016/j.jphys.2021.05.002
Cote A. T., Phillips A. A., Harris K. C., Sandor G. G., Panagiotopoulos C., Devlin A. M. (2015). Obesity and arterial stiffness in children: Systematic review and meta-analysis. Arterioscler. Thromb. Vasc. Biol. 35 (4), 1038–1044. doi:10.1161/atvbaha.114.305062
Davis C. L., Pollock N. K., Waller J. L., Allison J. D., Dennis B. A., Bassali R., et al. (2012). Exercise dose and diabetes risk in overweight and obese children: A randomized controlled trial. Jama 308 (11), 1103–1112. doi:10.1001/2012.jama.10762
Desquilbet L., Mariotti F. (2010). Dose-response analyses using restricted cubic spline functions in public health research. Stat. Med. 29 (9), 1037–1057. doi:10.1002/sim.3841
Dipietro L., Zhang Y., Mavredes M., Simmens S. J., Whiteley J. A., Hayman L. L., et al. (2020). Physical activity and cardiometabolic risk factor clustering in young adults with obesity. Med. Sci. Sports Exerc 52 (5), 1050–1056. doi:10.1249/mss.0000000000002214
González-Ruíz K., Correa-Bautista J. E., Izquierdo M., García-Hermoso A., Martínez-Vizcaíno V., Lobelo F., et al. (2022). Exercise dose on hepatic fat and cardiovascular health in adolescents with excess of adiposity. Pediatr. Obes. 17 (4), e12869. doi:10.1111/ijpo.12869
Guo Y., Chen P., Chen W. (2011). Effect of weight reduction on the somatometric measurements, blood lipid and blood insulin of obese children and adolescents through diet and exercise. Chin. J. Sports Med. 30, 426–431. doi:10.16038/j.1000-6710.2011.05.008
Haapanen N., Miilunpalo S., Vuori I., Oja P., Pasanen M. (1996). Characteristics of leisure time physical activity associated with decreased risk of premature all-cause and cardiovascular disease mortality in middle-aged men. Am. J. Epidemiol. 143 (9), 870–880. doi:10.1093/oxfordjournals.aje.a008830
Hansen B. H., Anderssen S. A., Andersen L. B., Hildebrand M., Kolle E., Steene-Johannessen J., et al. (2018). Cross-sectional associations of reallocating time between sedentary and active behaviours on cardiometabolic risk factors in young people: An international children's accelerometry database (ICAD) analysis. Sports Med. 48 (10), 2401–2412. doi:10.1007/s40279-018-0909-1
Jenkins G. P., Evenson K. R., Herring A. H., Hales D., Stevens J. (2017). Cardiometabolic correlates of physical activity and sedentary patterns in U.S. Youth. Med. Sci. Sports Exerc 49 (9), 1826–1833. doi:10.1249/mss.0000000000001310
Júdice P. B., Hetherington-Rauth M., Northstone K., Andersen L. B., Wedderkopp N., Ekelund U., et al. (2020). Changes in physical activity and sedentary patterns on cardiometabolic outcomes in the transition to adolescence: International children's accelerometry database 2.0. J. Pediatr. 225, 166–173. doi:10.1016/j.jpeds.2020.06.018
Kahlmeier S., Wijnhoven T. M., Alpiger P., Schweizer C., Breda J., Martin B. W. (2015). National physical activity recommendations: Systematic overview and analysis of the situation in European countries. BMC Public Health 15, 133. doi:10.1186/s12889-015-1412-3
Khera A. V., Cuchel M., de la Llera-Moya M., Rodrigues A., Burke M. F., Jafri K., et al. (2011). Cholesterol efflux capacity, high-density lipoprotein function, and atherosclerosis. N. Engl. J. Med. 364 (2), 127–135. doi:10.1056/NEJMoa1001689
Kraus W. E., Torgan C. E., Duscha B. D., Norris J., Brown S. A., Cobb F. R., et al. (2001). Studies of a targeted risk reduction intervention through defined exercise (STRRIDE). Med. Sci. Sports Exerc 33 (10), 1774–1784. doi:10.1097/00005768-200110000-00025
Li J. J., Liu H. H., Li S. (2022). Landscape of cardiometabolic risk factors in Chinese population: A narrative review. Cardiovasc Diabetol. 21 (1), 113. doi:10.1186/s12933-022-01551-3
Liu J., Zhu L., Liao J., Liu X. (2021). Effects of extreme weight loss on cardiometabolic health in children with metabolic syndrome: A metabolomic study. Front. Physiol. 12, 731762. doi:10.3389/fphys.2021.731762
Liu J., Zhu L., Xu J., Su Y., Sun Y., Liao J., et al. (2021). Diagnostic criteria and value of multiple-index joint diagnosis of exercise intensity in obese children and adolescents. J. Shanghai Inst. Sport 45, 54–61. doi:10.16099/j.sus.2021.10.005
Makover M. E., Shapiro M. D., Toth P. P. (2022). There is urgent need to treat atherosclerotic cardiovascular disease risk earlier, more intensively, and with greater precision: A review of current practice and recommendations for improved effectiveness. Am. J. Prev. Cardiol. 12, 100371. doi:10.1016/j.ajpc.2022.100371
Matthews D. R., Hosker J. P., Rudenski A. S., Naylor B. A., Treacher D. F., Turner R. C. (1985). Homeostasis model assessment: Insulin resistance and beta-cell function from fasting plasma glucose and insulin concentrations in man. Diabetologia 28 (7), 412–419. doi:10.1007/bf00280883
Mayorga-Vega D., Casado-Robles C., López-Fernández I., Viciana J. (2021). A comparison of the utility of different step-indices to translate the physical activity recommendation in adolescents. J. Sports Sci. 39 (4), 469–479. doi:10.1080/02640414.2020.1826667
Meng C., Yucheng T., Shu L., Yu Z. (2022). Effects of school-based high-intensity interval training on body composition, cardiorespiratory fitness and cardiometabolic markers in adolescent boys with obesity: A randomized controlled trial. BMC Pediatr. 22 (1), 112. doi:10.1186/s12887-021-03079-z
Migueles J. H., Cadenas-Sanchez C., Ekelund U., Delisle Nyström C., Mora-Gonzalez J., Löf M., et al. (2017). Accelerometer data collection and processing criteria to assess physical activity and other outcomes: A systematic review and practical considerations. Sports Med. 47 (9), 1821–1845. doi:10.1007/s40279-017-0716-0
Misra A., Nigam P., Hills A. P., Chadha D. S., Sharma V., Deepak K. K., et al. (2012). Consensus physical activity guidelines for Asian Indians. Diabetes Technol. Ther. 14 (1), 83–98. doi:10.1089/dia.2011.0111
Mora-Gonzalez J., Migueles J. H., Esteban-Cornejo I., Cadenas-Sanchez C., Pastor-Villaescusa B., Molina-García P., et al. (2019). Sedentarism, physical activity, steps, and neurotrophic factors in obese children. Med. Sci. Sports Exerc 51 (11), 2325–2333. doi:10.1249/mss.0000000000002064
Park M. J., Choi K. M. (2022). Association between variability of metabolic risk factors and cardiometabolic outcomes. Diabetes Metab. J. 46 (1), 49–62. doi:10.4093/dmj.2021.0316
Piercy K. L., Troiano R. P., Ballard R. M., Carlson S. A., Fulton J. E., Galuska D. A., et al. (2018). The physical activity guidelines for Americans. Jama 320 (19), 2020–2028. doi:10.1001/jama.2018.14854
Rich C., Geraci M., Griffiths L., Sera F., Dezateux C., Cortina-Borja M. (2013). Quality control methods in accelerometer data processing: Defining minimum wear time. PLoS One 8 (6), e67206. doi:10.1371/journal.pone.0067206
Rosenson R. S. (2016). The high-density lipoprotein puzzle: Why classic epidemiology, genetic epidemiology, and clinical trials conflict? Arterioscler. Thromb. Vasc. Biol. 36 (5), 777–782. doi:10.1161/atvbaha.116.307024
Santos H. O., Lavie C. J. (2021). Weight loss and its influence on high-density lipoprotein cholesterol (hdl-C) concentrations: A noble clinical hesitation. Clin. Nutr. ESPEN 42, 90–92. doi:10.1016/j.clnesp.2021.01.033
Shah B., Tombeau Cost K., Fuller A., Birken C. S., Anderson L. N. (2020). Sex and gender differences in childhood obesity: Contributing to the research agenda. BMJ Nutr. Prev. Health 3 (2), 387–390. doi:10.1136/bmjnph-2020-000074
Sigal R. J., Alberga A. S., Goldfield G. S., Prud'homme D., Hadjiyannakis S., Gougeon R., et al. (2014). Effects of aerobic training, resistance training, or both on percentage body fat and cardiometabolic risk markers in obese adolescents: The healthy eating aerobic and resistance training in youth randomized clinical trial. JAMA Pediatr. 168 (11), 1006–1014. doi:10.1001/jamapediatrics.2014.1392
Skrede T., Stavnsbo M., Aadland E., Aadland K. N., Anderssen S. A., Resaland G. K., et al. (2017). Moderate-to-vigorous physical activity, but not sedentary time, predicts changes in cardiometabolic risk factors in 10-y-old children: The active smarter kids study. Am. J. Clin. Nutr. 105 (6), 1391–1398. doi:10.3945/ajcn.116.150540
Sriram K., Mulder H. S., Frank H. R., Santanam T. S., Skinner A. C., Perrin E. M., et al. (2021). The dose-response relationship between physical activity and cardiometabolic health in adolescents. Am. J. Prev. Med. 60 (1), 95–103. doi:10.1016/j.amepre.2020.06.027
Strizich G., Kaplan R. C., Sotres-Alvarez D., Diaz K. M., Daigre A. L., Carnethon M. R., et al. (2018). Objectively measured sedentary behavior, physical activity, and cardiometabolic risk in hispanic youth: Hispanic community health study/study of latino youth. J. Clin. Endocrinol. Metab. 103 (9), 3289–3298. doi:10.1210/jc.2018-00356
Suglia S. F., Koenen K. C., Boynton-Jarrett R., Chan P. S., Clark C. J., Danese A., et al. (2018). Childhood and adolescent adversity and cardiometabolic outcomes: A scientific statement from the American heart association. Circulation 137 (5), e15–e28. doi:10.1161/cir.0000000000000536
Thompson D., Markovitch D., Betts J. A., Mazzatti D., Turner J., Tyrrell R. M. (2010). Time course of changes in inflammatory markers during a 6-mo exercise intervention in sedentary middle-aged men: A randomized-controlled trial. J. Appl. Physiol. 108 (4), 769–779. doi:10.1152/japplphysiol.00822.2009
Väistö J., Eloranta A. M., Viitasalo A., Tompuri T., Lintu N., Karjalainen P., et al. (2014). Physical activity and sedentary behaviour in relation to cardiometabolic risk in children: Cross-sectional findings from the physical activity and nutrition in children (PANIC) study. Int. J. Behav. Nutr. Phys. Act. 11, 55. doi:10.1186/1479-5868-11-55
Väistö J., Haapala E. A., Viitasalo A., Schnurr T. M., Kilpeläinen T. O., Karjalainen P., et al. (2019). Longitudinal associations of physical activity and sedentary time with cardiometabolic risk factors in children. Scand. J. Med. Sci. Sports 29 (1), 113–123. doi:10.1111/sms.13315
Wang R., Chen P. J., Chen W. H. (2011). Diet and exercise improve neutrophil to lymphocyte ratio in overweight adolescents. Int. J. Sports Med. 32 (12), 982–986. doi:10.1055/s-0031-1283185
Weir J. B. (1949). New methods for calculating metabolic rate with special reference to protein metabolism. J. Physiol. 109 (1-2), 1–9. doi:10.1113/jphysiol.1949.sp004363
Wong P. C., Chia M. Y., Tsou I. Y., Wansaicheong G. K., Tan B., Wang J. C., et al. (2008). Effects of a 12-week exercise training programme on aerobic fitness, body composition, blood lipids and C-reactive protein in adolescents with obesity. Ann. Acad. Med. Singap 37 (4), 286–293.
Wu N., Bredin S. S. D., Jamnik V. K., Koehle M. S., Guan Y., Shellington E. M., et al. (2021). Association between physical activity level and cardiovascular risk factors in adolescents living with type 1 diabetes mellitus: A cross-sectional study. Cardiovasc Diabetol. 20 (1), 62. doi:10.1186/s12933-021-01255-0
Xing R., Huang W. Y., Sit C. H. (2021). Validity of accelerometry for predicting physical activity and sedentary time in ambulatory children and young adults with cerebral palsy. J. Exerc Sci. Fit. 19 (1), 19–24. doi:10.1016/j.jesf.2020.06.006
Yan Y., Liu J., Zhao X., Cheng H., Huang G., Mi J., et al. (2019). Regional adipose compartments confer different cardiometabolic risk in children and adolescents:: The China child and adolescent cardiovascular health study. Mayo Clin. Proc. 94 (10), 1974–1982. doi:10.1016/j.mayocp.2019.05.026
Zhan Y., Ma S., Chen C., Liu S., Zhang C., Cao Z., et al. (2017). Physical activity guidelines for children and youth in China. Chin. J. Evid. Based Pediatr. 12, 401–409. doi:10.3969/j.issn.1673-5501.2017.06.00
Zhu Z., Tang Y., Zhuang J., Liu Y., Wu X., Cai Y., et al. (2019). Physical activity, screen viewing time, and overweight/obesity among Chinese children and adolescents: An update from the 2017 physical activity and fitness in China-the youth study. BMC Public Health 19 (1), 197. doi:10.1186/s12889-019-6515-9
Keywords: physical activity, cardiometabolic risk, obesity, dose-response relationship, children and adolescents
Citation: Chen Z and Zhu L (2023) Dose–response relationship between physical activity and cardiometabolic risk in obese children and adolescents: A pre-post quasi-experimental study. Front. Physiol. 14:1070653. doi: 10.3389/fphys.2023.1070653
Received: 15 October 2022; Accepted: 09 January 2023;
Published: 19 January 2023.
Edited by:
Ahmad Alkhatib, University of Taipei, TaiwanReviewed by:
Richard Lewanczuk, University of Alberta, CanadaCopyright © 2023 Chen and Zhu. This is an open-access article distributed under the terms of the Creative Commons Attribution License (CC BY). The use, distribution or reproduction in other forums is permitted, provided the original author(s) and the copyright owner(s) are credited and that the original publication in this journal is cited, in accordance with accepted academic practice. No use, distribution or reproduction is permitted which does not comply with these terms.
*Correspondence: Lin Zhu, MTEyNTFAZ3pzcG9ydC5lZHUuY24=
Disclaimer: All claims expressed in this article are solely those of the authors and do not necessarily represent those of their affiliated organizations, or those of the publisher, the editors and the reviewers. Any product that may be evaluated in this article or claim that may be made by its manufacturer is not guaranteed or endorsed by the publisher.
Research integrity at Frontiers
Learn more about the work of our research integrity team to safeguard the quality of each article we publish.