- 1Department of Oral and Maxillofacial Surgery, Faculty of Dentistry, Universiti Kebangsaan Malaysia, Kuala Lumpur, Malaysia
- 2Department of Craniofacial Diagnostics and Biosciences, Faculty of Dentistry, Universiti Kebangsaan Malaysia, Kuala Lumpur, Malaysia
- 3Department of Oral and Maxillofacial Surgery, Peking University School of Stomatology, Beijing, China
Medication related osteonecrosis of the jaw (MRONJ) is a condition caused by inhibition of the osteoclast activity by the anti-resorptive and anti-angiogenic drugs. Clinically, there is an exposure of the necrotic bone or a fistula which fails to heal for more than 8 weeks. The adjacent soft tissue is inflamed and pus may be present as a result of the secondary infection. To date, there is no consistent biomarker that could aid in the diagnosis of the disease. The aim of this review was to explore the literature on the microRNAs (miRNAs) related to medication related osteonecrosis of the jaw, and to describe the role of each miRNA as a biomarker for diagnostic purpose and others. Its role in therapeutics was also searched. It was shown that miR-21, miR-23a, and miR-145 were significantly different in a study involving multiple myeloma patients as well as in a human-animal study while miR-23a-3p and miR-23b-3p were 12- to 14-fold upregulated compared to the control group in an animal study. The role of the microRNAs in these studies were for diagnostics, predictor of progress of MRONJ and pathogenesis. Apart from its potential diagnostics role, microRNAs have been shown to be bone resorption regulator through miR-21, miR-23a and miR-145 and this could be utilized therapeutically.
Introduction
Osteonecrosis of the jaw or more commonly known as medication related osteonecrosis (MRONJ) differs from osteonecrosis of other bones in relation to its pathophysiology and treatment. MRONJ occurs as a result of the adverse effect of the antiresorptive or the antiangiogenic drugs (Ruggiero et al., 2014). MRONJ was first documented by Marx, (2003).
The American Association of Oral and Maxillofacial Surgeons Position Paper in 2014 and 2022 (updated version) emphasized on the following “must have criteria” to fulfil the definition of MRONJ (Ruggiero et al., 2014; Ruggiero et al., 2022). The “must have” criteria include.
i. The patients must currently or previously received treatment with antiresorptive or in combination with immune modulators or antiangiogenic agents;
ii. The exposed bone or bone that may be probed through an intraoral or extraoral fistula(e) which has been persisted for more than 8 weeks; and
iii. No history of radiation therapy to the jaws or obvious metastatic disease to the jaws.
The Italian Society for Maxillofacial Surgery (SICMF) and the Italian Society of Oral Pathology Medicine (SIPMO) defined MRONJ as the adverse drug reaction described as the progressive destruction and death of bone that affects the mandible or maxilla of patients who were exposed to the treatment which consist the antiresorptive and antiangiogenic agents and in the absence of previous radiation treatment’ (Bedogni et al., 2012). This definition was affirmed by the Consensus Conference held at the Symposium of the Italian Society of Oral Pathology and Medicine (SIPMO) on 20 October 2018, in Ancona (IT) (Campisi et al., 2020).
In relation to the clinical characteristics, previous literature showed that patients at risk were mostly females, with mean age of 65.3 years and mandible was the most commonly affected jawbone (Cheng et al., 2005; McGowan et al., 2018).
Among the primary diseases reported in the literature, multiple myeloma showed the highest involvement, followed by breast cancer, osteoporosis and prostate cancer (McGowan et al., 2018). Other risk factors are described in the following section.
The clinical appearance of MRONJ can described according to the stage of the disease which were described as Stage 0 to Stage 3 (Ruggiero et al., 2014; Ruggiero et al., 2022).
Stage of MRONJ
There are three stages of MRONJ (Stage 1–3) (Ruggiero et al., 2014; Ruggiero et al., 2022).
Stage 0
Stage 0 is described as a potential precursor of MRONJ as it does not involve an exposed bone or a fistula. The symptoms include odontalgia, jaw pain, which may radiate to the temporomandibular joint region, maxillary sinus pain, or altered sensation of the maxilla and mandibular areas (Ruggiero et al., 2022). There may be presence of an intra or extraoral swelling and mobility of a tooth which could not be associated with periodontal disease. There are changes in the dentoalveolar area which could not be related to any established oral diseases such as loss of the alveolar bone, changes to the trabecular pattern of the sclerotic bone and no new bone formation in the extraction socket, presence of osteosclerosis regions and thickening of the periodontal ligament.
Stage 1
There is an exposed necrotic bone or a fistula with no evidence inflammation. The radiographic findings may be similar to Stage 0 and localized to the alveolar bone region.
Stage 2
There is an exposed necrotic bone or a fistula with evidence of infection and inflammation these patients are symptomatic. The radiographic findings may be similar to Stage 0 and localized to the alveolar bone region.
Stage 3
There is an exposed and necrotic bone or fistulae that probes to the bone, with evidence of infection, and one or more of the complications such as exposed necrotic bone extending beyond the region of the alveolar bone, pathological fracture, extraoral fistula, oral antral or oral-nasal communication or osteolysis extending to the inferior border of the mandible or sinus floor.
Treatment of MRONJ can be either conservative or non-surgical or surgery. Conservative management concentrates on patient education and motivation, reassurance, control of pain and infection (Ruggiero et al., 2022) while surgical intervention involves removing the infected areas and this require margins beyond the borders of the necrotic bone to an area of vital, bleeding bone (Ruggiero et al., 2022). The prognosis of the disease depends on the cancer type and MRONJ among multiple myeloma patients showed poorer healing compared to breast and prostate cancers (Wei et al., 2021).
Epidemiology of MRONJ
The prevalence of MRONJ among osteoporotic patients on bisphosphonate (BP) was shown to be between 0% and 0.04%, and most reports showed low prevalence of less than 0.001% (Hong et al., 2010; Khan et al., 2011; Urade et al., 2011; Lee et al., 2013). In patients with malignant bone diseases where higher dose of BP was administered, the prevalence was reported to be between 0% and 0.186% (Aragon-Ching et al., 2009; Baqain et al., 2010; Assaf et al., 2013).
The cumulative incidence rate between 2012 and 2014 was shown to be 20.9 per 100,000 person-years (Kim et al., 2021). With regards to denosumab, the incidence of MRONJ ranged from 0 to 30.2 per 100,000 patient-years (Cummings et al., 2009; Orwoll et al., 2012; Papapoulos et al., 2012; Bone et al., 2013).
In relation to route of administration, the incidence of MRONJ ranged from 1.04 to 1.69 per 100,000 patient-years amongst those who were treated with oral antiresorptive agents (Aragon-Ching et al., 2009; Baqain et al., 2010; Fizazi et al., 2011; Hoff et al., 2011; Khan et al., 2011; Urade et al., 2011; Assaf et al., 2013; Lee et al., 2013; Ulmner et al., 2014). The incidence was shown to be higher when intravenous bisphosphonates was administered, i.e., from 0 to 90 per 100,000 patient-years (Lyles et al., 2007; Tennis et al., 2012; Sieber et al., 2013). Regarding the duration of treatment, it was observed that most patients developed MRONJ after receiving BPs for more than a year (Auzina et al., 2019). The incidence was reported to increase to 0.21% if the drugs were consumed for a period of more than 4 years (Pazianas et al., 2007).
Pathophysiology
MRONJ is a unique type of osteonecrosis which involves the maxilla and mandible (Aghaloo et al., 2015). The paradox of MRONJ pathophysiology led to the following theories, 1) the inhibition of osteoclastic bone resorption and remodelling 2) inhibition of angiogenesis 3) inflammation and infection 4) immune dysfunction 5) soft tissue toxicity (Ruggiero et al., 2014; Aghaloo et al., 2015).
Theory of altered bone remodelling
Osteoclast is the target cell for the treatment of the metastatic bone malignancy and osteoporosis. The medication, such as the BPs, targets the osteoclast and inhibits its function by specific mechanism, therefore the altered bone remodelling is the fundamental hypothesis for MRONJ (Kimmel, 2007).
The BPs are grouped into 1) non-nitrogen-containing bisphosphonates, for example,: etidronate, clodronate, and tiludronate and 2) nitrogen-containing bisphosphonates such as alendronate, risedronate, ibandronate, pamidronate, and zoledronic acid with different mechanism on the osteoclastic apoptosis pathway. Generally, the BPs exert its effect on the osteoclasts by inhibiting bone calcification and hydroxyapatite breakdown (Drake et al., 2008).
Denosumab, another antiresorptive that is designed to have strong affinity towards receptor activator of nuclear factor-κ signalling/osteoprotegerin (RANK/RANKL/OPG) of the osteoclasts. By binding to this receptor, denosumab blocks the formation and activation of the osteoclasts (Baud’huin et al., 2007). Both denusomab and BPs have detrimental effect on the osteoclasts but in different forms of mechanism. These drugs not only induce osteoclast apoptosis but also affect the cytoskeletal disruption, changing intracellular protein traffic, and blocking the intracellular signal transduction pathways (Reszka & Rodan, 2003). Inhibition of bone remodelling and resorption will then affect the ability of the bone to undergo physiological process of repair following injury. This will consequently lead to necrosis of the bone (Lee et al., 2016).
Theory of angiogenesis inhibition
Zolendronic acid directly inhibits angiogenesis and together with vascular impairment, this could lead to the development of MRONJ (Chang et al., 2018). The mechanism of action of angiogenesis inhibition is by interfering with the adhesion and migration of human endothelial cells to interrupt tumour invasion and metastases (McLeod et al., 2012). On the other hand, the antiangiogenic medications, such as the VEGF inhibitors, tyrosine kinase receptor inhibitors, and immunomodulatory drugs were also shown to be associated with MRONJ (Gacche & Meshram, 2014; Akita et al., 2018; Vallina et al., 2019). Tyrosine kinase receptor family is involved in tumor growth, pathological angiogenesis and the progression (metastasis) of cancer. Sunitinib inhibits members of the tyrosine kinase receptor family including platelet-derived growth receptors (PDGFRD and PDGFRE), vascular endothelial growth factors (VEGFR1, VEGFR2 and VEGFR3), stem cell factor receptor KIT, tyrosine kinase type 3 (FLT3), the colony stimulating factor 1R (CSF-1R) and the neurotrophic factor receptor derived from the glial cell line (RET) (Hoefert and Eufinger, 2010; Ramírez et al., 2015).
MRONJ was more commonly observed in patients treated in combination between sunitinib and zolendronic acid rather than sunitib alone (Vallina et al., 2019), and MRONJ was more evident with zolendronic acid-sunitinib and not with zolendronic acid and sorafenib, pazopanib, temsirolimus or immunotherapy based on IL-2 (Smidt-Hansen et al., 2013; Ripamonti et al., 2016). This is because sunitinib is more potent against VEGFR and PDGF than the other drugs (Koch et al., 2011; Fusco et al., 2015). In addition, this drug can also cause gingival inflammation and mucositis, delayed wound healing and infection (Vallina et al., 2019).
It is generally accepted that by targeting vascular signalling molecules such as vascular endothelial growth factor (VEGF), the process of tumour invasion and metastases could be inhibited (Zalavras & Lieberman, 2014). However, long term exposure to these agents not only affecting the diseased tissue but also the normal tissue (Zalavras & Lieberman, 2014).
Physiologically, vast formation of new blood vessels will take place after injury in the bone as angiogenesis is the key component of bone repair. During bone repair, a period of necrosis and hypoxia is considered normal phase of healing. New blood vessels bring oxygen and nutrients to the highly metabolically active regenerating callus as well as inflammatory cells, cartilage and bone precursor cells to reach the injury site. However, when this process of vascularization is altered or disrupted, pathologic conditions of healing takes place and this is when necrosis of the bone is inevitable (Lee et al., 2016).
Theory of inflammation and infection
This theory was initially proposed following reports from several animal studies (Gotcher and Jee, 1981; Mawardi et al., 2009; Aghaloo et al., 2011; Lopez-Jornet et al., 2011; Aguirre et al., 2012; Kang et al., 2013). Animals on antiresorptive treatment that had extraction of the diseased teeth showed impaired healing with mucosal defects, prominent inflammatory infiltrate in the sockets, bone exposure, and areas of osteonecrosis (Aghaloo et al., 2014). Previously, Aghaloo et al. (2011) showed osteocyte necrosis precedes clinical bone exposure in their rat model of BRONJ with aggresive periodontal disease. Extraction of such teeth would injure the surrounding gingival tissues and expose the necrotic bone.
Another study investigated the effect of progressive periodontal disease and MRONJ in the osteoporotic rat which had bisphosphonates intraperitoneally (Li et al., 2016). While alveolar bone resorption from progressive periodontal disease was inhibited, the risk of developing BRONJ was shown to be high (Li et al., 2016).
Hallmer et al. explored the relation between oral flora and MRONJ using the 16S ribosomal RNA pyrosequencing techniques and showed that anaerobic bacteria representative of periodontal microflora, has an effect on the initiation of MRONJ (Hallmer et al., 2017).
Mice that had extractions of healthy teeth exhibited normal mucosal healing and woven bone formation in the sockets. It was also shown that BRONJ did not develop after dental extraction preceded by antibiotic prophylaxis and followed by mucoperiosteal closure in mice receiving bisphosphonates (Abtahi et al., 2013).
In a clinical study that investigated the oral microbiome composition among patients with BRONJ, patients taking bisphosphonates without ONJ, and healthy controls (Kalyan et al., 2015). There was no difference in the oral bacterial community among the groups. Instead, the composition of the oral microbiota across individuals was strongly associated with the function of their systemic leukocytes. Gene expression for RANK, TNF-alpha, and aryl hydrocarbon receptor by peripheral blood leukocytes was found related to the composition of the oral microbiota. These genes expressed by leukocytes are related to immune and stress resiliency (Nguyen et al., 2013; Walsh and Choi, 2014).
Theory of immune dysfunction
Altered numbers and patterns of T-cells in human and rat MRONJ necrotic bone samples was profound compared to healthy patients and non-MRONJ sites (Qu et al., 2020).
The T cells in the form of alpha beta T cells and gamma delta T cells recognize antigen differently and the role for gamma delta T cells in antimicrobial immunity has been firmly established (Kaufmann, 1996).
The alpha beta T cells were found to stimulate bone loss, while the innate lymphocytes, the gamma delta T cells, were found essential for bone regeneration (Ono et al., 2016). These gamma delta T cells produce interleukin-17A (IL-17A), which promotes bone formation and fracture healing (Kalyan, 2016). The association between MRONJ and deficiency of gamma delta T cells was shown by Kalyan et al. (2013). This study found a significant loss of gamma delta T cells in osteoporotic patients on bisphosphonate therapy. Apart from deficiency of gamma delta T cells, the patients also had underlying conditions that compromised their immune integrity (Kalyan, 2016).
A comprehensive review about the local mucosal and bone immune response in relation to MRONJ can be read from Zhang et al. (2021).
Theory of soft tissue toxicity
BPs have been shown to be toxic to both oral keratinocytes and fibroblasts, and negatively affect cell cell viability, apoptosis, proliferation and migration (Landesberg et al., 2008; Kobayashi et al., 2010; Cozin et al., 2011; Bullock et al., 2022). During cell proliferation which is crucial wound healing and restoration of the mucosal barrier, keratinocytes were prevented from completing their cell cycles while fibroblasts became apoptotic when treated with BPs (Bullock et al., 2022). In addition, disrupted transforming growth factor beta 1 (TGFβ1) signaling was discovered with delayed periodontal repair in BRONJ human biopsy tissue (Wehrhan et al., 2011). The loss of TGFβ1-related cellular activation in BRONJ-affected oral mucosa connective tissue could describe the delayed wound healing and lack of mucosal regeneration in BRONJ lesions (Wehrhan et al., 2011).
Risk factors for MRONJ
McGowan et al. (2018) in their systematic review listed the medical and dental risk factors for MRONJ. Dental extraction and periodontal disease were the most commonly reported dental risk factors while chemotherapy, corticosteroids and smoking were the most frequently reported medical risk factors (McGowan et al., 2018). It is the concurrent infection, and not the tooth extraction, that acts as the key risk factor for the development of MRONJ (Otto et al., 2015; Soutome et al., 2018; Hasegawa et al., 2019). In addition, dental extraction with additional osteotomy (Bodem et al., 2015), and root amputation (Hasegawa et al., 2019), increased the risk of MRONJ.
In contrast to the review reported by McGowan et al. (2018), corticosteroids and chemotherapy did not show significant association with the occurrence of MRONJ (Bodem et al., 2015; Hasegawa et al., 2019). Smoking and alcohol tobacco also did not show associations with MRONJ (Yamazaki et al., 2012; Soutome et al., 2018; Hasegawa et al., 2019).
In relation to the medication of concern, higher risk was shown when the medication had been ongoing for 8 months or more (Hasegawa et al., 2019). The risk ratio for high-dose of intravenous medication versus low-dose oral administration was 14.6 (95% confidence interval 1.7–125.8) (Yamazaki et al., 2012). Studies also showed that the risk of MRONJ was greater when the anti-angiogenic drugs were combined with the BPs (Christodoulou et al., 2009; Fusco et al., 2015).
High susceptibility of the jaw bones to MRONJ
The maxillary and mandibular bones originated from the neural crest origin channels (Cheng et al., 2009). The alveolar bone is a part of the maxillary and the mandibular bone and its main function is to support the teeth. In comparison to the limbs and vertebra column, the alveolar bone has a dynamic structure where the bone constantly remodel and adapt to the functional needs (Cheng et al., 2009). Unlike the limbs and vertebral column, the alveolar bone develops as a membrane bone. Significantly, the origin of the alveolar bone influences the phenotype of the osteoblasts and osteoclasts (Kasperk et al., 1995). Osteoblasts from the alveolar bone have an increased rate of cell division in comparison to other bones (Hall, 1999). The behaviour and biochemical structure of osteoclasts originated from alveolar bone are also different from the long bones (Hall, 1999). The overall rate of turnover of the alveolar bone was shown to be ten times greater than that of the long bones (Dixon et al., 1997). The reason for rapid alveolar bone remodeling is mainly due to the mechanical loading stimulus (forces) from mastication (Halldin et al., 2011; Inoue et al., 2019) Bisphosphonates inhibit normal bone remodeling, resulting in the accumulation of microdamage and impaired mechanical properties of the bone (Bertoldo et al., 2007; Weinstein et al., 2009). This decrease in bone remodeling despite the microdamage induced by chewing and local inflammation results in constant exposure of bone to pathogenic microorganisms. These conditions predispose bone tissue to necrosis (Hoefert et al., 2010).
Dilemma in elucidating MRONJ diagnostic markers
MRONJ is diagnosed clinically when there is an exposure of the bone or fistula in the oral cavity or externally. Precaution could be taken earlier prior to this clinical appearance. Despite several theories describing the possible pathophysiology, at present, there is no specific and reliable diagnostic marker available.
There were many biomarkers that have been proposed to support the diagnosis of MRONJ, for example, Receptor Activator of NF-kB Ligand (RANKL) and osteoprotegerin (OPG) (Bagan et al., 2017), total alkaline phosphatase, bone-specific alkaline phosphatase (BAP), osteocalcin, C-telopeptide (CTX), vascular endothelial growth factor (VEGF), triiodothyronine (T3), thyroxine (T4), thyroid-stimulating hormone (TSH), 25-hydroxyvitamin D, and C-reactive protein (Thumbigere-Math et al., 2016), CTX, undercarboxylated Osteocalcin (Glu-OC), Tartrate-resistant acid phosphatase 5b (TRACP 5b), RANKL, and OPG (Kim et al., 2016) and N-telopeptide (NTX) and BAP (Kolokythas et al., 2015), however, none could provide consistent diagnostic results (Yang et al., 2018). Recently, researchers from Spain showed an important discovery through the proteomic and bioinformatics analyses where three potential protein biomarkers, MMP9, AACT, and HBD were identified (Lorenzo-Pouso et al., 2022).
Novel approach has been proposed to diagnose diseases using circulating microRNAs, which have been shown with high specificity and stability in the body fluids (Turchinovich et al., 2012). MicroRNA biomarkers can be used to indicate presence of a pathology or the stage, progression, or genetic link of pathogenesis (Kocerha et al., 2011; Wang et al., 2011; Weir et al., 2011; Elfimova et al., 2012; Li et al., 2013; Recchioni et al., 2013; Scott et al., 2015; Wang et al., 2015; Biswas, 2018; Hu et al., 2018). The diagnostic performances of the circulating microRNAs have been validated such as in hepatocellular carcinoma (Zhou et al., 2011), heart diseases (Egea et al., 2012) and osteoporosis (Hackl et al., 2016).
In relation to medical intervention, there are some clinical breakthrough reported in various areas. Firstly, Miravirsen, a locked nucleic acid (LNA)-modified antimiR-122 that effectively combats hepatitis C virus infection (Janssen et al., 2013). Secondly, RG-012, an antagomir against miR-21, which reduces fibrogenesis of organs associated with Alport syndrome (Gomez et al., 2015), an X-linked disease which is characterized by hearing loss, visual impairment and kidney disease caused by mutations of the COL4A5 gene (Genetics Home Reference, 2020). Thirdly, Remlarsen or MRG-201, a miRNA mimic that is used for the treatment of pathological cutaneous fibrosis, or other fibrotic diseases, such as idiopathic pulmonary fibrosis (Gallant-Behm et al., 2019). The mechanism of action is by restoring the levels of miR-29b, which is a negative regulator of the extracellular matrix deposition processes (Gallant-Behm et al., 2019). Finally, Cobomarsen (or MRG-106), a LNA antagomir that targets miR-155 which is the focus of treatment for certain types of lymphoma and leukemia (Seto et al., 2018), and Mesomir, a miRNA mimic that could replace miR-16, a tumor suppressor that is reduced in malignant pleural mesothelioma (Reid et al., 2013).
The aim of this review is to describe the role of each miRNA as a biomarker for diagnostic purposes and others. The potentials of miRNAs in therapeutic modalities were also searched.
MicroRNA
MicroRNAs (miRNAs) are a group of non-protein-coding RNAs comprising 19–25 nucleotides, which are highly preserved during evolution (Parikh et al., 2014). miRNAs control gene expression post-transcriptionally by binding to various mRNA targets (Sotiropoulou et al., 2009).
MicroRNA and the osteoclasts
The BPs act on the mature osteoclasts by either inactivation or increased apoptosis of the osteoclasts and suppress resorption while denosumab is a fully human monoclonal anti-RANKL antibody that inhibits binding of RANKL to RANK, blocks osteoclast maturation, function and survival, thus reducing bone resorption (Hanley et al., 2012).
miRNAs play a central role in the process of osteoclast growth, differentiation, apoptosis, and bone resorption (Musolino et al., 2018). During normal physiological conditions, osteoclastogenesis is regulated by the osteoblasts and stromal cells, both of which provide two essential factors, macrophage colony-stimulating factor (M-CSF) and receptor activator of nuclear factor NF-ĸB ligand (RANKL) (Ji et al., 2016).
The following miRNAs were expressed in promoting osteoclastogenesis: miR-29, miR-31-5p, hsa-miR-422a, hsa-miR-148a-3p, miR-183-5p, miR-214-3p and miR-9718, while the following miRNAs inhibit osteoclastogenesis: miR-7b-5p, miR-26a-5p, miR-34a-5p, miR-124-3p, miR-125a-5p, miR-146a-5p, miR-218-5p and miR-503-5p (Ge et al., 2017). In addition, three miRNAs were shown with the promotion and inhibition effects: miR-21-5p, hsa-miR-133a-3p and miR-223-3p (Ji et al., 2016) (Table 1).
MicroRNA and MRONJ
Evidence of the expression of miRNA and MRONJ can be observed from both human and animal studies. Their characteristics are shown in Table 2.
Human studies
There were two studies that used humans as subjects. In the first study, miRNA was investigated in multiple myeloma patients with and without MRONJ. The following miRNAs were significantly over-expressed in MRONJ patients versus controls: miR-16-1, miR-21, miR-23a, miR-28, miR-101-1, miR-124-1, miR-129, miR-139, miR-145, miR-149, miR-202, miR-221, miR-424, miR-520. Of the above miRNAs, six were very significantly upregulated in their patients compared to the controls: miR-16-1, miR-149, miR-23a and miR-145, miR-129-1 and miR-221 (Musolino et al., 2018).
The second study also included human subjects concurrently with the animal study. The results were similar to the animals’ study where the level of miR-21 and miR-23a were elevated and miR-145 was declined (Yang et al., 2018).
Animal studies
Seven miRNAs (miR-21, miR-23a, miR-28, miR-124-1, miR-129-1, miR-145 and miR-149) from study Muslino et al. (2018) were selected by Yang et al. (2018) to be tested in their animal study. This study aimed to evaluate the diagnostic role and the prediction of MRONJ initiation of the miRNA (Yang et al., 2018). The validation was performed only in the animal study and not for human. They found that the expression of miR-21, miR-23a and miR-145 were significantly different when compared between MRONJ and the controls.
For prediction of MRONJ initiation, only miR-21 could distinguish between one of the MRONJ groups (MRONJ-4 weeks) from the control group. The authors concluded that a combined indice (from miR-21 + miR-23a + miR-145 logistic regression model) was a better diagnostic factor than the individual miRNA and it was also a potential predictor for MRONJ progress.
Another animal study analysed the extraction sockets in zolendronic acid treated rats and controls. This study aimed to investigate the role of miRNA in the pathogenesis of MRONJ. Upregulation of six miRNAs, i.e.,.miR-23a-3p, miR-23b-3p, miR27a-3p and miR-24-3p (these MiRNAs are associated with inhibition of osteoblast maturation) and miR-34-5p and miR-652-3p (anti-angiogenic activity) were shown. The expression of miR-23a-3p and miR-23b-3p were 12- to 14-fold upregulated compared to the control group (Surajkulwatana et al., 2020).
The summary of these studies are shown in Table 2.
The description of miR-21, miR-23a, miR-145 and miR-23b-3p are shown below.
miR-23a
miR-23a principally targets the interleukins (ILs) (Musolino et al., 2018), tumour necrosis factor, TNF-related targets (Musolino et al., 2018), Fos proto-oncogene (FOS) (Musolino et al., 2018), other genes involved in bone tissue homeostasis such as osteoclast stimulating factor 1 (OSTF1), secreted protein acidic and cysteine rich (SPARC), and SPOCK1 (Musolino et al., 2018) and low density lipoprotein receptor-related protein5 (LRP5) which is closely related with steroid-associated femoral head necrosis (Yang et al., 2018).
miR-23a inhibitor improves osteonecrosis in an animal model (Dong et al., 2017). Selective expression of miR-23a was shown in microvascular endothelial cells in vivo (Larsson et al., 2009). miR-23a may have some relation in angiogenesis as it is among the highly expressed miRNAs in the human umbilical vein endothelial cells (Poliseno et al., 2006).
miR-23b-3p
miR-23b-3p belongs to cluster of miR-23 of the microRNA family, which had been reported to be involved in osteoporosis and in regulation of differentiation of osteoblasts (Zeng et al., 2017; Liu et al., 2018). It was shown that miR-23b-3p regulates differentiation of osteoclasts by targeting the phosphatase and tensin (PTEN) gene (Chai et al., 2019).
miR-21
miR-21 targets Suppressor of Mothers against Decapentaplegic7 (Smad7), sprouty RTK signaling antagonist 1 (Spry1) and periodontal-ligament-associated protein-1 (PLAP1) to improve osteogenic differentiation (Santini et al., 2003; Oteri et al., 2008; Allegra et al., 2010). miR-21 determines upregulation of osteoclastogenesis via enhancing receptor activator of nuclear factor kappa-Β ligand (RANKL) and suppressing osteoprotegerin (OPG) expression (Alonci et al., 2007). miR-21 is related to particle induced osteolysis pathogenesis (Allegra et al., 2007), and knocking down miR-21 leads to osteoclastogenesis restriction (Katz et al., 2011). Circulating miR-21 is upregulated during MRONJ progress, which is consistent with miR-21 variation in pro-osteoclastogenesis (Yang et al., 2018).
miR-145
miR-145 principal targets are the ILs (Musolino et al., 2018) and bone homeostasis genes like the syntaxin binding protein 5 like (STXBP5L), osteomodulin, secreted protein acidic and cysteine rich (SPARC), and SPARC/osteonectin, cwcv and kazal-like domains proteoglycan 2 (SPOCK2) (Musolino et al., 2018).
In addition, the highly upregulated miRNAs and their target genes in the human study are shown in Table 3.
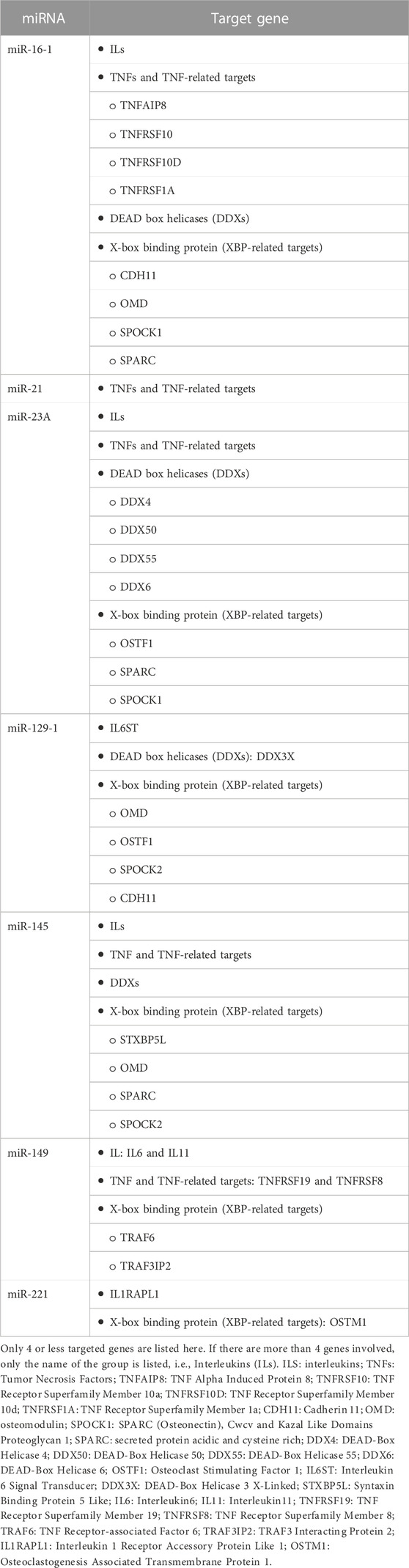
TABLE 3. The most upregulated miRNAs and their predicted targets (Musolino et al., 2018).
Table 3 below shows the miRNAs involved in MRONJ and the target genes in the human study. Only hub genes TNF, interleukin (IL), X-box binding protein (XBP), and DEAD box helicases (DDX) are included in this table as these genes and pathways might have a central action in the progression of MRONJ in multiple myeloma patients (Sun et al., 2016).
Future clinical use
MRONJ has been the subject of controversy ever since the first case was reported in 2003 (Ruggiero et al., 2014). A multidisciplinary approach plays a fundamental role in the management of this lesion (Ruggiero et al., 2014). Comprehensive dental care prior to commencement of the antiresorptive or antiangiogenic drug treatment considerably reduces the risk of developing MRONJ.
It is widely accepted that miRNAs play a pivotal role in osteogenic differentiation, including osteogenesis and cartilage growth (Maes et al., 2008) and have been shown to control numerous cellular processes such as differentiation, apoptosis, angiogenesis, and cell metabolism (Bartel, 2009).
Based from the literature described above, there are miRNAs that have been identified to be associated with MRONJ. More work is required so that miRNAs can be utilised as 1) a new diagnostic biomarker and 2) potential therapeutic modalities.
New diagnostic biomarker and therapeutic modality
Emerging evidences showed novel properties of miRNAs as biomarkers in diagnosing MRONJ (Musolino et al., 2018; Yang et al., 2018). Yang et al. (2018) concluded that the altered levels of miR-21, miR-23a and miR-145 were significant between MRONJ and control group and they were detectable as early as 1 week following induction of MRONJ (Yang et al., 2018).
miR-23a and miR-520 were shown under-expressed in patients with multiple myeloma without MRONJ and were over-expressed in MRONJ subjects (Musolino et al., 2018). This miRNAs control cell cycle and angiogenesis and may be used as treatment of MRONJ (Musolino et al., 2018). Blocking the expression of both miRNAs could be the new therapeutic for MRONJ. miR-23A inhibitor has been shown to improve osteonecrosis in an animal model (Dong et al., 2017).
Sun et al. (2016) reported the overexpression of miR-145 during osteoblast differentiation in a human study and Yu et al. (2018) also reported its role in inhibiting RANKL-induced monocytes related osteoclastogenesis in an animal study.
miR-21 was noted to promote osteogenic differentiation by targeting Smad7, Spry1, and PLAP1. miR-21 is also important in upregulating osteoclastogenesis via increasing RANKL and suppressing osteoprotegerin (OPG) expression while the knockdown of miR-21 inhibited osteoclastogenesis. During the progress of MRONJ, the circulating miR-21 was found to be upregulated (Yang et al., 2018).
The above showed a few potential miRNAs that could be utilised for diagnostic tool or therapeutic modality. Although miRNA-related studies in MRONJ are limited, a panel of miRNAs rather than single miRNA could be combined to increase diagnostic sensitivity and/or specificity (Hanna et al., 2019).
Limitation of the review
There are very few studies that investigate miRNA in relation to MRONJ either as a diagnostic marker or predictor of progress or role in the pathogenesis. Only one study investigated the role of miRNA using human subjects in the primary and validation phase. In addition, the sample size was also small.
Conclusion
At present, reliable and stable biomarkers for the diagnosis of MRONJ are still far from reality. Although obtaining the miRNA from the body fluid and tissue is extremely technically challenged, successful work on the multipe myeloma patients with MRONJ have been shown. Further exploration into the diagnostic and therapeutic potentials of miR-21, miR-23a, and miR-145, focusing on the reversal of the MRONJ, may produce a novel breakthrough.
Author contributions
RR: Conceptualization, Final draft. SSMY: Writing-original draft, editing and final draft. SHY: Editing and final draft. MAR: Editing and final draft. PX: Final draft. CG: Final draft.
Conflict of interest
The authors declare that the research was conducted in the absence of any commercial or financial relationships that could be construed as a potential conflict of interest.
Publisher’s note
All claims expressed in this article are solely those of the authors and do not necessarily represent those of their affiliated organizations, or those of the publisher, the editors and the reviewers. Any product that may be evaluated in this article, or claim that may be made by its manufacturer, is not guaranteed or endorsed by the publisher.
References
Abtahi, J., Agholme, F., and Aspenberg, P. (2013). Prevention of osteonecrosis of the jaw by mucoperiosteal coverage in a rat model. Int. J. Oral Maxillofac. Surg. 42, 632–636. doi:10.1016/j.ijom.2013.02.007
Aghaloo, T., Hazboun, R., and Tetradis, S. (2015). Pathophysiology of osteonecrosis of the jaws. Oral Maxillofac. Surg. Clin. North Am. 27 (4), 489–496. doi:10.1016/j.coms.2015.06.001
Aghaloo, T. L., Cheong, S., Bezouglaia, O., Kostenuik, P., Atti, E., Dry, S. M., et al. (2014). RANKL inhibitors induce osteonecrosis of the jaw in mice with periapical disease. J. Bone Min. Res. 29 (4), 843–854. doi:10.1002/jbmr.2097
Aghaloo, T. L., Kang, B., Sung, E. C., Shoff, M., Ronconi, M., Gotcher, J. E., et al. (2011). Periodontal disease and bisphosphonates induce osteonecrosis of the jaws in the rat. J. Bone Min. Res. 26 (8), 1871–1882. doi:10.1002/jbmr.379
Aguirre, J. I., Akhter, M. P., Kimmel, D. B., Pingel, J. E., Williams, A., Jorgensen, M., et al. (2012). Oncologic doses of zoledronic acid induce osteonecrosis of the jaw-like lesions in rice rats (Oryzomys palustris) with periodontitis. J. Bone Min. Res. 27 (10), 2130–2143. doi:10.1002/jbmr.1669
Akita, Y., Kuroshima, S., Nakajima, K., Hayano, H., Kanai, R., Sasaki, M., et al. (2018). Effect of anti-angiogenesis induced by chemotherapeutic monotherapy, chemotherapeutic/bisphosphonate combination therapy and anti-VEGFAmAb therapy on tooth extraction socket healing in mice. J. Bone Min. Metab. 36, 547–559. doi:10.1007/s00774-017-0872-1
Allegra, A., Alonci, A., Penna, G., Granata, A., Nastro, E., Oteri, G., et al. (2010). Bisphosphonates induce apoptosis of circulating endothelial cells in multiple myeloma patients and in subjects with bisphosphonate-induced osteonecrosis of the jaws. Acta Haematol. 124 (2), 79–85. doi:10.1159/000313787
Allegra, A., Oteri, G., Nastro, E., Alonci, A., Bellomo, G., Del Fabro, V., et al. (2007). Patients with bisphosphonates-associated osteonecrosis of the jaw have reduced circulating endothelial cells. Hematol. Oncol. 25 (4), 164–169. doi:10.1002/hon.819
Alonci, A., Allegra, A., Bellomo, G., Quartarone, E., Oteri, G., Nastro, E., et al. (2007). Patients with bisphosphonate-associated osteonecrosis of the jaw have unmodified levels of soluble vascular endothelial growth factor receptor 1. Leuk. Lymphoma 48 (9), 1852–1854. doi:10.1080/10428190701509806
Aragon-Ching, J. B., Ning, Y. M., Chen, C. C., Latham, L., Guadagnini, J. P., Gulley, J. L., et al. (2009). Higher incidence of Osteonecrosis of the Jaw (ONJ) in patients with metastatic castration resistant prostate cancer treated with anti-angiogenic agents. Cancer Invest. 27, 221–226. doi:10.1080/07357900802208608
Assaf, A. T., Smeets, R., Riecke, B., Weise, E., Gröbe, A., Blessmann, M., et al. (2013). Incidence of bisphosphonate-related osteonecrosis of the jaw in consideration of primary diseases and concomitant therapies. Anticancer Res. 33, 3917–3924.
Auzina, D., Slaidina, A., Sevastjanova, M., Erts, R., Lejnieks, A., and Lejniece, S. (2019). A population based study of multiple myeloma patients with medication-related osteonecrosis of the jaw. Stomatologija 21 (1), 13–17.
Bagan, L., Jimenez, Y., Leopoldo, M., Rubert, A., and Bagan, J. (2017). Serum levels of RANKL and OPG, and the RANKL/OPG ratio in bisphosphonate-related osteonecrosis of the jaw: Are they useful biomarkers for the advanced stages of osteonecrosis? Med. Oral Patol. Oral Cir. Bucal. 22, e542–e547. doi:10.4317/medoral.22128
Baqain, Z. H., Sawair, F. A., Tamimi, Z., Nazzal, B., Edwan, G. A., Almasad, J. K., et al. (2010). Osteonecrosis of jaws related to intravenous bisphosphonates: The experience of a Jordanian teaching hospital. Ann. R. Coll. Surg. Engl. 92, 489–494. doi:10.1308/003588410X12699663903395
Bartel, D. P. (2009). MicroRNAs: Target recognition and regulatory functions. Cell 136, 215–233. doi:10.1016/j.cell.2009.01.002
Baud’huin, M. L., Duplomb, L., Velasco, C. R., Fortun, Y., Heymann, D., and Padrines, M. (2007). Key roles of the OPG–RANK–RANKL system in bone oncology. Expert Rev. Anticancer Ther. 7 (2), 221–232. doi:10.1586/14737140.7.2.221
Bedogni, A., Fusco, V., Agrillo, A., and Campisi, G. (2012). Learning from experience. Proposal of a refined definition and staging system for bisphosphonate-related osteonecrosis of the jaw (BRONJ). Oral Dis. 18 (6), 621–623. doi:10.1111/j.1601-0825.2012.01903.x
Bertoldo, F., Santini, D., and Lo Cascio, V. (2007). Bisphosphonates and osteomyelitis of the jaw: a pathogenic puzzle. Nat Clin Pract Oncol. 4(12), 711–721. doi:10.1038/ncponc1000
Biswas, S. (2018). MicroRNAs as therapeutic agents: The future of the battle against cancer. Curr. Top. Med. Chem. 18, 2544–2554. doi:10.2174/1568026619666181120121830
Bodem, J. P., Kargus, S., Eckstein, S., Saure, D., Engel, M., Hoffmann, J., et al. (2015). Incidence of bisphosphonate-related osteonecrosis of the jaw in high-risk patients undergoing surgical tooth extraction. J. Cranio-Maxillofac. Surg. 43 (4), 510–514. doi:10.1016/j.jcms.2015.02.018
Bone, H. G., Chapurlat, R., Brandi, M. L., Brown, J. P., Czerwinski, E., Krieg, M. A., et al. (2013). The effect of three or six years of denosumab exposure in women with postmenopausal osteoporosis: Results from the FREEDOM extension. J. Clin. Endocrinol. Metab. 98, 4483–4492. doi:10.1210/jc.2013-1597
Bullock, G., Miller, C. A., McKechnie, A., and Hearnden, V. (2022). A review into the effects of pamidronic acid and zoledronic acid on the oral mucosa in medication-related osteonecrosis of the jaw. Front. Oral Health. 2, 822411. doi:10.3389/froh.2021.822411
Campisi, G., Mauceri, R., Bertoldo, F., Bettini, G., Biasotto, M., Colella, G., et al. (2020). Medication-related osteonecrosis of jaws (MRONJ) prevention and diagnosis: Italian Consensus update 2020. Int. J. Environ. Res. Public Health. 17 (16), 5998. doi:10.3390/ijerph17165998
Chai, J., Xu, L., and Liu, N. (2019). miR-23b-3p regulates differentiation of osteoclasts by targeting PTEN via the PI3k/AKT pathway. Arch. Med. Sci. 18 (6), 1542–1557. doi:10.5114/aoms.2019.87520
Chang, J., Hakam, A. E., and McCauley, L. K. (2018). Current understanding of the pathophysiology of osteonecrosis of the jaw. Curr. Osteoporos. Rep. 16 (5), 584–595. doi:10.1007/s11914-018-0474-4
Cheng, A., Daly, C. G., Logan, R. M., Stein, B., and Goss, A. N. (2009). Alveolar bone and the bisphosphonates. Aust. Dent. J. 54 (1), 51–61. doi:10.1111/j.1834-7819.2009.01143.x
Cheng, A., Mavrokokki, A., Carter, G., Stein, B., Fazzalari, N. L., Wilson, D. F., et al. (2005). The dental implications of bisphosphonates and bone disease. Aust. Dent. J. 50 (2), S4–S13. doi:10.1111/j.1834-7819.2005.tb00384.x
Christodoulou, C., Pervena, A., Klouvas, G., Galani, E., Falagas, M. E., Tsakalos, G., et al. (2009). Combination of bisphosphonates and antiangiogenic factors induces osteonecrosis of the jaw more frequently than bisphosphonates alone. Oncology 76, 209–211. doi:10.1159/000201931
Cozin, M., Pinker, B. M., Solemani, K., Zuniga, J. M., Dadaian, S. C., Cremers, S., et al. (2011). Novel therapy to reverse the cellular effects of bisphosphonates on primary human oral fibroblasts. J. Oral Maxillofac. Surg. 69, 2564–2578. doi:10.1016/j.joms.2011.03.005
Cummings, S. R., San Martin, J., McClung, M. R., Siris, E. S., Eastell, R., Reid, I. R., et al. (2009). Denosumab for prevention of fractures in postmenopausal women with osteoporosis. N. Engl. J. Med. 361, 756–765. doi:10.1056/NEJMoa0809493
Dixon, R. B., Trickler, N. D., and Garetto, L. P. (1997). Bone turnover in elderly canine mandible and tibia. J. Dent. Res. 76, 2579.
Dong, Y., Li, T., Li, Y., Ren, S., Fan, J., and Weng, X. (2017). MicroRNA-23a-3p inhibitor decreases osteonecrosis incidence in a rat model. Mol. Med. Rep. 16, 9331–9336. doi:10.3892/mmr.2017.7808
Drake, M. T., Clarke, B. L., and Khosla, S. (2008). Bisphosphonates: Mechanism of action and role in clinical practice. Mayo Clin. Proc. 83 (9), 1032–1045. doi:10.4065/83.9.1032
Egea, V., Schober, A., and Weber, C. (2012). Circulating miRNAs: Messengers on the move in cardiovascular disease. Thromb. Haemost. 108, 590–591. doi:10.1160/TH12-08-0613
Elfimova, N., Schlattjan, M., Sowa, J. P., Dienes, H. P., Canbay, A., and Odenthal, M. (2012). Circulating microRNAs: Promising candidates serving as novel biomarkers of acute hepatitis. Front. Physiol. 3, 476. doi:10.3389/fphys.2012.00476
Fizazi, K., Carducci, M., Smith, M., Damião, R., Brown, J., Karsh, L., et al. (2011). Denosumab versus zoledronic acid for treatment of bone metastases in men with castration-resistant prostate cancer: A random ised, double-blind study. Lancet 377, 813–822. doi:10.1016/S0140-6736(10)62344-6
Fusco, V., Porta, C., Saia, G., Paglino, C., Bettini, G., Scoletta, M., et al. (2015). Osteonecrosis of the jaw in patients with metastatic renal cell cancer treated with bisphosphonates and targeted agents: Results of an Italian multicenter study and review of the literature. Clin. Genitourin. Cancer. 13, 287–294. doi:10.1016/j.clgc.2014.12.002
Gacche, R. N., and Meshram, R. J. (2014). Angiogenic factors as potential drug target: Efficacy and limitations of anti-angiogenic therapy. Biochim. Biophys. Acta 1846 (1), 161–179. doi:10.1016/j.bbcan.2014.05.002
Gallant-Behm, C. L., Piper, J., Lynch, J. M., Seto, A. G., Hong, S. J., Mustoe, T. A., et al. (2019). A microRNA-29 mimic (Remlarsen) represses extracellular matrix expression and fibroplasia in the skin. J. Invest. Dermatol. 139 (5), 1073–1081. doi:10.1016/j.jid.2018.11.007
Ge, C., Zhao, G., Li, B., Li, Y., Cawthorn, W. P., MacDougald, O. A., et al. (2017). Genetic inhibition of PPAR. S112 phosphorylation reduces bone formation and stimulates marrow adipogenesis. Bone 26 (107), 1–9. doi:10.1016/j.bone.2017.10.023
Genetics Home Reference (2020). Alport syndrome. Available at: https://ghr.nlm.nih.gov/condition/alport-syndrome#inheritance (Accessed July 7, 2020).
Gomez, I. G., MacKenna, D. A., Johnson, B. G., Kaimal, V., Roach, A. M., Ren, S., et al. (2015). Anti-microRNA-21 oligonucleotides prevent Alport nephropathy progression by stimulating metabolic pathways. J. Clin. Investig. 125 (1), 141–156. doi:10.1172/JCI75852
Gotcher, J. E., and Jee, W. S. (1981). The progress of the periodontal syndrome in the rice rat. I. Morphometric and autoradiographic studies. J. Periodontal Res. 16, 275–291. doi:10.1111/j.1600-0765.1981.tb00976.x
Hackl, M., Heilmeier, U., Weilner, S., and Grillari, J. (2016). Circulating microRNAs as novel biomarkers for bone diseases - Complex signatures for multifactorial diseases? Mol. Cell Endocrinol. 432, 83–95. doi:10.1016/j.mce.2015.10.015
Halldin, A., Jimbo, R., Johansson, C. B., Wennerberg, A., Jacobsson, M., Albrektsson, T., et al. (2011). The effect of static bone strain on implant stability and bone remodeling. Bone 49 (4), 783–789. doi:10.1016/j.bone.2011.07.003
Hallmer, F., Bjornland, T., Andersson, G., Becktor, J. P., Kristoffersen, A. K., and Enersen, M. (2017). Bacterial diversity in medication-related osteonecrosis of the jaw. Oral Surg. Oral Med. Oral Pathol. Oral Radiol. 123, 436–444. doi:10.1016/j.oooo.2016.11.011
Hanley, D. A., Adachi, J. D., Bell, A., and Brown, V. (2012). Denosumab: Mechanism of action and clinical outcomes. Int. J. Clin. Pract. 66 (12), 1139–1146. doi:10.1111/ijcp.12022
Hanna, J., Hossain, G. S., and Kocerha, J. (2019). The potential for microRNA therapeutics and clinical research. Front. Genet. 10, 478. doi:10.3389/fgene.2019.00478
Hasegawa, T., Hayashida, S., Kondo, E., Takeda, Y., Miyamoto, H., Kawaoka, Y., et al. (2019). Medication-related osteonecrosis of the jaw after tooth extraction in cancer patients: A multicenter retrospective study. Osteoporos. Int. 30 (1), 231–239. doi:10.1007/s00198-018-4746-8
Hoefert, S., and Eufinger, H. (2010). Sunitinib may raise the risk of bisphosphonate-related osteonecrosis of the jaw: Presentation of three cases. Oral Surg. Oral Med. Oral Pathol. Oral Radiol. Endod. 110, 463–469. doi:10.1016/j.tripleo.2010.04.049
Hoefert, S., Schmitz, I., Tannapfel, A., and Eufinger, H. H. (2010). Importance of microcracks in etiology of bisphosphonate-related osteonecrosis of the jaw: A possible pathogenetic model of symptomatic and non-symptomatic osteonecrosis of the jaw based on scanning electron microscopy findings. Clin. Oral Invest. 14 (3), 271–284. doi:10.1007/s00784-009-0300-6
Hoff, A. O., Toth, B., Hu, M., Hortobagyi, G. N., and Gagel, R. F. (2011). Epidemiology and risk factors for osteonecrosis of the jaw in cancer patients. Ann. N.Y. Acad. Sci. 1218, 47–54. doi:10.1111/j.1749-6632.2010.05771.x
Hong, J. W., Nam, W., Cha, I. H., Chung, S. W., Choi, H. S., Kim, K. M., et al. (2010). Oral bisphosphonate-related osteonecrosis of the jaw: The first report in asia. Osteoporos. Int. 21, 847–853. doi:10.1007/s00198-009-1024-9
Hu, W., Tan, C., He, Y., Zhang, G., Xu, Y., and Tang, J. (2018). Functional miRNAs in breast cancer drug resistance. Onco. Targets Ther. 11, 1529–1541. doi:10.2147/OTT.S152462
Inoue, M., Ono, T., Kameo, Y., Sasaki, F., Ono, T., Adachi, T., et al. (2019). Forceful mastication activates osteocytes and builds a stout jawbone. Sci. Rep. 9, 4404. doi:10.1038/s41598-019-40463-3
Janssen, H. L., Reesink, H. W., Lawitz, E. J., Zeuzem, S., Rodriguez-Torres, M., Patel, K., et al. (2013). Treatment of HCV infection by targeting microRNA. N. Engl. J. Med. 368, 1685–1694. doi:10.1056/NEJMoa1209026
Ji, X., Chen, X., and Yu, X. (2016). MicroRNAs in osteoclastogenesis and function: Potential therapeutic targets for osteoporosis. Int. J. Mol. Sci. 17, 349. doi:10.3390/ijms17030349
Kalyan, S. (2016). It may seem inflammatory, but some Tcells are innately healing to the bone. J. Bone Min. Res. 31 (11), 1997–2000. doi:10.1002/jbmr.2875
Kalyan, S., Quabius, E. S., Wiltfang, J., Monig, H., and Kabelitz, D. (2013). Can peripheral blood gammadelta T cells predict osteonecrosis of the jaw? An immunological perspective on the adverse drug effects of aminobisphosphonate therapy. J. Bone Min. Res. 28 (4), 728–735. doi:10.1002/jbmr.1769
Kalyan, S., Wang, J., Quabius, E. S., Huck, J., Wiltfang, J., Baines, J. F., et al. (2015). Systemic immunity shapes the oral microbiome and susceptibility to bisphosphonate-associated osteonecrosis of the jaw. J. Transl. Med. 13, 212. doi:10.1186/s12967-015-0568-z
Kang, B., Cheong, S., Chaichanasakul, T., Bezouglaia, O., Atti, E., Dry, S. M., et al. (2013). Periapical disease and bisphosphonates induce osteonecrosis of the jaws in mice. J. Bone Min. Res. 28, 1631–1640. doi:10.1002/jbmr.1894
Kasperk, C., Wergedahl, J., Strong, D., Farley, J., Wangerin, K., Gropp, H., et al. (1995). Human bone cell phenotypes differ depending on their skeletal site of origin. J. Clin. Endocrinol. Metab. 80, 2511–2517. doi:10.1210/jcem.80.8.7629252
Katz, J., Gong, Y., Salmasinia, D., Hou, W., Burkley, B., Ferreira, P., et al. (2011). Genetic polymorphisms and other risk factors associated with bisphosphonate induced osteonecrosis of the jaw. Int. J. Oral Maxillofac. Surg. 40 (6), 605–611. doi:10.1016/j.ijom.2011.02.002
Kaufmann, S. H. (1996). gamma/delta and other unconventional T lymphocytes: what do they see and what do they do? Proc. Natl. Acad. Sci. U.S.A. 93 (6), 2272–2279. doi:10.1073/pnas.93.6.2272
Khan, A. A., Rios, L. P., Sándor, G. K. B., Khan, N., Peters, E., Rahman, M. O., et al. (2011). Bisphosphonate-associated osteonecrosis of the jaw in ontario: A survey of oral and maxillofacial surgeons. J. Rheumatol. 38, 1396–1402. doi:10.3899/jrheum.100221
Kim, J. W., Cha, I. H., Kim, S. J., and Kim, M. R. (2016). Biomarkers for bisphosphonate-related osteonecrosis of the jaw. Clin. Implant Dent. Relat. Res. 18, 281–291. doi:10.1111/cid.12297
Kim, S. H., Lee, Y. K., Kim, T. Y., Ha, Y. C., Jang, S., and Kim, H. Y. (2021). Incidence of and risk for osteonecrosis of the jaw in Korean osteoporosis patients treated with bisphosphonates: A nationwide cohort study. Bone 143, 115650. doi:10.1016/j.bone.2020.115650
Kimmel, D. B. (2007). Mechanism of action, pharmacokinetic and pharmacodynamic profile, and clinical applications of nitrogen-containing bisphosphonates. J. Dent. Res. 86 (11), 1022–1033. doi:10.1177/154405910708601102
Kobayashi, Y., Hiraga, T., Ueda, A., Wang, L., Matsumoto-Nakano, M., Hata, K., et al. (2010). Zoledronic acid delays wound healing of the tooth extraction socket, inhibits oral epithelial cell migration, and promotes proliferation and adhesion to hydroxyapatite of oral bacteria, without causing osteonecrosis of the jaw, in mice. J. Bone Min. Metab. 28, 165–175. doi:10.1007/s00774-009-0128-9
Kocerha, J., Kouri, N., Baker, M., Finch, N., DeJesus-Hernandez, M., Gonzalez, J., et al. (2011). Altered microRNA expression in frontotemporal lobar degeneration with TDP-43 pathology caused by progranulin mutations. BMC Genomics 12, 527. doi:10.1186/1471-2164-12-527
Koch, F. P., Walter, C., Hansen, T., Jäger, E., and Wagner, W. (2011). Osteonecrosis of the jaw related to sunitinib. J. Oral Maxillofac. Surg. 15, 63–66. doi:10.1007/s10006-010-0224-y
Kolokythas, A., Karras, M., Collins, E., Flick, W., Miloro, M., and Adami, G. (2015). Salivary biomarkers associated with bone deterioration in patients with medication-related osteonecrosis of the jaws. J. Oral Maxillofac. Surg. 73, 1741–1747. doi:10.1016/j.joms.2015.03.034
Landesberg, R., Cozin, M., Cremers, S., Woo, V., Kousteni, S., Sinha, S., et al. (2008). Inhibition of oral mucosal cell wound healing by bisphosphonates. J. Oral Maxillofac. Surg. 66, 839–847. doi:10.1016/j.joms.2008.01.026
Larsson, E., Fredlund Fuchs, P., Heldin, J., Barkefors, I., Bondjers, C., Genové, G., et al. (2009). Discovery of microvascular miRNAs using public gene expression data: miR-145 is expressed in pericytes and is a regulator of Fli1. Genome Med. 1 (11), 108. doi:10.1186/gm108
Lee, D., Heo, D. N., Kim, H. J., Ko, W. K., Lee, S. J., Heo, M., et al. (2016). Inhibition of osteoclast differentiation and bone resorption by bisphosphonate-conjugated gold nanoparticles. Sci. Rep. 6, 27336. doi:10.1038/srep27336
Lee, J. K., Kim, K. W., Choi, J. Y., Moon, S. Y., Kim, S. G., Kim, C. H., et al. (2013). Bisphosphonates-related osteonecrosis of the jaw in korea: A preliminary report. J. Korean Assoc. Oral Maxillofac. Surg. 39, 9–13. doi:10.5125/jkaoms.2013.39.1.9
Li, C. L., Lu, W. W., Seneviratne, C. J., Leung, W. K., Zwahlen, R. A., and Zheng, L. W. (2016). Role of periodontal disease in bisphosphonate-related osteonecrosis of the jaws in ovariectomized rats. Clin. Oral Implants Res. 27 (1), 1–6. doi:10.1111/clr.12502
Li, Y. J., Xu, M., Gao, Z. H., Wang, Y. Q., Yue, Z., Zhang, Y. X., et al. (2013). Alterations of serum levels of BDNF-related miRNAs in patients with depression. PLoS One 8, e63648. doi:10.1371/journal.pone.0063648
Liu, H., Wei, W., Wang, X., Guan, X., Chen, Q., Pu, Z., et al. (2018). miR-23b-3p promotes the apoptosis and inhibits the proliferation and invasion of osteosarcoma cells by targeting SIX1. Mol. Med. Rep. 18 (6), 5683–5692. doi:10.3892/mmr.2018.9611
Lopez-Jornet, P., Camacho-Alonso, F., Martínez-Canovas, A., Molina-Miñano, F., Gómez-García, F., and Vicente-Ortega, V. (2011). Perioperative antibiotic regimen in rats treated with pamidronate plus dexamethasone and subjected to dental extraction: A study of the changes in the jaws. J. Oral Maxillofac. Surg. 69, 2488–2493. doi:10.1016/j.joms.2011.02.059
Lorenzo-Pouso, A. I., Bravo, S. B., Carballo, J., Chantada-Vázquez, M. D. P., Bagán, J., Bagán, L., et al. (2022). Quantitative proteomics in medication-related osteonecrosis of the jaw: A proof-of-concept study. Oral Dis. 2022, 14201. Apr 4. Epub ahead of print. doi:10.1111/odi.14201
Lyles, K. W., Colón-Emeric, C. S., Magaziner, J. S., Adachi, J. D., Pieper, C. F., Mautalen, C., et al. (2007). Zoledronic acid and clinical fractures and mortality after hip fracture. N. Engl. J. Med. 357, 1799–1809. doi:10.1056/NEJMoa074941
Maes, O. C., An, J., Sarojini, H., Wu, H., and Wang, E. (2008). Changes in MicroRNA expression patterns in human fibroblasts after low-LET radiation. J. Cell Biochem. 103, 824–834. doi:10.1002/jcb.21878
Marx, R. E. (2003). Pamidronate (aredia) and zoledronate (zometa) induced avascular necrosis of the jaws: A growing epidemic. J. Oral Maxillofac. Surg. 61 (9), 1115–1117. doi:10.1016/s0278-2391(03)00720-1
Mawardi, H., Treister, N., Richardson, P., Anderson, K., Munshi, N., Faiella, R. A., et al. (2009). Sinus tracts-an early sign of bisphosphonate-associated osteonecrosis of the jaws? J. Oral Maxillofac. Surg. 67, 593–601. doi:10.1016/j.joms.2008.09.031
McGowan, K., McGowan, T., and Ivanovski, S. (2018). Risk factors for medication-related osteonecrosis of the jaws: A systematic review. Oral Dis. 24 (4), 527–536. doi:10.1111/odi.12708
McLeod, N. M., Brennan, P. A., and Ruggiero, S. L. (2012). Bisphosphonate osteonecrosis of the jaw: A historical and contemporary review. Surgeon 10 (1), 36–42. doi:10.1016/j.surge.2011.09.002
Musolino, C., Oteri, G., Allegra, A., Mania, M., D'Ascola, A., Avenoso, A., et al. (2018). Altered microRNA expression profile in the peripheral lymphoid compartment of multiple myeloma patients with bisphosphonate-induced osteonecrosis of the jaw. Ann. Hematol. 97 (7), 1259–1269. doi:10.1007/s00277-018-3296-7
Nguyen, N. T., Hanieh, H., Nakahama, T., and Kishimoto, T. (2013). The roles of aryl hydrocarbon receptor in immune responses. Int. Immunol. 25 (6), 335–343. doi:10.1093/intimm/dxt011
Ono, T., Okamoto, K., Nakashima, T., Nitta, T., Hori, S., Iwakura, Y., et al. (2016). IL-17-producing γδ T cells enhance bone regeneration. Nat. Commun. 7, 10928. doi:10.1038/ncomms10928
Orwoll, E., Teglbjærg, C. S., Langdahl, B. L., Chapurlat, R., Czerwinski, E., Kendler, D. L., et al. (2012). A randomized, placebo-controlled study of the effects of denosumab for the treatment of men with low bone mineral density. J. Clin. Endocrinol. Metab. 97, 3161–3169. doi:10.1210/jc.2012-1569
Oteri, G., Allegra, A., Bellomo, G., Alonci, A., Nastro, E., Penna, G., et al. (2008). Reduced serum levels of interleukin 17 in patients with osteonecrosis of the jaw and in multiple myeloma subjects after bisphosphonates administration. Cytokine 43 (2), 103–104. doi:10.1016/j.cyto.2008.05.010
Otto, S., Tröltzsch, M., Jambrovic, V., Panya, S., Probst, F., Ristow, O., et al. (2015). Tooth extraction in patients receiving oral or intravenous bisphosphonate administration: A trigger for BRONJ development? J. Craniomaxillofac. Surg. 43, 847–854. doi:10.1016/j.jcms.2015.03.039
Papapoulos, S., Chapurlat, R., Libanati, C., Brandi, M. L., Brown, J. P., Czerwiński, E., et al. (2012). Five years of denosumab exposure in women with postmenopausal osteoporosis: Results from the first two years of the FREEDOM extension. J. Bone Min. Res. 27, 694–701. doi:10.1002/jbmr.1479
Parikh, A., Lee, C., Joseph, P., Marchini, S., Baccarini, A., Kolev, V., et al. (2014). MicroRNA-181a has a critical role in ovarian cancer progression through the regulation of the epithelial-mesenchymal transition. Nat. Commun. 5, 2977. doi:10.1038/ncomms3977
Pazianas, M., Miller, P., Blumentals, W. A., Bernal, M., and Kothawala, P. (2007). A review of the literature on osteonecrosis of the jaw in patients with osteoporosis treated with oral bisphosphonates: Prevalence, risk factors, and clinical characteristics. Clin. Ther. 29 (8), 1548–1558. doi:10.1016/j.clinthera.2007.08.008
Poliseno, L., Tuccoli, A., Mariani, L., Evangelista, M., Citti, L., Woods, K., et al. (2006). MicroRNAs modulate the angiogenic properties of HUVECs. Blood 108, 3068–3071. doi:10.1182/blood-2006-01-012369
Qu, X., Wang, Z., Zhou, T., and Shan, L. (2020). Determination of the molecular mechanism by which macrophages and γδ-T cells contribute to ZOL-induced ONJ. Aging (Albany NY) 12, 20743–20752. doi:10.18632/aging.104006
Ramírez, L., López-Pintor, R. M., Casañas, E., Arriba, L., and Hernández, G. (2015). New non-bisphosphonate drugs that produce osteonecrosis of the jaws. Oral Health Prev. Dent. 13, 385–393. doi:10.3290/j.ohpd.a34055
Recchioni, R., Marcheselli, F., Olivieri, F., Ricci, S., Procopio, A. D., and Antonicelli, R. (2013). Conventional and novel diagnostic biomarkers of acute myocardial infarction: A promising role for circulating microRNAs. Biomarkers 18, 547–558. doi:10.3109/1354750X.2013.833294
Reid, G., Pel, M. E., Kirschner, M. B., Cheng, Y. Y., Mugridge, N., Weiss, J., et al. (2013). Restoring expression of miR-16: A novel approach to therapy for malignant pleural mesothelioma. Ann. Oncol. 24 (12), 3128–3135. doi:10.1093/annonc/mdt412
Reszka, A. A., and Rodan, G. A. (2003). Bisphosphonate mechanism of action. Curr. Rheumatol. Rep. 5, 65–74. doi:10.1007/s11926-003-0085-6
Ripamonti, C. I., Lucchesi, M., and Giusti, R. (2016). Prevention and management of osteonecrosis of the jaw secondary to bone-targeted therapy in patients with kidney cancer. Curr. Opin. Support Palliat. Care. 10, 273–280. doi:10.1097/SPC.0000000000000219
Ruggiero, S. L., Dodson, T. B., Aghaloo, T., Carlson, E. R., Ward, B. B., and Kademani, D. (2022). American Association of Oral and Maxillofacial Surgeons' position paper on medication-related osteonecrosis of the jaws-2022 update. J. Oral Maxillofac. Surg. 80 (5), 920–943. doi:10.1016/j.joms.2022.02.008
Ruggiero, S. L., Dodson, T. B., Fantasia, J., Goodday, R., Aghaloo, T., Mehrotra, B., et al. (2014). American Association of oral and maxillofacial surgeons position paper on medication-related osteonecrosis of the jaw—2014 update. J. Oral Maxillofac. Surg. 72, 1938–1956. doi:10.1016/j.joms.2014.04.031
Santini, D., Vincenzi, B., Dicuonzo, G., Avvisati, G., Massacesi, C., Battistoni, F., et al. (2003). Zoledronic acid induces significant and long-lasting modifications of circulating angiogenic factors in cancer patients. Clin. Cancer Res. 9, 2893–2897.
Scott, K. A., Hoban, A. E., Clarke, G., Moloney, G. M., Dinan, T. G., and Cryan, J. F. (2015). Thinking small: Towards microRNA-based therapeutics for anxiety disorders. Expert Opin. Investig. Drugs 24, 529–542. doi:10.1517/13543784.2014.997873
Seto, A. G., Beatty, X., Lynch, J. M., Hermreck, M., Tetzlaff, M., Duvic, M., et al. (2018). Cobomarsen, an oligonucleotide inhibitor of miR-155, co-ordinately regulates multiple survival pathways to reduce cellular proliferation and survival in cutaneous T-cell lymphoma. Br. J. Haematol. 183 (3), 428–444. doi:10.1111/bjh.15547
Sieber, P., Lardelli, P., Kraenzlin, C. A., Kraenzlin, M. E., and Meier, C. (2013). Intravenous bisphosphonates for postmenopausal osteoporosis: Safety profiles of zoledronic acid and ibandronate in clinical practice. Clin. Drug Investig. 33, 117–122. doi:10.1007/s40261-012-0041-1
Smidt-Hansen, T., Folkmar, T. B., Fode, K., Agerbaek, M., and Donskov, F. (2013). Combination of zoledronic Acid and targeted therapy is active but may induce osteonecrosis of the jaw in patients with metastatic renal cell carcinoma. J. Oral Maxillofac. Surg. 71, 1532–1540. doi:10.1016/j.joms.2013.03.019
Sotiropoulou, G., Pampalakis, G., Lianidou, E., and Mourelatos, Z. (2009). Emerging roles of microRNAs as molecular switches in the integrated circuit of the cancer cell. RNA 15 (8), 1443–1461. doi:10.1261/rna.1534709
Soutome, S., Hayashida, S., Funahara, M., Sakamoto, Y., Kojima, Y., Yanamoto, S., et al. (2018). Factors affecting development of medication-related osteonecrosis of the jaw in cancer patients receiving high-dose bisphosphonate or denosumab therapy: Is tooth extraction a risk factor? PLoS One 13 (7), e0201343. doi:10.1371/journal.pone.0201343
Sun, K., Wang, J., Liu, F., Ji, Z., Guo, Z., Zhang, C., et al. (2016). Ossotide promotes cell differentiation of human osteoblasts from osteogenesis imperfecta patients by up-regulating miR-145. Biomed. Pharmacother. 83, 1105–1110. doi:10.1016/j.biopha.2016.08.025
Surajkulwatana, T., Vacharaksa, A., and Srithanyarat, S. S. (2020). “MicroRNA profiles associated with bone healing of the extraction socket in medication-related osteonecrosis of the jaw in rat model: A pilot study,” in Proceedings of RSU International Research Conference, 1 May 2020, 201–211.
Tennis, P., Rothman, K. J., Bohn, R. L., Tan, H., Zavras, A., Laskarides, C., et al. (2012). Incidence of osteonecrosis of the jaw among users of bisphosphonates with selected cancers or osteoporosis. Pharmacoepidemiol. Drug Saf. 21, 810–817. doi:10.1002/pds.3292
Thumbigere-Math, V., Michalowicz, B. S., Hughes, P. J., Basi, D. L., Tsai, M. L., Swenson, K. K., et al. (2016). Serum markers of bone turnover and angiogenesis in patients with bisphosphonate-related osteonecrosis of the jaw after discontinuation of long-term intravenous bisphosphonate therapy. J. Oral Maxillofac. Surg. 74, 738–746. doi:10.1016/j.joms.2015.09.028
Turchinovich, A., Weiz, L., and Burwinkel, B. (2012). Extracellular miRNAs: The mystery of their origin and function. Trends biochem. Sci. 37 (11), 460–465. doi:10.1016/j.tibs.2012.08.003
Ulmner, M., Jarnbring, F., and Törring, O. (2014). Osteonecrosis of the jaw in Sweden associated with the oral use of bisphosphonate. J. Oral Maxillofac. Surg. 72, 76–82. doi:10.1016/j.joms.2013.06.221
Urade, M., Tanaka, N., Furusawa, K., Shimada, J., Shibata, T., Kirita, T., et al. (2011). Nationwide survey for bisphosphonate-related osteonecrosis of the jaws in Japan. J. Oral Maxillofac. Surg. 69, e364–e371. doi:10.1016/j.joms.2011.03.051
Vallina, C., Ramirez, L., and Torres, J. (2019). Osteonecrosis of the jaws produced by sunitinib: A systematic review. Med. Oral Patol. Oral Cir. Bucal 24, 1789. doi:10.4317/medoral.22858
Walsh, M. C., and Choi, Y. (2014). Biology of the RANKL-RANK-OPG System in Immunity, Bone, and Beyond. Front Immunol. 5, 511. doi:10.3389/fimmu.2014.00511
Wang, J., Tan, L., Tan, L., Tian, Y., Ma, J., Tan, C. C., et al. (2015). Circulating microRNAs are promising novel biomarkers for drug-resistant epilepsy. Sci. Rep. 5, 10201. doi:10.1038/srep10201
Wang, R., Li, N., Zhang, Y., Ran, Y., and Pu, J. (2011). Circulating microRNAs are promising novel biomarkers of acute myocardial infarction. Intern. Med. 50, 1789–1795. doi:10.2169/internalmedicine.50.5129
Wehrhan, F., Hyckel, P., GuentschStockmann, A. P., Schlegel, K. A., and Neukam, F. W. (2011). Bisphosphonate-associated osteonecrosis of the jaw is linked to suppressed tgfβ1-signaling and increased galectin-3 expression: A histological study on biopsies. J. Transl. Med. 9, 102. doi:10.1186/1479-5876-9-102
Wei, L. Y., Kok, S. H., Lee, Y. C., Chiu, W. Y., Wang, J. J., Cheng, S. J., et al. (2021). Prognosis of medication-related osteonecrosis of the jaws in cancer patients using antiresorptive agent zoledronic acid. J. Formos. Med. Assoc. 120 (8), 1572–1580. doi:10.1016/j.jfma.2020.11.017
Weir, D. W., Sturrock, A., and Leavitt, B. R. (2011). Development of biomarkers for Huntington’s disease. Lancet Neurol. 10, 573–590. doi:10.1016/S1474-4422(11)70070-9
Weinstein, R. S., Roberson, P. K., and Manolagas, S. C. (2009). Giant osteoclast formation and long-term oral bisphosphonate therapy. N Engl J Med. 360(1), 53–62. doi:10.1056/NEJMoa0802633
Yamazaki, T., Yamori, M., Ishizaki, T., Asai, K., Goto, K., Takahashi, K., et al. (2012). Increased incidence of osteonecrosis of the jaw after tooth extraction in patients treated with bisphosphonates: A cohort study. Int. J. Oral Maxillofac. Surg. 41 (11), 1397–1403. doi:10.1016/j.ijom.2012.06.020
Yang, R., Tao, Y., Wang, C., Shuai, Y., and Jin, L. (2018). Circulating microRNA panel as a novel biomarker to diagnose bisphosphonate-related osteonecrosis of the jaw. Int. J. Med. Sci. 15 (14), 1694–1701. doi:10.7150/ijms.27593
Yu, F. Y., Xie, C. Q., Sun, J. T., Peng, W., and Huang, X. W. (2018). Overexpressed miR-145 inhibits osteoclastogenesis in RANKL-induced bone marrow-derived macrophages and ovariectomized mice by regulation of Smad3. Life Sci. 202, 11–20. doi:10.1016/j.lfs.2018.03.042
Zalavras, C. G., and Lieberman, J. R. (2014). Osteonecrosis of the femoral head: Evaluation and treatment. J. Am. Acad. Orthop. Surg. 22 (7), 455–464. doi:10.5435/JAAOS-22-07-455
Zeng, H. C., Bae, Y., Dawson, B. C., Chen, Y., Bertin, T., Munivez, E., et al. (2017). MicroRNA miR-23a cluster promotes osteocyte differentiation by regulating TGF-β signalling in osteoblasts. Nat. Commun. 8, 15000. doi:10.1038/ncomms15000
Zhang, W., Gao, L., Ren, W., Li, S., Zheng, J., Li, S., et al. (2021). The role of the immune response in the development of medication-related osteonecrosis of the jaw. Front. Immunol. 12, 606043. doi:10.3389/fimmu.2021.606043
Keywords: microRNA, osteoclast, diagnosis, therapeutics, medication related osteonecrosis of the jaw (MRONJ)
Citation: Mohd Yunus SS, Soh HY, Abdul Rahman M, Peng X, Guo C and Ramli R (2023) MicroRNA in medication related osteonecrosis of the jaw: a review. Front. Physiol. 14:1021429. doi: 10.3389/fphys.2023.1021429
Received: 17 August 2022; Accepted: 07 April 2023;
Published: 26 April 2023.
Edited by:
Vittorio Fusco, Azienda Ospedaliera Nazionale SS. Antonio e Biagio e Cesare Arrigo, ItalyReviewed by:
Massimo Del Fabbro, University of Milan, ItalyAmerigo Giudice, University Magna Graecia of Catanzaro, Italy
Copyright © 2023 Mohd Yunus, Soh, Abdul Rahman, Peng, Guo and Ramli. This is an open-access article distributed under the terms of the Creative Commons Attribution License (CC BY). The use, distribution or reproduction in other forums is permitted, provided the original author(s) and the copyright owner(s) are credited and that the original publication in this journal is cited, in accordance with accepted academic practice. No use, distribution or reproduction is permitted which does not comply with these terms.
*Correspondence: Roszalina Ramli, roszalina@ppukm.ukm.edu.my