- 1Rehabilitation Medicine Center, West China Hospital, Sichuan University, Chengdu, China
- 2Key Laboratory of Rehabilitation Medicine in Sichuan Province, West China Hospital, Sichuan University, Chengdu, China
- 3School of Rehabilitation Sciences, West China School of Medicine, Sichuan University, Chengdu, China
- 4Institute of Cardiovascular & Medical Sciences, University of Glasgow, Glasgow, United Kingdom
Acute high-altitude diseases, including acute mountain sickness (AMS), high-altitude cerebral edema (HACE), and high-altitude pulmonary edema (HAPE), have been recognized as potentially lethal diseases for altitude climbers. Various preconditioning stimuli, including hyperbaric oxygen (HBO), have been proposed to prevent acute high-altitude diseases. Herein, we reviewed whether and how HBO preconditioning could affect high-altitude diseases and summarized the results of current trials. Evidence suggests that HBO preconditioning may be a safe and effective preventive method for acute high-altitude diseases. The proposed mechanisms of HBO preconditioning in preventing high-altitude diseases may involve: 1) protection of the blood-brain barrier and prevention of brain edema, 2) inhibition of the inflammatory responses, 3) induction of the hypoxia-inducible factor and its target genes, and 4) increase in antioxidant activity. However, the optimal protocol of HBO preconditioning needs further exploration. Translating the beneficial effects of HBO preconditioning into current practice requires the “conditioning strategies” approach. More large-scale and high-quality randomized controlled studies are needed in the future.
1 Introduction
With the rapid development in transportation from low to high altitudes and the growing interest in adventure travel, more than 100 million people now travel to high altitudes for work or pleasure annually. The decreased barometric pressure and ambient oxygen tension at high altitudes can trigger a range of physiological responses, leading to maladaptive reactions and acute altitude illness (Luks, 2015), including acute mountain sickness (AMS), high-altitude cerebral edema (HACE), and high-altitude pulmonary edema (HAPE) (Clark and Sheraton, 2021). AMS is by far the most common high-altitude disease, with a reported incidence of more than 25% and 50% in people ascending to 3,500 and above 6,000 m, respectively (Meier et al., 2017). HACE rarely occurs at altitudes lower than 4,000 m, with an incidence of 0.5%–1% between 4,200 and 5,500 m. The incidence of HAPE is less than 0.2% if individuals spend three days climbing to an altitude of 4,000–5,000 m, but can be up to 7% in a single-day climb (Luks et al., 2017). Moreover, 85%–100% of individuals diagnosed with HACE also develop HAPE (Turner et al., 2021).
Hyperbaric oxygen therapy (HBOT) refers to the inhalation of 100% oxygen at a pressure higher than normal atmospheric pressure (Jain, 2016). A growing body of studies demonstrated that HBO preconditioning might prevent high-altitude diseases. Therefore, this review aimed to address whether and how hyperbaric oxygen (HBO) preconditioning may affect acute high-altitude diseases, and to summarize the results of current trials.
2 High-altitude diseases
2.1 Clinical manifestation and diagnosis
According to the 2018 Lake Louise AMS Score (Roach et al., 2018), AMS was defined as the presence of headache in an unacclimatized person who had recently arrived at an altitude above 2,500 m, along with the presence of one or more of the following: gastrointestinal symptoms (anorexia, nausea, or vomiting), fatigue or weakness, dizziness or light-headedness. HACE, which usually occurs between 24 and 72 h at altitudes above 4,000 m, is characterized by neurological findings such as ataxia, decreased consciousness, and slurred speech (Jensen and Vincent, 2022). The classic evaluation of HACE includes an abnormal neurological examination, in which ataxia is usually the earliest finding. Laboratory tests may reveal an increased white blood cell count and lumbar puncture may show open pressure elevation. Magnetic resonance imaging may demonstrate a distinct swelling and hypertonicity of the posterior spleen and of the corpus callosum on T2 images (Hackett and Roach, 2004).
HAPE may occur within one to five days after reaching an altitude above 2,500 to 3,000 m, with the symptoms of excessive exertional dyspnea, cough, chest tightness, and decreased exercise performance (Luks et al., 2017). It would also have any two of the following findings: tachycardia, central cyanosis, rales/hiss, and shortness of breath. If detectable, a chest x-ray (CXR) may demonstrate patchy alveolar infiltration with normal mediastinal/cardiac size, and an ultrasound may demonstrate B-lines indicating pulmonary edema. Electrocardiogram might show right axis deviation and/or signs of ischemia. Rapid correction of clinical status and percutaneous arterial oxygen saturation with supplemental oxygen is a pathognomonic feature of HAPE in patients with infiltrates on CXR films. Even with laboratory tests, their role is limited and clinicians should always take into account the coexistence of AMS and/or HACE (Jensen and Vincent, 2022).
2.2 Pathophysiological processes
The hypobaric hypoxic environment at high altitudes has a great impact on human physiological functions and physical activities (Agrawal et al., 2017). Hyperventilation, polycythemia, hypoxic pulmonary vasoconstriction, alterations in the oxygen affinity of hemoglobin, a rise in oxidative enzymes, and an increased concentration of capillaries in peripheral muscle are classical responses of the body to high altitude. For AMS patients, arterial oxygen saturation (SaO2) and tension (PaO2) are a bit lower and the alveolar-arterial oxygen tension is a bit greater at high altitudes compared to healthy controls (Luks et al., 2017).
The pathogenesis of AMS and HACE remains controversial, but some evidence suggests that it is induced by elevated intracranial pressure (Dipasquale et al., 2016). Some experts believe that increased cerebral blood flow raises central nervous system pressure. Low arterial PaO2 leads to cerebral vasodilation, despite the opposite effect of reduced arterial partial pressure of carbon dioxide that accompanies hyperventilation. Other causes such as increased microvascular permeability and abnormal sodium and water balance may also cause AMS (West, 2012). Matrix metalloproteinase-9 (MMP-9) causes blood-brain barrier (BBB) destruction, brain edema, and neuroinflammation (Pan et al., 2020).
HAPE occurs as a response of the vasculature of the lungs to hypoxia. At high altitude, the body reacts to hypoxia by hyperventilating, which is known as the hypoxic ventilatory response. In hypoxic conditions, the reduced supply of nitric oxide (NO) and prostaglandin E2 (Bian et al., 2022) can cause excessive rise in pulmonary artery pressure (PAP). Increased PAP prior to the development of edema is the key pathophysiological factor of HAPE (Mulchrone et al., 2020).
3 Measures to improve high-altitude endurance
A slow ascent is the primary strategy for preventing altitude diseases (Luks et al., 2017). Furthermore, pharmaceutical agents (Ritchie et al., 2012) such as acetazolamide, calcium-channel blockers, and phosphodiesterase 5 inhibitors contribute to the prevention and treatment of acute high-altitude diseases. The Wilderness Medical Society has published guidelines on the use of hyperbaric chambers for treating severe AMS and HACE (Luks et al., 2019), but symptoms can recur when the patient leaves the chamber on the highlands. Therefore, the question remains whether it would be better to use HBO preconditioning to improve individual high-altitude endurance and prevent AMS.
4 HBO preconditioning for high-altitude diseases
Numerous studies have explored the effectiveness of HBO preconditioning for high-altitude diseases (study characteristics are shown in Table 1).
4.1 HBO preconditioning for human responses to high-altitude and AMS
HBO preconditioning may alleviate the body’s hypoxic response at high altitudes, improve cognitive and physical function, and prevent AMS.
First, HBO preconditioning could improve the SaO2 (Wang et al., 2009; Yang et al., 2014) and PaO2 level, reduce heart rate and pulse rates, alleviate AMS symptoms in humans, and the effects could last for a week (Ma G. Q. W. et al., 2008). Moreover, under hyperbaric conditions, one can dissolve enough oxygen, that is 6 vol% in the plasma, to satisfy the typical physical needs (Jain, 2016). HBO preconditioning increases plasma expression of dopamine, epinephrine, and adrenocorticotropic hormone, and then accelerates the establishment of a new homeostasis that promotes the acclimatization of the organism in a high-altitude environment (Li et al., 2015). Pan et al. (2008) compared the preconditioning effects between hypoxia preconditioning and HBO preconditioning, and observed that both methods increased endothelin and promoted altitude acclimatization.
Second, HBO preconditioning could improve individuals’ cognitive and physical performance at high altitudes. Studies (Ma, 2007; Wang et al., 2009) found that people receiving HBO preconditioning twice had better memory and responsiveness ability compared to control group. HBO preconditioning could also reduce lactic acid levels in the body, help in reducing fatigue and refresh the workers. Shi et al. (Shi Lu et al., 2015) observed that three to seven sessions of HBO preconditioning increased glucose levels after physical loading and reduced blood lactate (BLA), lactate dehydrogenase and Na + -K + -ATPase levels at the same time. Moreover, three-day HBO preconditioning showed the best efficacy. It turns out that short-term HBO preconditioning can significantly improve energy metabolism in individuals under physical load at high altitude, accelerate glycogenolysis, and lead to better utilization of oxygen by muscles, thus alleviating fatigue and enhancing work efficiency.
Regarding body organs, the photoreceptor system of the eye is the most sensitive to hypoxia. Previous studies showed that HBO preconditioning could improve the health of the retina, dark adaptation, and orienting attention (Ma G. Q. W. et al., 2008; Shi Lu et al., 2015). There is a functional decline in coordinated movement when cerebral hypoxia occurs, and HBO preconditioning can enhance body coordination. A study of Liang et al. (2007) showed that HBO preconditioning can improve the body’s blood oxygen dispersion, enhance aerobic metabolism, and generate more ATP. Therefore, it can enhance the body’s ability to tolerate hypoxia and reduce body damage. Two HBO pretreatments reversed tissue hypoxia and improved post-exercise haemodynamic indexes (Cui et al., 2007a), and this effect lasted for approximately a week. Xiao and Hu. (2017) found that the incidence of AMS was lower after five HBO pre-treatments compared to the control group (30% vs. 90%). Liu et al. (2011) found that three HBO pretreatments reduced the levels of interleukin 6 (IL-6) and MMP-9 in mice with AMS, but the difference was not significant. Therefore, further studies are needed to confirm the prophylactic effect of HBO preconditioning.
4.2 HBO preconditioning for HACE and HAPE
Studies on the prevention of HACE and HAPE with hyperbaric oxygen preconditioning are mainly animal studies.
Previous studies found that heat shock protein (HSP) 70-mediated HBO preconditioning could prevent high-altitude brain edema, cognitive deficits, and hippocampal oxidative stress in rats (Lin et al., 2012a; Lin et al., 2012b). Zhou et al. (2017) found that HBO preconditioning significantly alleviated the increase of BBB permeability and water content in rats caused by high-altitude hypoxia, and had a preventive effect on HACE.
As for the preventive effects of HBO preconditioning on HAPE, the mechanisms may involve HSP70 and aquaporin (AQP) (Tsai et al., 2014; Lin et al., 2015). AQP1 and AQP5 provide the main pathway for water transport through the pulmonary microvascular endothelium and are involved in the pathogenesis of pulmonary edema. Studies showed that HBO preconditioning alleviated the upregulation of AQP1 and AQP5 in the lung, thus preventing HAPE (Li et al., 2010; Li et al., 2011a; Li et al., 2011b). Cui et al. (2008) reported that HBO preconditioning significantly increased heart rate, PAP, right ventricular systolic pressure, right ventricular end-diastolic pressure, and the maximum rate of rise/decline of right ventricular pressure in rats at high altitude. In another study by Cui et al. (2014), water contents in the tissues of the rats in the HBO preconditioning groups were all decreased markedly than those in the control groups. In addition, the morphology of lungs and heart were more intact in HBO preconditioning group. These studies may suggest that HBO preconditioning can improve hemodynamics and protect cardiopulmonary function in acute hypoxic rats.
4.3 The proposed mechanisms of HBO preconditioning in preventing high-altitude diseases
Based on the existing evidence, we propose some potential mechanisms for HBO preconditioning in the prevention of high-altitude disease (Figure 1).
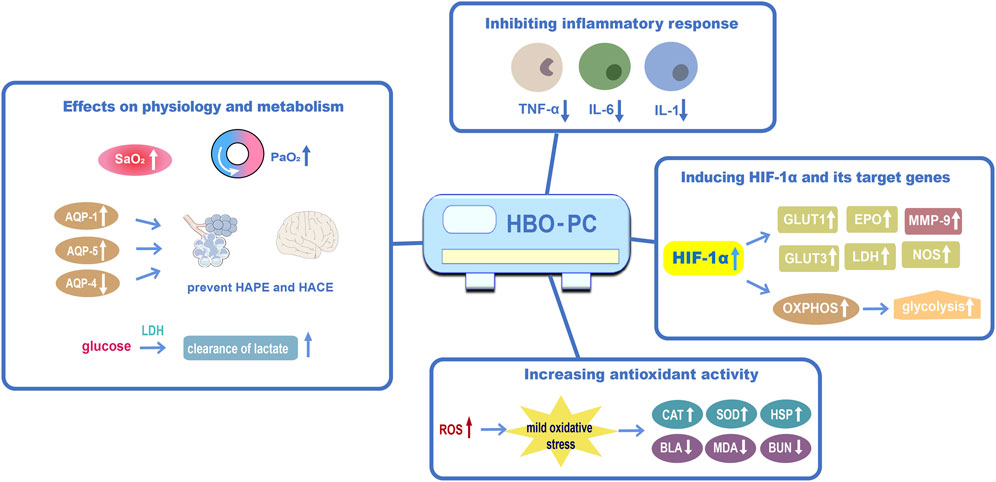
FIGURE 1. The proposed mechanisms of HBO preconditioning in preventing high-altitude disease (1) Effects of HBO preconditioning on physiology and metabolism against acute high-altitude diseases; (2) HBO preconditioning may inhibit inflammatory responsiveness by reducing apoptosis and shifting mitochondria; (3) HBO preconditioning may induce HIF-1α and its target genes; (4) HBO preconditioning could increase antioxidant activity. Abbreviations: HBO-PC, hyperbaric oxygen preconditioning; PaO2, partial pressure of oxygen; SaO2, oxygen saturation; AQP-1, aquaporin 1; AQP-5, aquaporin 5; AQP-4, aquaporin 4; IL-6, interleukin 6; HIF-1α, hypoxia-inducible factor-1α; GLUT1, glucose transporter 1; EPO, erythropoietin; MMP-9, matrix metalloproteinase-9; GLUT3, glucose transporter 3; NOS, Nitric oxide synthase; OXPHOS, oxidative phosphorylation; ROS, reactive oxygen species; CAT, catalase; HSP, heat-shock protein; BLA, blood lactic acid; MDA, malondialdehyde; BUN, blood urea nitrogen.
4.3.1 Inhibiting inflammatory responses
HBO preconditioning may play an important role in inhibiting inflammatory responses to prevent high-altitude diseases. Qi et al. (2013) reported that HBO preconditioning reduced inflammatory factors such as interleukin 1, tumor necrosis factor-α, and IL-6 in rats. HBO provides oxygen and thus promotes NO synthesis. Cui et al. (2007c) found that HBO preconditioning could boost blood circulation, increase NO concentrations, and reduce hypoxic tissue injury in the human body at high altitudes, and this effect can last for more than eight days.
4.3.2 Generating HIF-1α
HIF-1 is a crucial modulator for inducing genes that promote cellular adaptation and survival under hypoxic conditions (Semenza, 1998). Some pharmacotherapies have been proven to prevent AMS by regulating the HIF-1 signaling pathway (Wang et al., 2019; Yan et al., 2021). Recent studies have shown that HBO can increase the expression of HIF-1α by stabilizing and activating it (Salhanick et al., 2006; Duan et al., 2015; Salmón-González et al., 2021).
Theoretically, hyperoxia should reduce HIF, then this raises a question regarding how HBO increases HIF. The specific mechanisms remain unclear, but the following hypotheses have been discussed. First, the brain tissue undergoes relative hypoxia following HBO preconditioning, as the oxygen level is reduced to 21% of normal levels after the 100% O2 level in the HBO chamber. Hence, repeated HBO preconditioning may generate a hyperoxia and hypoxia cycle and induce an accumulation of HIF-1α (Fratantonio et al., 2021). Second, in addition to hypoxia, other stimuli such as cytokines, growth factors, hormones, and viral proteins can induce HIF-1 (Gu et al., 2008). Third, a possible mechanism is the production of ROS by HBO preconditioning. The stabilization of HIF-1α is related to increased ROS (Guzy et al., 2005). Intermittent hyperoxia produces a state that mimics hypoxia through a reduced ROS/clearance capacity ratio (Hadanny and Efrati, 2020). These findings suggest that HBO preconditioning may increase HIF in a delayed manner to promote altitude acclimation.
4.3.3 Increasing antioxidant activity
Acute hypobaric hypoxia increased the malondialdehyde (MDA) level (Agrawal et al., 2017). Previous studies found that HBO preconditioning could reduce MDA, blood urea nitrogen levels, and BLA in high altitudes. In addition, HBO preconditioning could increase superoxide dismutase, thus we can infer that HBO preconditioning could increase the activity of antioxidants and degrade serum lactate levels, and has a preventive effect on AMS (Cui et al., 2007b; Gao et al., 2008).
4.4 The protocol of preconditioning for preventing acute high-altitude diseases
The common HBO preconditioning pressure in these included studies was two ATA. The number of HBO preconditioning sessions ranged from one to seven, while two to five sessions were the common choices. Almost every study chose to do the HBO preconditioning once a day. The neuroprotective effect induced by HBO preconditioning is short-term and usually disappears in approximately two weeks (Qi et al., 2020). Therefore, climbers should preferably be exposed to HBO preconditioning within two weeks before climbing. The optimal regimen of HBO preconditioning for preventing high-altitude diseases still needs further exploration.
4.5 Safety of HBO preconditioning
HBOT is used in an increasing number of conditions and is seen to be a generally safe treatment. The possible side effects of HBO involve barotrauma, oxygen poisoning, visual abnormalities, and claustrophobia (Jain, 2016). Middle ear barotrauma, which has been reported in up to 2% of treated individuals, is by far the most common and benign side effect. It can be avoided or decreased by teaching autoinflation skills or by inserting tympanostomy tubes. Claustrophobia is another common symptom that calls for assurance, counseling, and even medication. Progressive myopia is typically temporary and reversible after stopping HBO sessions. Pulmonary dyspnea with cough and inspiratory pain are caused by oxygen toxicity, from the multiple exposures required for chronic treatments. Rarely, at greater oxygen pressures, during acute therapies in acidotic patients do more severe seizures occur. A retrospective analysis found that rigorous operational procedures (chamber monitoring and assessment prior to HBO treatment) were essential to increase the safety of patients. When used properly, HBOT could be one of the safest medical techniques (Hadanny et al., 2016).
5 Discussion
To translate HBO preconditioning paradigm to the clinic, it is critical to identify vulnerable groups at high risk for acute high-altitude diseases. Previous studies have shown that AMS history, level of residence, or recent high-altitude exposure and ascending rate are significant predictors of high-altitude diseases (Erik and Swenson, 2014). Obesity (Yang et al., 2015), age, and gender may be associated with the incidence of high-altitude disease (Maloney and Broeckel, 2005). However, exact risk factors are yet to be identified.
For climbers, it is convenient to take HBO preconditioning. Hyperbaric chambers can be categorized into monoplace chambers and multiplace chambers. The monoplace chamber can only accommodate one person, and the multiplace chamber allows for the treatment of several patients at the same time (Lind, 2015). There are also portable chambers (Butler et al., 2011). Those portable chambers are for single use and can fit into a backpack when deflated, which are carried on most high-altitude expeditions. The drawback is that they are very limited in the pressure, and the highest common pressure that can be achieved is about 1.4 ATA. The portable chamber is mainly used in the treatment of high-altitude diseases. As it is an U.S. Food and Drug Administration-approved indication, HBOT can be implemented quickly once the treatment has been optimized for humans (Lippert and Borlongan, 2019).
To date, HBOT has been widely used in the treatment of high-altitude disease; however, the preventive role of HBOT pretreatment has been largely overlooked. To the best of our knowledge, this is the first study to review the effect of HBO preconditioning in preventing acute high-altitude diseases. Both animal studies and clinical studies showed that HBO preconditioning may be an effective modality to prevent high-altitude diseases. The proposed mechanism of HBO preconditioning in preventing high-altitude diseases may involve: 1) the protection of the BBB and prevention of brain edema, 2) the inhibition of inflammatory responses, 3) the induction of HIF-1α and its target genes, and 4) the increase in antioxidant activity. Based on the current results, multi-sessions of hyperbaric oxygen preconditioning may have a better preventive effect on acute altitude disease compared to a single session. The combined effect of pre- and post-climbing HBO use also warrants further study. With further confirmed role and the development of an optimal protocol of hyperbaric oxygen preconditioning, it will be beneficial to lower the morbidity of altitude diseases, and reduce the life, health and economic loss.
Data availability statement
The original contributions presented in the study are included in the article/supplementary material, further inquiries can be directed to the corresponding author.
Author contributions
JY: Writing–Original draft (lead). CH: Conceptualization (lead); writing–review and editing (equal). XC: Writing–review and editing (equal). MZ: Writing–original draft (supporting); writing–review and editing (equal). HM: Conceptualization (supporting); writing–original draft (supporting). QL: Revising the manuscript. All authors have approved the final manuscript.
Funding
This work was supported by 1·3·5 project for disciplines of excellence–Clinical Research Incubation Project, West China Hospital, Sichuan University [Grant No:2021HXFH063].
Conflict of interest
The authors declare that the research was conducted in the absence of any commercial or financial relationships that could be construed as a potential conflict of interest.
Publisher’s note
All claims expressed in this article are solely those of the authors and do not necessarily represent those of their affiliated organizations, or those of the publisher, the editors and the reviewers. Any product that may be evaluated in this article, or claim that may be made by its manufacturer, is not guaranteed or endorsed by the publisher.
References
Agrawal A., Rathor R., Suryakumar G. (2017). Oxidative protein modification alters proteostasis under acute hypobaric hypoxia in skeletal muscles: A comprehensive in vivo study. Cell Stress Chaperones 22, 429–443. doi:10.1007/s12192-017-0795-8
Bian S. Z., Zhang C., Rao R. S., Ding X. H., Huang L. (2022). Systemic blood predictors of elevated pulmonary artery pressure assessed by non-invasive echocardiography after acute exposure to high altitude: A prospective cohort study. Front. Cardiovasc Med. 9, 866093. doi:10.3389/fcvm.2022.866093
Bu X., Yang X., Zhang D., Ma H. (2021). Effect of single hyperbaric oxygen treatment on attention networks in young migrants in Tibet. Acta Physiol. Sin. 73, 286–294. doi:10.13294/j.aps.2021.0016
Butler G. J., Al-Waili N., Passano D. V., Ramos J., Chavarri J., Beale J., et al. (2011). Altitude mountain sickness among tourist populations: A review and pathophysiology supporting management with hyperbaric oxygen. J. Med. Eng. Technol. 35, 197–207. doi:10.3109/03091902.2010.497890
Clark S. T., Sheraton M. (2021). EMS high-altitude field prophylaxis and treatment. Treasure Island (FL): StatPearls Publishing.
Cui J., Gao L., Bai X., Zhang X., Ha Z., Li B., et al. (2009). Effects of hyperbaric oxygen preconditioning on blood gas and level of blood lactic acid at high altitude. J. High Alt. Med. 19, 8–9. doi:10.3969/j.issn.1007-3809.2009.01.004
Cui J., Gao L., Jin X., Zhang X., Li B., Ha Z., et al. (2007a). Effects of hyperbaric oxygen on hemorheology in healthy adults acute exposed high altitudes. Chin. J. Hemorheol. 17, 421–424. doi:10.3969/j.issn.1009-881X.2007.03.028
Cui J., Gao L., Zhang X., Jin X., Li B., Ha Z., et al. (2007b). Effects of hyperbaric oxygen preconditioning on free radical and serum lactic acid in young men at high altitudes. Chin. J. Naut. Med. Hyperbaric Med. 14, 278. doi:10.3760/cma.j.issn.1009-6906.2007.05.006
Cui J., Gao L., Zhang X., Jin X., Li B., Ha Z., et al. (2007c). Effects of hyperbaric oxygen preconditioning on NO and NOS at high altitude. J. High Alt. Med. 17, 4–7. doi:10.3969/j.issn.1007-3809.2007.02.004
Cui J. H., Li B., Zhang X. Z., Gao Y. Q., Liu F. Y., Gao W. X. (2008). Effects of hyperbaric oxygen preconditioning on cardiac muscle and heart function in acute hypoxia rats. Chin. J. Naut. Med. Hyperbar. Med. 15, 276–278. doi:10.3760/cma.j.issn.1009-6906.2008.05.006
Cui J. H., Wu P. F., Gao L., Li N. H., Wang Y., Li B., et al. (2014). Effects of hyperbraic oxygen pretreatment on the ultrastructure in acute hypoxia rats. Chin. J. Naut. Med. Hyperbar. Med. 21, 377–380. doi:10.3760/cma.j.issn.1009-6906.2014.06.005
Dipasquale D. M., Muza S. R., Gunn A. M., Li Z., Zhang Q., Harris N. S., et al. (2016). Evidence for cerebral edema, cerebral perfusion, and intracranial pressure elevations in acute mountain sickness. Brain Behav. 6, e00437. doi:10.1002/brb3.437
Duan S., Shao G., Yu L., Ren C. (2015). Angiogenesis contributes to the neuroprotection induced by hyperbaric oxygen preconditioning against focal cerebral ischemia in rats. Int. J. Neurosci. 125, 625–634. doi:10.3109/00207454.2014.956101
Fratantonio D., Virgili F., Zucchi A., Lambrechts K., Latronico T., Lafère P., et al. (2021). Increasing oxygen partial pressures induce a distinct transcriptional response in human pbmc: A pilot study on the "normobaric oxygen paradox. Int. J. Mol. Sci. 22, 458. doi:10.3390/ijms22010458
Feng E., Dai S., Shi L., Li Y., Zhang Y., He W., et al. (2017). Changes of myocardial enzyme and cardiac function after load exercise in soldiers immigrated from plain to plateau and intervention effects of hyperbaric oxygen pretreatment. J. Med. Res. 46, 69–71. doi:10.11969/j.issn.1673-548X.2017.09.019
Gao L., Zhan Y., Zhou W. Q., Cui J. H., Zhang X. Z., Jin X. H., et al. (2008). Effects of hyperbaric oxygen pretreatment on level of MDA and SOD in hgh altitude immigration. J. High Alt. Med. 18, 1–4. doi:10.3969/j.issn.1007-3809.2008.03.001
Gu G. J., Li Y. P., Peng Z. Y., Xu J. J., Kang Z. M., Xu W. G., et al. (2008). Mechanism of ischemic tolerance induced by hyperbaric oxygen preconditioning involves upregulation of hypoxia-inducible factor-1alpha and erythropoietin in rats. J. Appl. Physiol. 104, 1185–1191. doi:10.1152/japplphysiol.00323.2007
Guzy R. D., Hoyos B., Robin E., Chen H., Liu L., Mansfield K. D., et al. (2005). Mitochondrial complex III is required for hypoxia-induced ROS production and cellular oxygen sensing. Cell Metab. 1, 401–408. doi:10.1016/j.cmet.2005.05.001
Hackett P. H., Roach R. C. (2004). High altitude cerebral edema. High. Alt. Med. Biol. 5, 136–146. doi:10.1089/1527029041352054
Hadanny A., Efrati S. (2020). The hyperoxic-hypoxic paradox. Biomolecules 10, 958. doi:10.3390/biom10060958
Hadanny A., Meir O., Bechor Y., Fishlev G., Bergan J., Efrati S. (2016). The safety of hyperbaric oxygen treatment-retrospective analysis in 2,334 patients. Undersea Hyperb. Med. 43, 113–122.
Hu S., Jin Q., Liu Z., Zhu G., Lin J., Wang X., et al. (2006). Effects of hyperbaric oxygen preconditioning on traumatic brain injury at simulated high altitude in rats. Mil. Med. Sci. 30, 541–543+561. doi:10.3969/j.issn.1674-9960.2006.06.011
Jensen J. D., Vincent A. L. (2022). High altitude cerebral edema. Treasure Island (FL): StatPearls Publishing. StatPearls.
Li Y., Shi L., Wu N., Liu J., Zhang Y., Zhang M., et al. (2015). Effects of hyperbaric oxygen preconditioning on human stress responses during acute exposure to high altitude. Zhonghua Lao Dong Wei Sheng Zhi Ye Bing Za Zhi 33, 731–734. doi:10.3760/cma.j.issn.1001-9391.2015.10.004
Li Z., Gao C., Wang Y., Liu F., Ma L., Deng C., et al. (2011a). Reducing pulmonary injury by hyperbaric oxygen preconditioning during simulated high altitude exposure in rats. J. Trauma 71, 673–679. doi:10.1097/TA.0b013e3181f5b073
Li Z., Ge H., Wu L. H., Ma L. L., Lv C. F., Gao H. X., et al. (2010). Effect of hyperbaric oxygen preconditioning on the expression of lung aquaporin-1 in the rat model of high altitude pulmonary edema. Chin J Naut Med Hyperbar Med 17, 280–283. doi:10.3760/cma.j.issn.1009-6906.2010.05.008
Li Z., Zhao L. M., Wang C., Liu X. H., Lv C. F., Gao C. J. (2011b). Effect of hyperbaric oxygen preconditioning on expression of lung aquaporin-5 in rat models of high altitude pulmonary edema. Med. J. Chin. PAPF 22, 938–942. doi:10.3969/j.issn.1004-3594.2011.11.007
Liang F., Gao C., Wang G. (2007). The effect of hyperbaric oxygen for re-treatment on level of interleukin-6 and neutrophil elastase in high altitude hypoxia rats. Med. J. Liaoning 21, 155–159. doi:10.3969/j.issn.1001-1722.2007.03.001
Lin H., Chang C. P., Lin H. J., Lin M. T., Tsai C. C. (2012a). Attenuating brain edema, hippocampal oxidative stress, and cognitive dysfunction in rats using hyperbaric oxygen preconditioning during simulated high-altitude exposure. J. Trauma Acute Care Surg. 72, 1220–1227. doi:10.1097/TA.0b013e318246ee70
Lin H. J., Chang C. P., Tsai M. C. (2015). Hyperbaric oxygen preconditioning attenuated high-altitude pulmonary edema via heat shock protein 70 overexpression. Faseb J. 29. doi:10.1096/fasebj.29.1_supplement.680.3
Lin M. T., Chio C. C., Chang C. P., Niu K. C., Chang C. K., Wang C. C. (2012b). Attenuating brain edema, hippocampal oxidative stress, and cognitive dysfunction in high altitude exposure by hyperbaric oxygen preconditioning. Brain Inj. 26, 488.
Lind F. (2015). A pro/con review comparing the use of mono- and multiplace hyperbaric chambers for critical care. Diving Hyperb. Med. 45, 56–60.
Lippert T., Borlongan C. V. (2019). Prophylactic treatment of hyperbaric oxygen treatment mitigates inflammatory response via mitochondria transfer. CNS Neurosci. Ther. 25, 815–823. doi:10.1111/cns.13124
Liu J., Wang Y., Wu L., Gao C. (2011). Influences of hyperbaric oxygen pretreatment on mice hypoxia tolerance ability. J. Cap. Med. Univ. 32, 670–673. doi:10.3969/j.issn.1006-7795.2011.05.018
Liu J., Yu P., Gao C., Liu F. (2007). “Effects of hyperbaric oxygen preconditioning on leukocyte interleukin-6 and matrix metalloproteinase-9 in acute low-pressure hypoxic rats,’ in The 16th National Conference on Hyperbaric Oxygen of the Chinese Medical Association, Qingdao, Shandong, China, 9.
Luks A. M., Auerbach P. S., Freer L., Grissom C. K., Keyes L. E., Mcintosh S. E., et al. (2019). Wilderness medical society clinical practice guidelines for the prevention and treatment of acute altitude illness: 2019 update. Wilderness Environ. Med. 30, S3–S18. doi:10.1016/j.wem.2019.04.006
Luks A. M. (2015). Physiology in medicine: A physiologic approach to prevention and treatment of acute high-altitude illnesses. J. Appl. Physiol. 118, 509–519. doi:10.1152/japplphysiol.00955.2014
Luks A. M., Swenson E. R., Bartsch P. (2017). Acute high-altitude sickness. Eur. Respir. Rev. 26, 160096. doi:10.1183/16000617.0096-2016
Ma G., Zhang X., Cui J., Li B., Jin X., Li S., et al. (2008). Effectiveness of hyperbaric oxygen in preventing acute moutain sickness. J. High Alt. Med. 18, 17–18. doi:10.3969/j.issn.1007-3809.2008.01.007
Ma G., Wang W., Cui J., Zhang X., Li B., Ha Z., et al. (2008). Effect of Hyperbaric oxygen preconditioning on anti-hypoxia. Clin. J. Med. Offic. 36, 105–106.
Ma Y., Ma G., Wang D., Gao L., Wang H., Ha Z., et al. (2008). Effects of hyperbaric oxygen on brain-body physiological functions of immigrators at high altitudes. Chin. J. Naut. Med & Hyperbar. Med 15, 81–83. doi:10.3760/cma.j.issn.1009-6906.2008.02.005
Ma Y. (2007). Hyperbaric oxygen preconditioning improves sleep hypoxemia and alertness in highland migrants. Chin. Behav. Med. Sci. 16, 644. doi:10.3760/cma.j.issn.1674-6554.2007.07.028
Maloney J. P., Broeckel U. (2005). Epidemiology, risk factors, and genetics of high-altitude-related pulmonary disease. Clin. Chest Med. 26, 395–404. doi:10.1016/j.ccm.2005.05.002
Meier D., Collet T. H., Locatelli I., Cornuz J., Kayser B., Simel D. L., et al. (2017). Does this patient have Acute Mountain Sickness?: The rational clinical examination systematic review. Jama 318, 1810–1819. doi:10.1001/jama.2017.16192
Mulchrone A., Moulton H., Eldridge M. W., Chesler N. C. (2020). Susceptibility to high-altitude pulmonary edema is associated with increased pulmonary arterial stiffness during exercise. J. Appl. Physiol. 128, 514–522. doi:10.1152/japplphysiol.00153.2019
Pan S. Y., Pan X. W., Yang Y. A. N. G, C., Li M. X., Zhang Y., Meng X. E., et al. (2008). Effects of early hyperbaric oxygen and hypobaric intervention on the levels of calcitonin gene related protein and endothelin in rats in hypobaric environment. Chin J Naut Med Hyperbar Med 15, 325–328. doi:10.3760/cma.j.issn.1009-6906.2008.06.002
Pan Y., Zhang Y., Yuan J., Ma X., Zhao Y., Li Y., et al. (2020). Tetrahydrocurcumin mitigates acute hypobaric hypoxia-induced cerebral oedema and inflammation through the NF-κB/VEGF/MMP-9 pathway. Phytother. Res. 34, 2963–2977. doi:10.1002/ptr.6724
Qi D., Yin X., Luran L. (2020). Neuroprotective mechanism of hyperbaric oxygen pretreatment and its application in cerebral infarction (in Chinese). BME Clin Med 24, 787–790. doi:10.13339/j.cnki.sglc.20201023.014
Qi Z., Gao C. J., Wang Y. B., Ma X. M., Zhao L., Liu F. J., et al. (2013). Effects of hyperbaric oxygen preconditioning on ischemia-reperfusion inflammation and skin flap survival. Chin. Med. J. Engl. 126, 3904–3909. doi:10.3760/cma.j.issn.0366-6999.20121165
Ritchie N., Baggott A., Todd W. (2012). Acetazolamide for the prevention of Acute Mountain sickness-A systematic review and meta-analysis. J. travel Med. 19, 298–307. doi:10.1111/j.1708-8305.2012.00629.x
Roach R. C., Hackett P. H., Oelz O., Bartsch P., Luks A. M., Macinnis M. J., et al. (2018). The 2018 Lake Louise Acute Mountain Sickness Score. High. Alt. Med. Biol. 19, 4–6. doi:10.1089/ham.2017.0164
Salhanick S. D., Belikoff B., Orlow D., Holt D., Reenstra W., Buras J. A. (2006). Hyperbaric oxygen reduces acetaminophen toxicity and increases HIF-1alpha expression. Acad. Emerg. Med. 13, 707–714. doi:10.1197/j.aem.2006.01.029
Salmón-González Z., Anchuelo J., Borregán J. C., Del Real A., Sañudo C., García-Unzueta M. T., et al. (2021). Hyperbaric oxygen therapy does not have a negative impact on bone signaling pathways in humans. Healthc. (Basel) 9, 1714. doi:10.3390/healthcare9121714
Semenza G. L. (1998). Hypoxia-inducible factor 1: Master regulator of O2 homeostasis. Curr. Opin. Genet. Dev. 8, 588–594. doi:10.1016/S0959-437X(98)80016-6
Shi Lu L. Y., Zhang Yanmeng, Jiang Xi, Enzhi Feng, Wang Yongcun, Duojie Gengsong, et al. (2015). Effects of hyperbaric oxygen preconditioning on human energy metabolism under high altitude hypoxia. Chin J Naut Med Hyperbar Med 22, 96–99. doi:10.3760/cma.j.issn.1009-6906.2015.02.003
Tsai M. C., Lin H. J., Lin M. T., Niu K. C., Chang C. P., Tsao T. C. (2014). High-altitude pulmonary edema can be prevented by heat shock protein 70-mediated hyperbaric oxygen preconditioning. J. Trauma Acute Care Surg. 77, 585–591. doi:10.1097/TA.0000000000000408
Turner R. E. F., Gatterer H., Falla M., Lawley J. S. (2021). High-altitude cerebral edema: Its own entity or end-stage acute mountain sickness? J. Appl. Physiol. 131, 313–325. doi:10.1152/japplphysiol.00861.2019
Wang H., Jin X., Liu N., Li S., Cui J., Gao L., et al. (2008). The effect of hyperbaric oxygen preconditioning in preventing acute mountain sickness. J. High Alt. Med. 18, 21–22. doi:10.3969/j.issn.1007-3809.2008.01.009
Wang H. Y., Cui J. H., Ma Y., Li B., Ma G. Q., Zhang X. Z., et al. (2009). Effects of hyperbaric oxygen on the mental and physical performance of immigrants from flatlands to highlands. Chin J Naut Med Hyperbar Med 16, 39–41. doi:10.3760/cma.j.issn.1009-6906.2009.01.015
Wang X., Hou Y., Li Q., Li X., Wang W., Ai X., et al. (2019). Rhodiola crenulata attenuates apoptosis and mitochondrial energy metabolism disorder in rats with hypobaric hypoxia-induced brain injury by regulating the HIF-1α/microRNA 210/ISCU1/2(COX10) signaling pathway. J. Ethnopharmacol. 241, 111801. doi:10.1016/j.jep.2019.03.028
Wang Y., Gao C.-J., Li Z., Liu F., Ma L., Zhang L., et al. (2012). Effects of hyperbaric oxygen preconditioning on the expressions of aquaporin 1 and aquaporin 5 in rats with acute high altitude pulmonary edema. Chin. J. Naut. Med. Hyperbaric Med. 19, 263–266, 279. doi:10.3760/cma.j.issn.1009-6906.2012.05.002
West J. B. (2012). High-altitude medicine. Am. J. Respir. Crit. Care Med. 186, 1229–1237. doi:10.1164/rccm.201207-1323CI
Xiao C. J., Hu J. J. (2017). The preventive effect of hyperbaric oxygen pretreatment on acute mountain sickness. J. Yangtze Univ. Sci. Ed. 14, 56–57. doi:10.3969/j.issn.1673-1409.2017.16.024
Yan X., Liu J., Zhu M., Liu L., Chen Y., Zhang Y., et al. (2021). Salidroside orchestrates metabolic reprogramming by regulating the Hif-1α signalling pathway in acute mountain sickness. Pharm. Biol. 59, 1540–1550. doi:10.1080/13880209.2021.1992449
Yang B., Sun Z. J., Cao F., Zhao H., Li C. W., Zhang J. (2015). Obesity is a risk factor for acute mountain sickness: A prospective study in tibet railway construction workers on Tibetan plateau. Eur. Rev. Med. Pharmacol. Sci. 19, 806–808. doi:10.5152/akd.2013.4585
Yang L., Shi L., Liu J., Mou J., Liu T., Li Y. (2014). Effects of hyperbaric oxygen pretreatment on human labor ability under acute hypoxia exposure. J. Clin. Exp. Med. 13, 1444–1448. doi:10.3969/j.issn.1671-4695.2014.17.021
Keywords: hyperbaric oxygenation, acute mountain sickness, high-altitude cerebral edema, preconditioning, high-altitude pulmonary edema
Citation: You J, Chen X, Zhou M, Ma H, Liu Q and Huang C (2023) Hyperbaric oxygen preconditioning for prevention of acute high-altitude diseases: Fact or fiction?. Front. Physiol. 14:1019103. doi: 10.3389/fphys.2023.1019103
Received: 14 August 2022; Accepted: 12 January 2023;
Published: 25 January 2023.
Edited by:
Matiram Pun, University of Calgary, CanadaReviewed by:
Sanjin Kovacevic, Faculty of Medicine, University of Belgrade, SerbiaSanjeeb Sudarshan Bhandari, UPMC Western Maryland Medical Center, United States
Copyright © 2023 You, Chen, Zhou, Ma, Liu and Huang. This is an open-access article distributed under the terms of the Creative Commons Attribution License (CC BY). The use, distribution or reproduction in other forums is permitted, provided the original author(s) and the copyright owner(s) are credited and that the original publication in this journal is cited, in accordance with accepted academic practice. No use, distribution or reproduction is permitted which does not comply with these terms.
*Correspondence: Cheng Huang, chenghuang_scu@163.com